Abstract
1. The electrical responses of single auditory nerve fibres or cochlear hair cells were recorded in the isolated half-head of the turtle Pseudemys scripta elegans. Responses to sound stimuli presented to the tympanum could be recorded for at least 4 hr after isolation.
2. Impulses were recorded extracellularly from single auditory nerve fibres. For tones of suprathreshold intensity the impulses occurred with a preferred phase relation (i.e. they were phase-locked) to the cycles of the sound stimulus. Nerve fibres had sharp tuning curves (Q10 db = 0·5-7·5) with single characteristic frequencies (c.f.) ranging from about 30 to 700 Hz. Best threshold sensitivities of fibres at their c.f. were in the region of 30-40 db sound pressure level with respect to 20 μPa.
3. Intracellular recordings were made from hair cells in the basilar papilla. Following injection of a fluorescent dye into a cell through the recording electrode, the dye was localized in a single hair cell in a transverse section of the cochlea.
4. Hair cells had resting potentials of about -50 mV, and, to low frequency tones, gave periodic responses graded with the intensity and frequency of the stimulus. Recordings were obtained from cells with characteristic frequencies between 70 and 670 Hz.
5. The voltage response to a pure tone at low sound pressure was sinusoidal for all frequencies of stimulation; at higher sound pressures a number of non-linearities were apparent in the response wave form. One of these was a steady depolarizing component, which, relative to the periodic component of the response, was most prominent at high frequencies.
6. The amplitude of the response evoked in a hair cell by a low intensity tone was linearly related to the sound pressure; for loud sounds, the response eventually reached a saturating amplitude, which in some cells was as great as 30-45 mV peak-to-peak.
7. The linear sensitivity of a hair cell is defined as the r.m.s. voltage for a linear response of the cell at its c.f. divided by the sound pressure at the tympanum. In the most sensitive cells this value was 30-90 mV/Pa.
8. If the frequency selectivity of a hair cell was expressed in terms of the sound pressure needed to produce a constant amplitude of response, the sharpness of this frequency selectivity was found to be virtually independent of the response criterion for responses between 1 and 10 mV; in the cells which gave the largest responses, the frequency selectivity expressed in this way was comparable to that of the nerve fibres. Cells with smaller maximum responses often had broader tuning curves.
9. Responses of hair cells to short low intensity tone bursts at the c.f. built up approximately exponentially during the tone, and decayed away exponentially when the tone was terminated. The terminal oscillations were at the c.f. of the cell, and independent of the frequency of stimulation.
10. From the time constant of the build up and decay of the linear response to a tone burst at the c.f. the sharpness of tuning of the cell was estimated and found to agree with that obtained from the responses of the cell to continuous tones. The most highly tuned cells had quality factors (Q3 db) in the range 5-10.
11. The c.f. of a hair cell was correlated with its position along the basilar membrane. Low frequency hair cells were located towards the apical or lagenar end and high frequency cells were found towards the basal or saccular end. On the assumption of an exponential distribution of c.f. with distance, each octave occupied about 94 μm along the membrane.
12. A hair cell's response to a click was a decaying oscillation at the characteristic frequency of the cell. From the initial polarity of the responses to condensation and rarefaction clicks it was concluded that the hair cell depolarized as a result of movements of the basilar membrane towards the scala vestibuli, and hyperpolarized for motion towards the scala tympani.
13. In the absence of deliberate sound stimulation, the hair cell voltage fluctuated continuously about its mean level. The principal frequency components in the noise were concentrated around the c.f. of the cell. The voltage noise in the hair cells showed no significant cross-correlation with sound pressure fluctuations at the tympanum.
Full text
PDF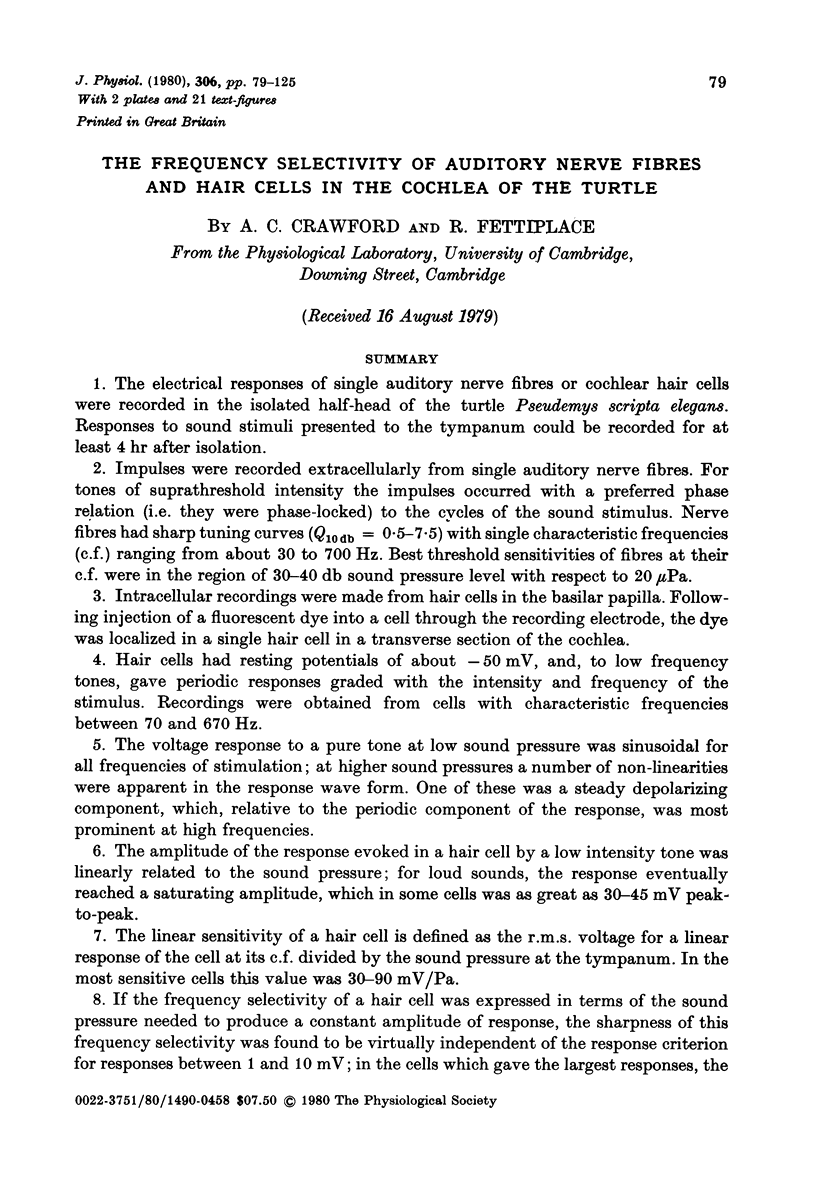
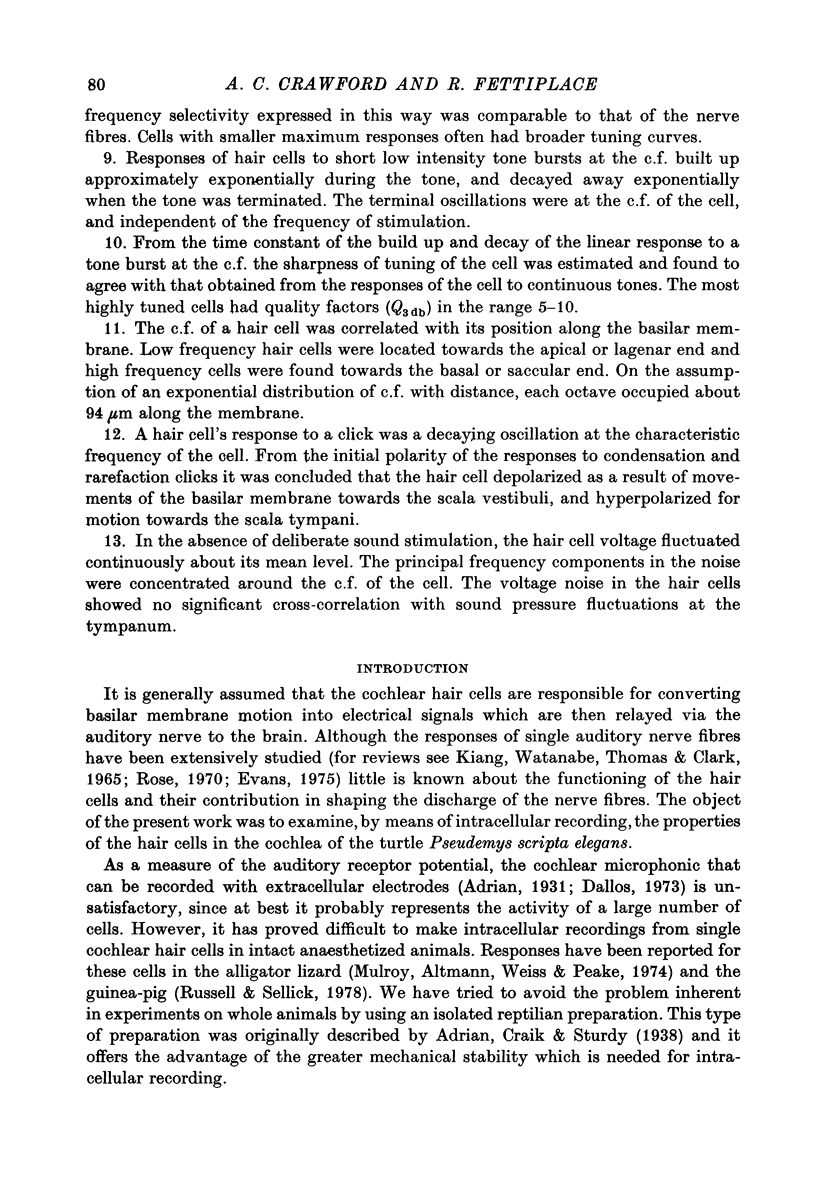
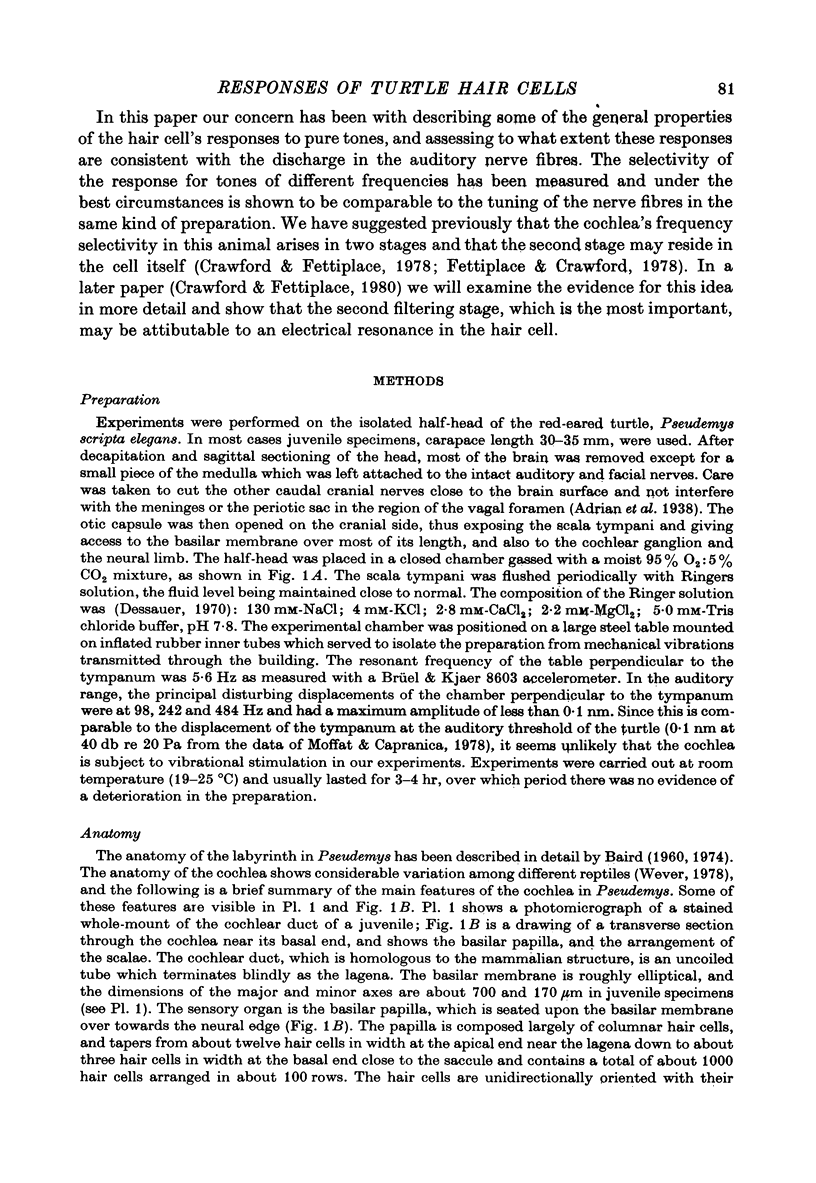
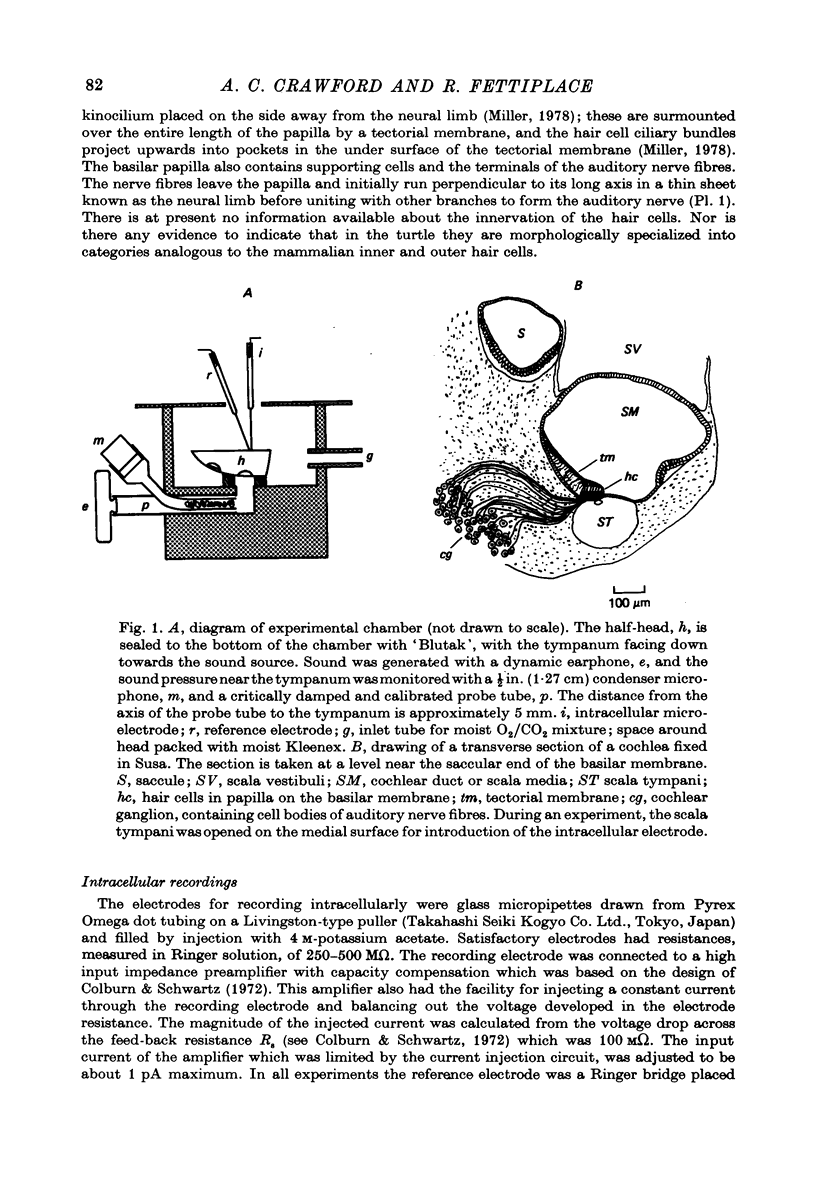
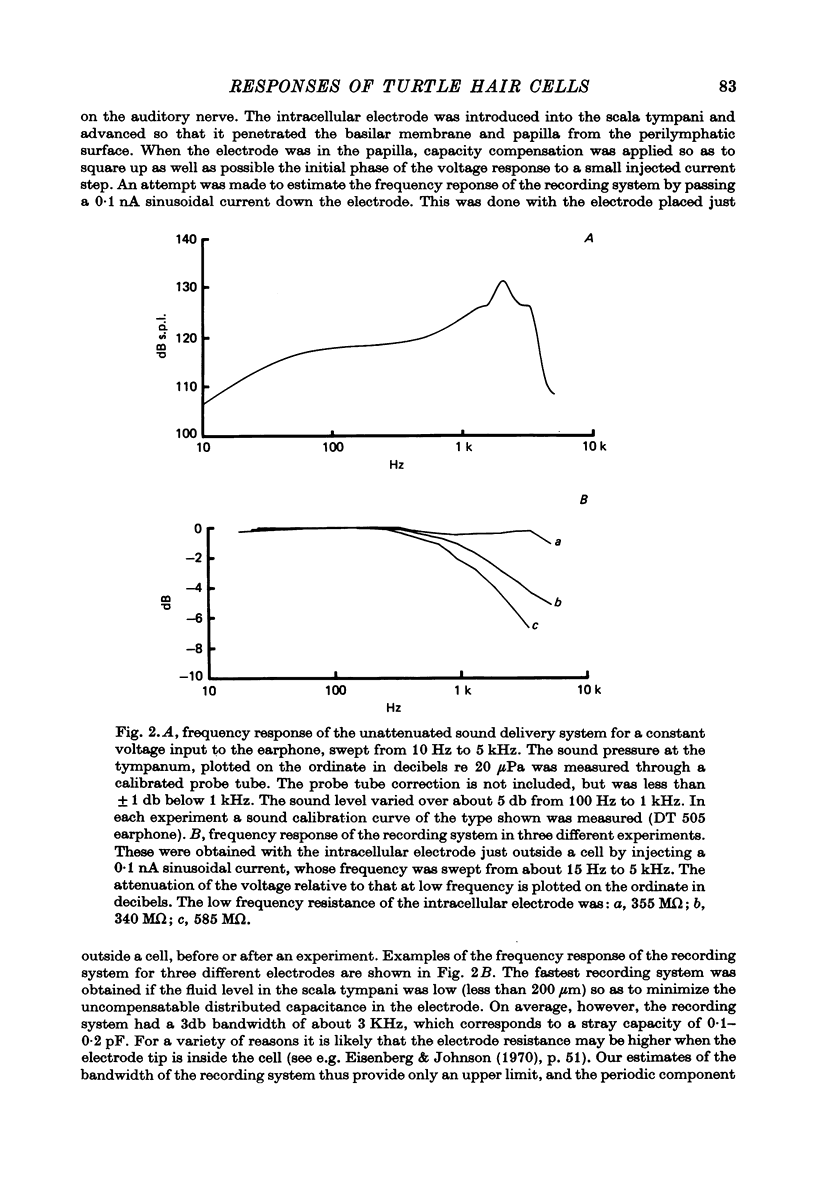
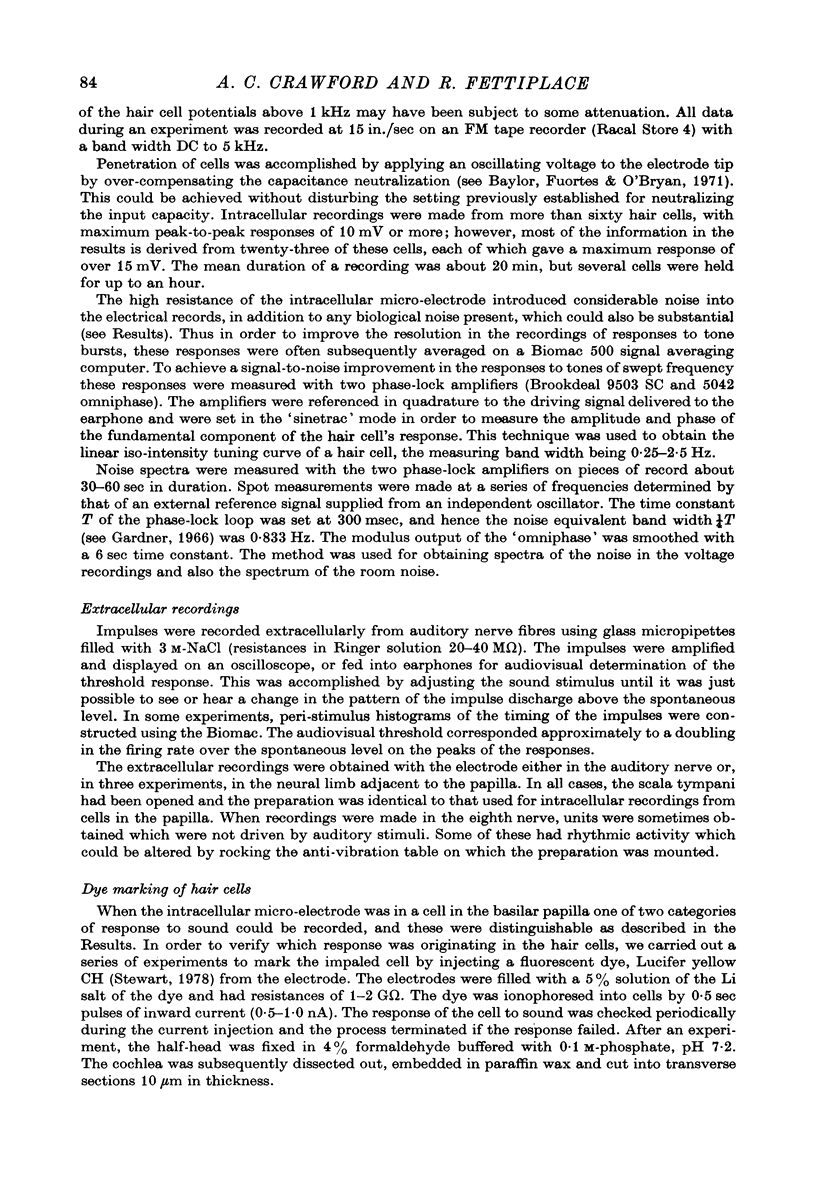
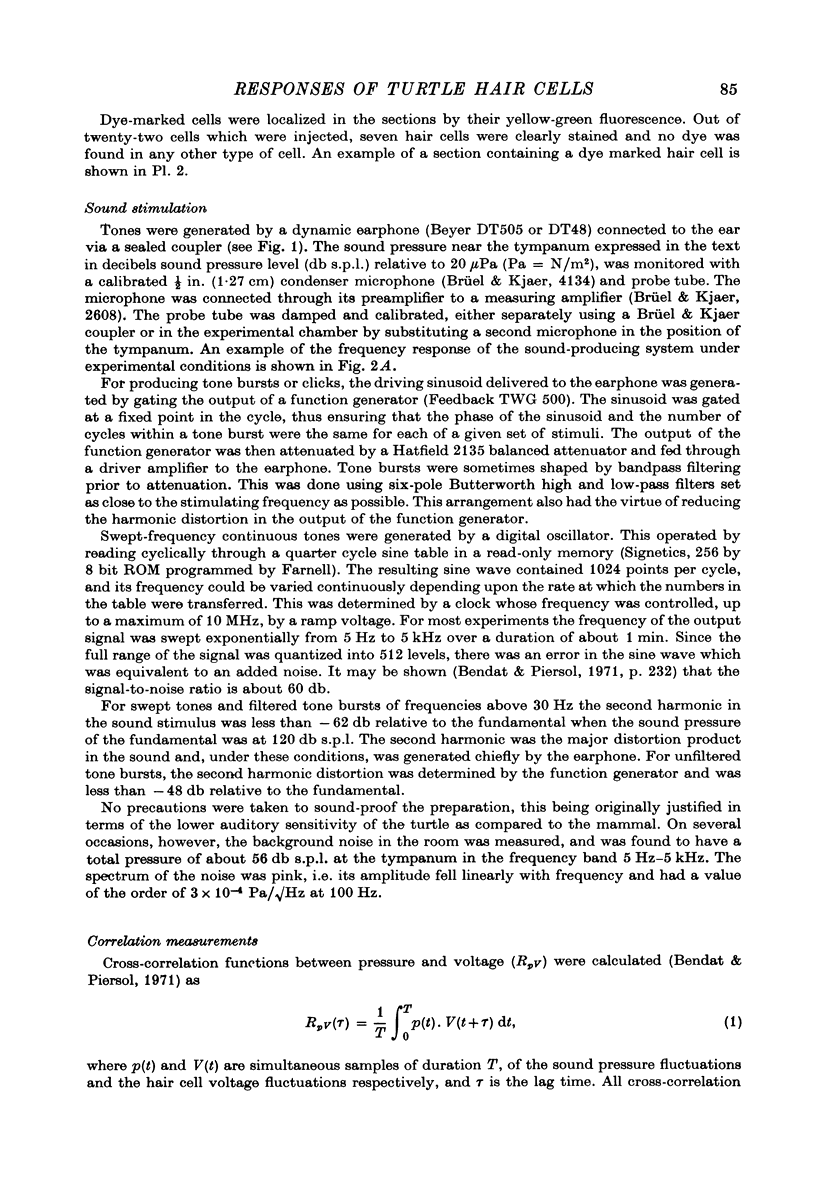
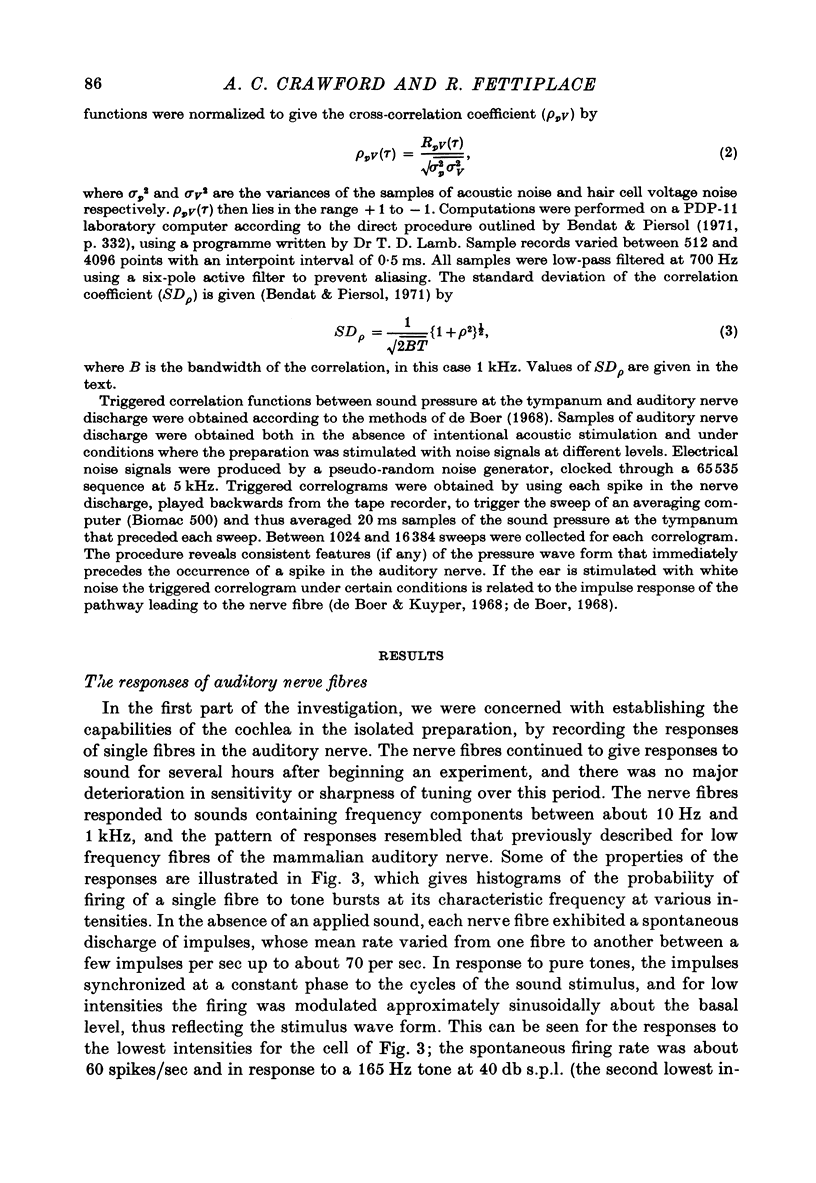
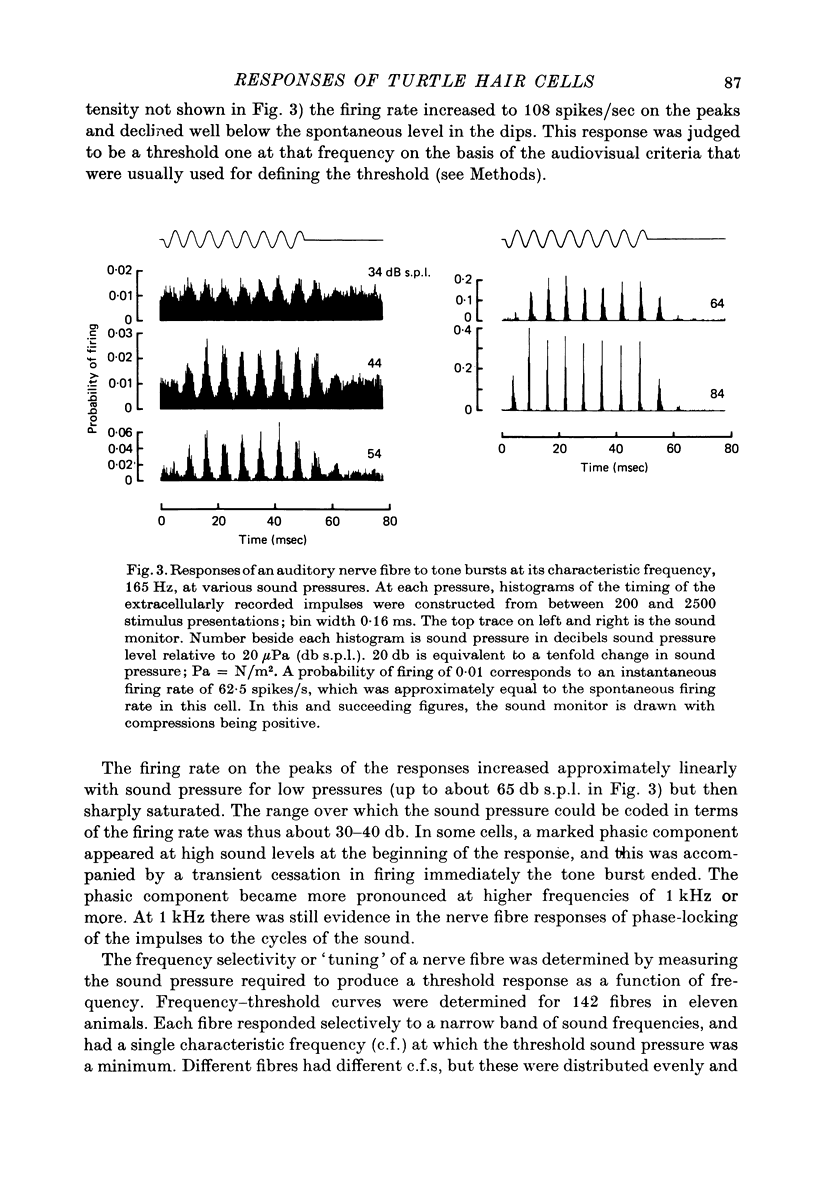
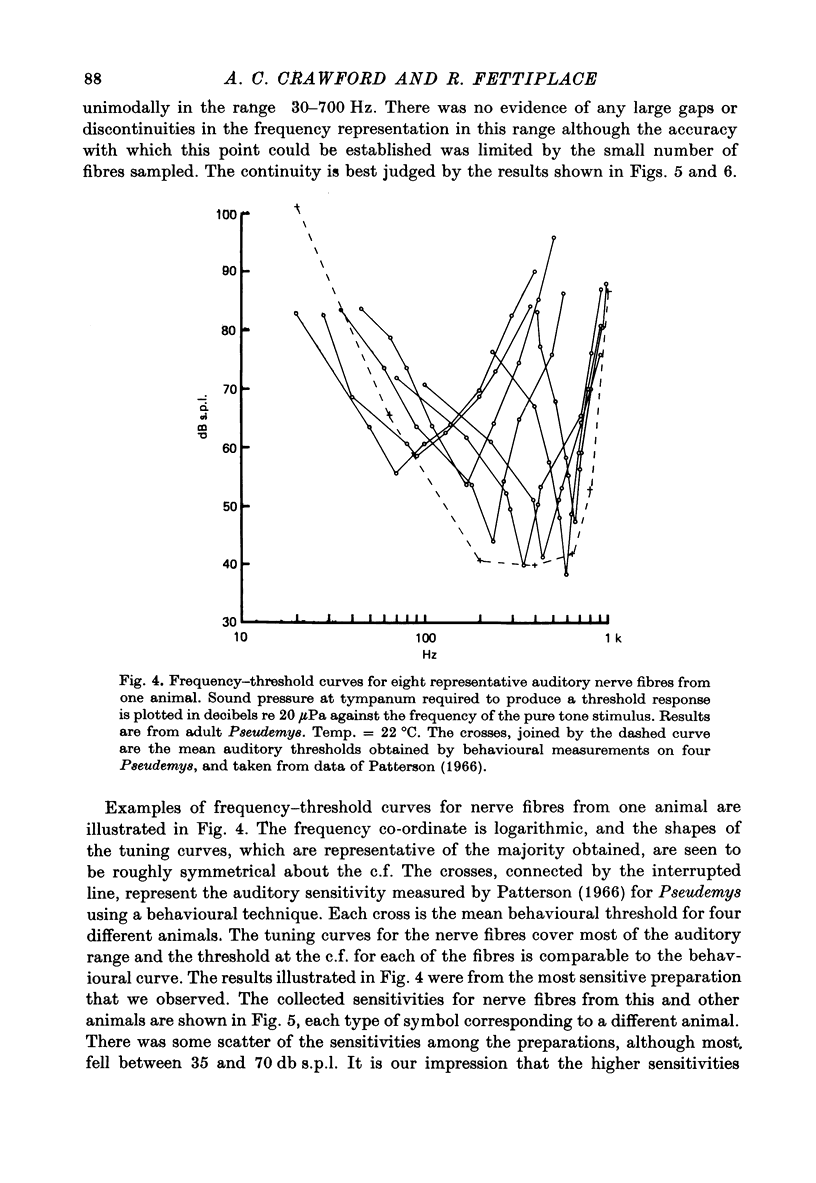
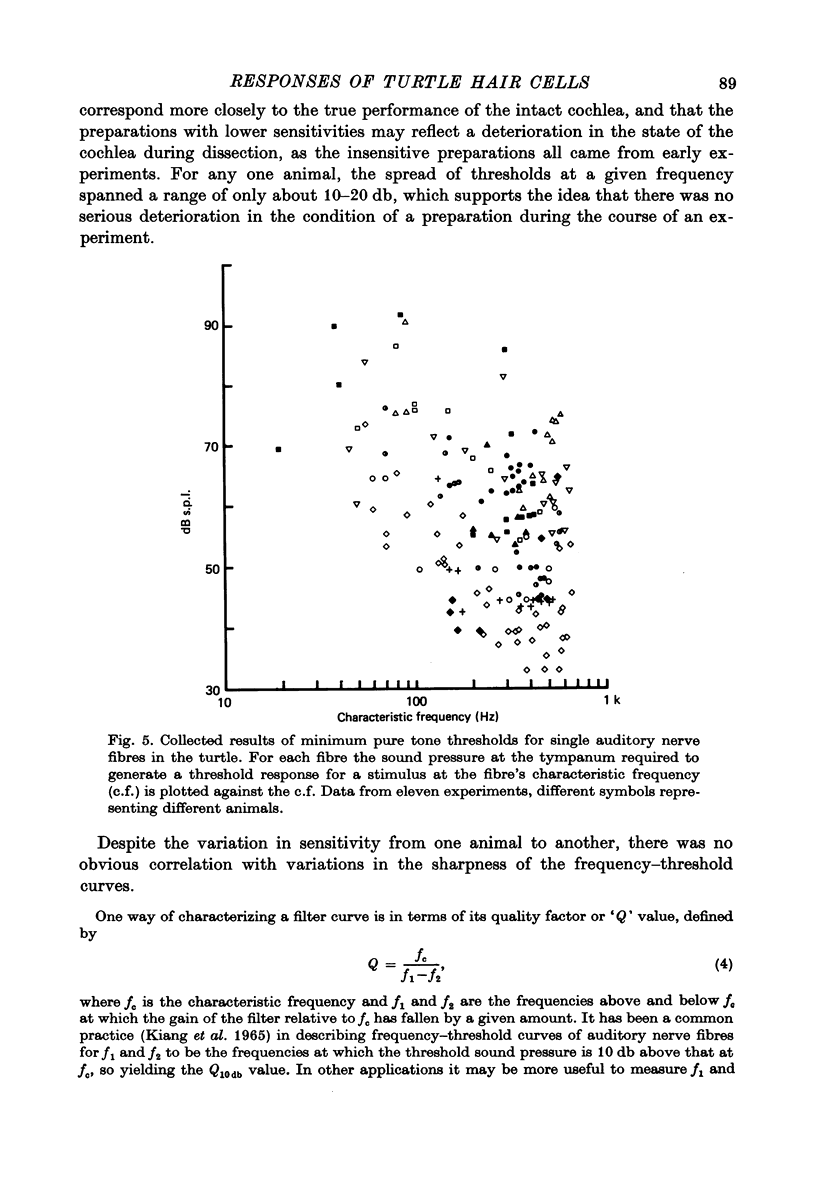
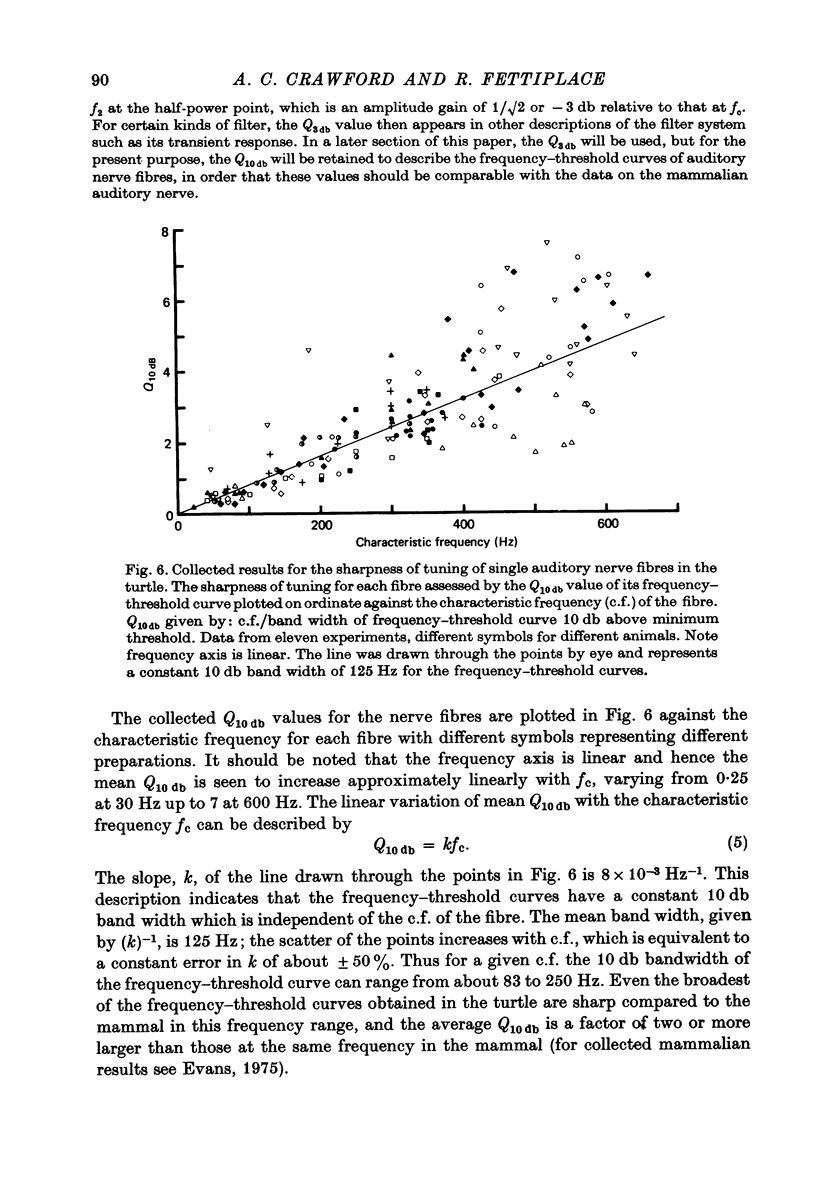
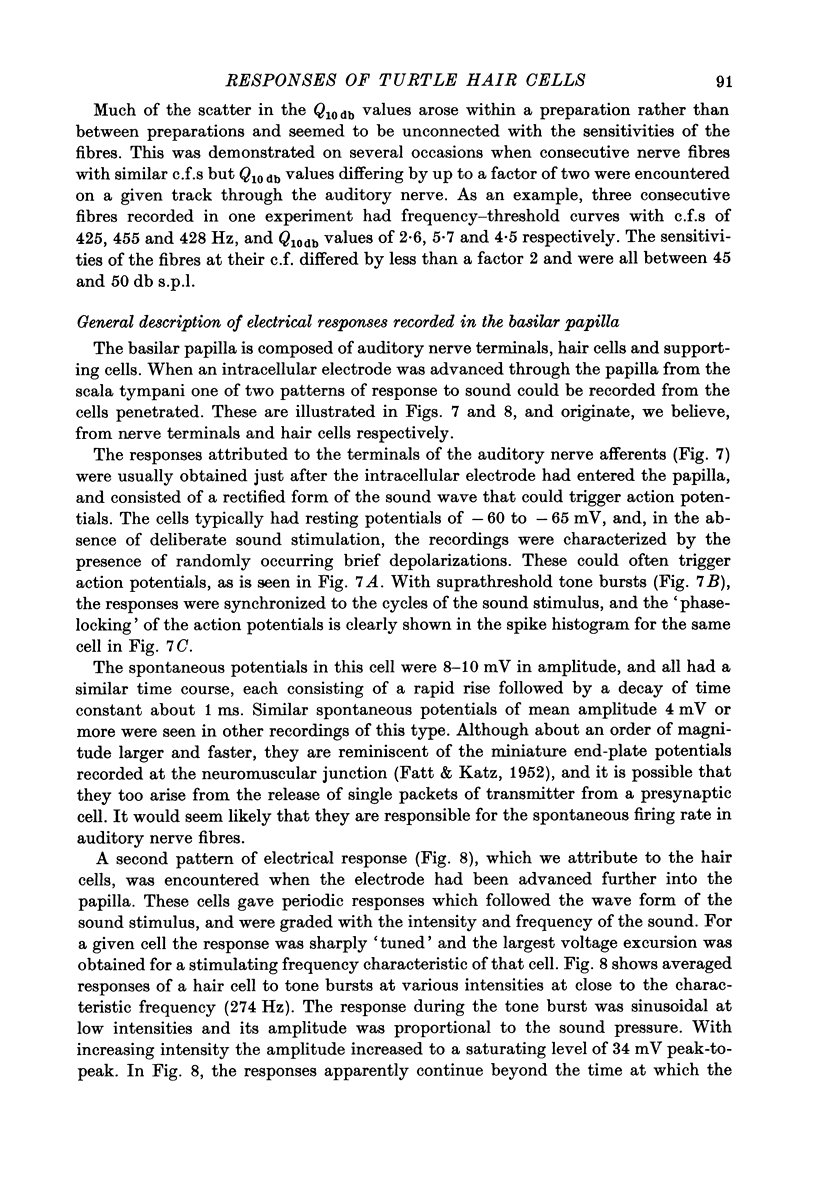
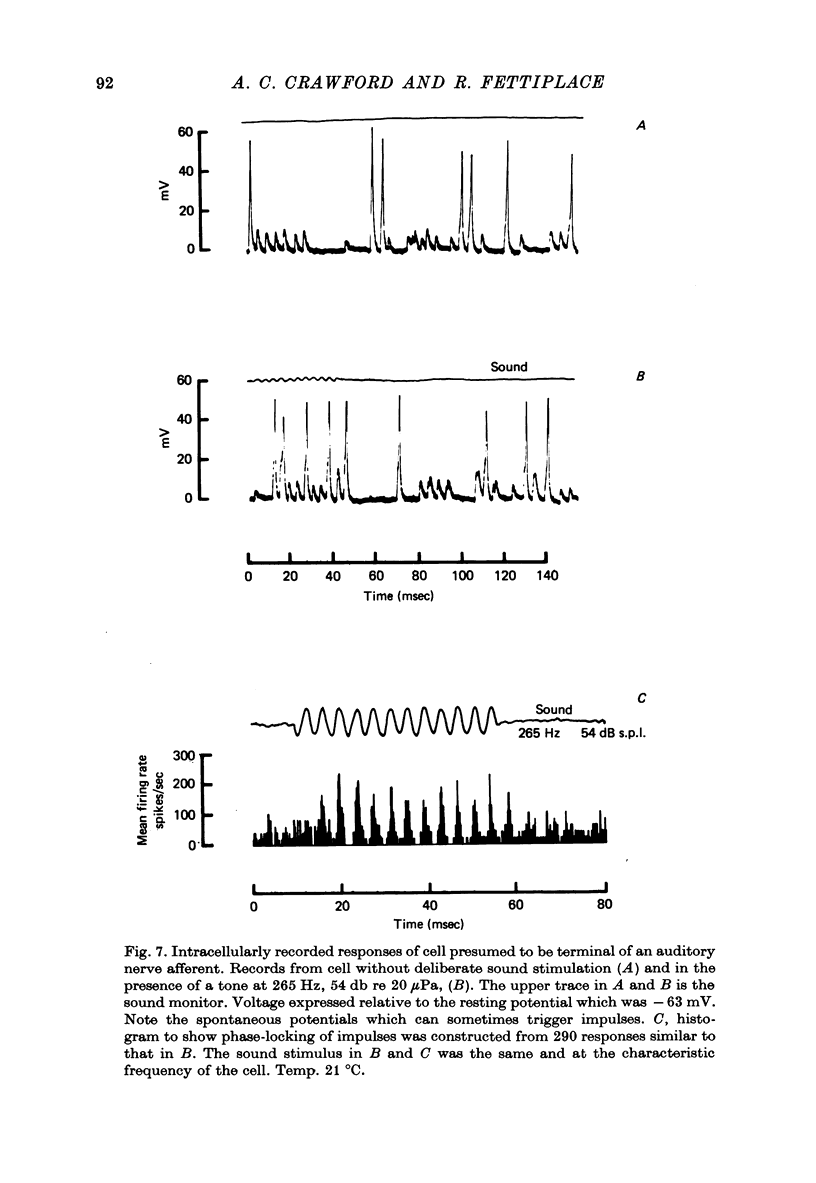
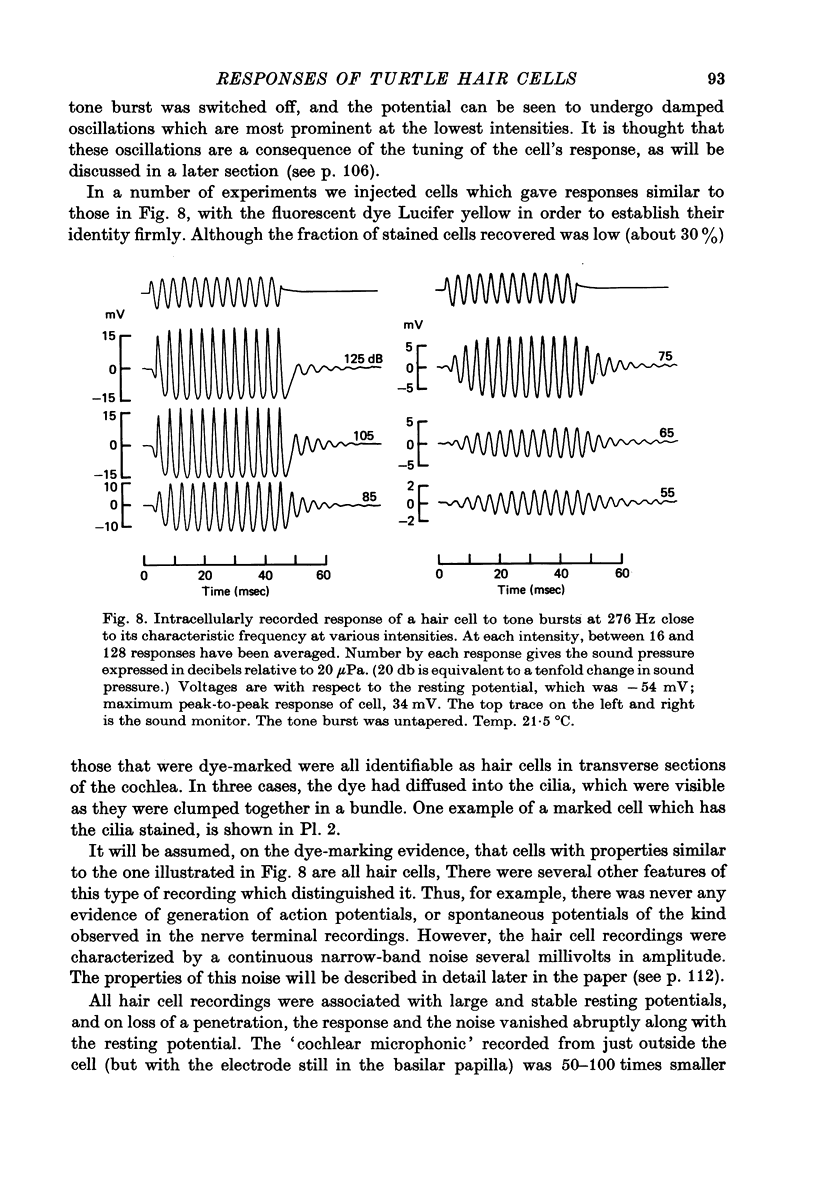
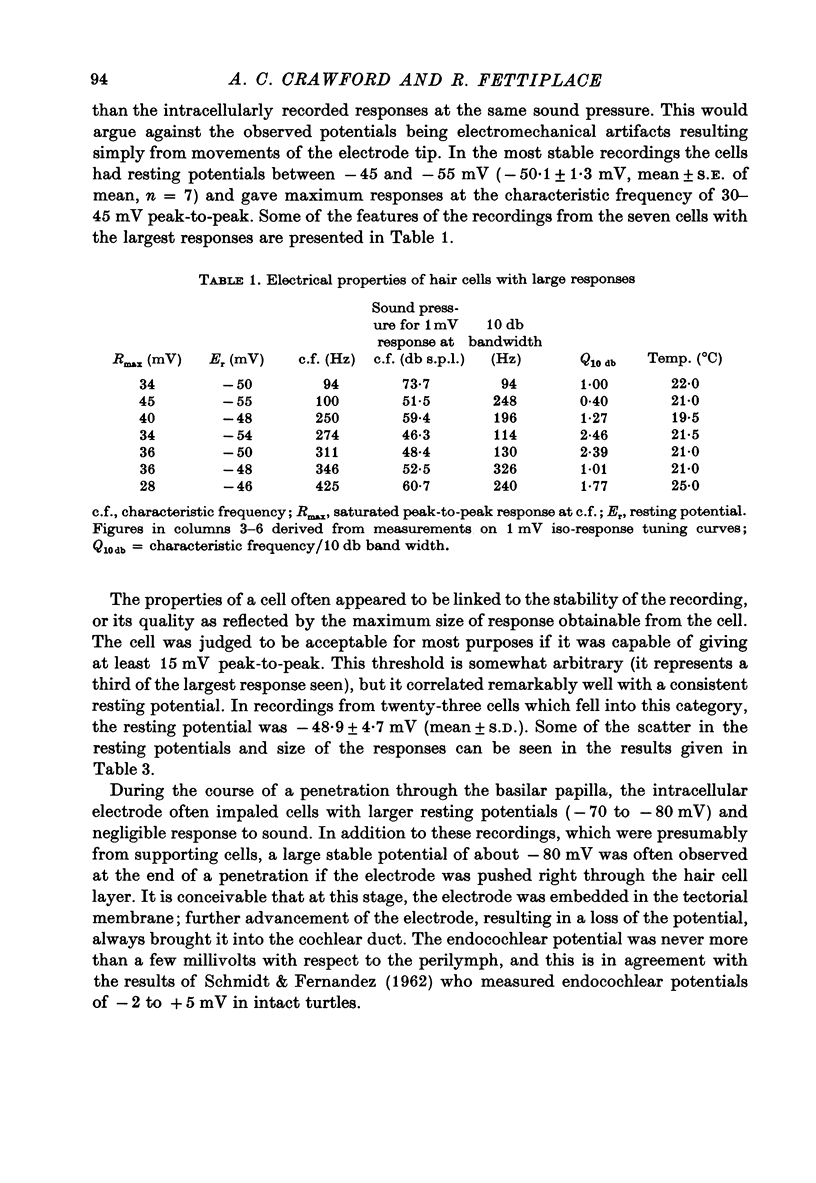
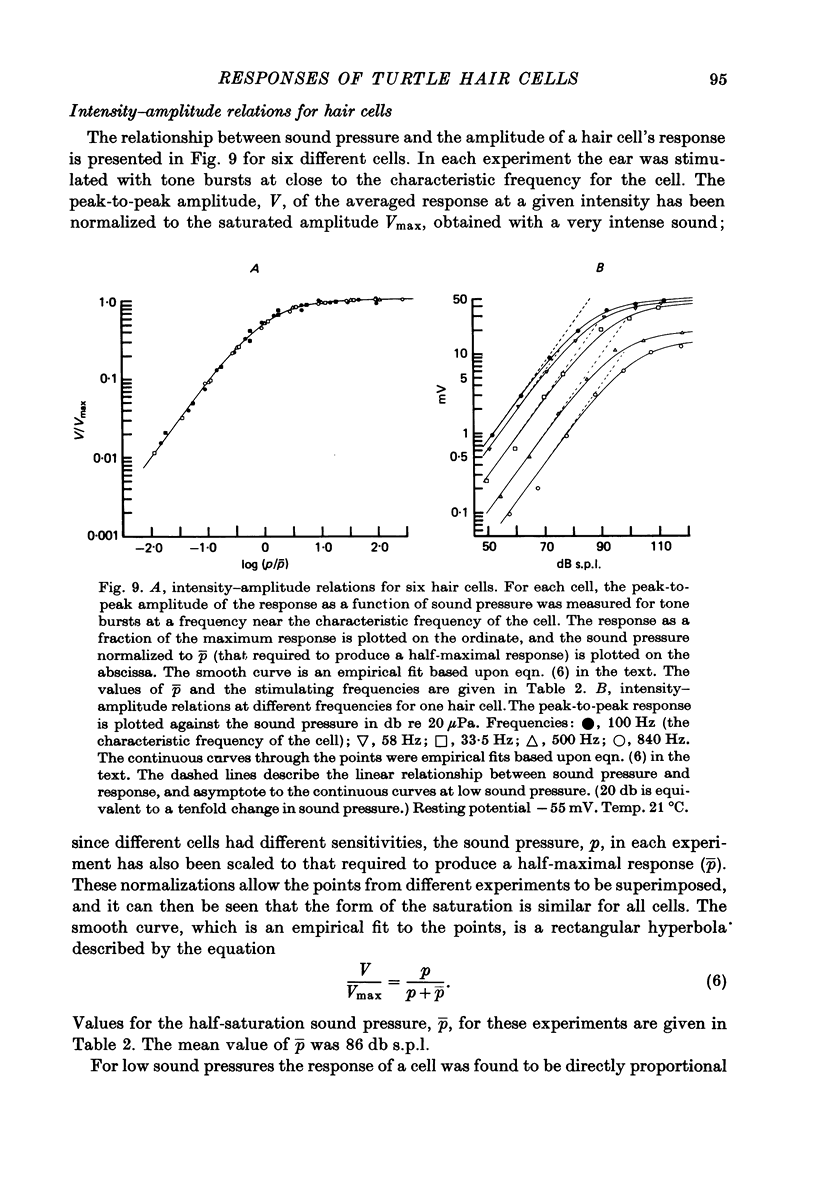
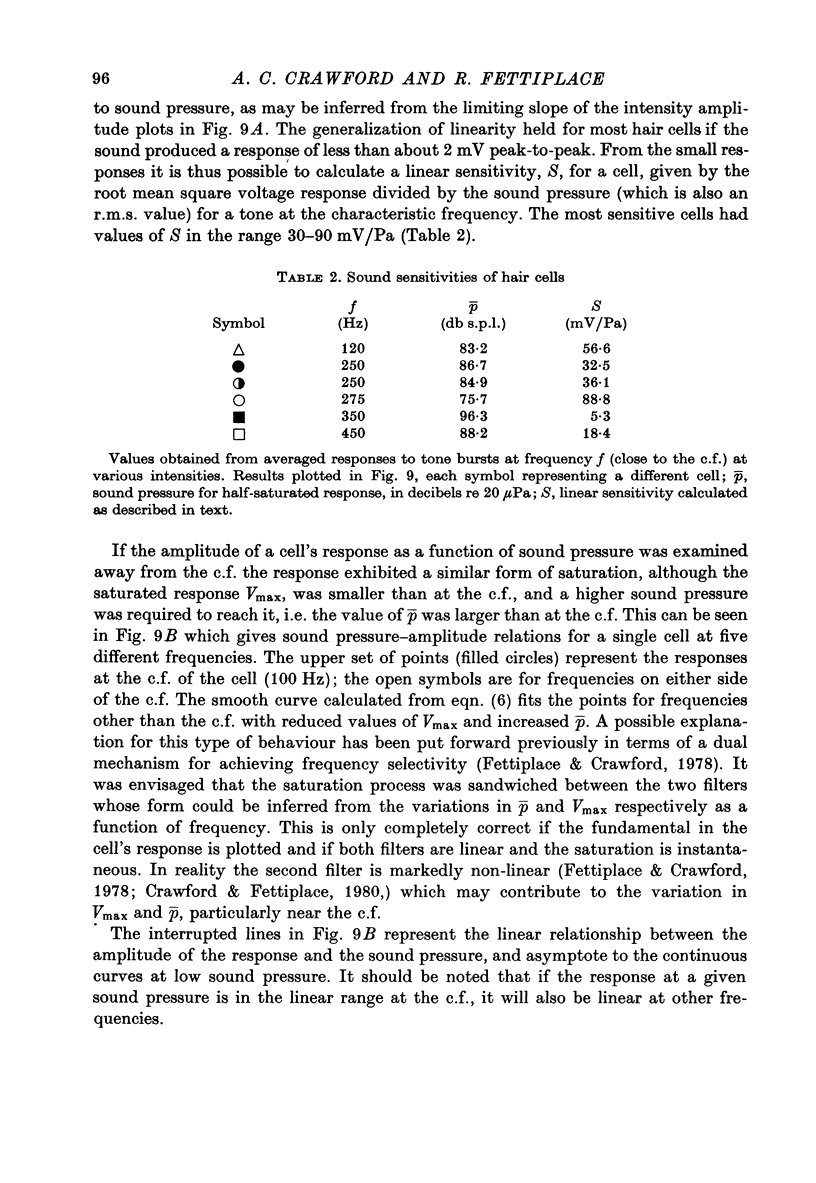
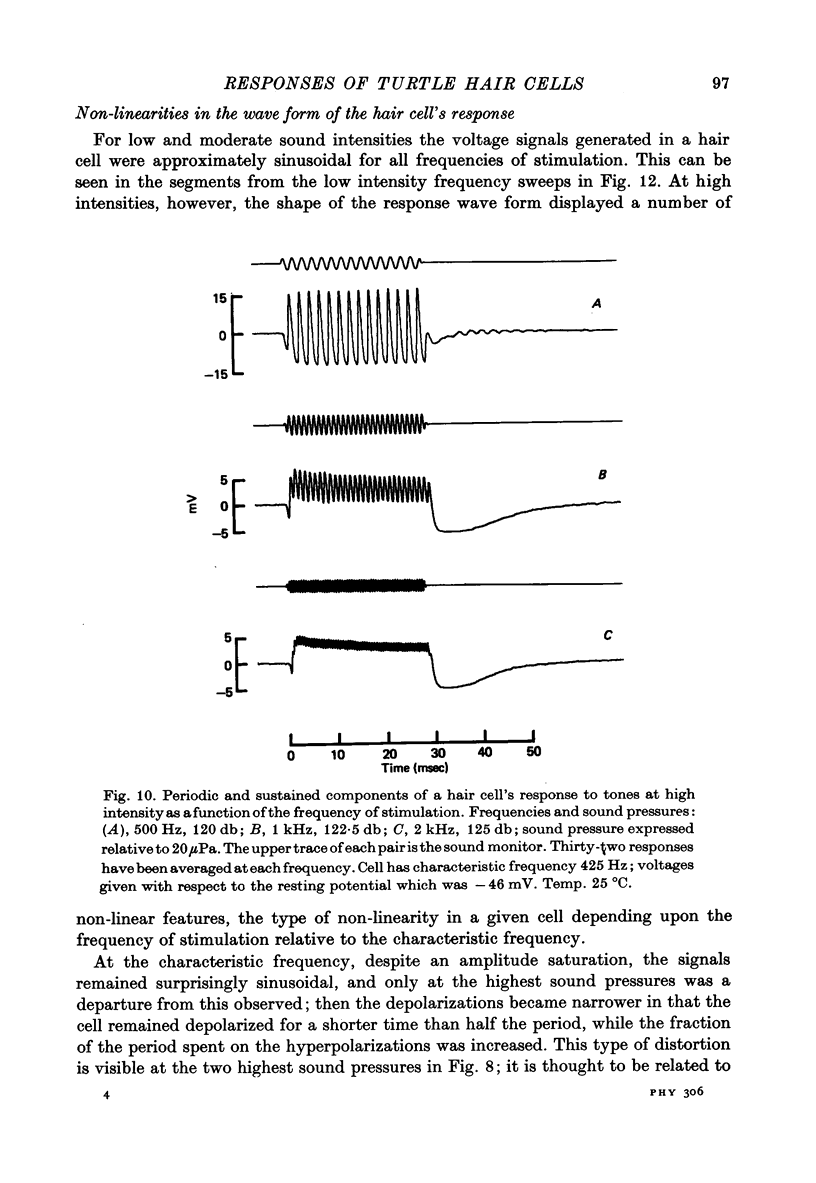
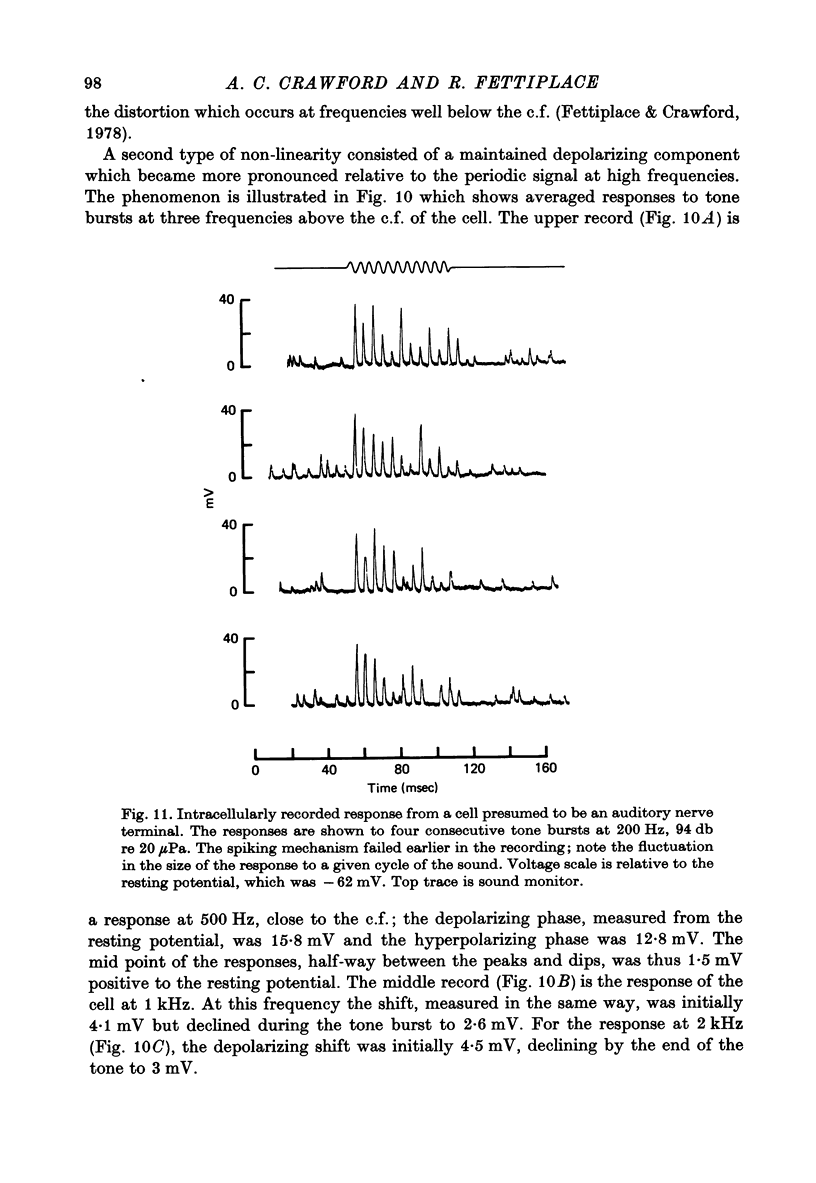
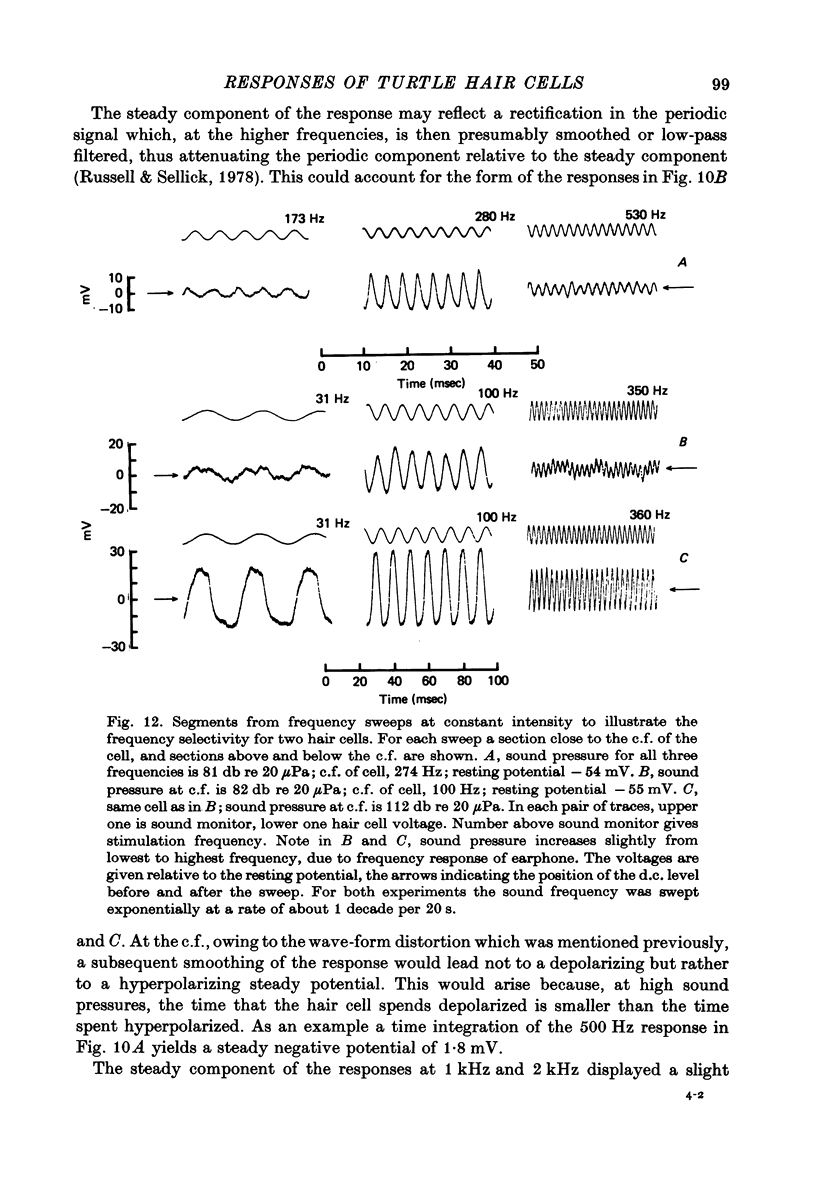
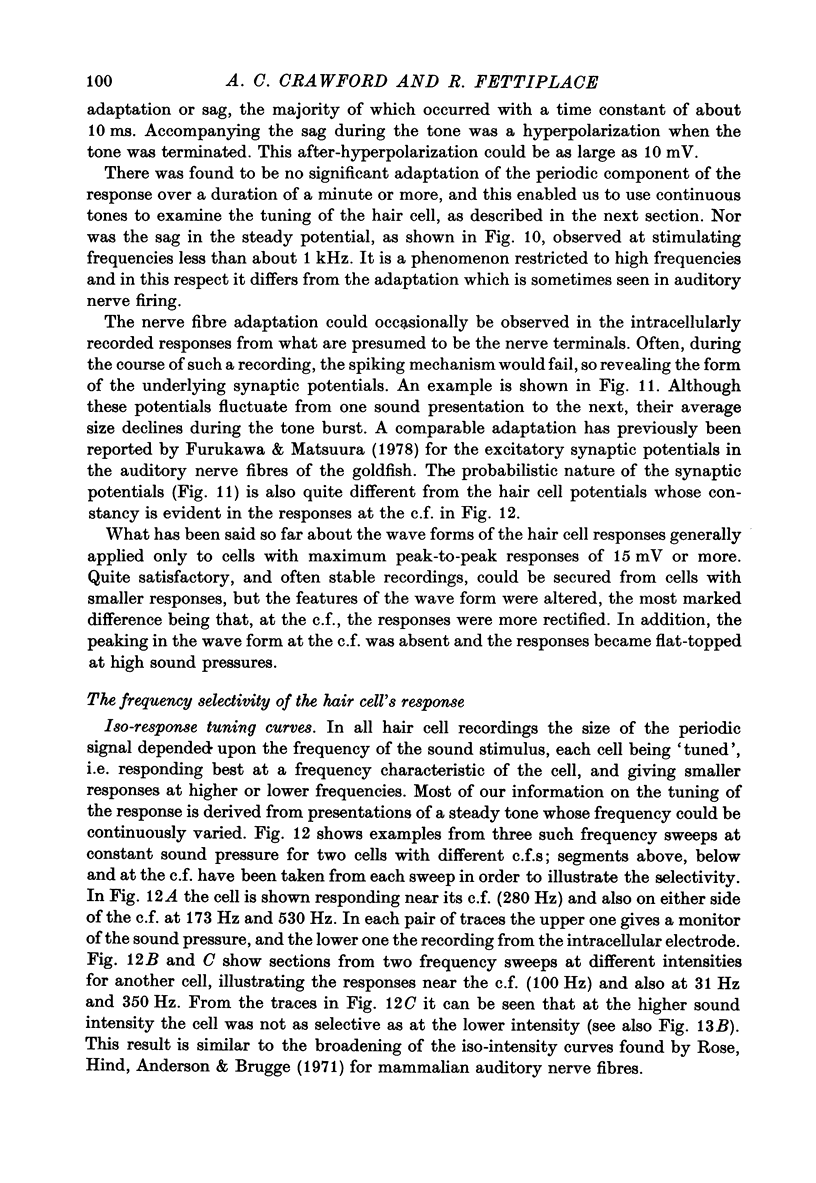
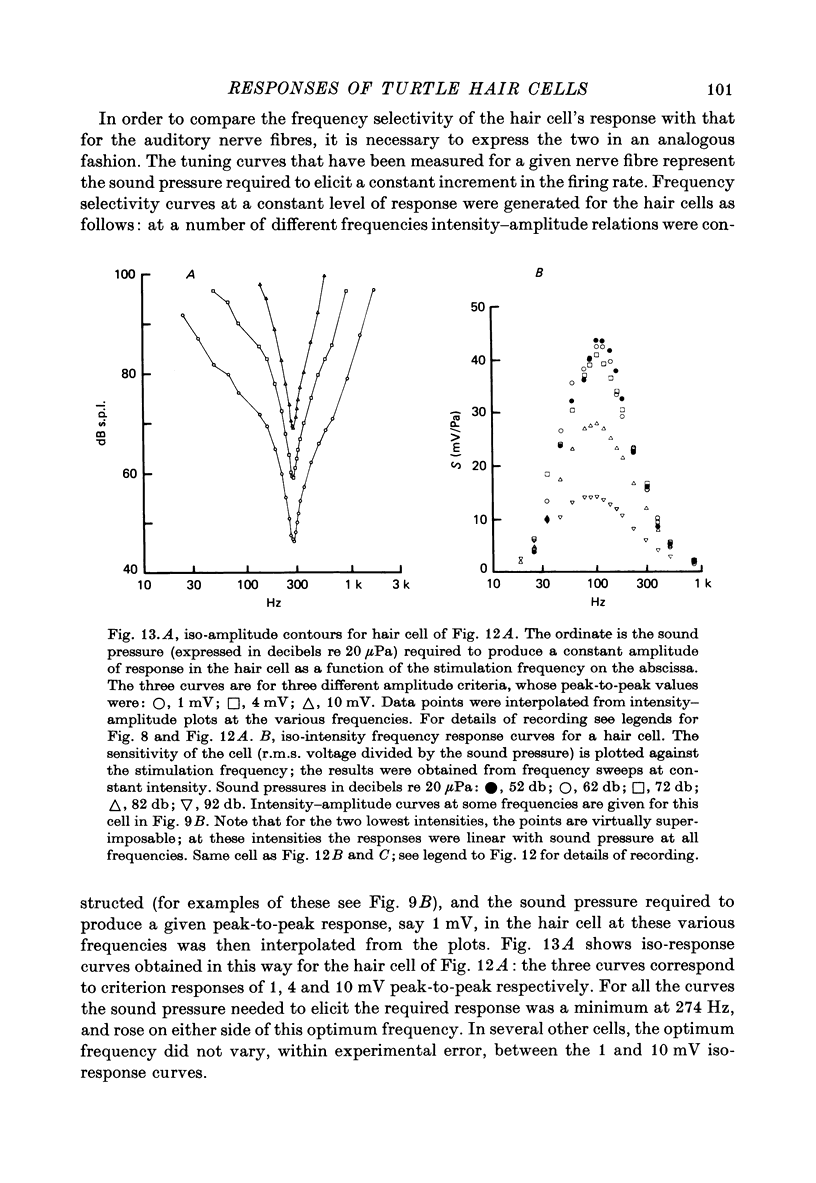
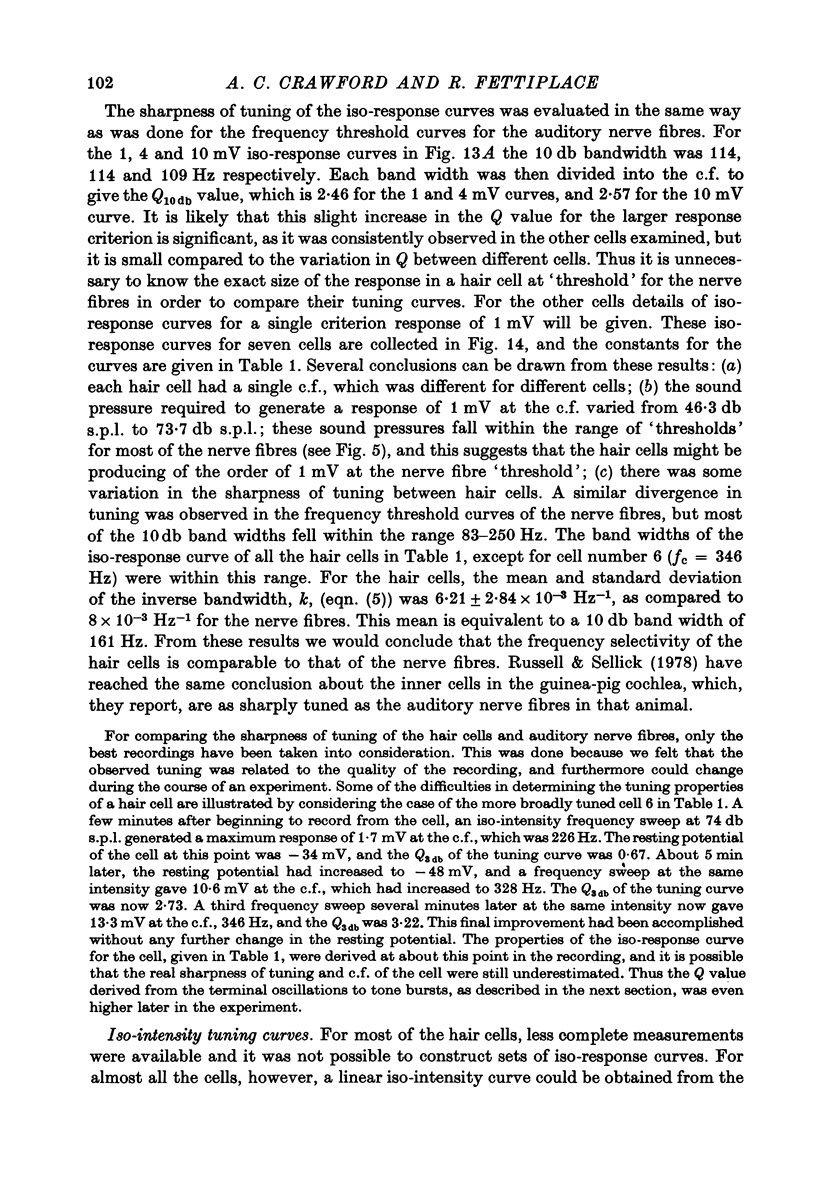
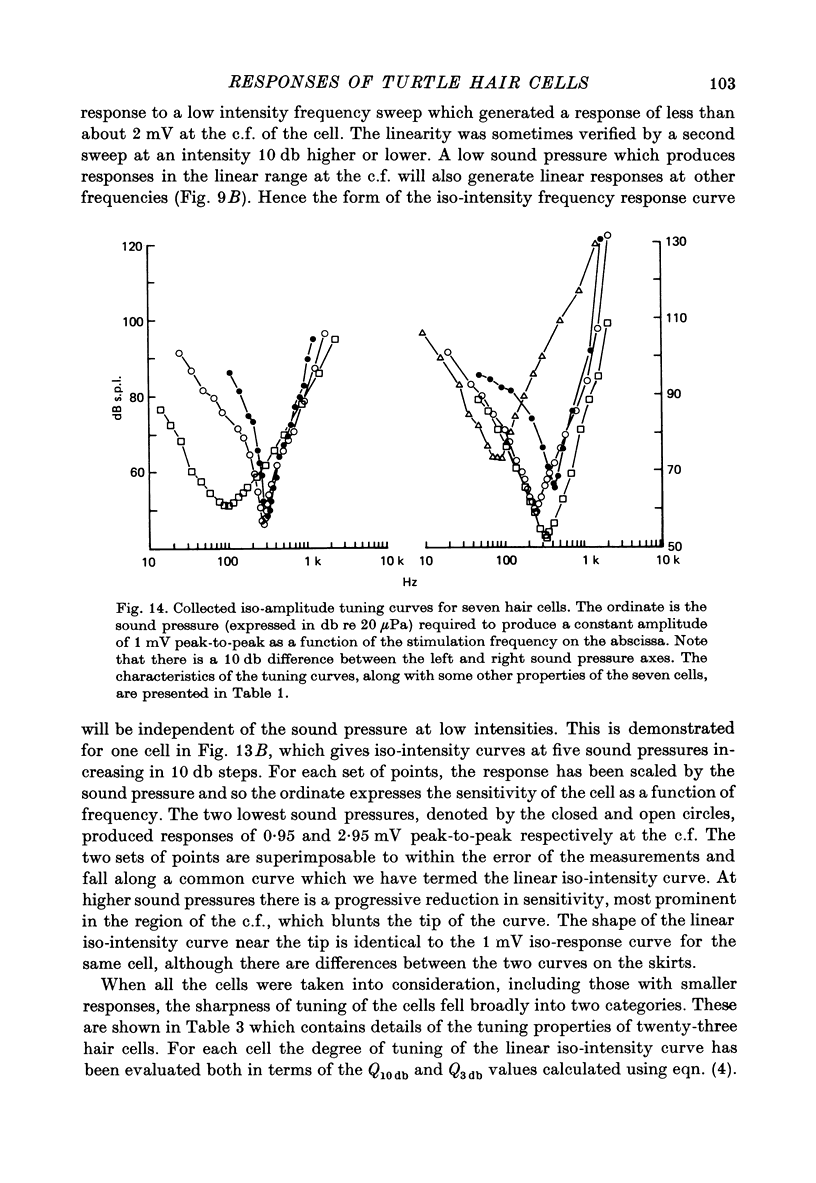
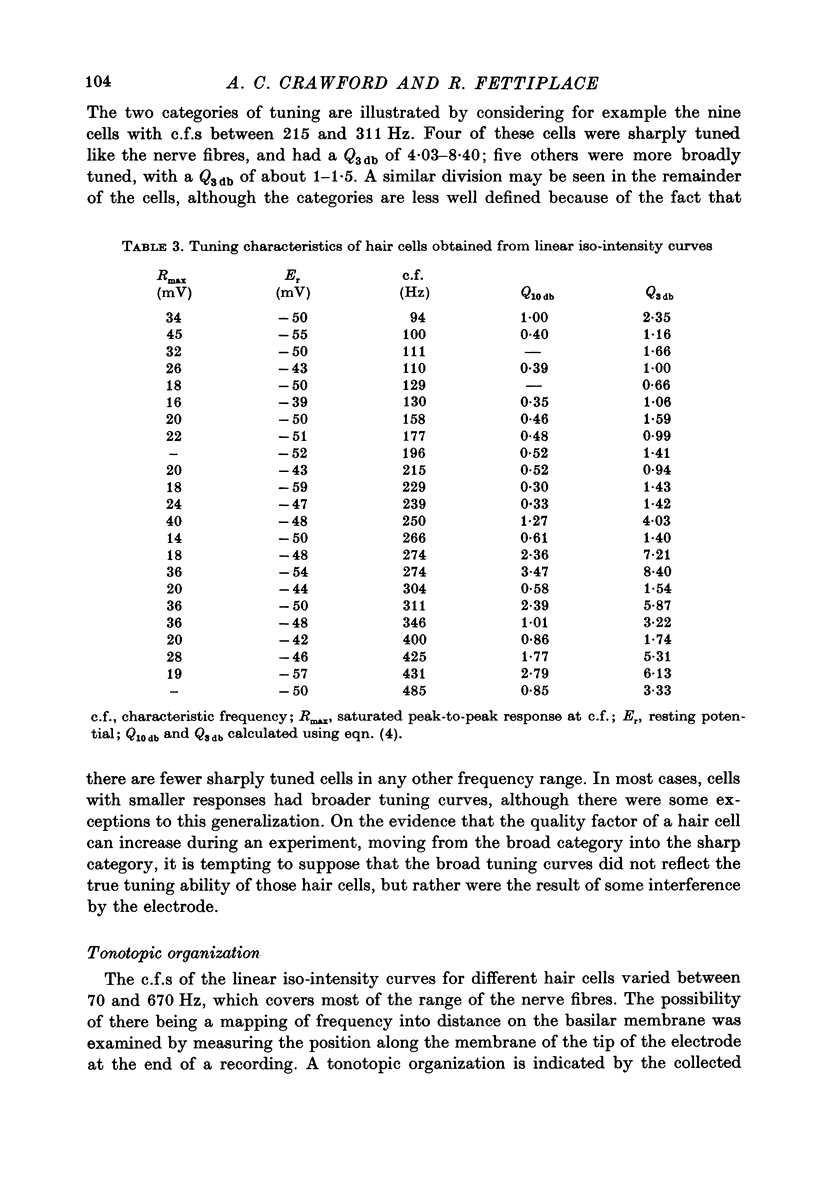
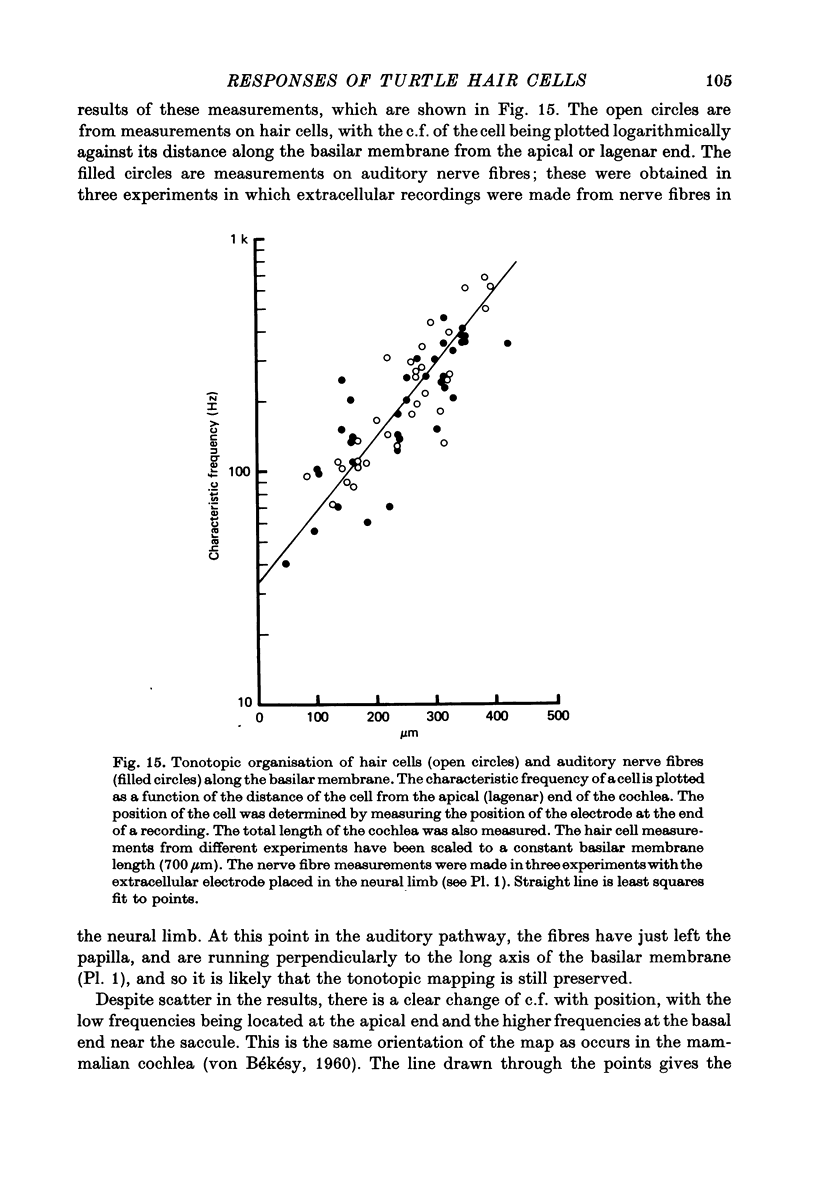
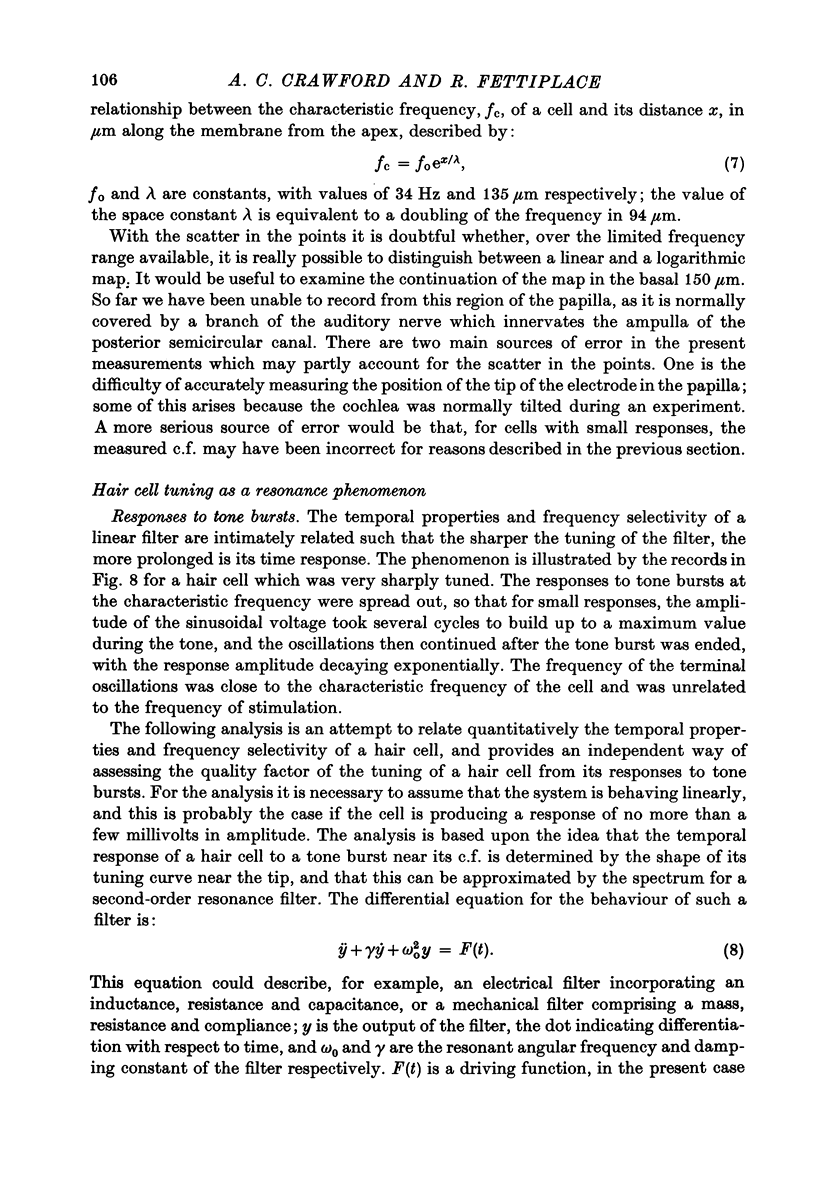
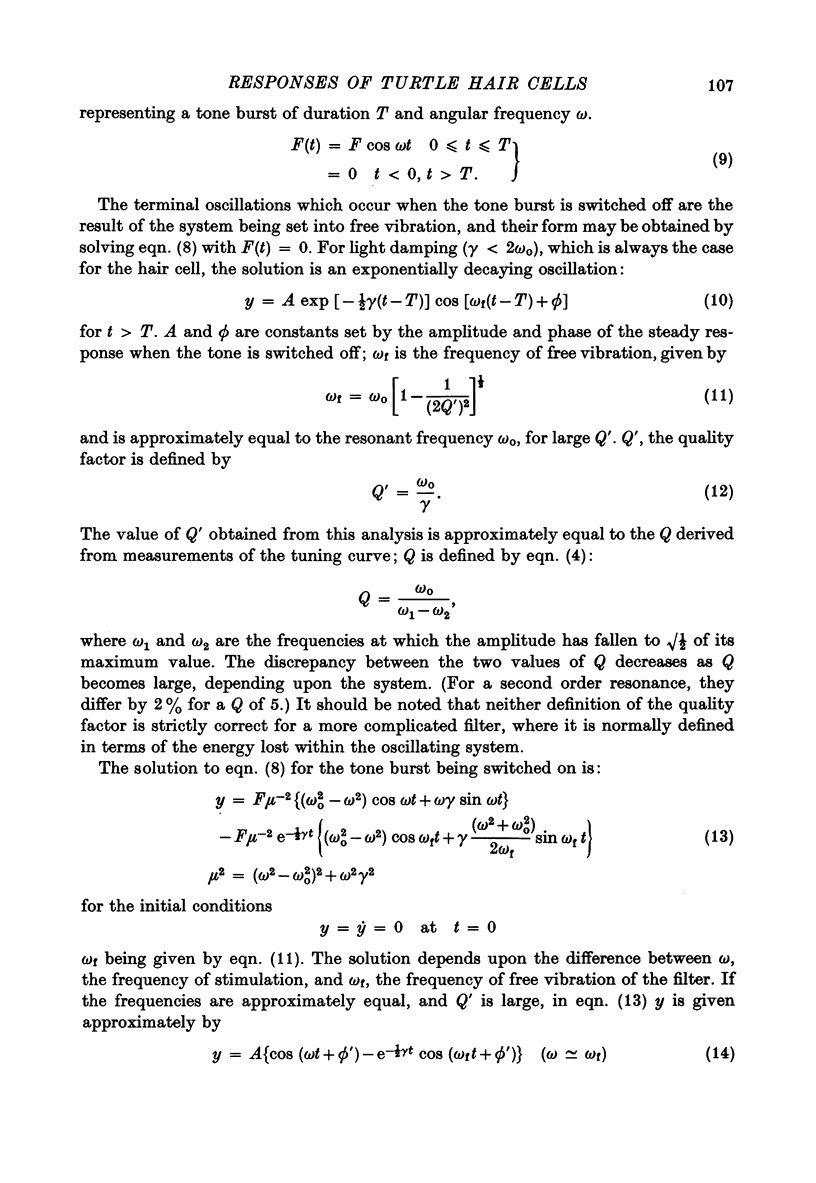
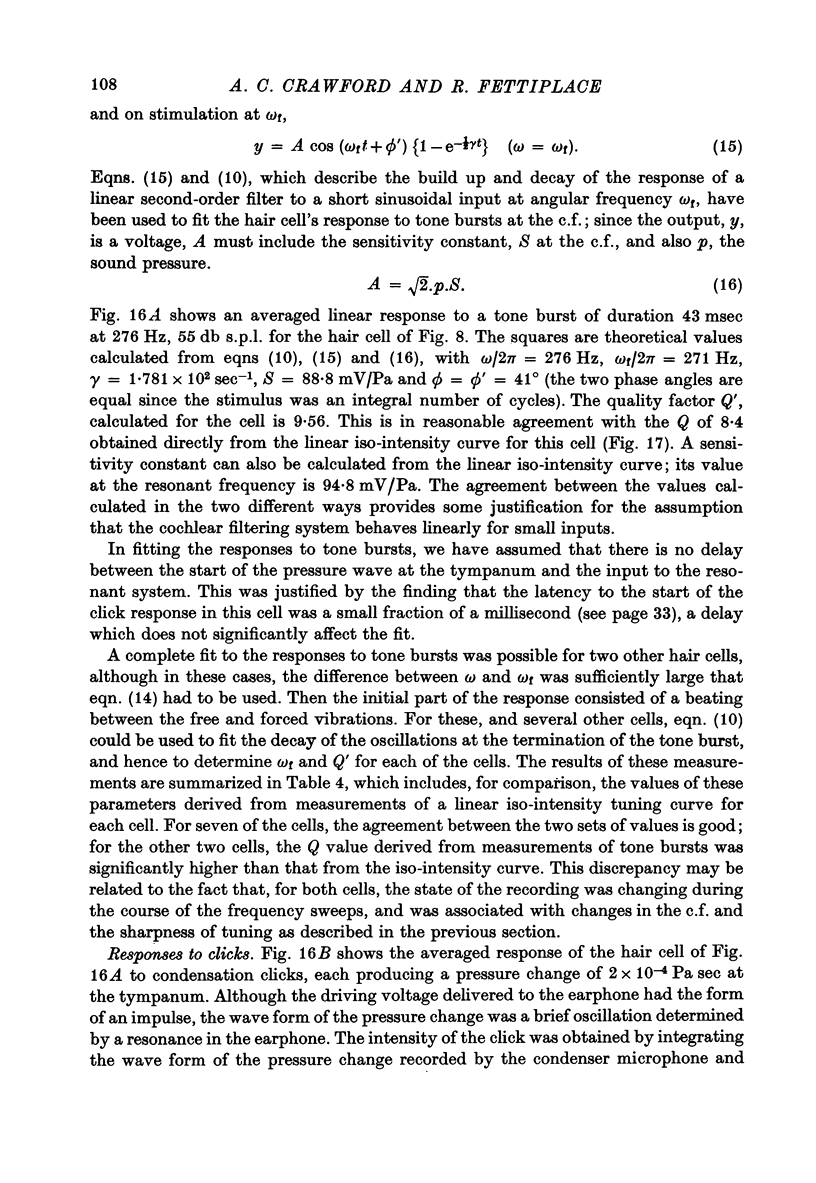
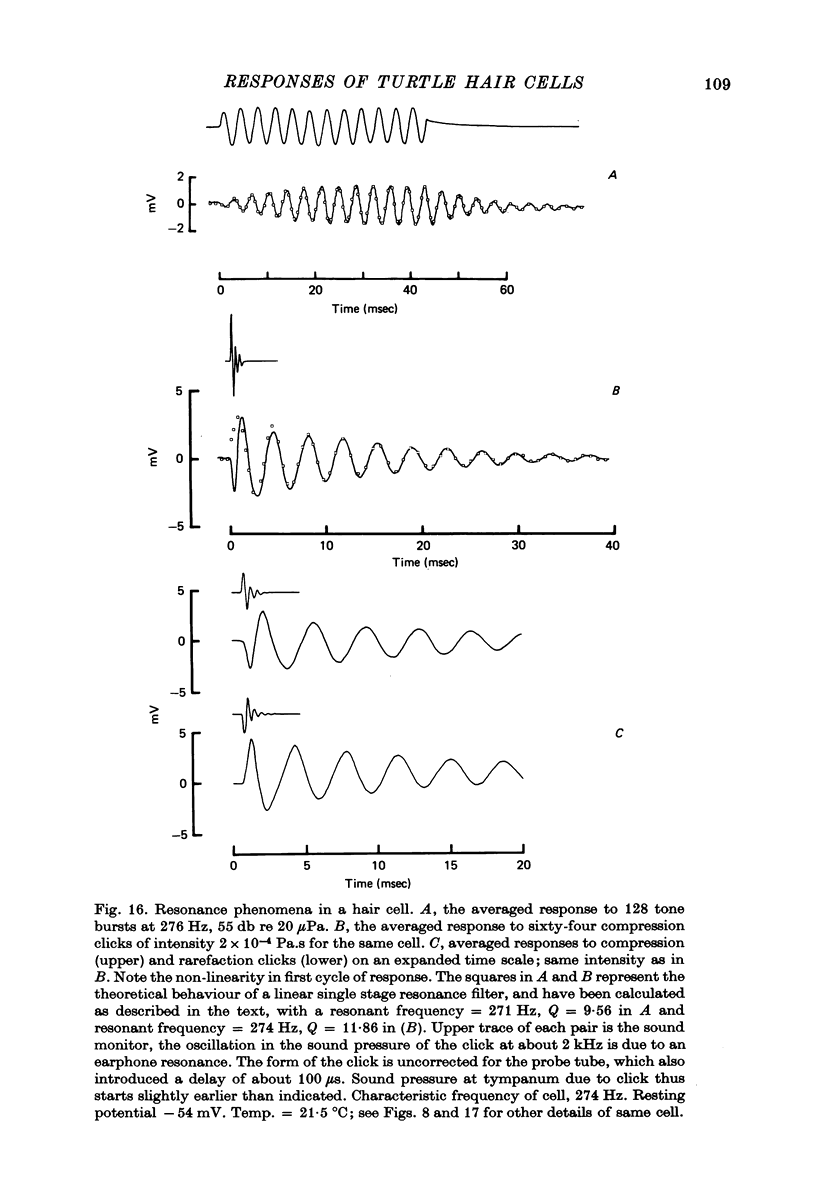
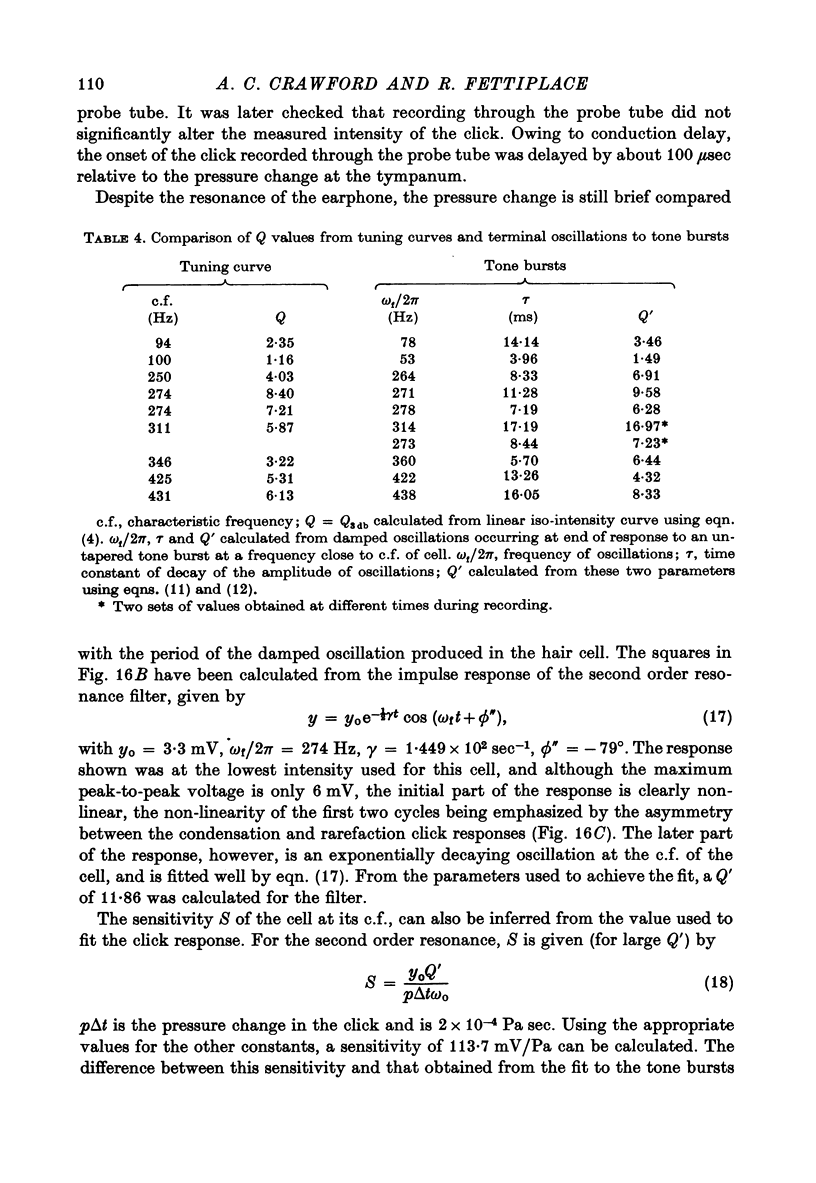
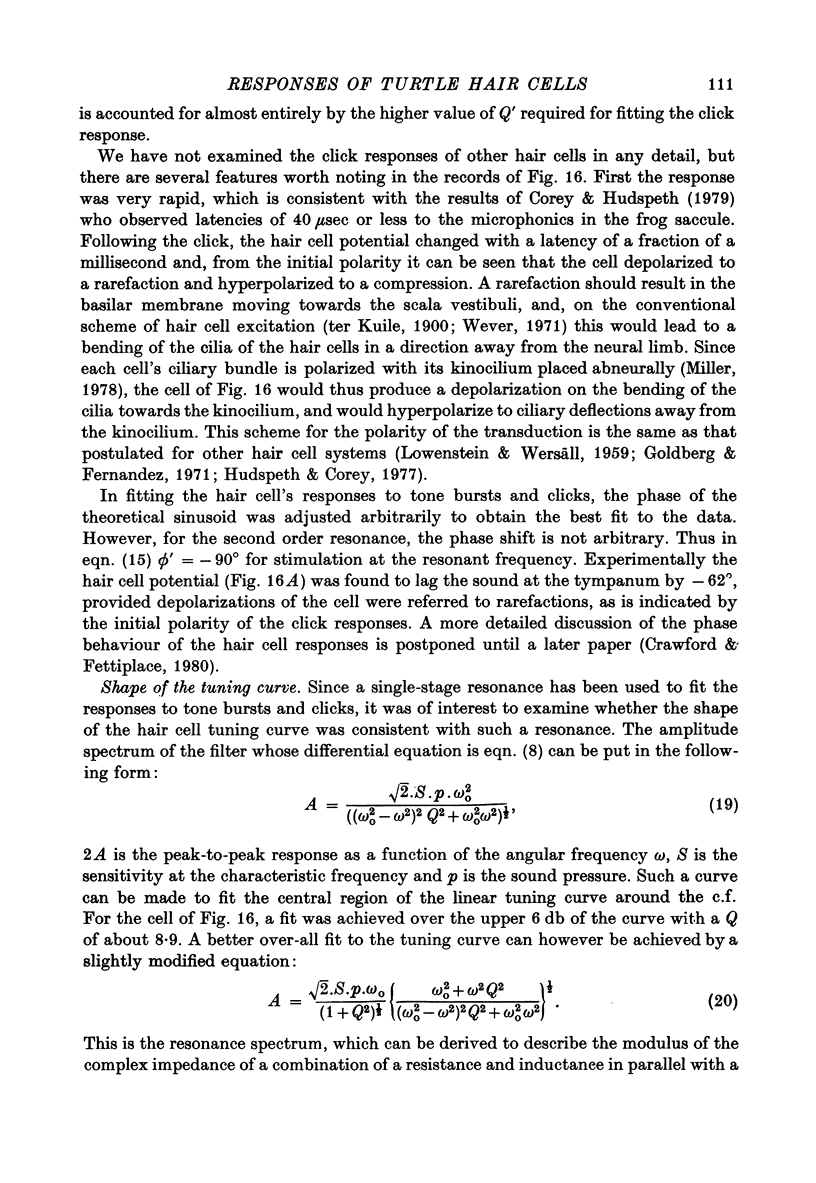
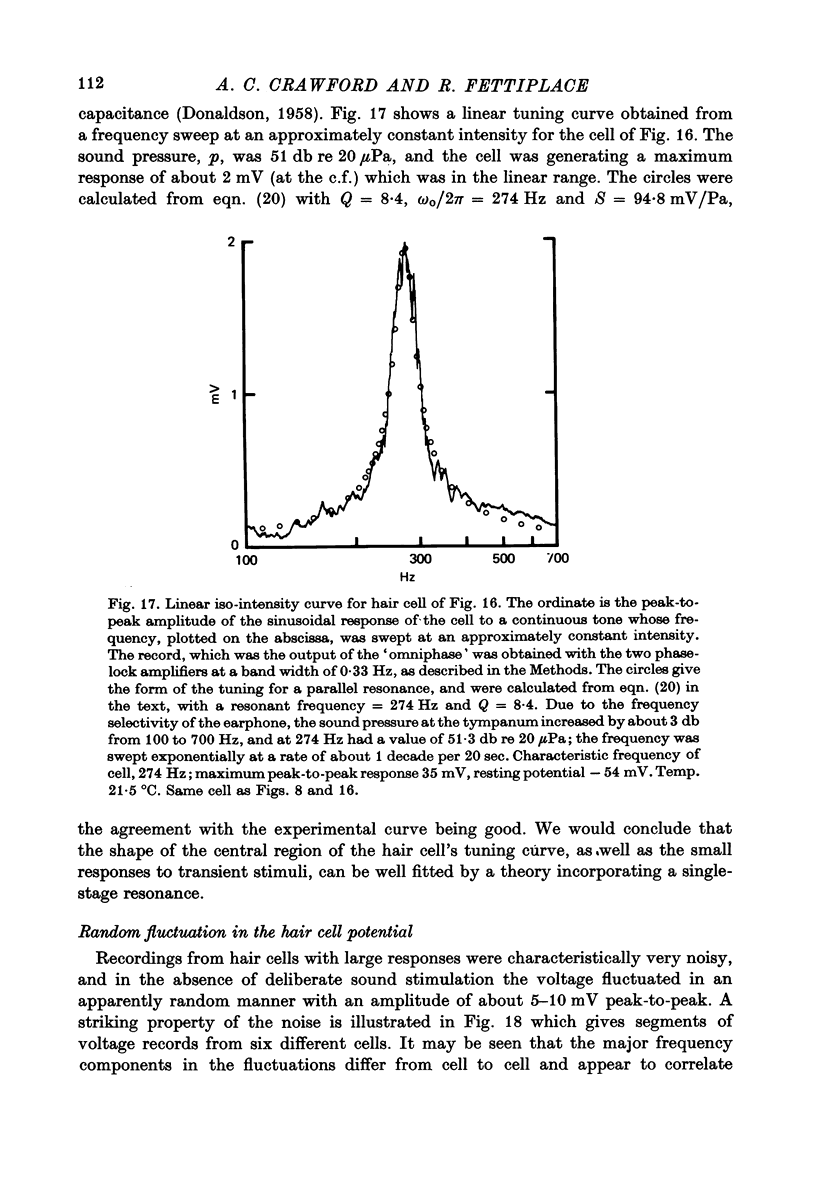
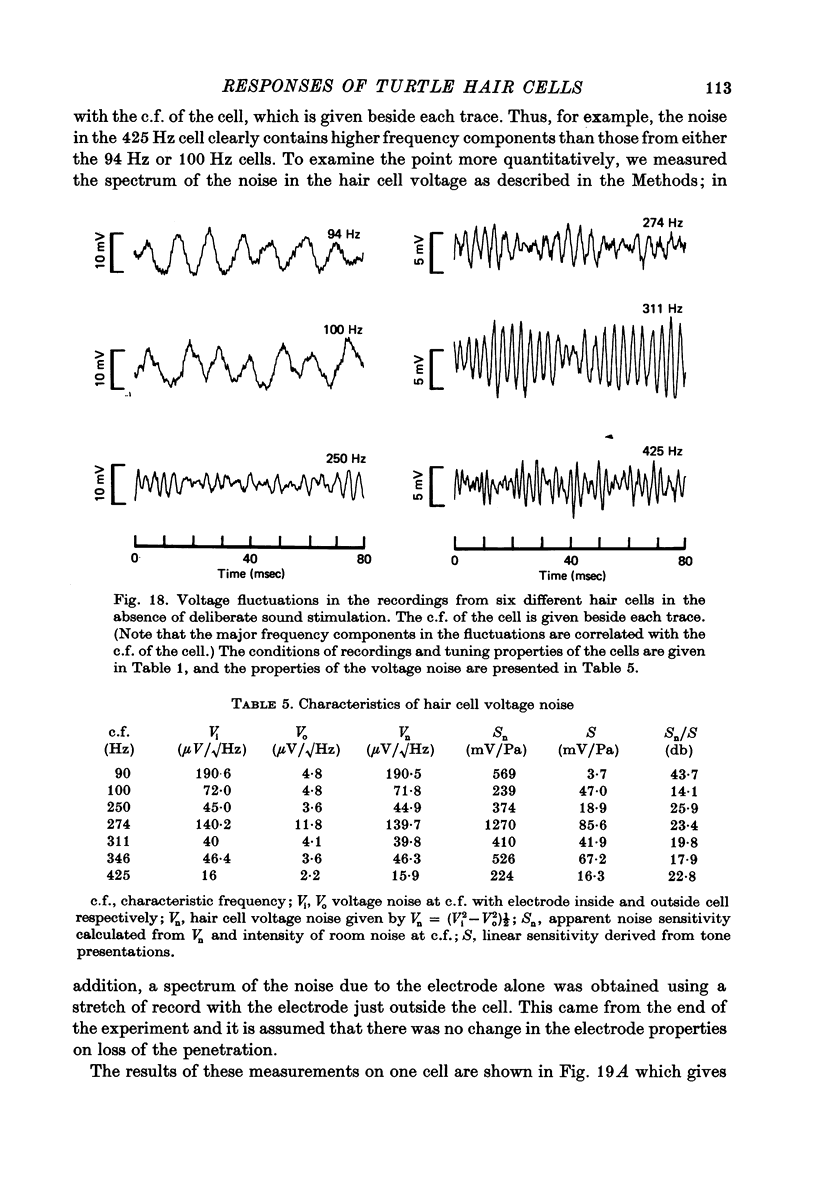
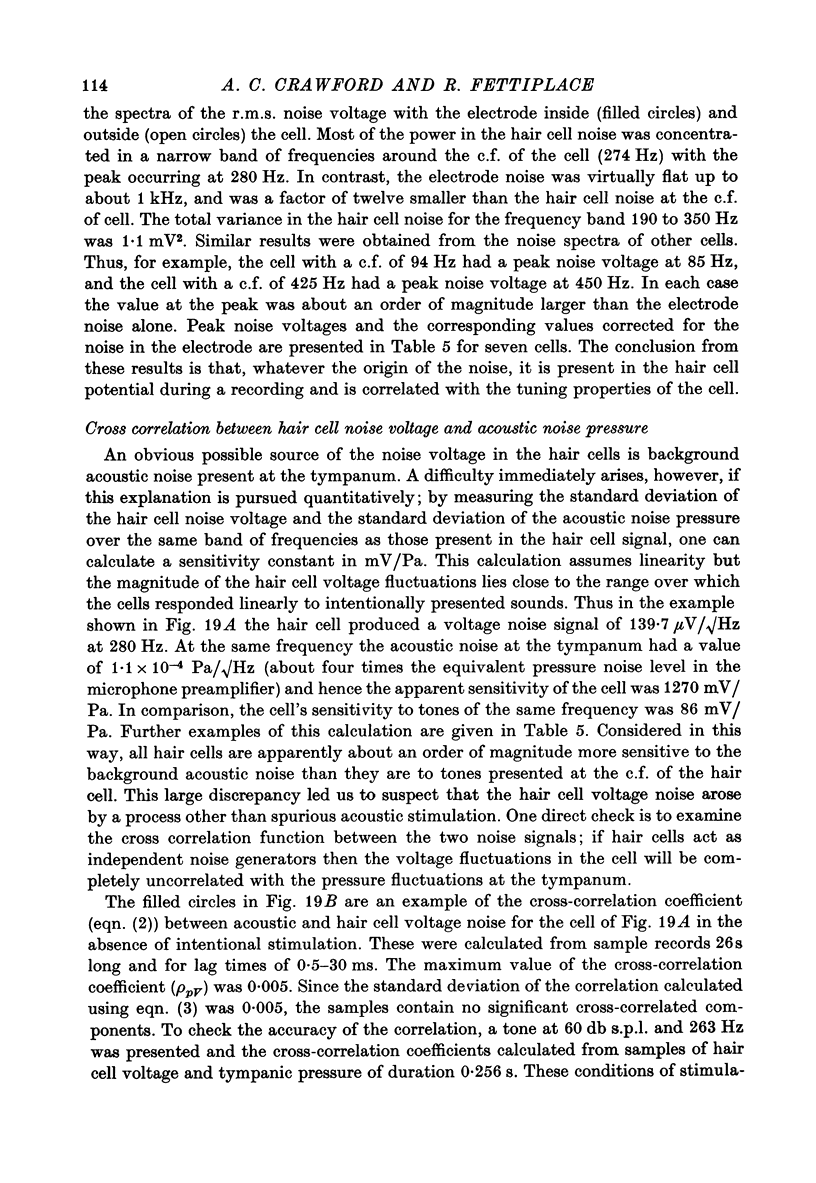
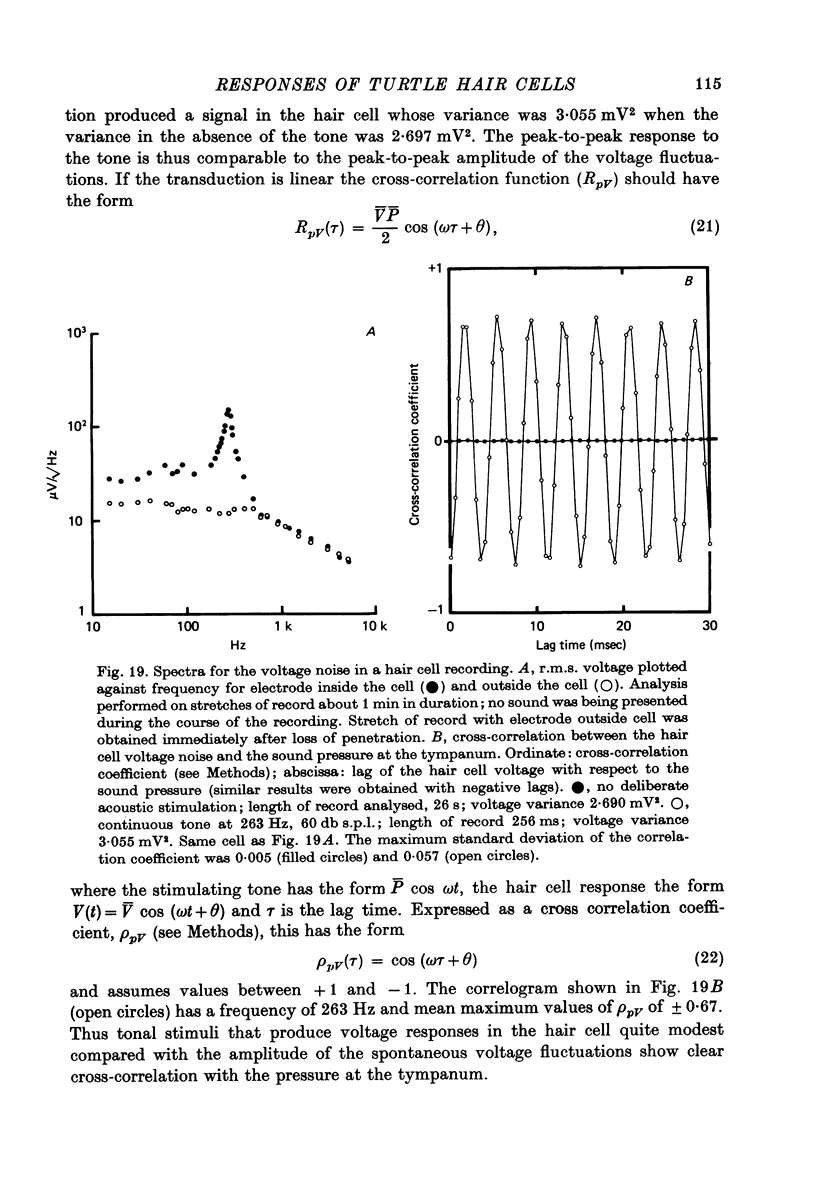
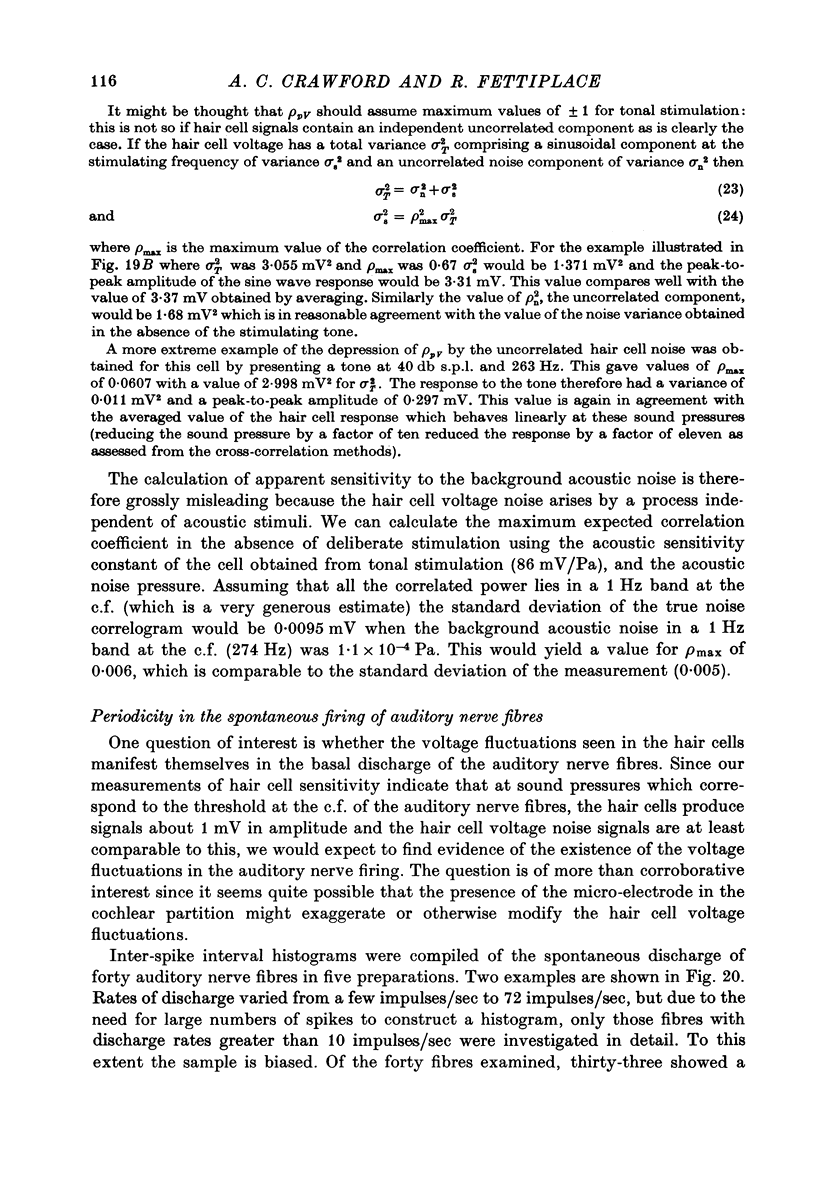
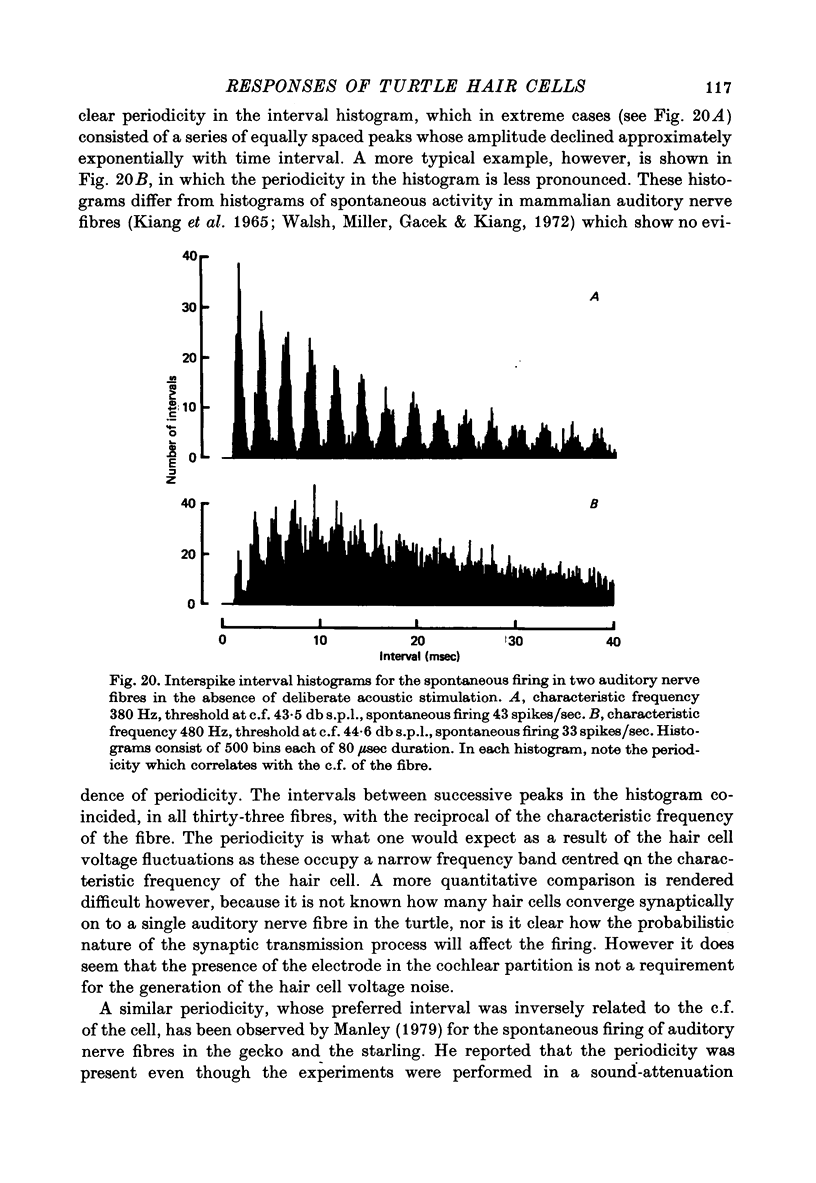
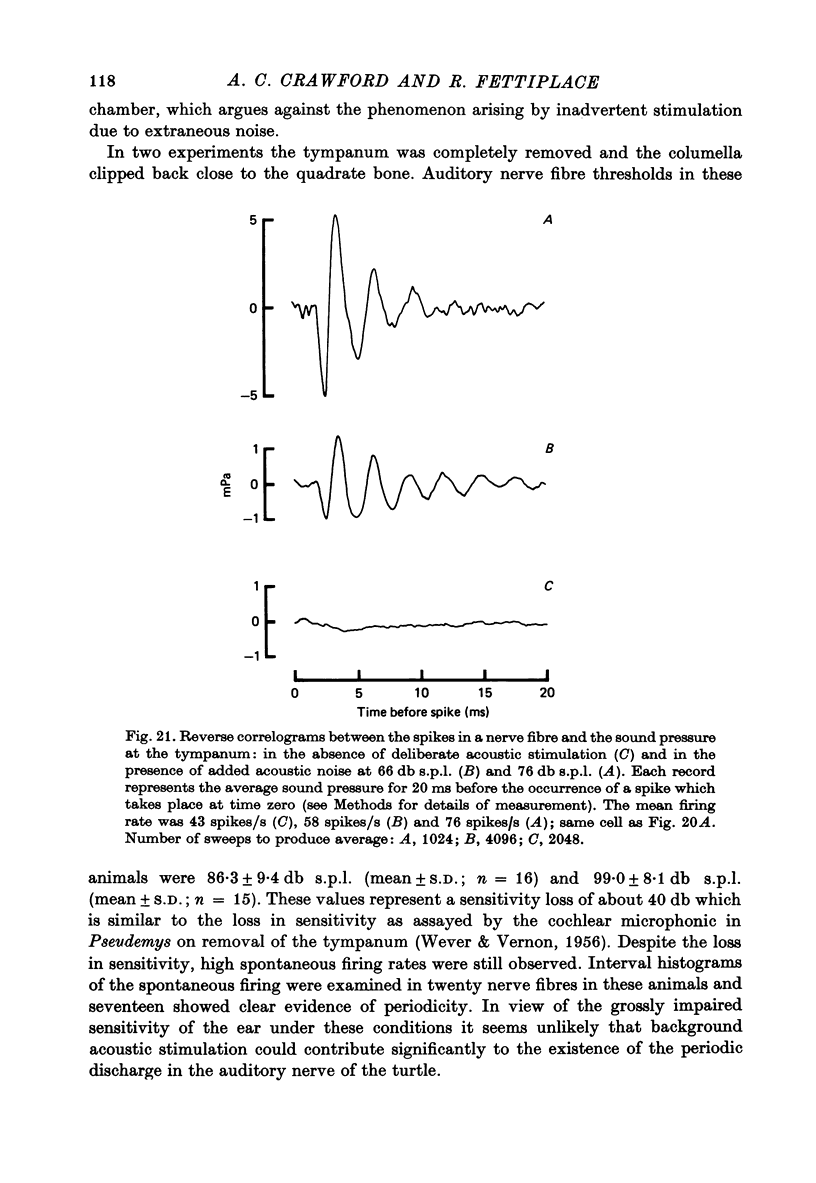
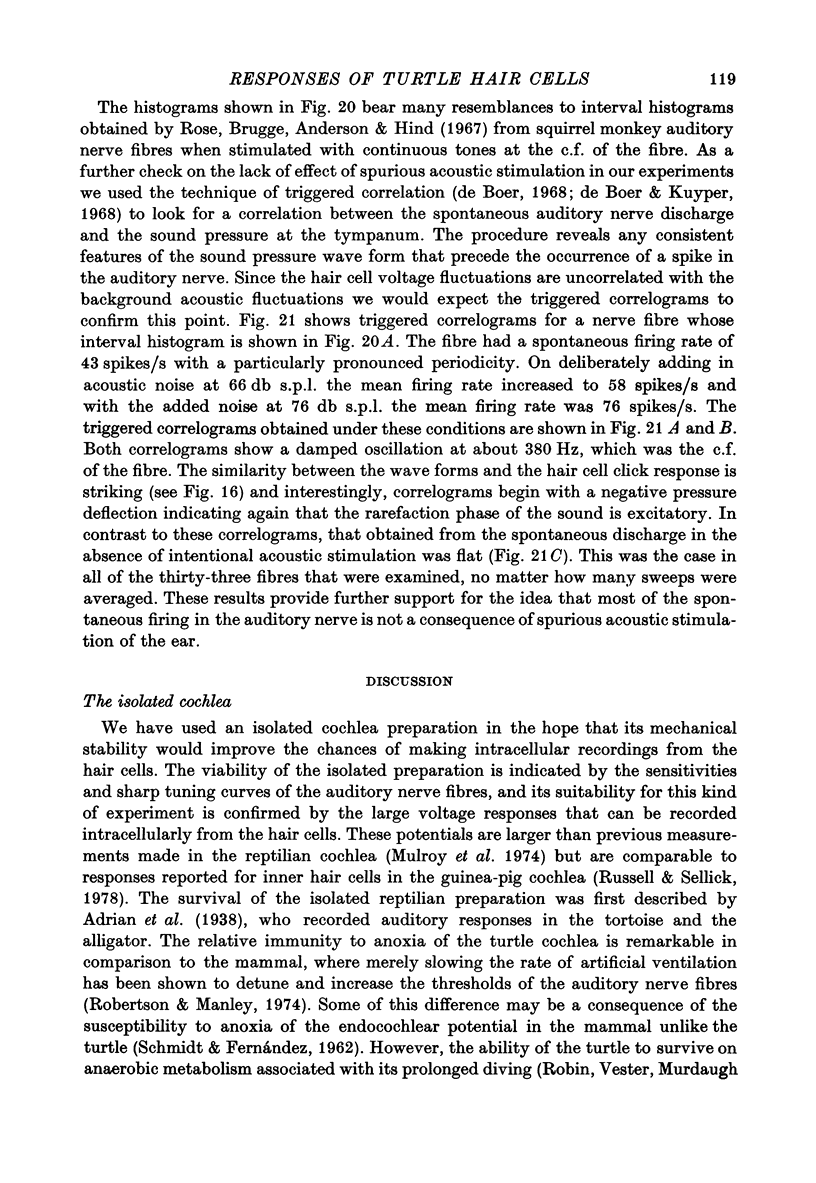
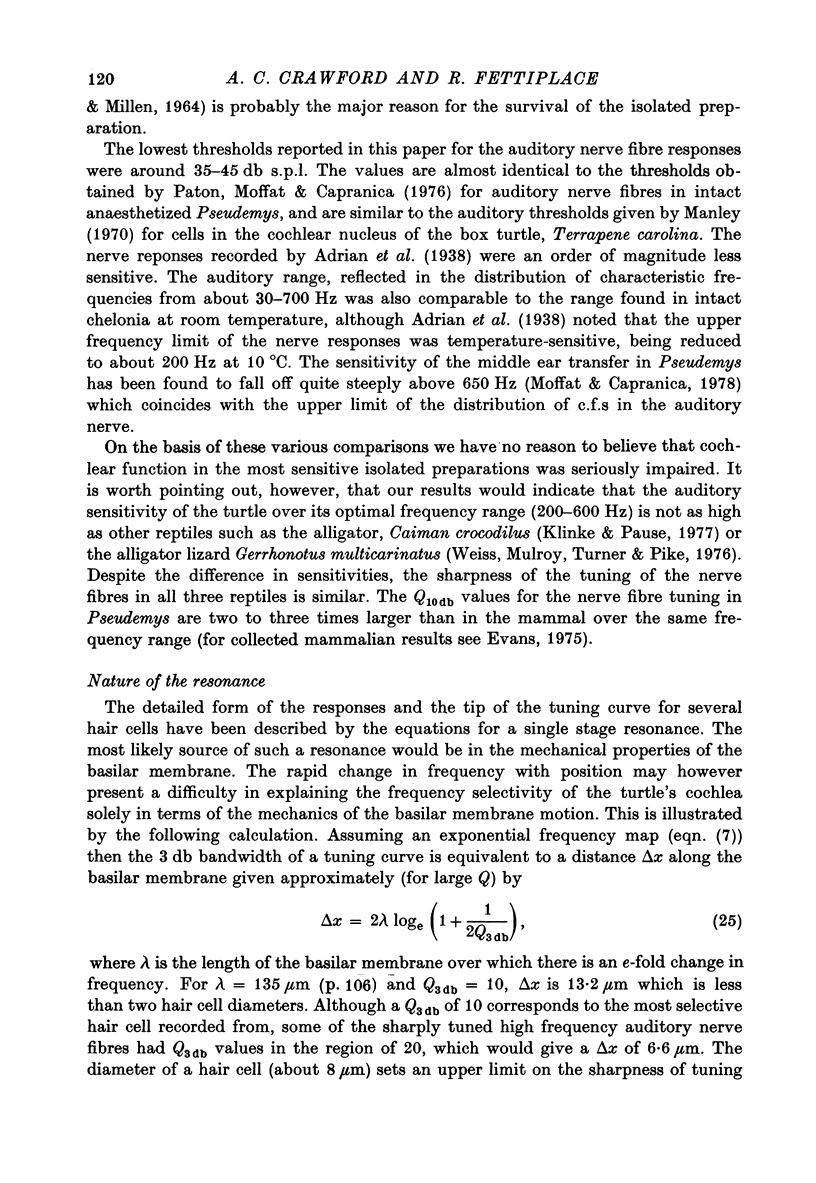
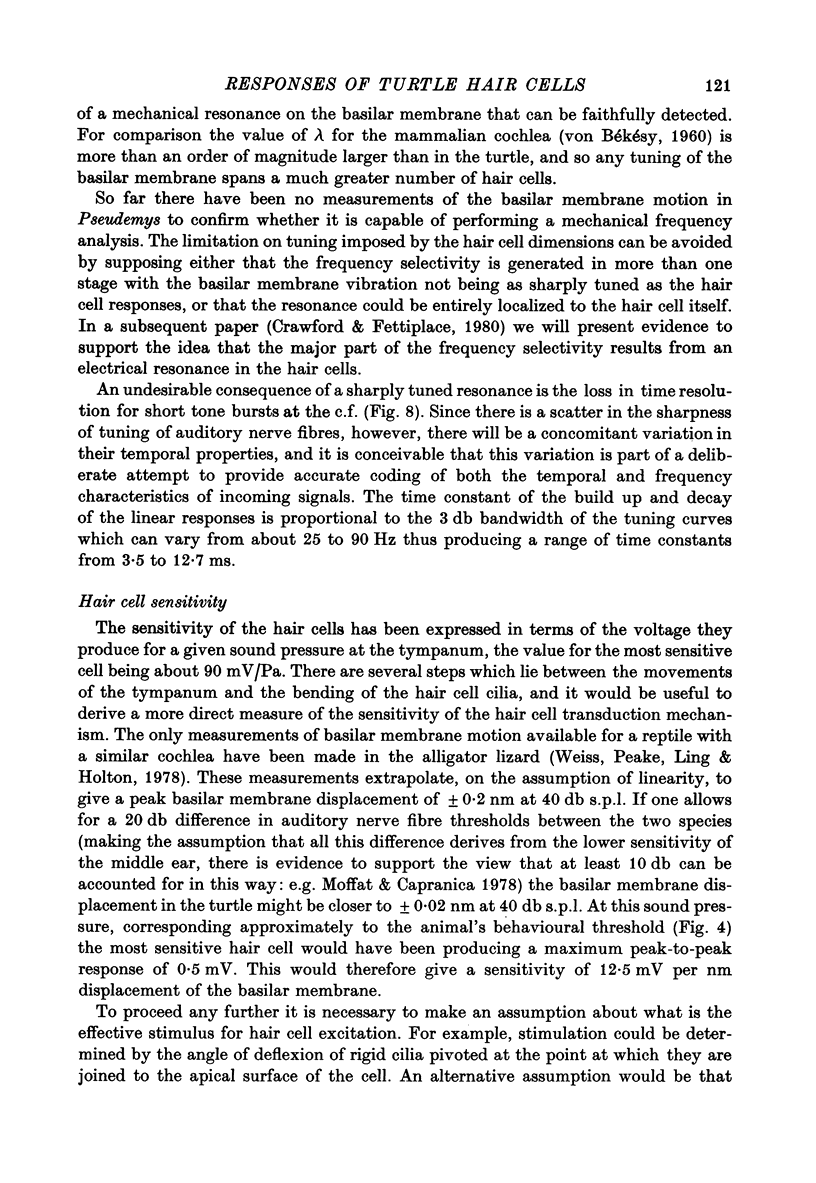
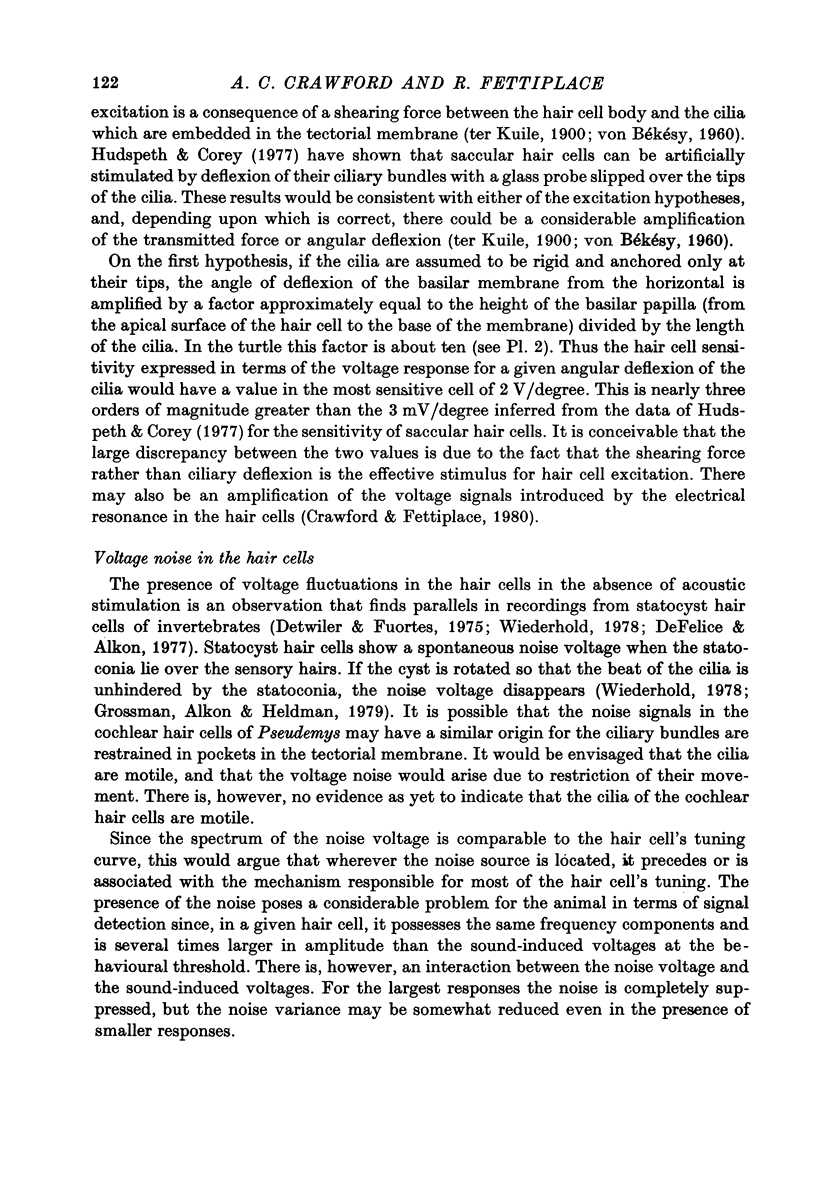
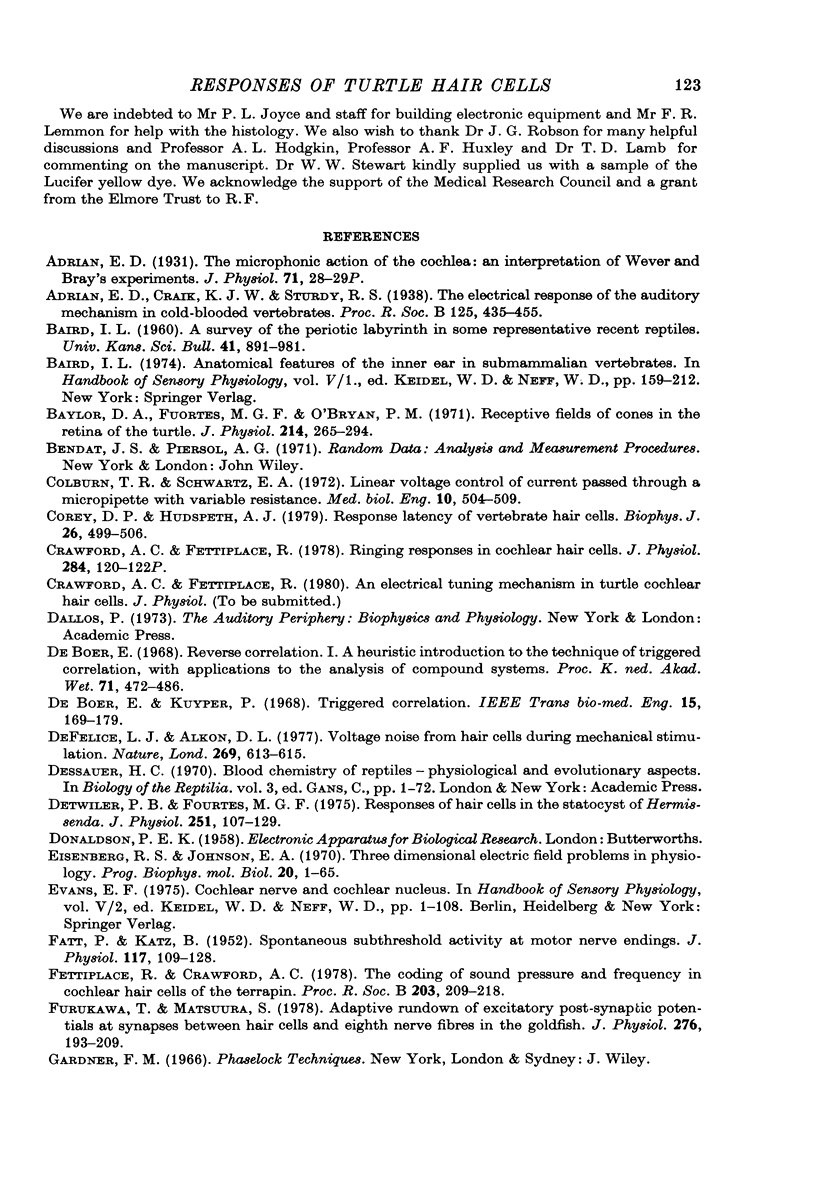
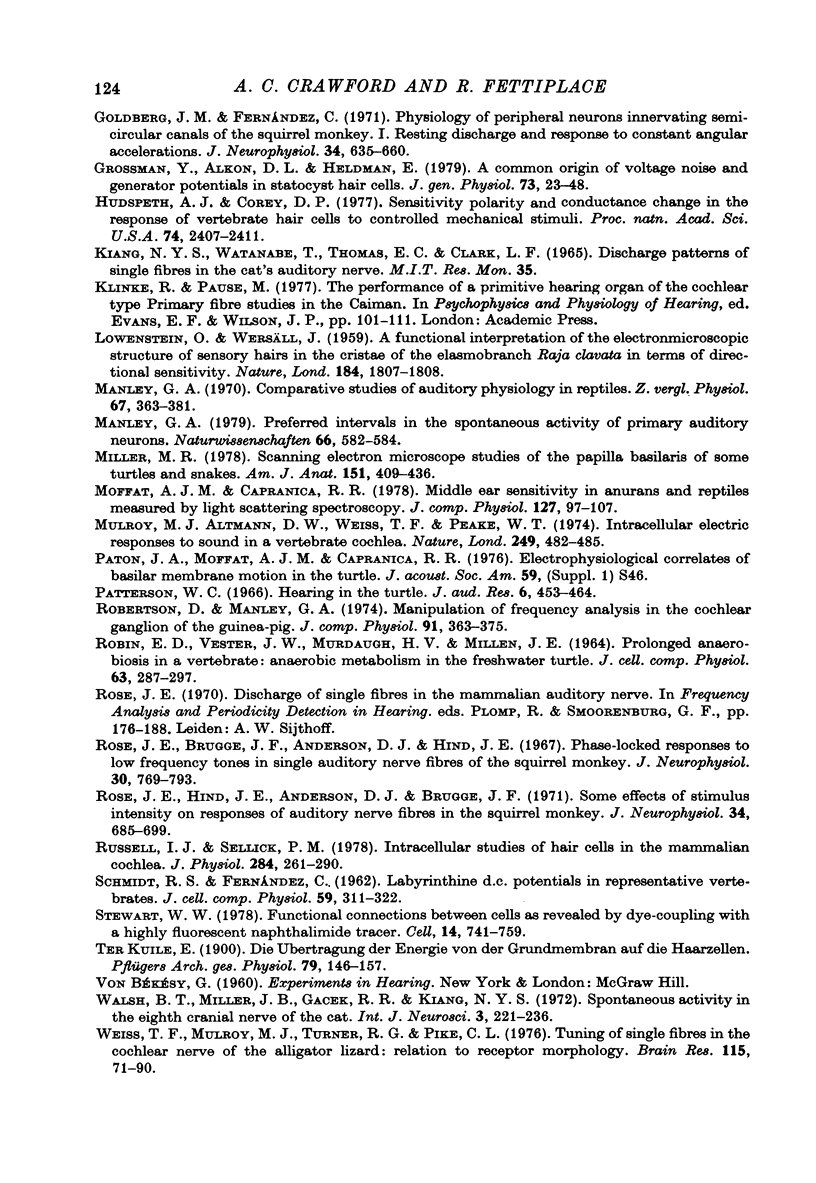
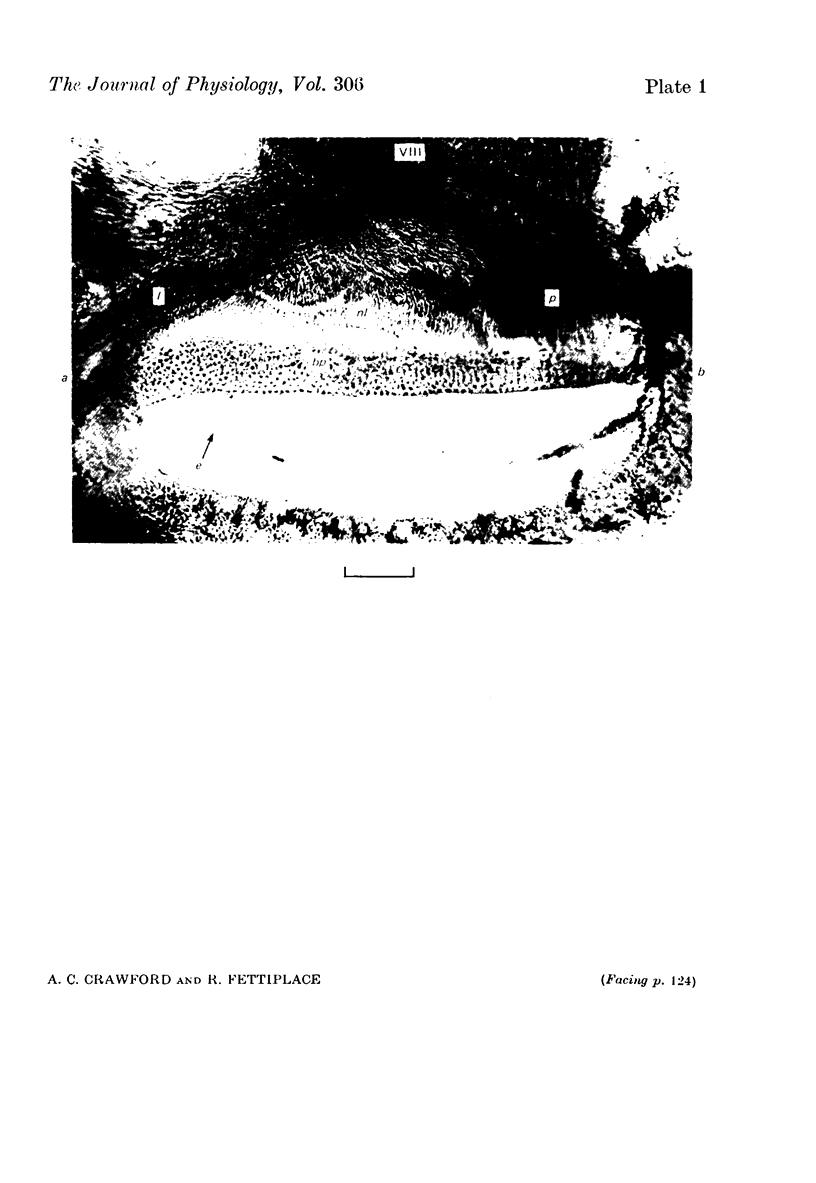
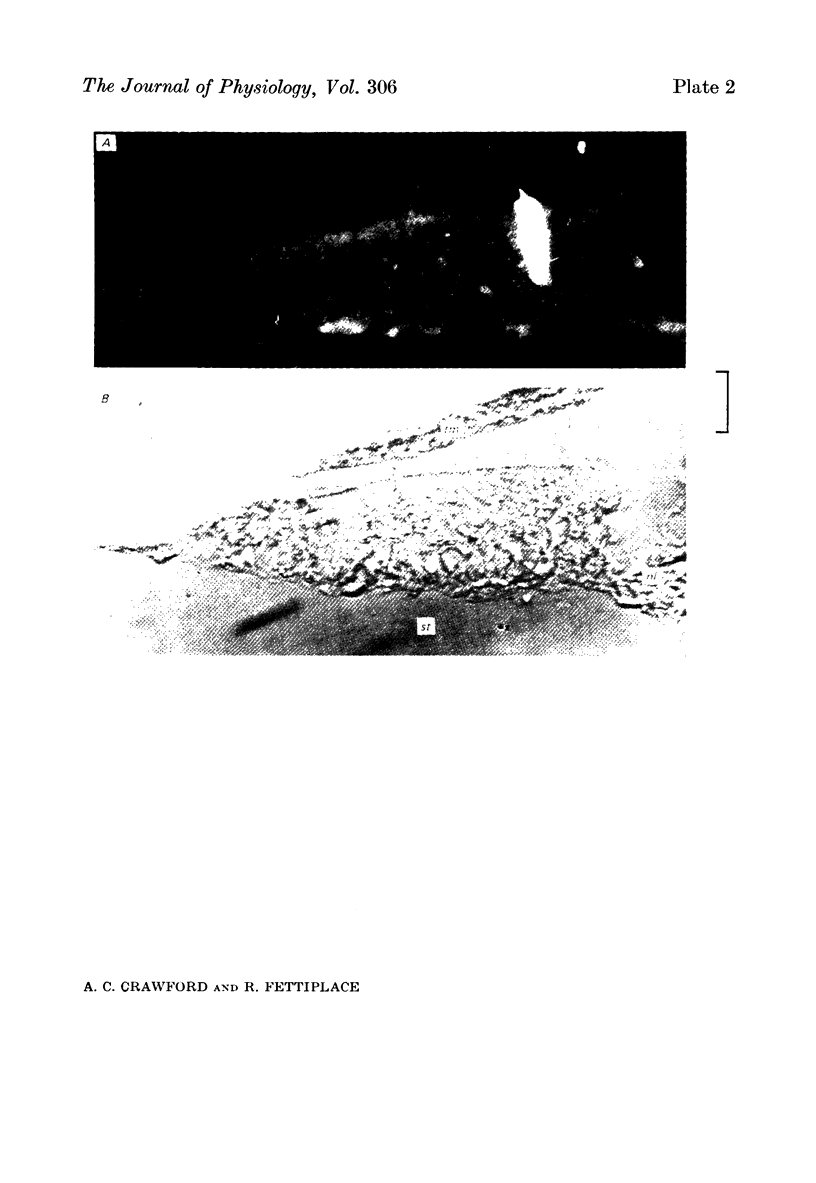
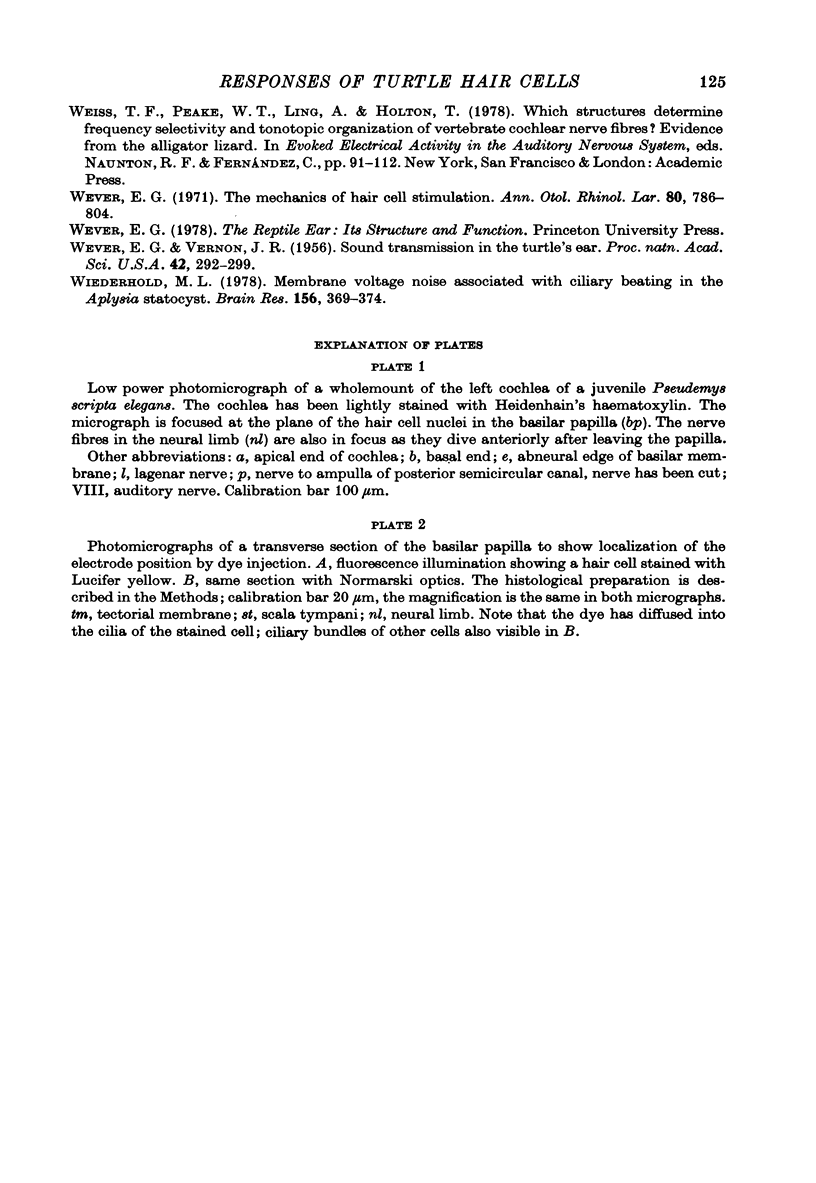
Images in this article
Selected References
These references are in PubMed. This may not be the complete list of references from this article.
- Baylor D. A., Fuortes M. G., O'Bryan P. M. Receptive fields of cones in the retina of the turtle. J Physiol. 1971 Apr;214(2):265–294. doi: 10.1113/jphysiol.1971.sp009432. [DOI] [PMC free article] [PubMed] [Google Scholar]
- Colburn T. R., Schwartz E. A. Linear voltage control of current passed through a micropipette with variable resistance. Med Biol Eng. 1972 Jul;10(4):504–509. doi: 10.1007/BF02474198. [DOI] [PubMed] [Google Scholar]
- Corey D. P., Hudspeth A. J. Response latency of vertebrate hair cells. Biophys J. 1979 Jun;26(3):499–506. doi: 10.1016/S0006-3495(79)85267-4. [DOI] [PMC free article] [PubMed] [Google Scholar]
- Crawford A. C., Fettiplace R. Ringing responses in cochlear hair cells of the turtle [proceedings]. J Physiol. 1978 Nov;284:120P–122P. [PubMed] [Google Scholar]
- DeFelice L. J., Alkon D. L. Voltage noise from hair cells during mechanical stimulation. Nature. 1977 Oct 13;269(5629):613–615. doi: 10.1038/269613a0. [DOI] [PubMed] [Google Scholar]
- Detwiler P. B., Fuortes M. G. Responses of hair cells in the statocyst of Hermissenda. J Physiol. 1975 Sep;251(1):107–129. doi: 10.1113/jphysiol.1975.sp011083. [DOI] [PMC free article] [PubMed] [Google Scholar]
- FATT P., KATZ B. Spontaneous subthreshold activity at motor nerve endings. J Physiol. 1952 May;117(1):109–128. [PMC free article] [PubMed] [Google Scholar]
- Fettiplace R., Crawford A. C. The coding of sound pressure and frequency in cochlear hair cells of the terrapin. Proc R Soc Lond B Biol Sci. 1978 Dec 4;203(1151):209–218. doi: 10.1098/rspb.1978.0101. [DOI] [PubMed] [Google Scholar]
- Furukawa T., Matsuura S. Adaptive rundown of excitatory post-synaptic potentials at synapses between hair cells and eight nerve fibres in the goldfish. J Physiol. 1978 Mar;276:193–209. doi: 10.1113/jphysiol.1978.sp012228. [DOI] [PMC free article] [PubMed] [Google Scholar]
- Goldberg J. M., Fernandez C. Physiology of peripheral neurons innervating semicircular canals of the squirrel monkey. I. Resting discharge and response to constant angular accelerations. J Neurophysiol. 1971 Jul;34(4):635–660. doi: 10.1152/jn.1971.34.4.635. [DOI] [PubMed] [Google Scholar]
- Grossman Y., Alkon D. L., Heldman E. A common origin of voltage noise and generator potentials in statocyst hair cells. J Gen Physiol. 1979 Jan;73(1):23–48. doi: 10.1085/jgp.73.1.23. [DOI] [PMC free article] [PubMed] [Google Scholar]
- Hudspeth A. J., Corey D. P. Sensitivity, polarity, and conductance change in the response of vertebrate hair cells to controlled mechanical stimuli. Proc Natl Acad Sci U S A. 1977 Jun;74(6):2407–2411. doi: 10.1073/pnas.74.6.2407. [DOI] [PMC free article] [PubMed] [Google Scholar]
- Manley G. A. Preferred intervals in the spontaneous activity of primary auditory neurons. Naturwissenschaften. 1979 Nov;66(11):582–584. doi: 10.1007/BF00368823. [DOI] [PubMed] [Google Scholar]
- Miller M. R. Scanning electron microscope studies of the papilla basilaris of some turtles and snakes. Am J Anat. 1978 Mar;151(3):409–435. doi: 10.1002/aja.1001510306. [DOI] [PubMed] [Google Scholar]
- Mulroy M. J., Altmann D. W., Weiss T. F., Peake W. T. Intracellular electric responses to sound in a vertebrate cochlea. Nature. 1974 May 31;249(456):482–485. doi: 10.1038/249482a0. [DOI] [PubMed] [Google Scholar]
- ROBIN E. D., VESTER J. W., MURDAUGH H. V., Jr, MILLEN J. E. PROLONGED ANAEROBIOSIS IN A VERTEBRATE: ANAEROBIC METABOLISM IN THE FRESHWATER TURTLE. J Cell Physiol. 1964 Jun;63:287–297. doi: 10.1002/jcp.1030630304. [DOI] [PubMed] [Google Scholar]
- Rose J. E., Brugge J. F., Anderson D. J., Hind J. E. Phase-locked response to low-frequency tones in single auditory nerve fibers of the squirrel monkey. J Neurophysiol. 1967 Jul;30(4):769–793. doi: 10.1152/jn.1967.30.4.769. [DOI] [PubMed] [Google Scholar]
- Rose J. E., Hind J. E., Anderson D. J., Brugge J. F. Some effects of stimulus intensity on response of auditory nerve fibers in the squirrel monkey. J Neurophysiol. 1971 Jul;34(4):685–699. doi: 10.1152/jn.1971.34.4.685. [DOI] [PubMed] [Google Scholar]
- Russell I. J., Sellick P. M. Intracellular studies of hair cells in the mammalian cochlea. J Physiol. 1978 Nov;284:261–290. doi: 10.1113/jphysiol.1978.sp012540. [DOI] [PMC free article] [PubMed] [Google Scholar]
- SCHMIDT R. S., FERNANDEZ C. Labyrinthine DC potentials in representative vertebrates. J Cell Comp Physiol. 1962 Jun;59:311–322. doi: 10.1002/jcp.1030590311. [DOI] [PubMed] [Google Scholar]
- Stewart W. W. Functional connections between cells as revealed by dye-coupling with a highly fluorescent naphthalimide tracer. Cell. 1978 Jul;14(3):741–759. doi: 10.1016/0092-8674(78)90256-8. [DOI] [PubMed] [Google Scholar]
- Weiss T. F., Mulroy M. J., Turner R. G., Pike C. L. Tuning of single fibers in the cochlear nerve of the alligator lizard: relation to receptor morphology. Brain Res. 1976 Oct 8;115(1):71–90. doi: 10.1016/0006-8993(76)90823-4. [DOI] [PubMed] [Google Scholar]
- Wever E. G. The mechanics of hair-cell stimulation. Ann Otol Rhinol Laryngol. 1971 Dec;80(6):786–804. doi: 10.1177/000348947108000606. [DOI] [PubMed] [Google Scholar]
- Wever E. G., Vernon J. A. SOUND TRANSMISSION IN THE TURTLE'S EAR. Proc Natl Acad Sci U S A. 1956 May;42(5):292–299. doi: 10.1073/pnas.42.5.292. [DOI] [PMC free article] [PubMed] [Google Scholar]
- Wiederhold M. L. Membrane voltage noise associated with ciliary beating in the Aplysia statocyst. Brain Res. 1978 Nov 10;156(2):369–374. doi: 10.1016/0006-8993(78)90521-8. [DOI] [PubMed] [Google Scholar]
- de Boer R., Kuyper P. Triggered correlation. IEEE Trans Biomed Eng. 1968 Jul;15(3):169–179. doi: 10.1109/tbme.1968.4502561. [DOI] [PubMed] [Google Scholar]