Abstract
1. Na channel reversal potentials were studied in perfused voltage clamped squid giant axons. The concentration dependence of ion selectivity was determined with both external and internal changes in Na and ammonium concentrations. 2. A tenfold change in the internal ammonium activity results in a 42 mV shift in the reversal potential, rather than the 56 mV shift expected from the Goldman, Hodgkin, Katz equation for a constant PNa/PNH4 ratio. However, changing [Na]o tenfold at constant internal [NH4] gives approximately the expected 56 mV shift. Therefore, the apparent channel selectivity depends upon the internal ammonium concentration but not the external Na concentration. 3. With ammonium outside and Na inside, the calculated permeability ratio is nearly constant, regardless of the permeant ion concentration. 4. Internal Cs ions can alter the Na/K permeability ratio. 5. The results are considered in terms of a three-barrier, two-site ionic permeation model.
Full text
PDF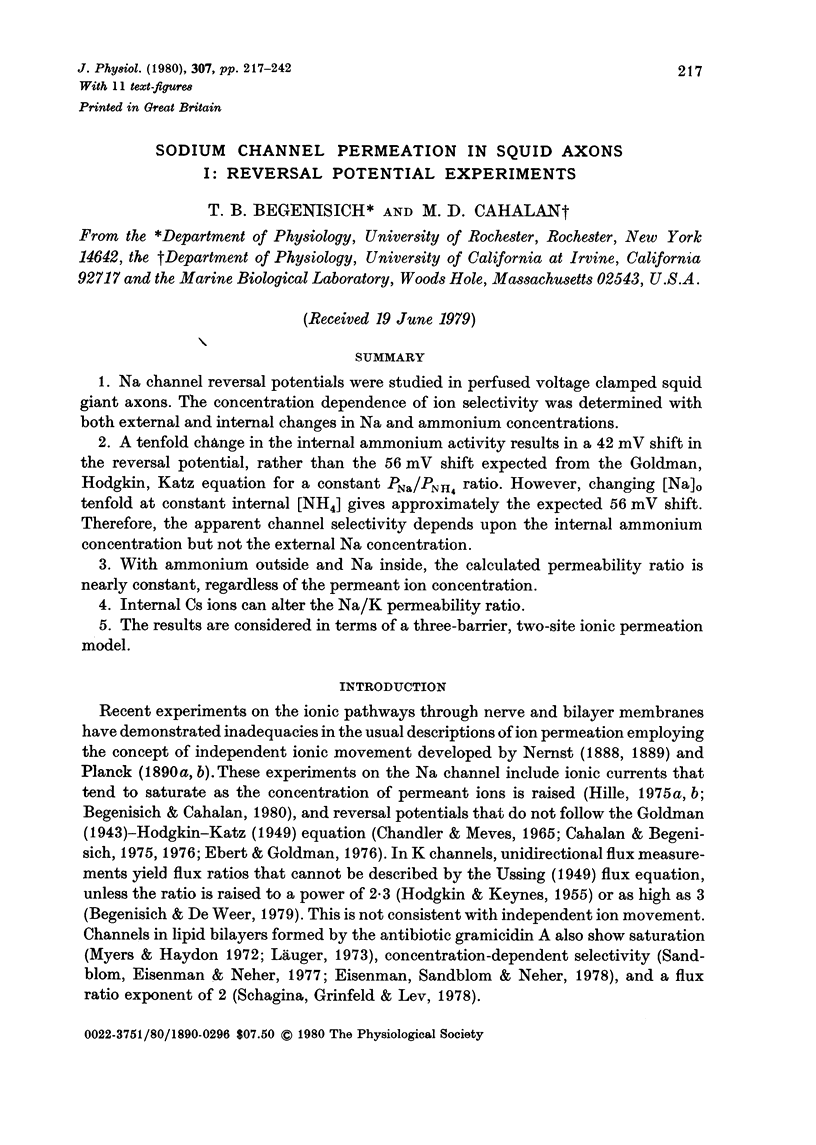
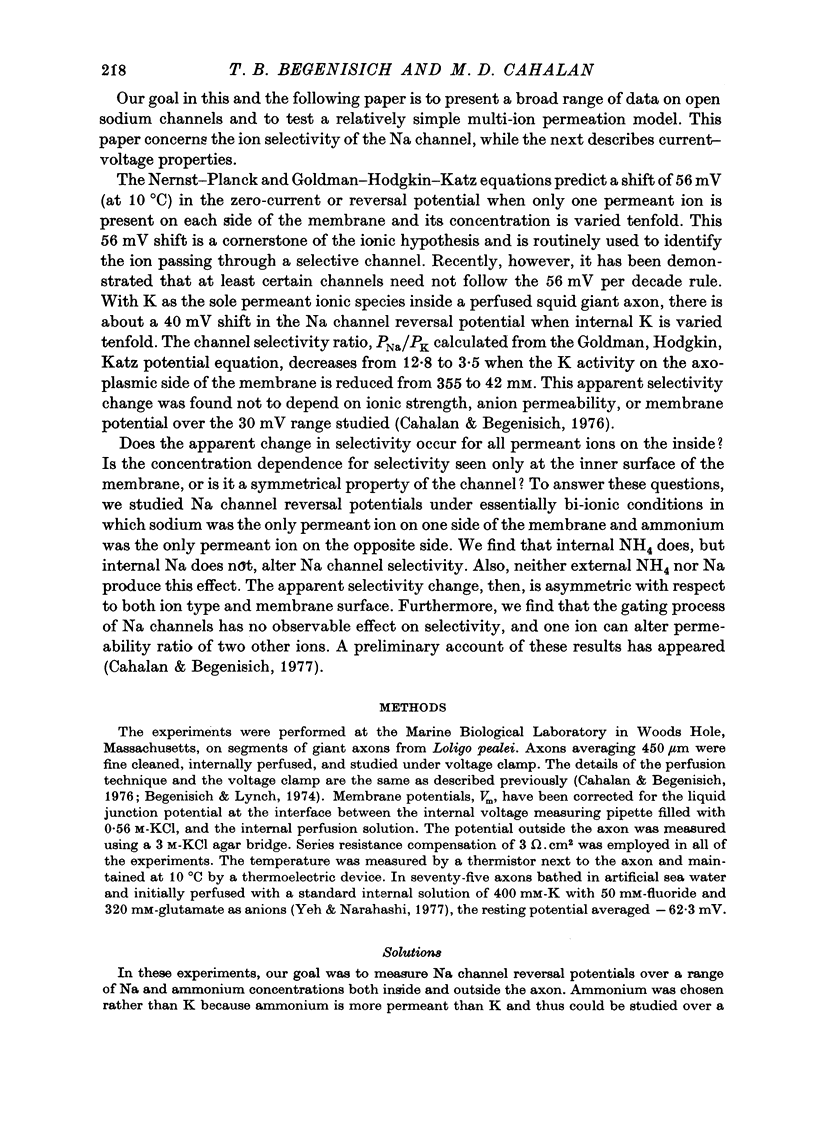
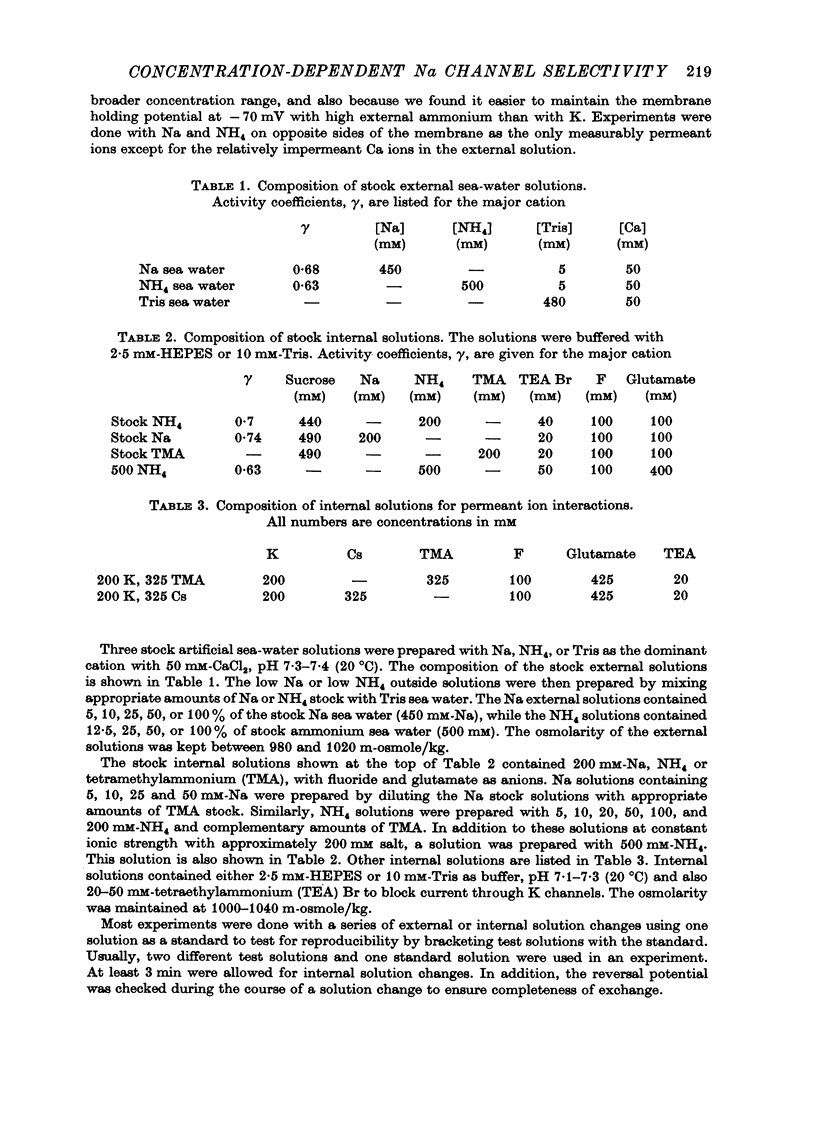
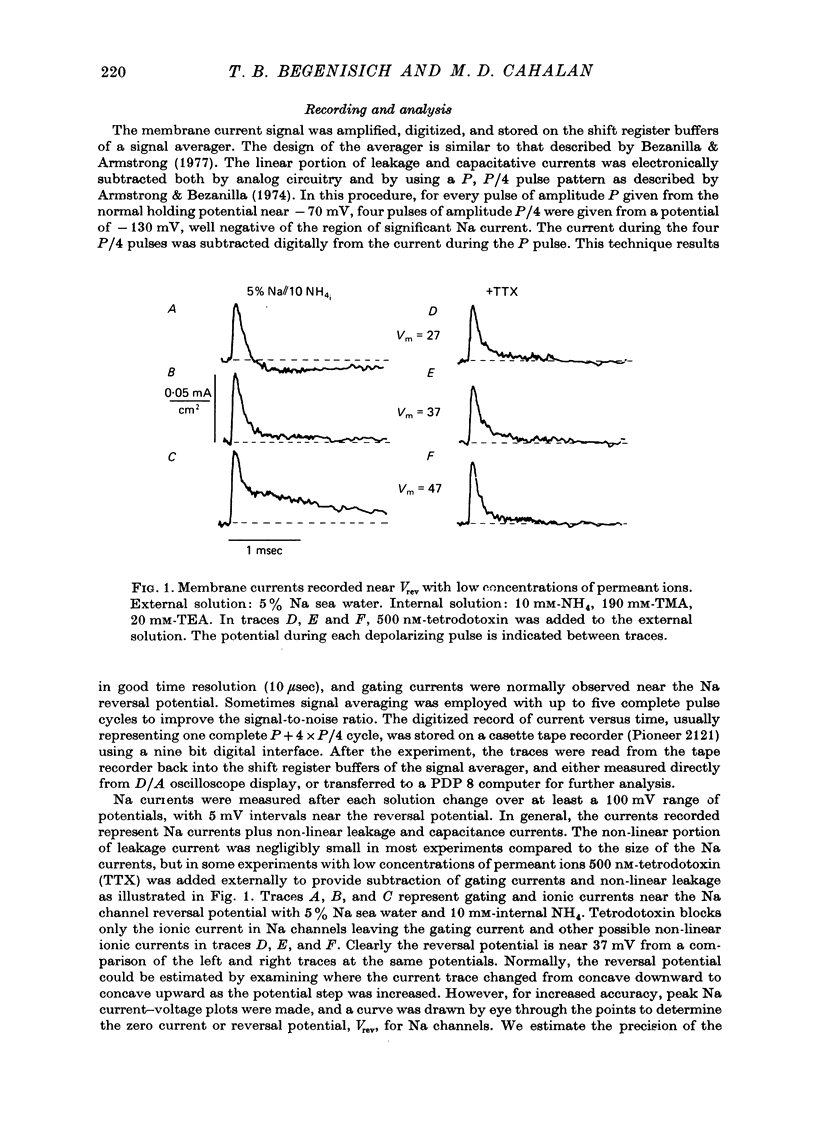
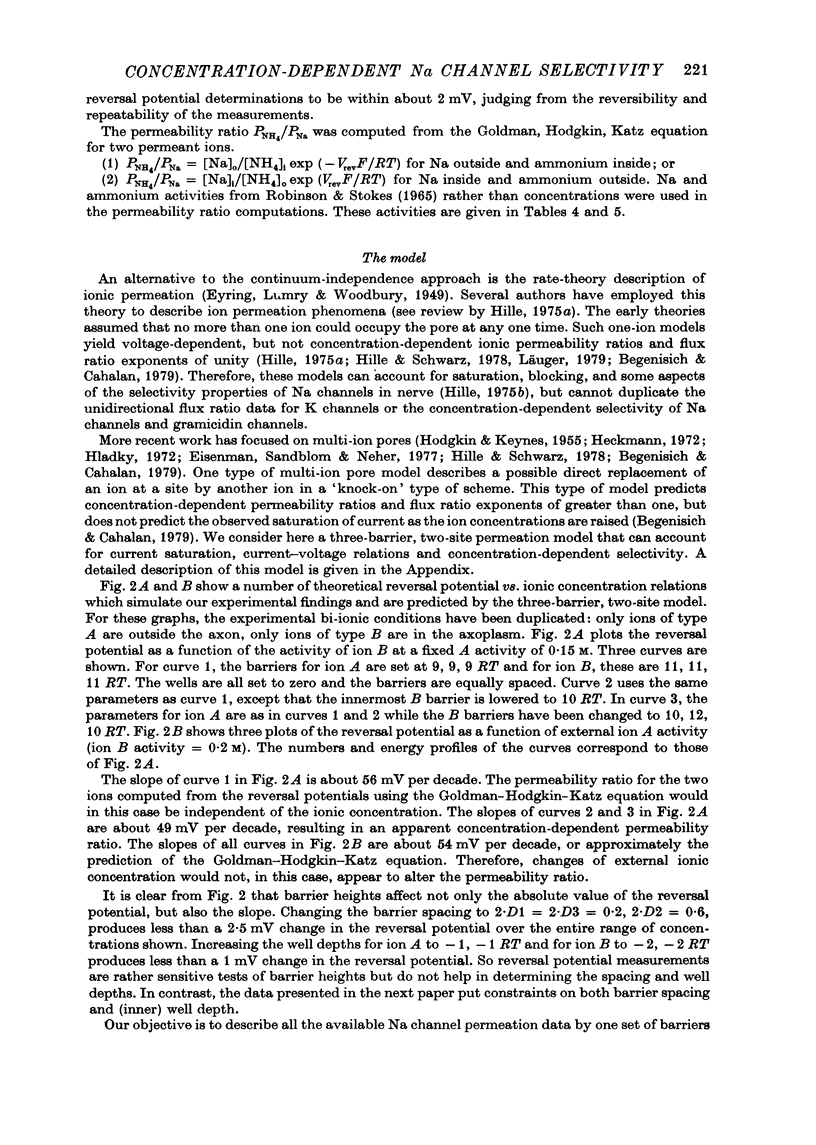
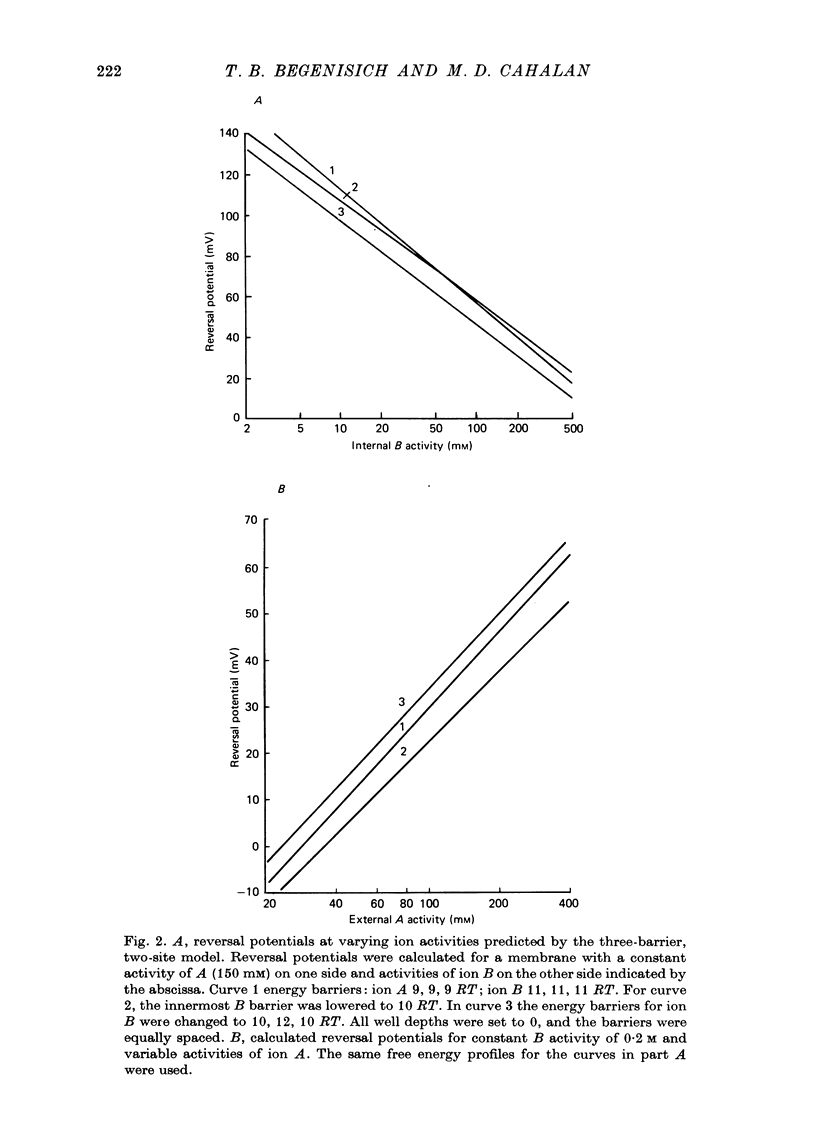
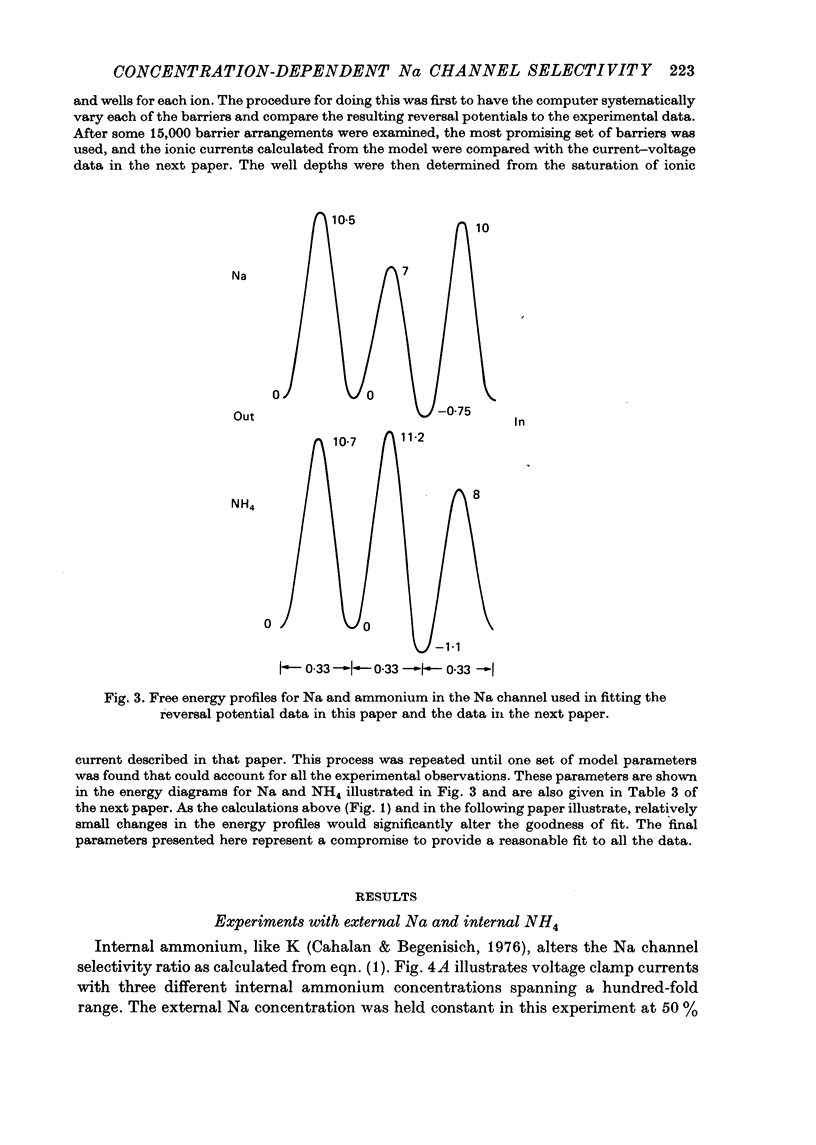
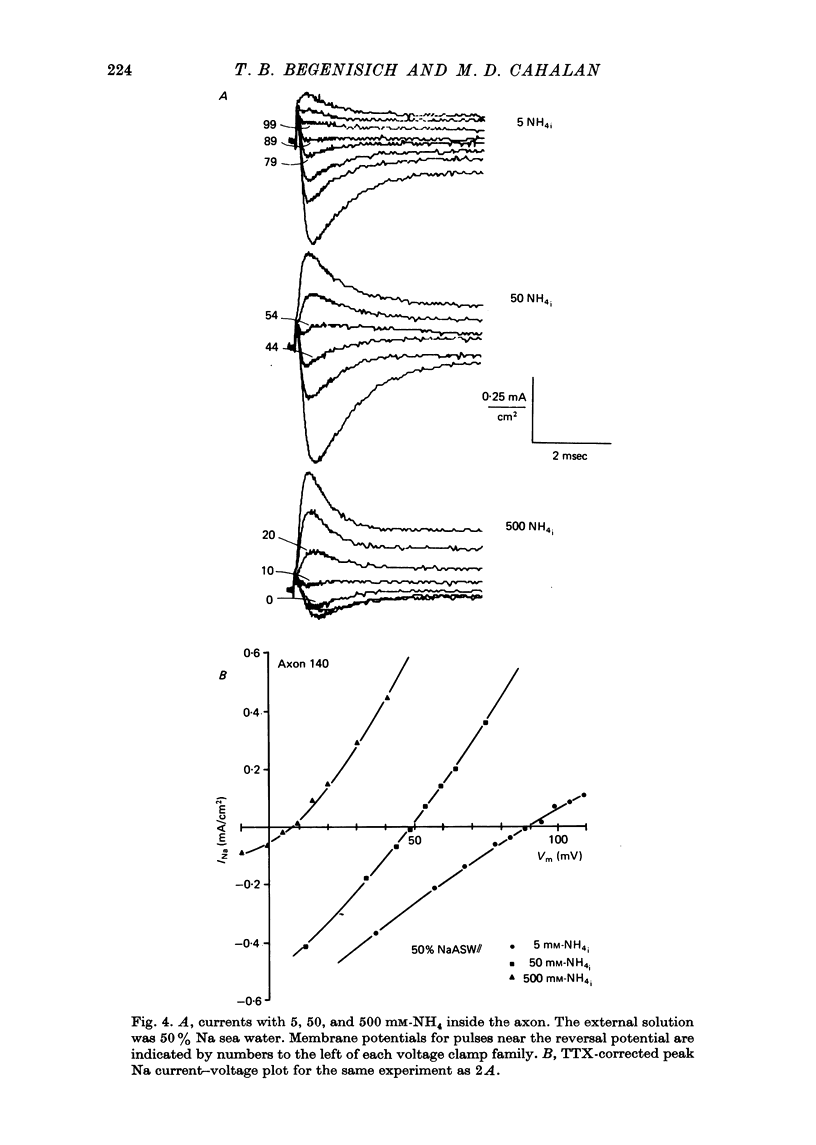
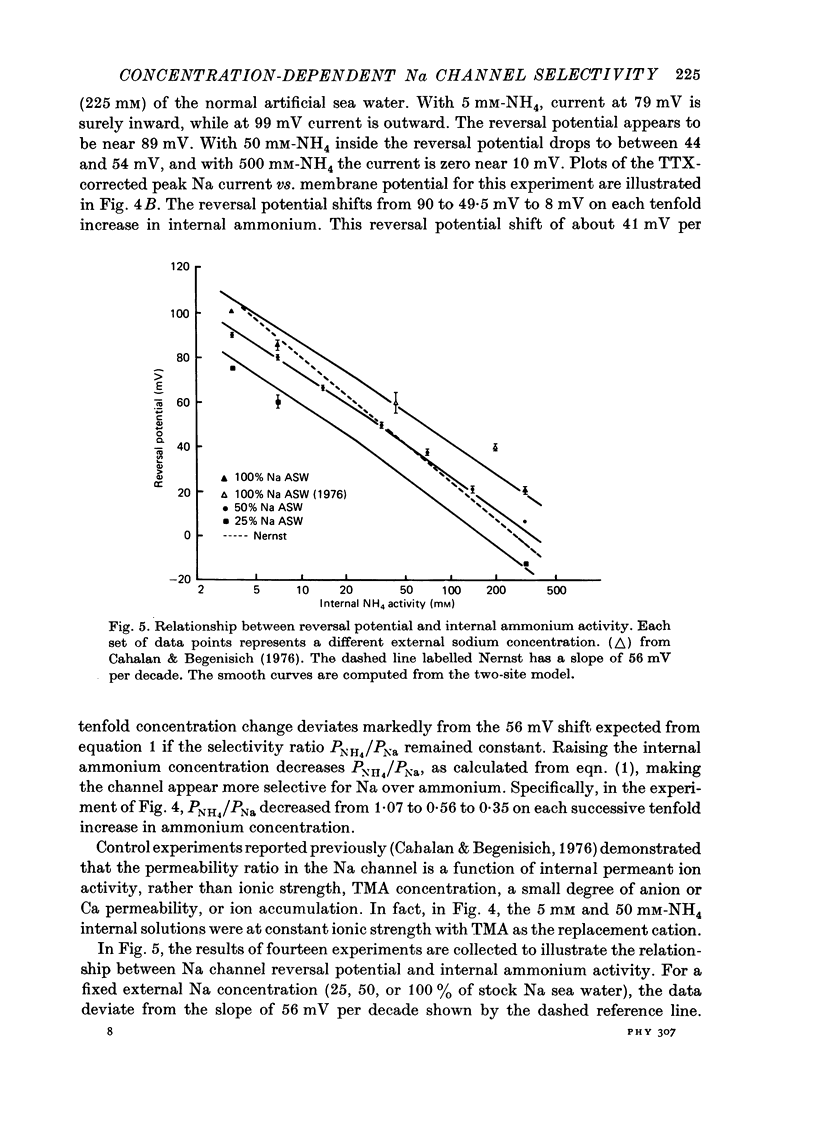
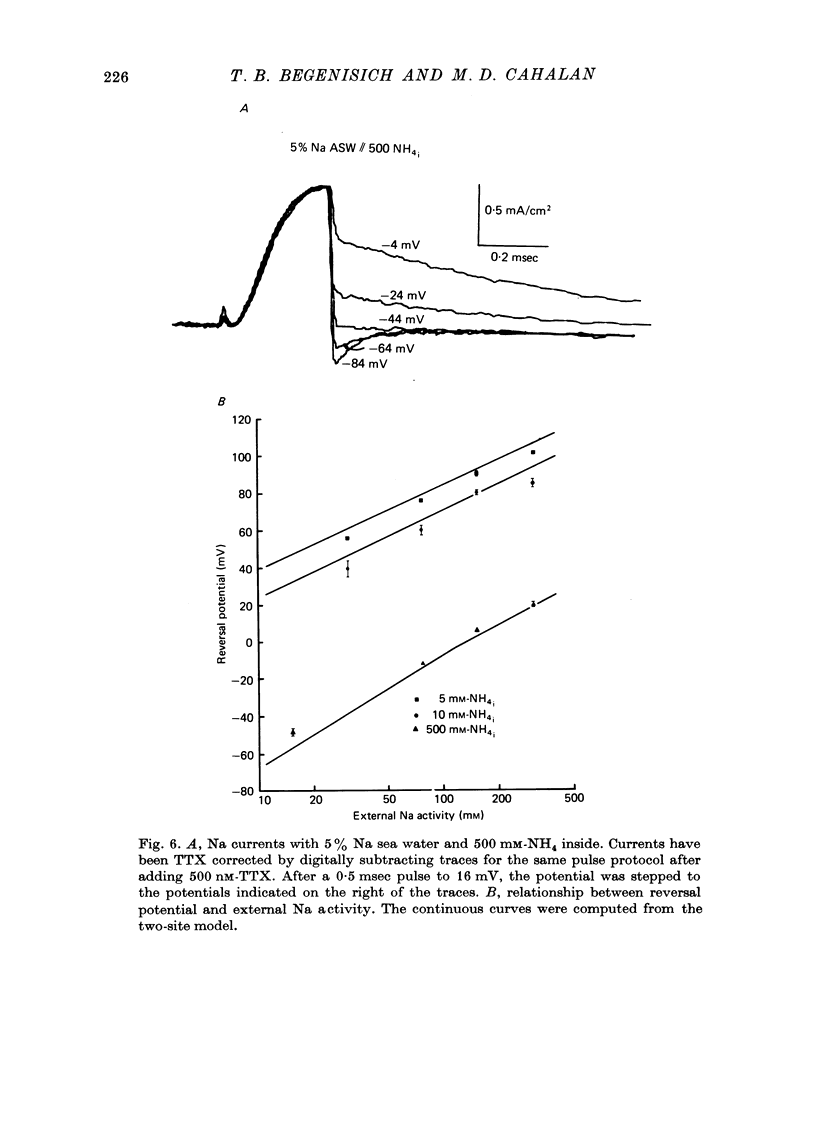
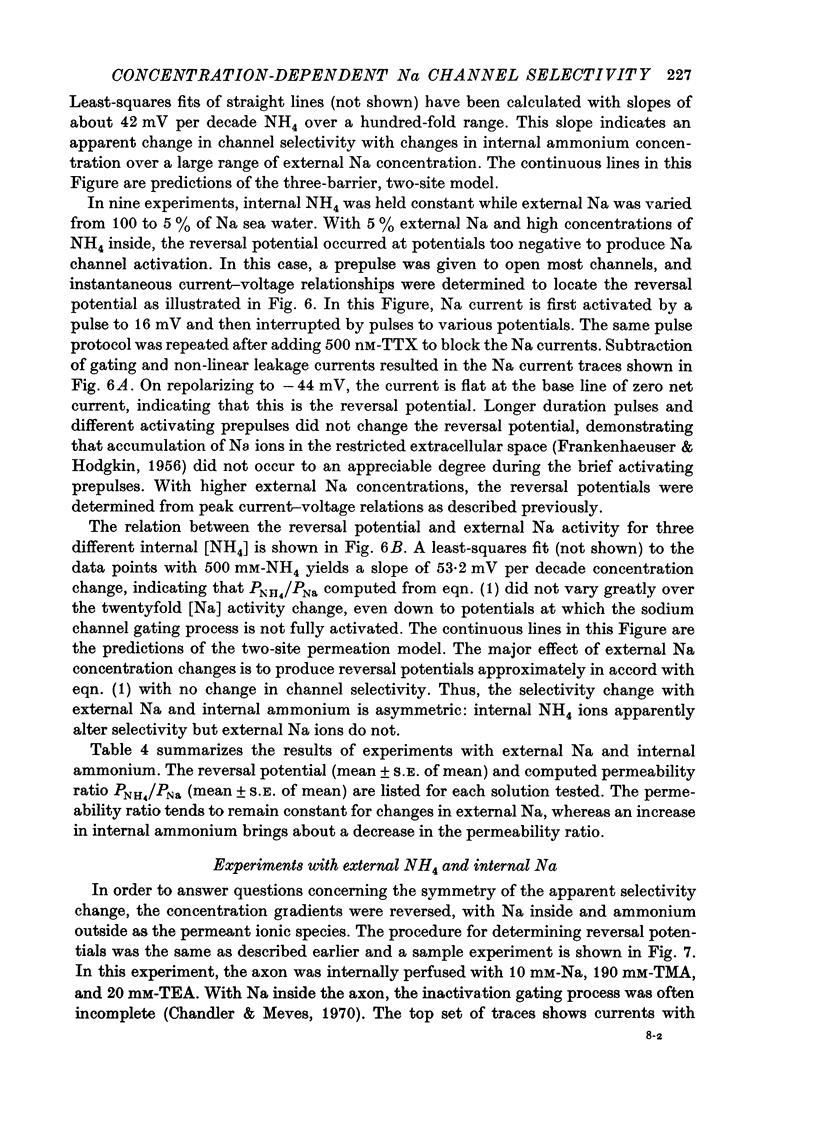
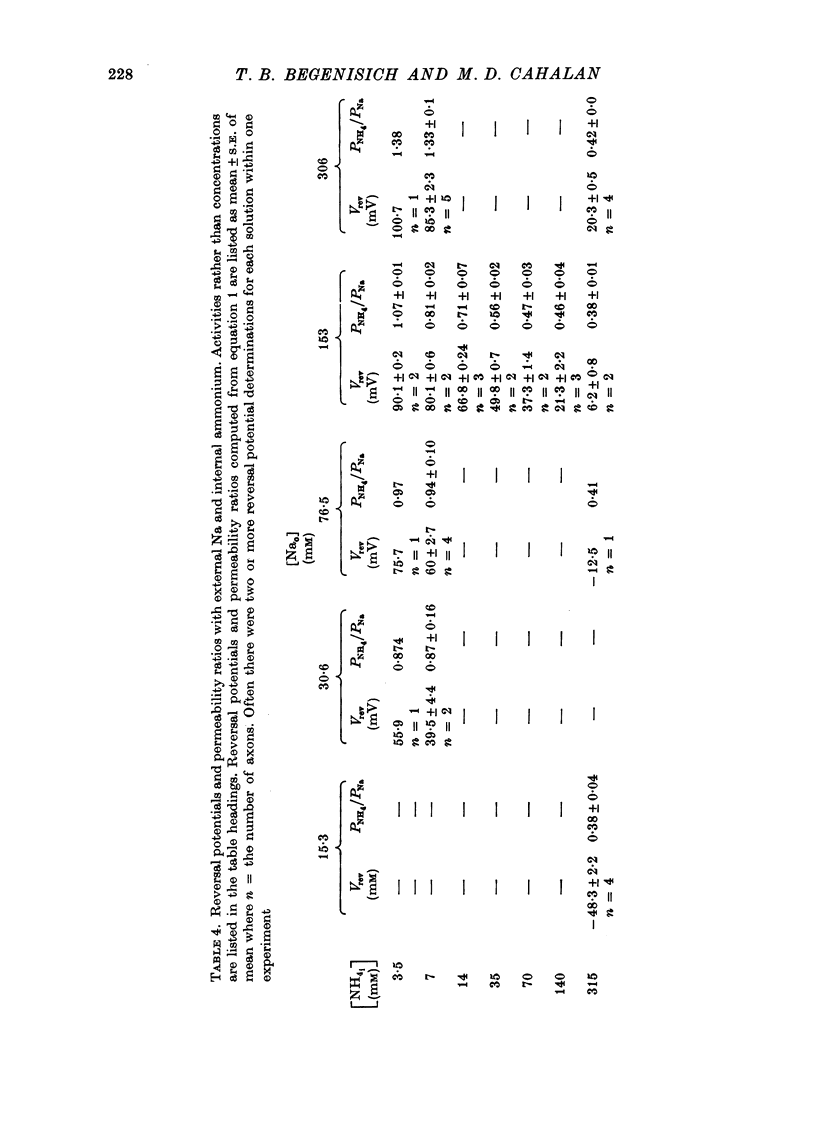
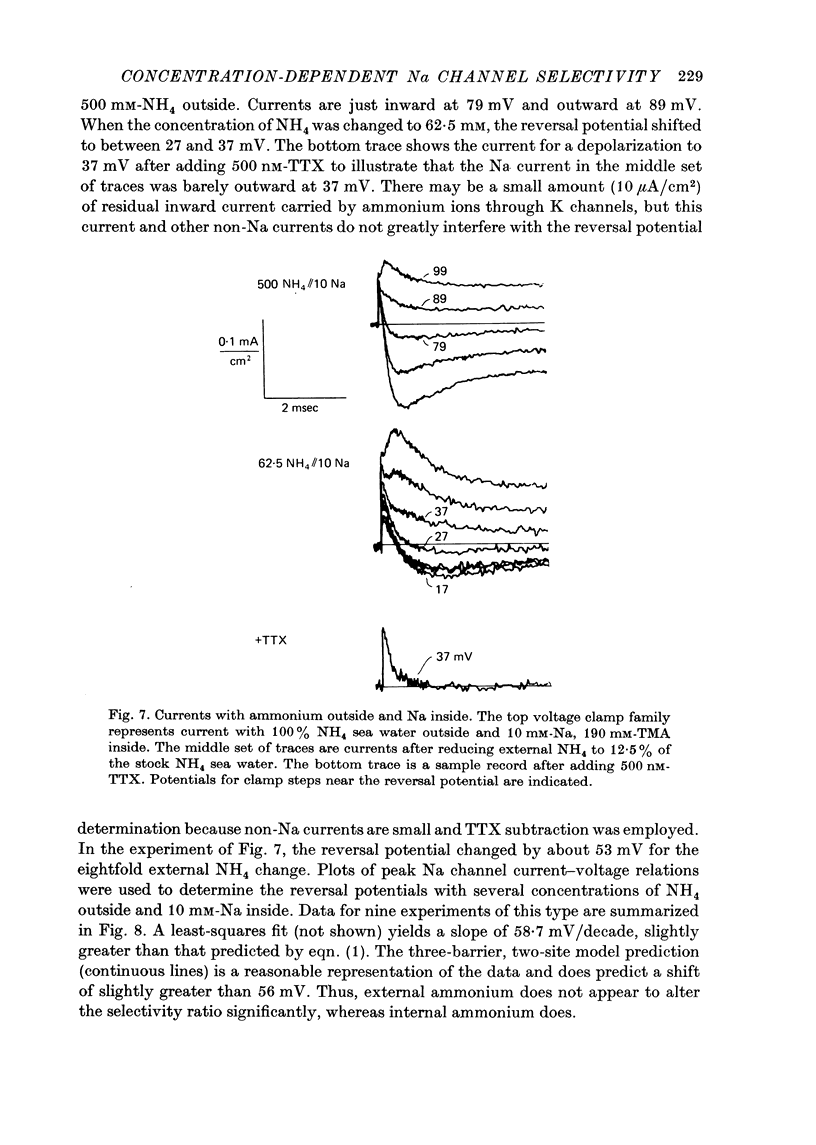
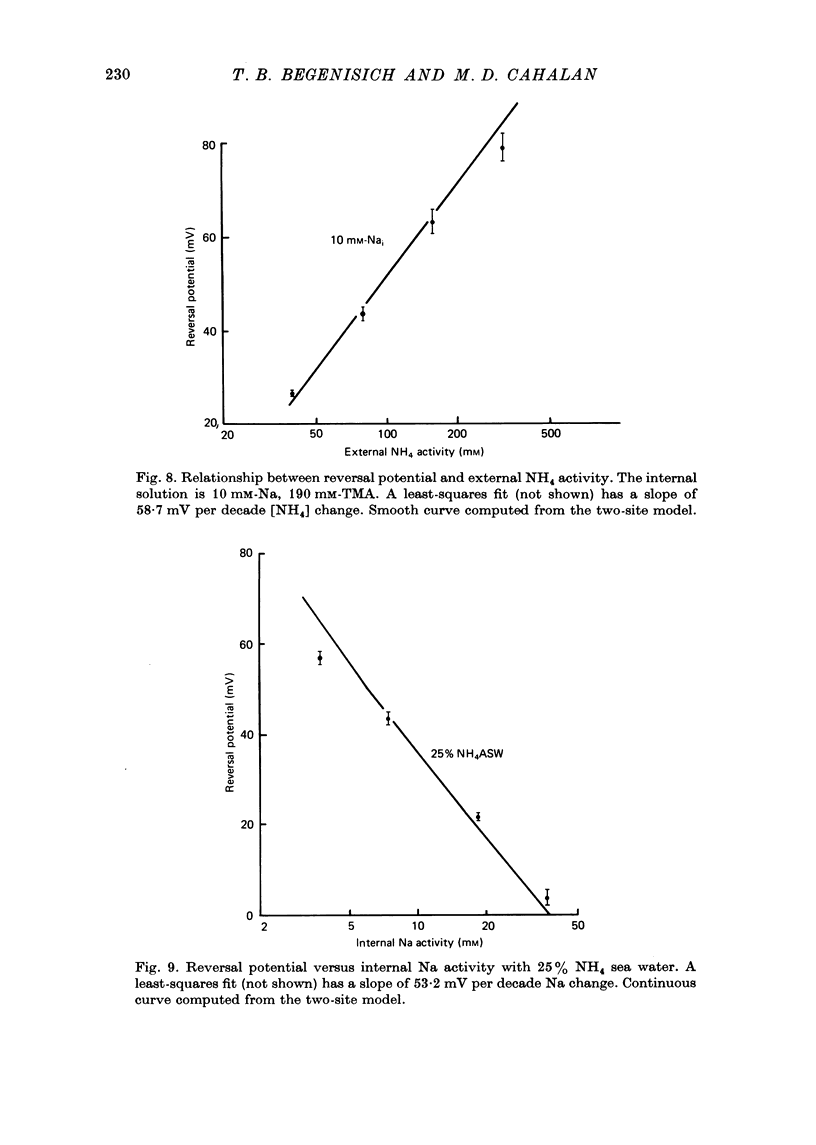
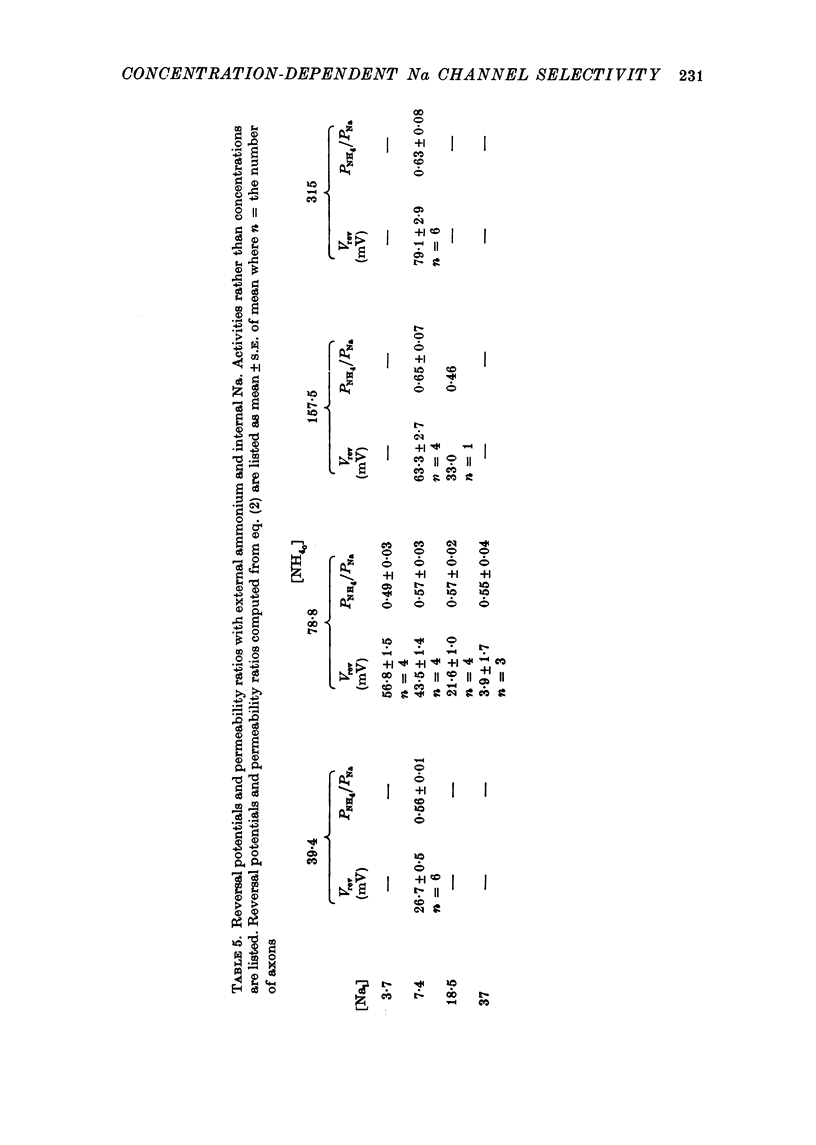
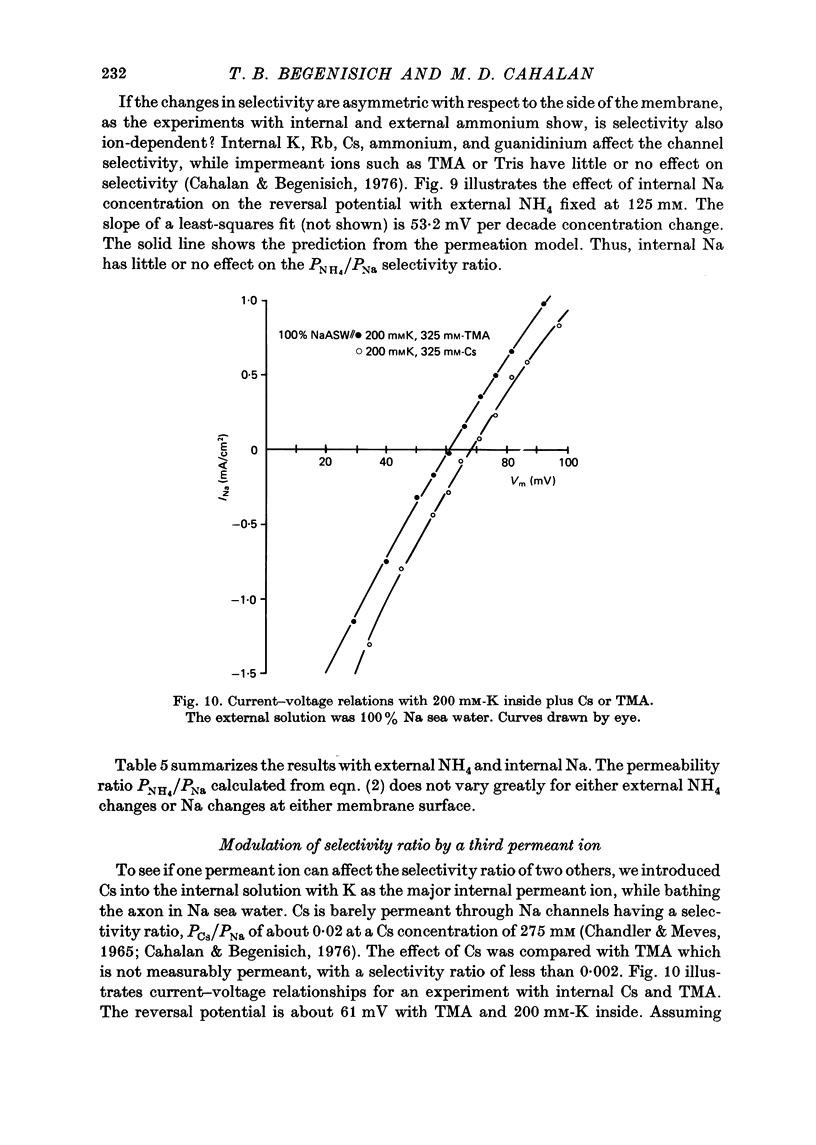
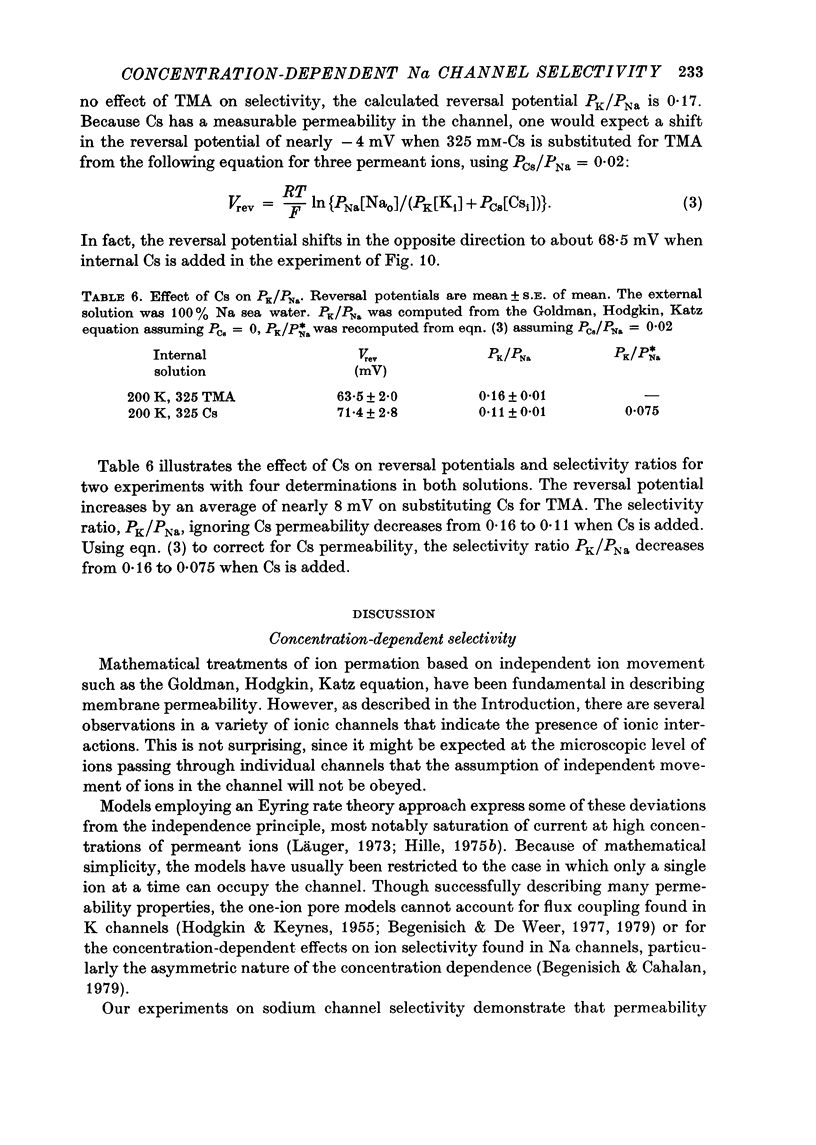
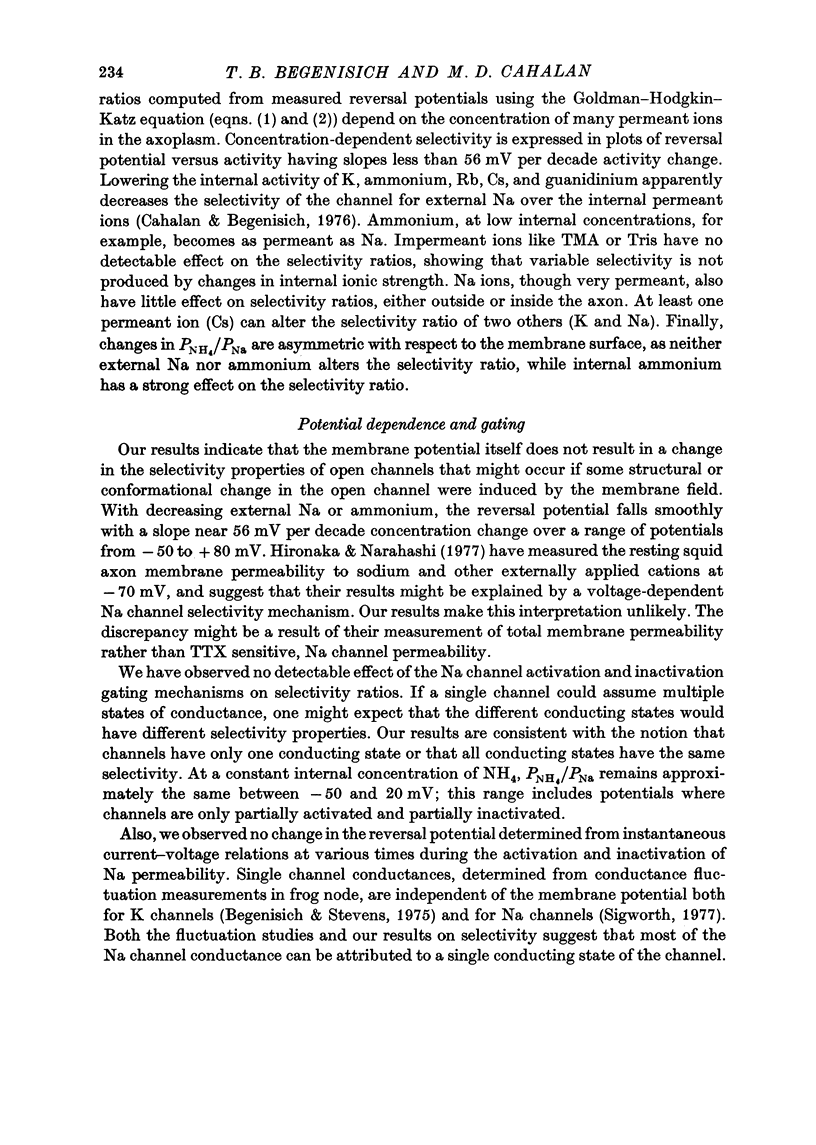
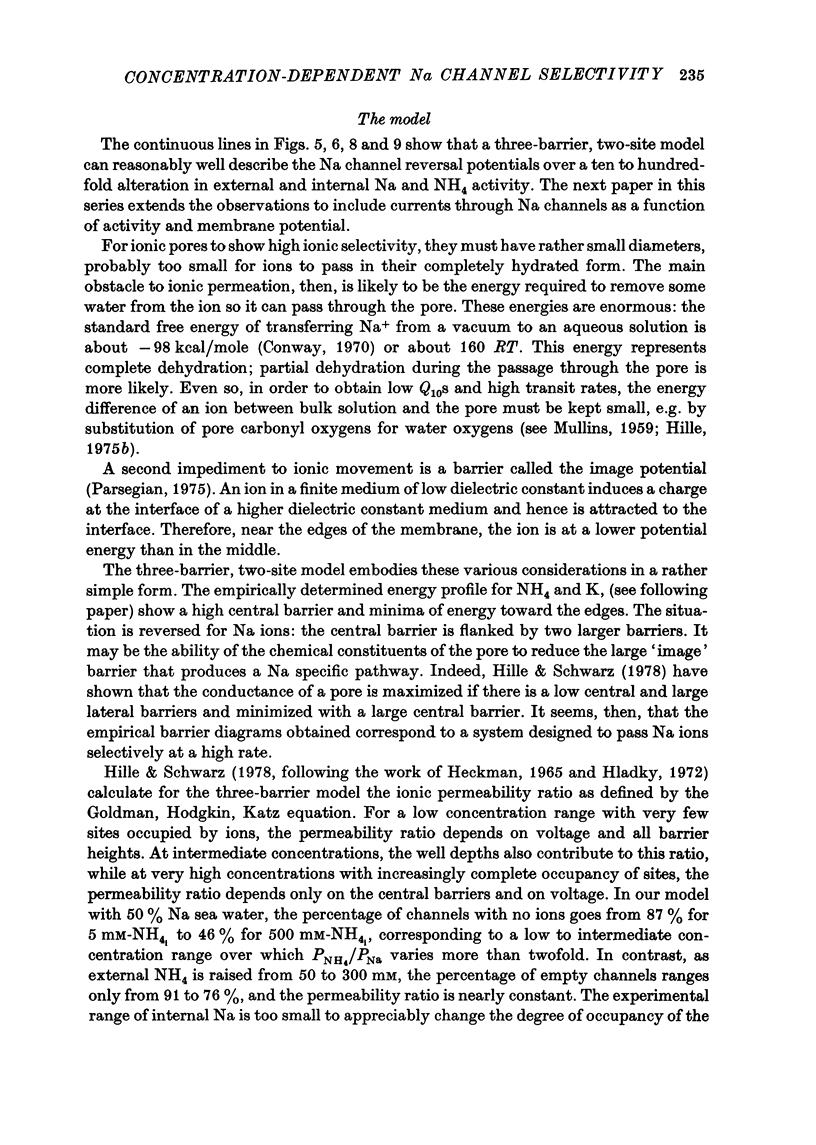
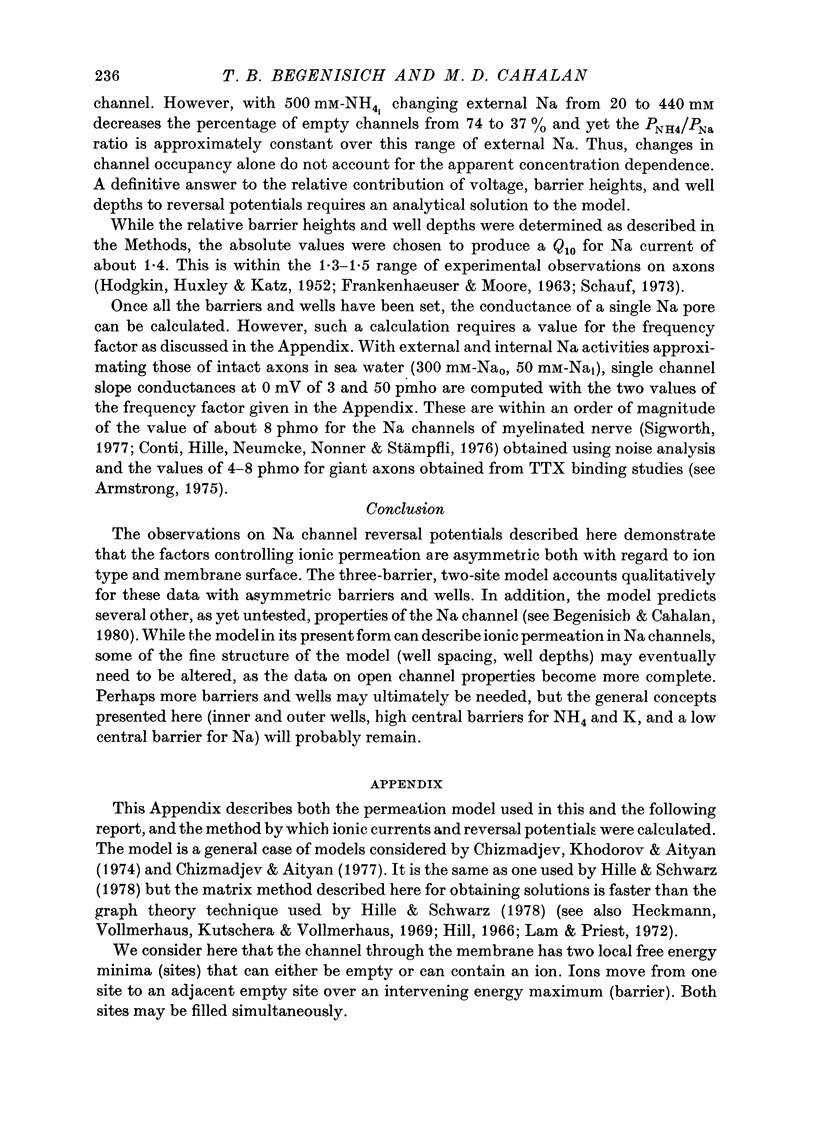
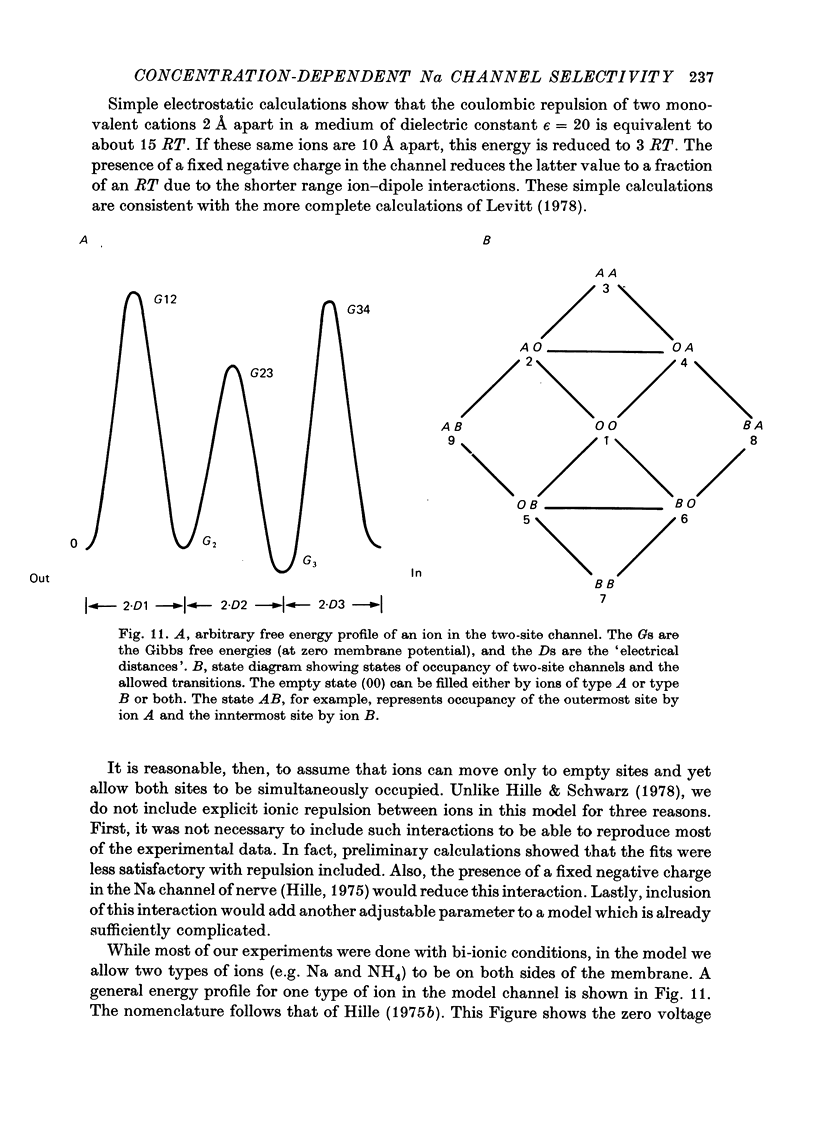
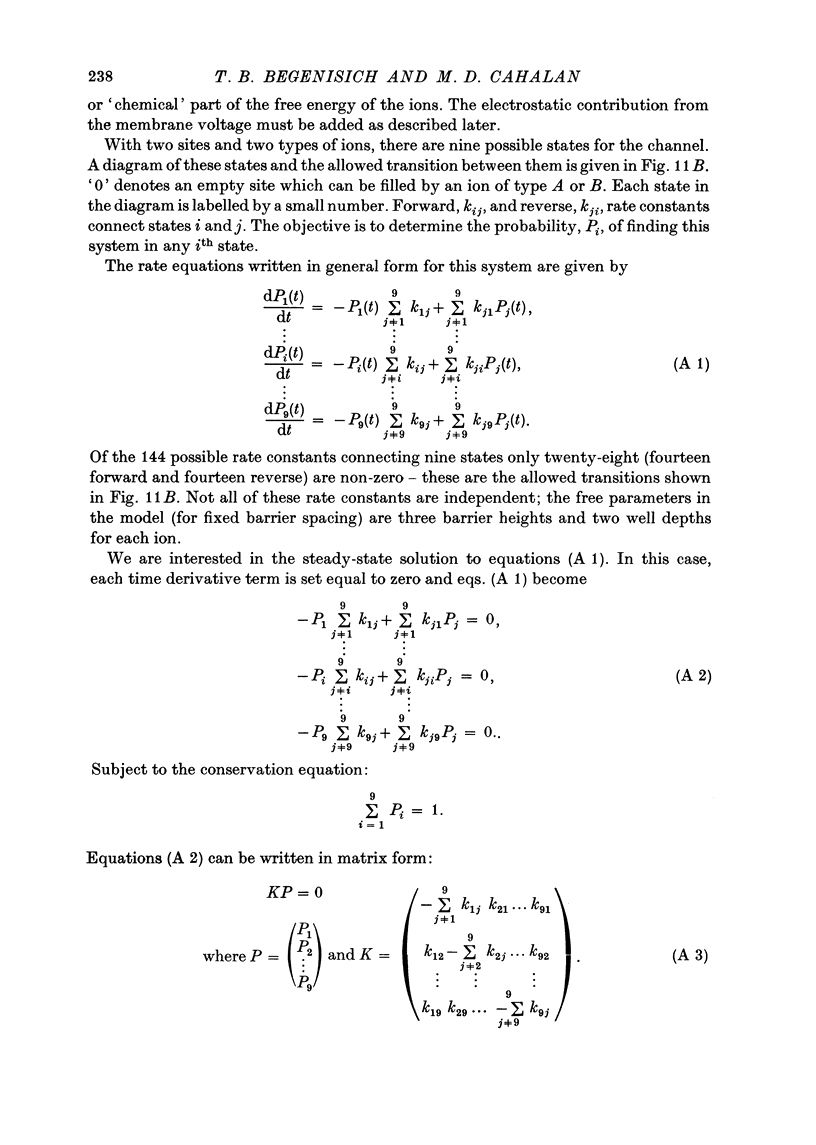
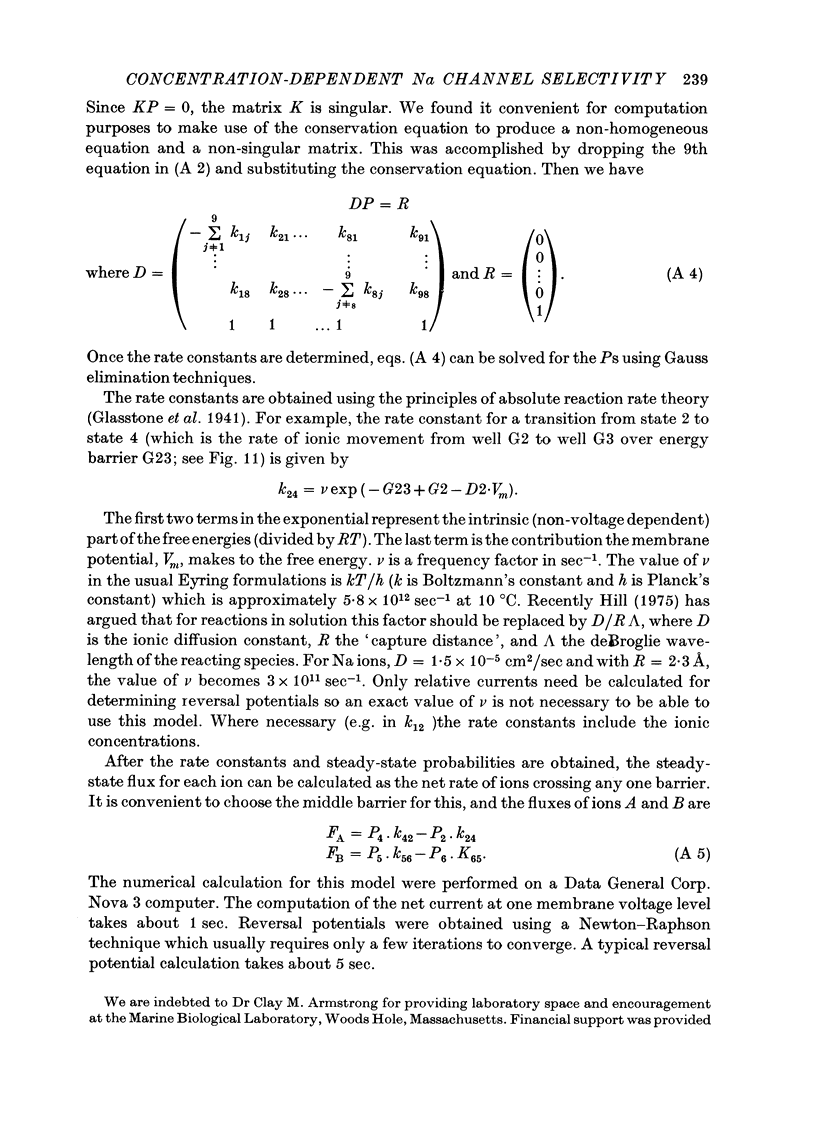
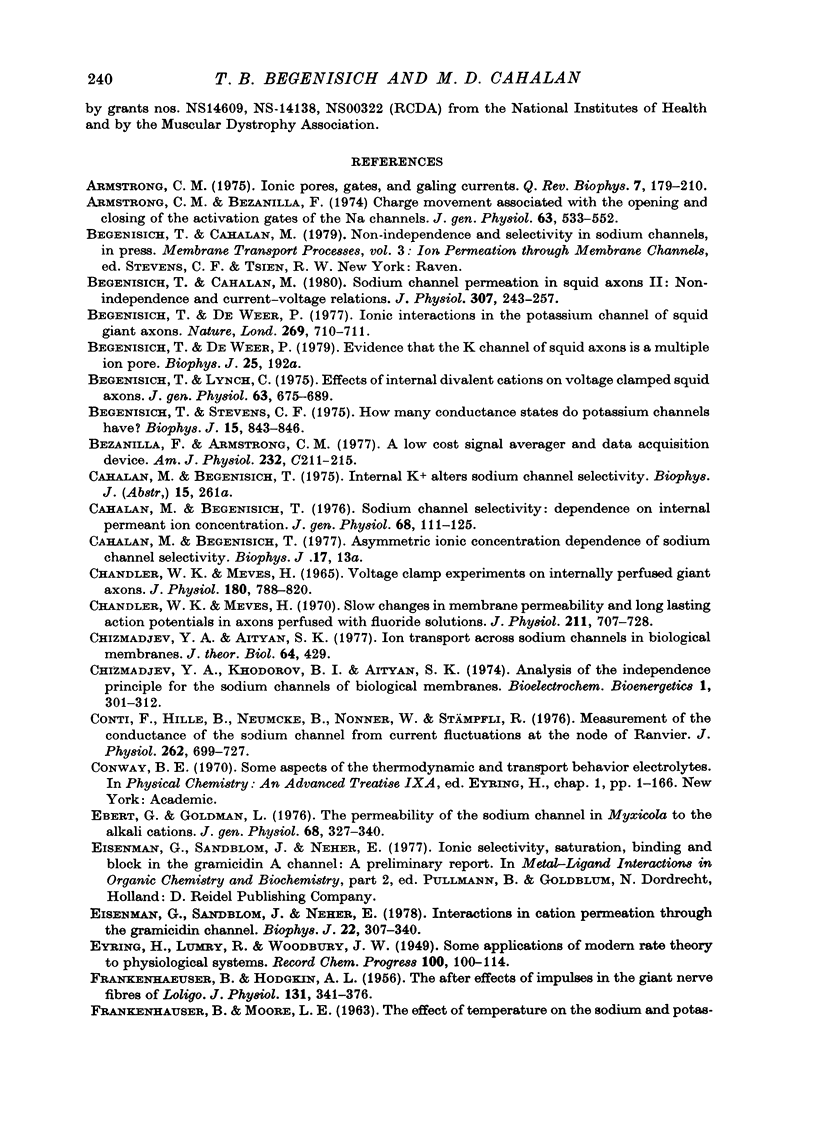
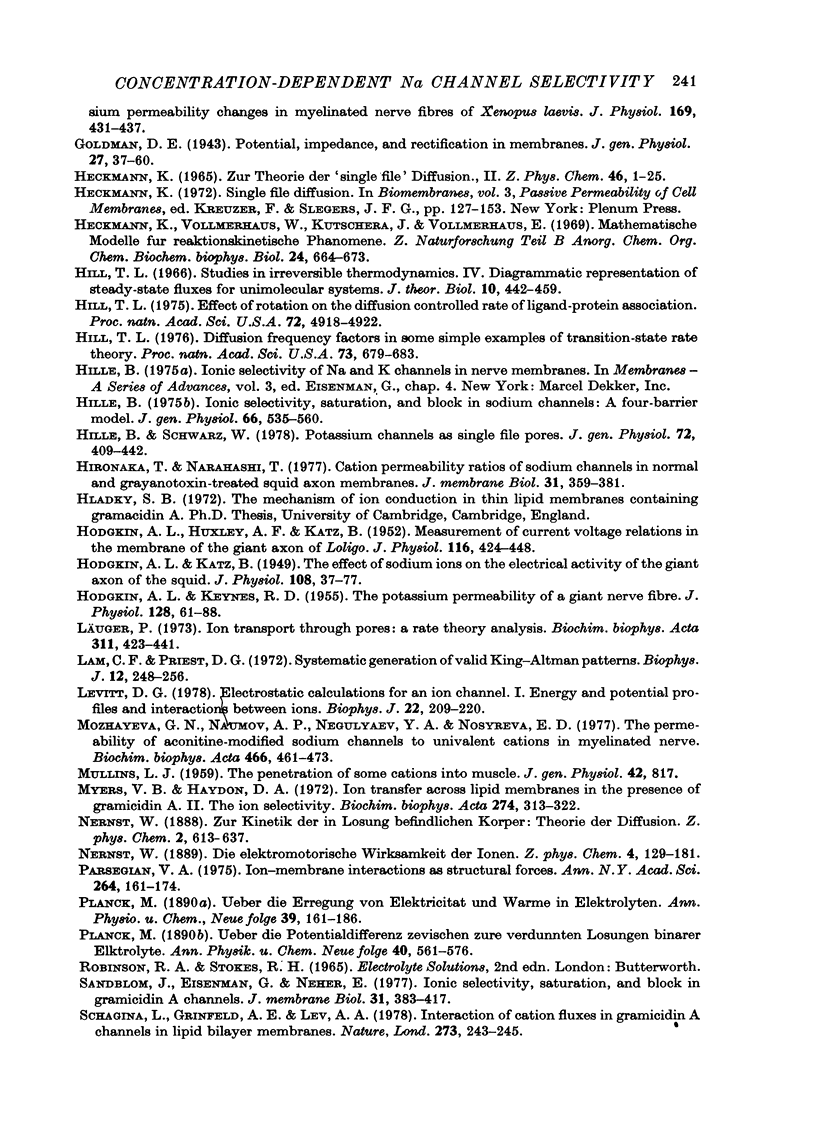
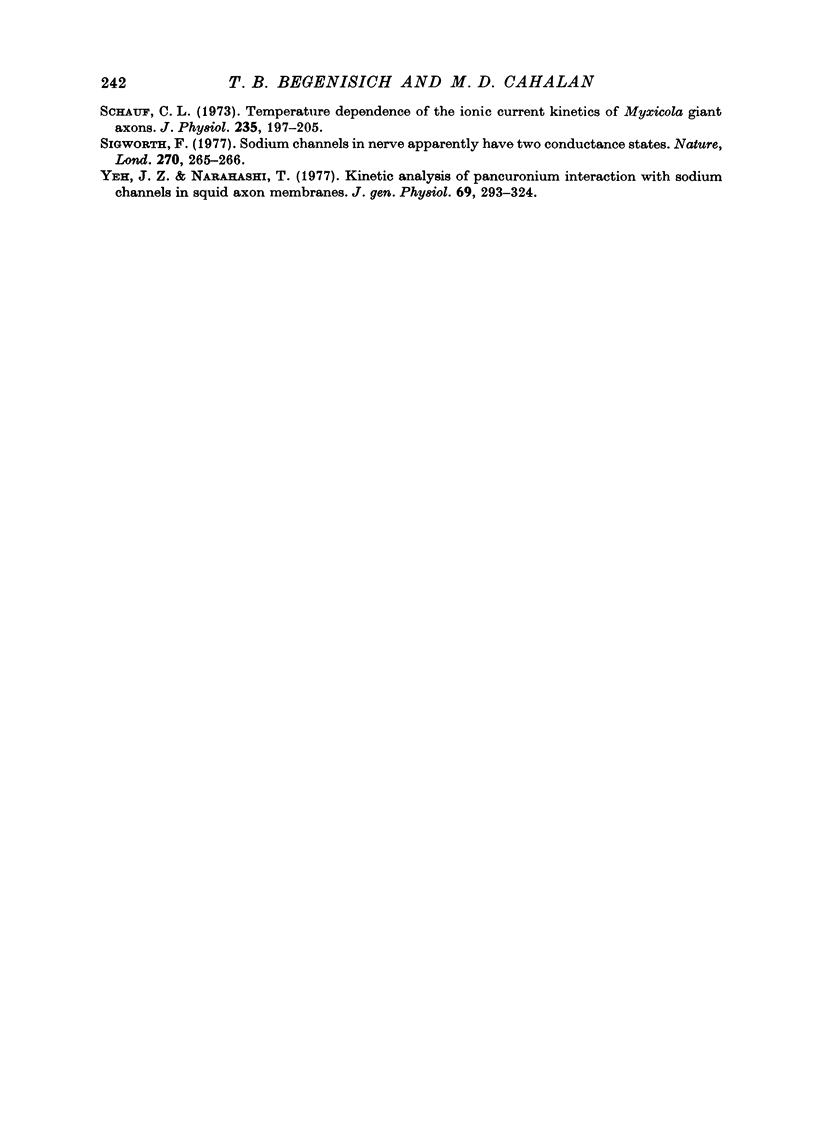
Selected References
These references are in PubMed. This may not be the complete list of references from this article.
- Armstrong C. M., Bezanilla F. Charge movement associated with the opening and closing of the activation gates of the Na channels. J Gen Physiol. 1974 May;63(5):533–552. doi: 10.1085/jgp.63.5.533. [DOI] [PMC free article] [PubMed] [Google Scholar]
- Armstrong C. M. Ionic pores, gates, and gating currents. Q Rev Biophys. 1974 May;7(2):179–210. doi: 10.1017/s0033583500001402. [DOI] [PubMed] [Google Scholar]
- Begenisich T. B., Cahalan M. D. Sodium channel permeation in squid axons. II: Non-independence and current-voltage relations. J Physiol. 1980 Oct;307:243–257. doi: 10.1113/jphysiol.1980.sp013433. [DOI] [PMC free article] [PubMed] [Google Scholar]
- Begenisich T., De Weer P. Ionic interactions in the potassium channel of squid giant axons. Nature. 1977 Oct 20;269(5630):710–711. doi: 10.1038/269710a0. [DOI] [PubMed] [Google Scholar]
- Begenisich T., Lynch C. Effects of internal divalent cations on voltage-clamped squid axons. J Gen Physiol. 1974 Jun;63(6):675–689. doi: 10.1085/jgp.63.6.675. [DOI] [PMC free article] [PubMed] [Google Scholar]
- Begenisich T., Stevens C. F. How many conductance states do potassium channels have? Biophys J. 1975 Aug;15(8):843–846. doi: 10.1016/S0006-3495(75)85858-9. [DOI] [PMC free article] [PubMed] [Google Scholar]
- Bezanilla F., Armstrong C. M. A low-cost signal averager and data-acquisition device. Am J Physiol. 1977 May;232(5):C211–C215. doi: 10.1152/ajpcell.1977.232.5.C211. [DOI] [PubMed] [Google Scholar]
- Cahalan M., Begenisich T. Sodium channel selectivity. Dependence on internal permeant ion concentration. J Gen Physiol. 1976 Aug;68(2):111–125. doi: 10.1085/jgp.68.2.111. [DOI] [PMC free article] [PubMed] [Google Scholar]
- Chandler W. K., Meves H. Slow changes in membrane permeability and long-lasting action potentials in axons perfused with fluoride solutions. J Physiol. 1970 Dec;211(3):707–728. doi: 10.1113/jphysiol.1970.sp009300. [DOI] [PMC free article] [PubMed] [Google Scholar]
- Chandler W. K., Meves H. Voltage clamp experiments on internally perfused giant axons. J Physiol. 1965 Oct;180(4):788–820. doi: 10.1113/jphysiol.1965.sp007732. [DOI] [PMC free article] [PubMed] [Google Scholar]
- Chizmadjev Y. A., Aityan S. K. Ion transport across sodium channels in biological membranes. J Theor Biol. 1977 Feb 7;64(3):429–453. doi: 10.1016/0022-5193(77)90281-8. [DOI] [PubMed] [Google Scholar]
- Conti F., Hille B., Neumcke B., Nonner W., Stämpfli R. Measurement of the conductance of the sodium channel from current fluctuations at the node of Ranvier. J Physiol. 1976 Nov;262(3):699–727. doi: 10.1113/jphysiol.1976.sp011616. [DOI] [PMC free article] [PubMed] [Google Scholar]
- Ebert G. A., Goldman L. The permeability of the sodium channel in Myxicola to the alkali cations. J Gen Physiol. 1976 Sep;68(3):327–340. doi: 10.1085/jgp.68.3.327. [DOI] [PMC free article] [PubMed] [Google Scholar]
- Eisenman G., Sandblom J., Neher E. Interactions in cation permeation through the gramicidin channel. Cs, Rb, K, Na, Li, Tl, H, and effects of anion binding. Biophys J. 1978 May;22(2):307–340. doi: 10.1016/S0006-3495(78)85491-5. [DOI] [PMC free article] [PubMed] [Google Scholar]
- FRANKENHAEUSER B., HODGKIN A. L. The after-effects of impulses in the giant nerve fibres of Loligo. J Physiol. 1956 Feb 28;131(2):341–376. doi: 10.1113/jphysiol.1956.sp005467. [DOI] [PMC free article] [PubMed] [Google Scholar]
- FRANKENHAEUSER B., MOORE L. E. THE EFFECT OF TEMPERATURE ON THE SODIUM AND POTASSIUM PERMEABILITY CHANGES IN MYELINATED NERVE FIBRES OF XENOPUS LAEVIS. J Physiol. 1963 Nov;169:431–437. doi: 10.1113/jphysiol.1963.sp007269. [DOI] [PMC free article] [PubMed] [Google Scholar]
- Goldman D. E. POTENTIAL, IMPEDANCE, AND RECTIFICATION IN MEMBRANES. J Gen Physiol. 1943 Sep 20;27(1):37–60. doi: 10.1085/jgp.27.1.37. [DOI] [PMC free article] [PubMed] [Google Scholar]
- HODGKIN A. L., HUXLEY A. F., KATZ B. Measurement of current-voltage relations in the membrane of the giant axon of Loligo. J Physiol. 1952 Apr;116(4):424–448. doi: 10.1113/jphysiol.1952.sp004716. [DOI] [PMC free article] [PubMed] [Google Scholar]
- HODGKIN A. L., KATZ B. The effect of sodium ions on the electrical activity of giant axon of the squid. J Physiol. 1949 Mar 1;108(1):37–77. doi: 10.1113/jphysiol.1949.sp004310. [DOI] [PMC free article] [PubMed] [Google Scholar]
- HODGKIN A. L., KEYNES R. D. The potassium permeability of a giant nerve fibre. J Physiol. 1955 Apr 28;128(1):61–88. doi: 10.1113/jphysiol.1955.sp005291. [DOI] [PMC free article] [PubMed] [Google Scholar]
- Heckmann K. Single file diffusion. Biomembranes. 1972;3:127–153. doi: 10.1007/978-1-4684-0961-1_9. [DOI] [PubMed] [Google Scholar]
- Hill T. L. Diffusion frequency factors in some simple examples of transition-state rate theory. Proc Natl Acad Sci U S A. 1976 Mar;73(3):679–683. doi: 10.1073/pnas.73.3.679. [DOI] [PMC free article] [PubMed] [Google Scholar]
- Hill T. L. Studies in irreversible thermodynamics. IV. Diagrammatic representation of steady state fluxes for unimolecular systems. J Theor Biol. 1966 Apr;10(3):442–459. doi: 10.1016/0022-5193(66)90137-8. [DOI] [PubMed] [Google Scholar]
- Hille B. Ionic selectivity, saturation, and block in sodium channels. A four-barrier model. J Gen Physiol. 1975 Nov;66(5):535–560. doi: 10.1085/jgp.66.5.535. [DOI] [PMC free article] [PubMed] [Google Scholar]
- Hille B., Schwarz W. Potassium channels as multi-ion single-file pores. J Gen Physiol. 1978 Oct;72(4):409–442. doi: 10.1085/jgp.72.4.409. [DOI] [PMC free article] [PubMed] [Google Scholar]
- Hironaka T., Narahashi T. Cation permeability ratios of sodium channels in normal and grayanotoxin-treated squid axon membranes. J Membr Biol. 1977 Mar 23;31(4):359–381. doi: 10.1007/BF01869413. [DOI] [PubMed] [Google Scholar]
- Lam C. F., Priest D. G. Enzyme kinetics. Systematic generation of valid King-Altman patterns. Biophys J. 1972 Mar;12(3):248–256. doi: 10.1016/S0006-3495(72)86084-3. [DOI] [PMC free article] [PubMed] [Google Scholar]
- Läuger P. Ion transport through pores: a rate-theory analysis. Biochim Biophys Acta. 1973 Jul 6;311(3):423–441. doi: 10.1016/0005-2736(73)90323-4. [DOI] [PubMed] [Google Scholar]
- MULLINS Lj. The penetration of some cations into muscle. J Gen Physiol. 1959 Mar 20;42(4):817–829. doi: 10.1085/jgp.42.4.817. [DOI] [PMC free article] [PubMed] [Google Scholar]
- Mozhayeva G. N., Naumov A. P., Negulyaev Y. A., Nosyreva E. D. The permeability of aconitine-modified sodium channels to univalent cations in myelinated nerve. Biochim Biophys Acta. 1977 May 2;466(3):461–473. doi: 10.1016/0005-2736(77)90339-x. [DOI] [PubMed] [Google Scholar]
- Myers V. B., Haydon D. A. Ion transfer across lipid membranes in the presence of gramicidin A. II. The ion selectivity. Biochim Biophys Acta. 1972 Aug 9;274(2):313–322. doi: 10.1016/0005-2736(72)90179-4. [DOI] [PubMed] [Google Scholar]
- Parsegian V. A. Ion-membrane interactions as structural forces. Ann N Y Acad Sci. 1975 Dec 30;264:161–171. doi: 10.1111/j.1749-6632.1975.tb31481.x. [DOI] [PubMed] [Google Scholar]
- Sandblom J., Eisenman G., Neher E. Ionic selectivity, saturation and block in gramicidin A channels: I. Theory for the electrical properties of ion selective channels having two pairs of binding sites and multiple conductance states. J Membr Biol. 1977 Mar 23;31(4):383–347. doi: 10.1007/BF01869414. [DOI] [PubMed] [Google Scholar]
- Schagina L. V., Grinfeldt A. E., Lev A. A. Interaction of cation fluxes in gramicidin A channels in lipid bilayer membranes. Nature. 1978 May 18;273(5659):243–245. doi: 10.1038/273243a0. [DOI] [PubMed] [Google Scholar]
- Schauf C. L. Temperature dependence of the ionic current kinetics of Myxicola giant axons. J Physiol. 1973 Nov;235(1):197–205. doi: 10.1113/jphysiol.1973.sp010384. [DOI] [PMC free article] [PubMed] [Google Scholar]
- Sigworth F. J. Sodium channels in nerve apparently have two conductance states. Nature. 1977 Nov 17;270(5634):265–267. doi: 10.1038/270265a0. [DOI] [PubMed] [Google Scholar]
- Yeh J. Z., Narahashi T. Kinetic analysis of pancuronium interaction with sodium channels in squid axon membranes. J Gen Physiol. 1977 Mar;69(3):293–323. doi: 10.1085/jgp.69.3.293. [DOI] [PMC free article] [PubMed] [Google Scholar]