Abstract
1. Quick stretches and releases were applied to small bundles of glycerinated fibres of rabbit psoas and insect fibrillar flight muscle. The resulting tension changes were recorded at various temperatures and amplitudes of length change. The results from the two preparations had many features in common. At temperatures near 0 degrees C the asymmetry of the initial tension recovery after stretch and release originally reported in living frog fibres by Huxley & Simmons (1971 alpha) was very obvious. 2. The complete tension course could be described as an elastic change occurring simultaneously with the length change followed by recovery consisting of the sum of a number of exponential terms. These terms usually corresponded to the phases discernible without curve fitting, but in some cases a monotonic rise or fall of tension was seen to consist of two components only after curve fitting. 3. After either stretch or release there was a phase of rapid tension recovery towards the value before the length change. The rate constant of this phase increased as the amplitude of stretch or release was increased to about 2 nm/half sarcomere. At higher amplitudes it remained nearly constant 4. At temperatures near 0 degrees C there was a second and much slower continuation of the recovery after stretch. The rate constant of this second phase was much more sensitive to temperature than that of the first phase and it became slower with increasing amplitude of stretch. As the temperature was raised the speed of the second phase approached the speed of the first phase so that at room temperatures the initial tension recovery after stretch and release was nearly symmetrical. 5. Under many conditions these processes were followed by a change in the opposite direction, the 'delayed tension' described by earlier workers. This third phase of tension change had about the same temperature sensitivity as the second phase of the recovery seen after stretch. The tension due to stretch activation was not maintained in rabbit muscle, resulting in a fourth possible phase, a recovery of tension towards the value before the length change. This was absent or of low amplitude in insect flight muscle. 6. We interpret these tension changes on the basis of an extension of the non-linear model described by White & Thorson (1972). The elastic tension change and the initial fast recovery are both supposed to be properties of the attached cross-bridges, whilst the slower recovery is considered to be due to the detachment of cross-bridges which happened to be attached at the instant the length change was applied. The delayed tension reflects the approach to equilibrium of the number of attached bridges, changed by an effect of muscle length on the attachment rate. The fact that the delayed tension is not maintained in rabbit psoas muscle may be due to the effect of length on attachment rate being transitory.
Full text
PDF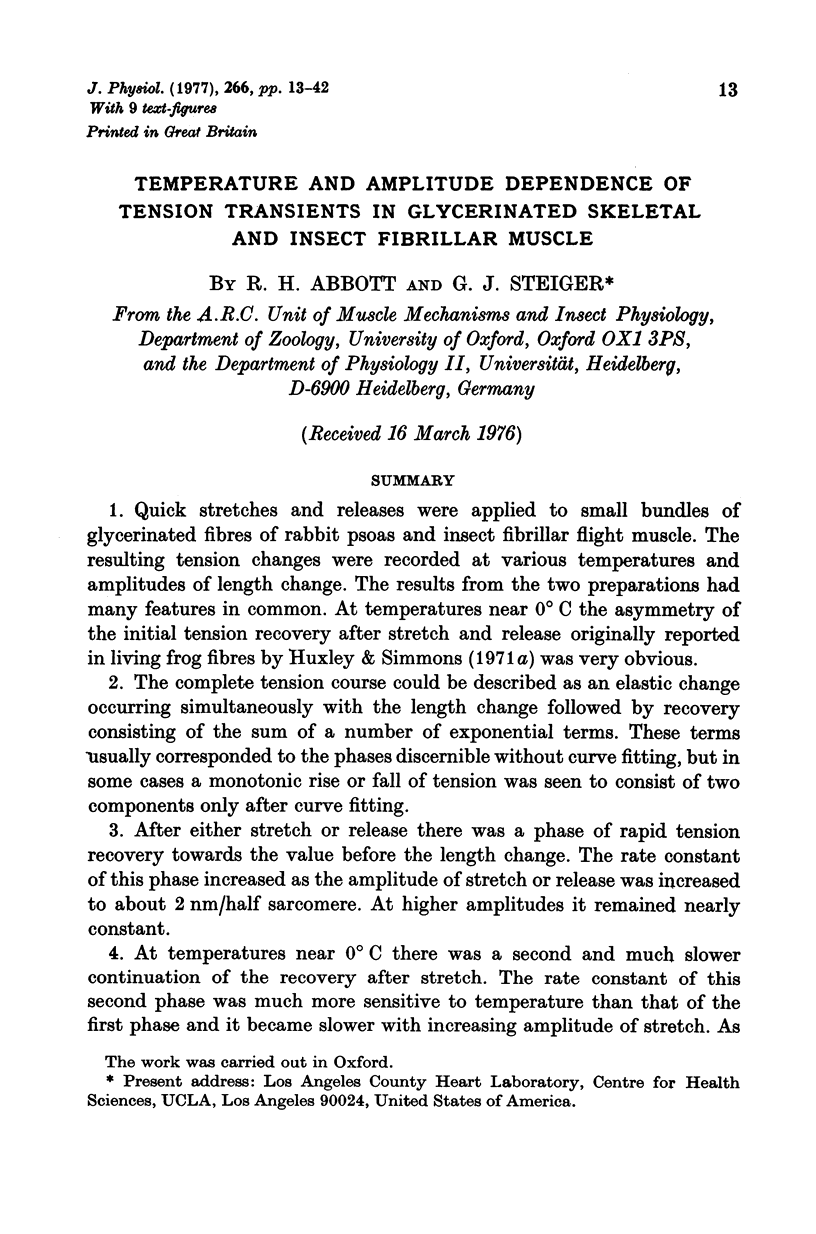
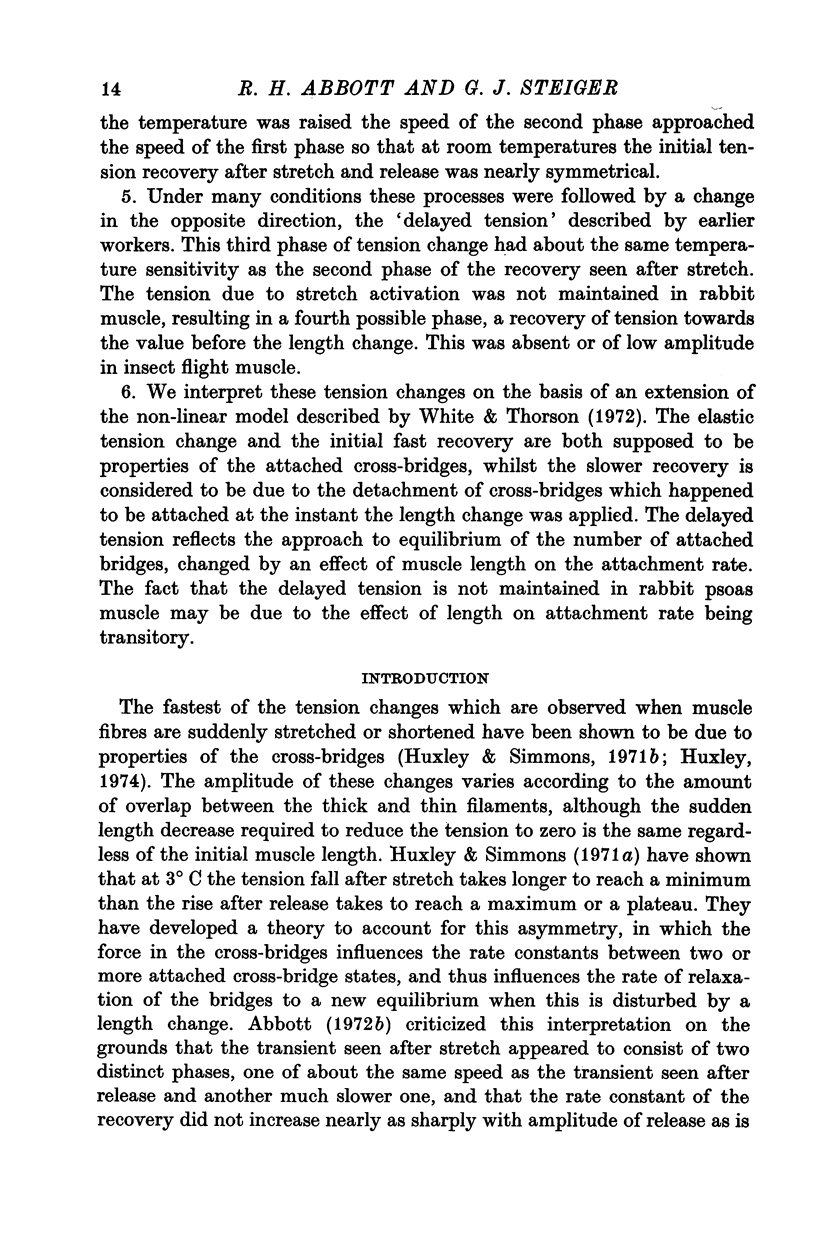
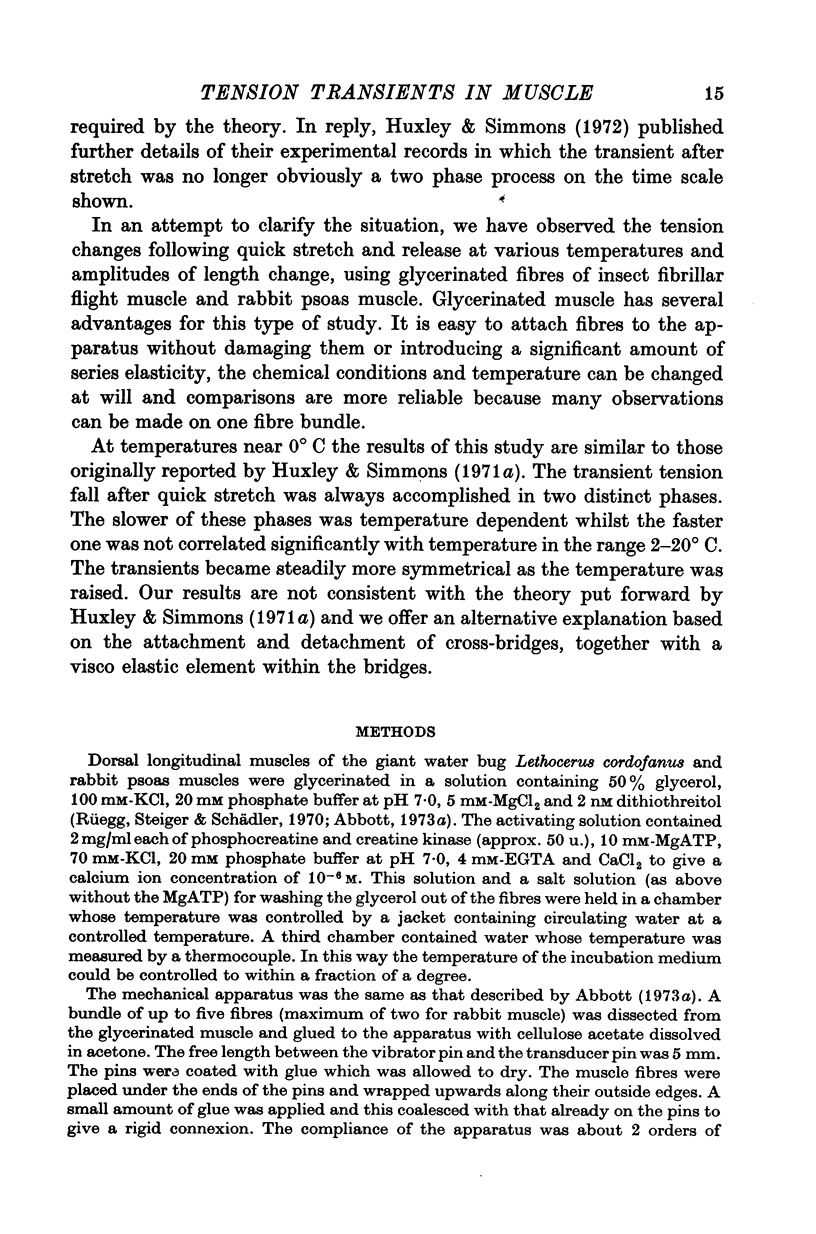
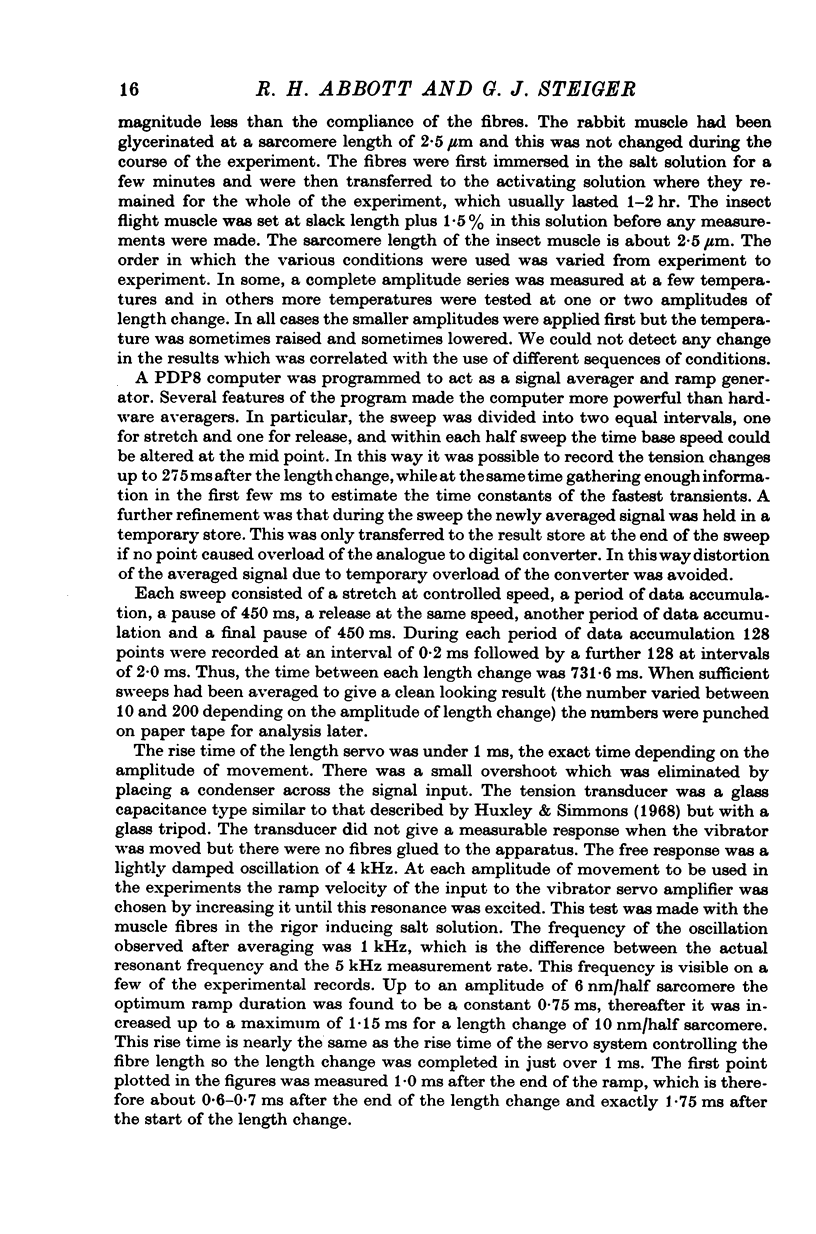
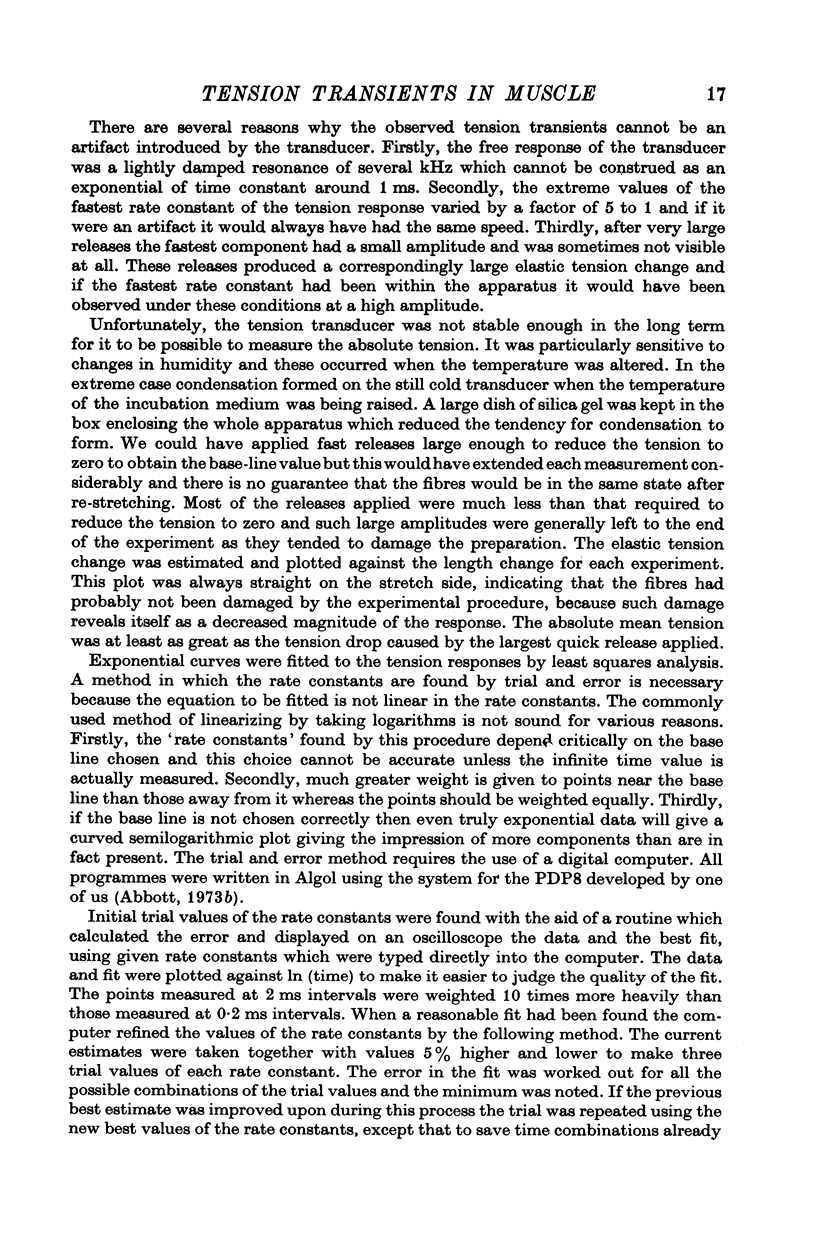
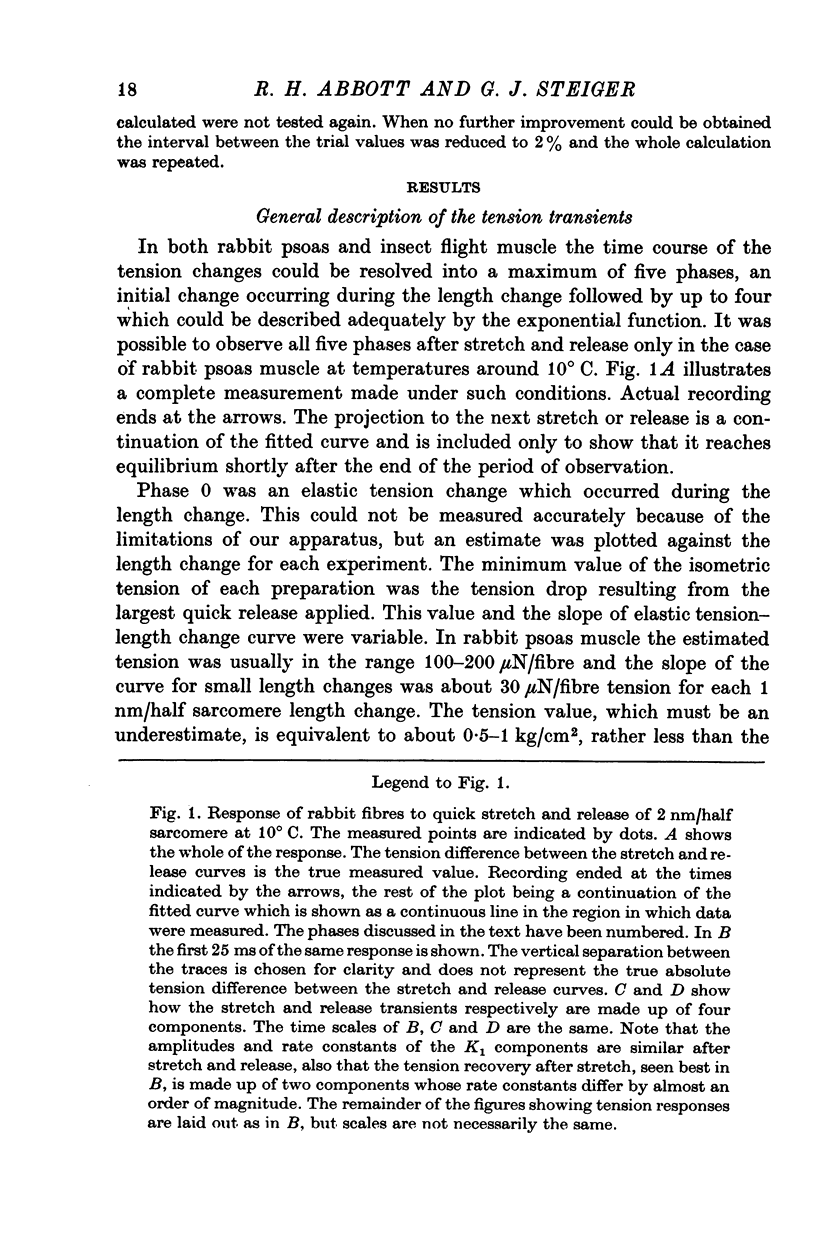
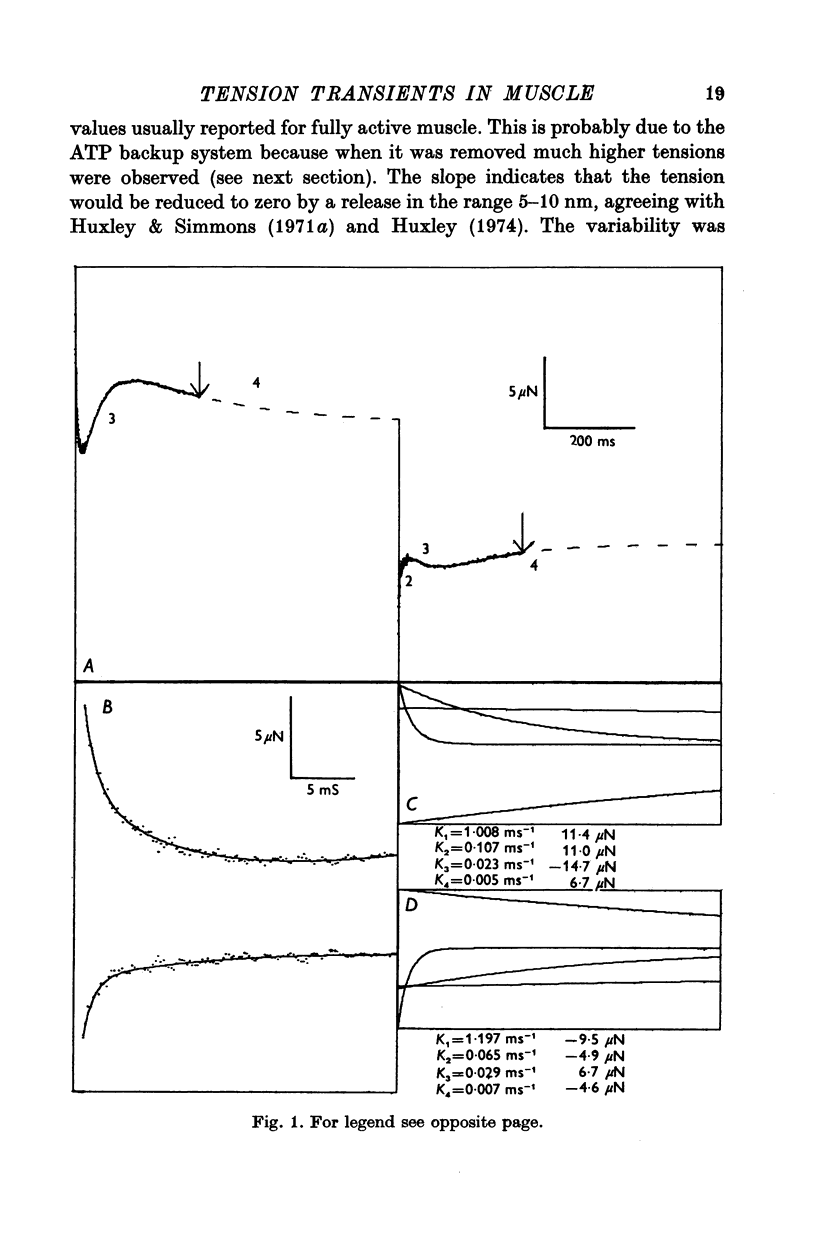
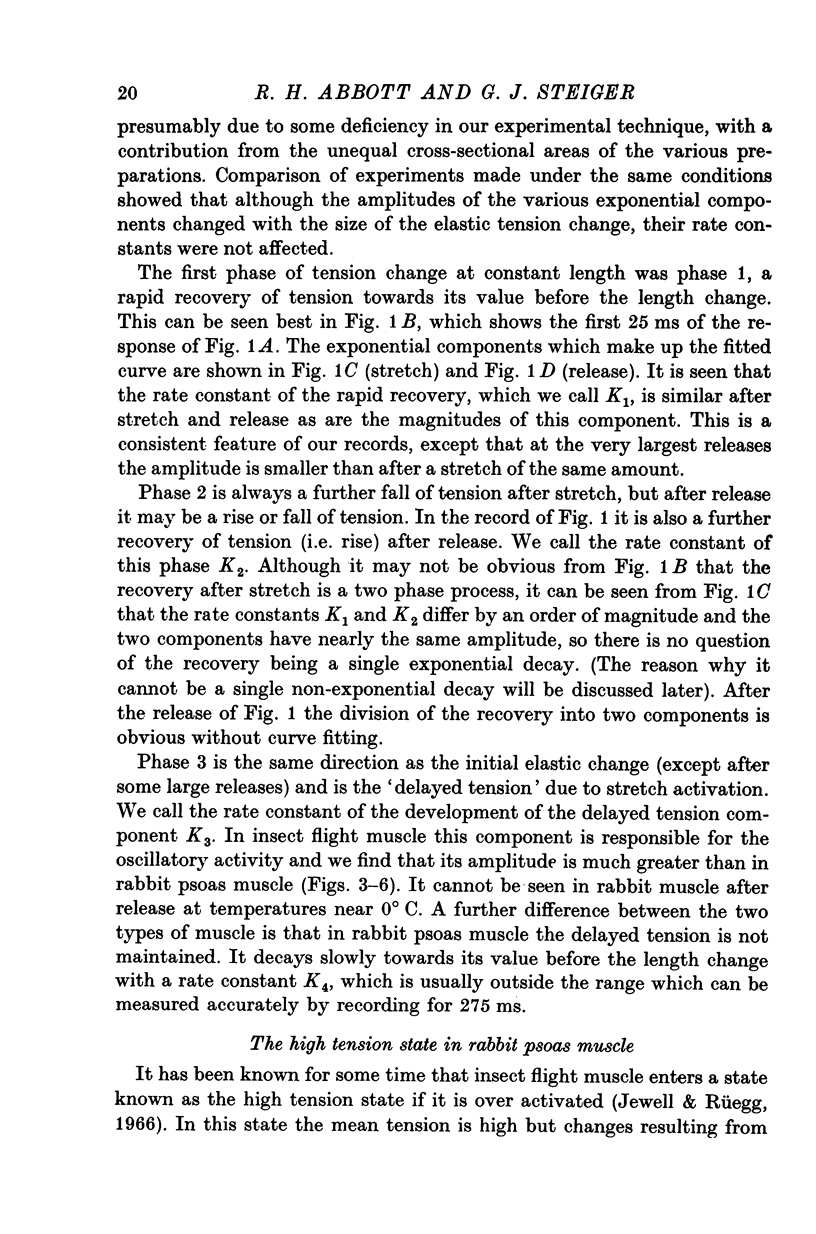
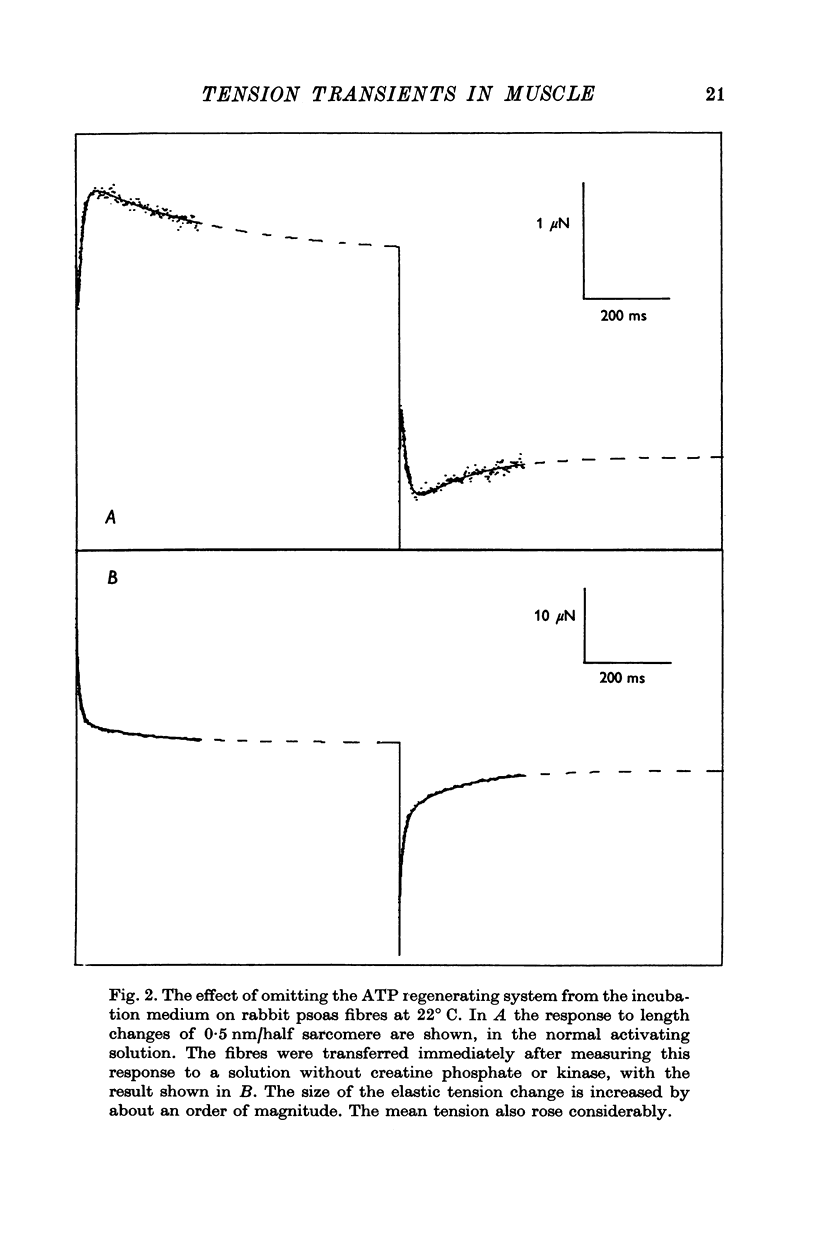
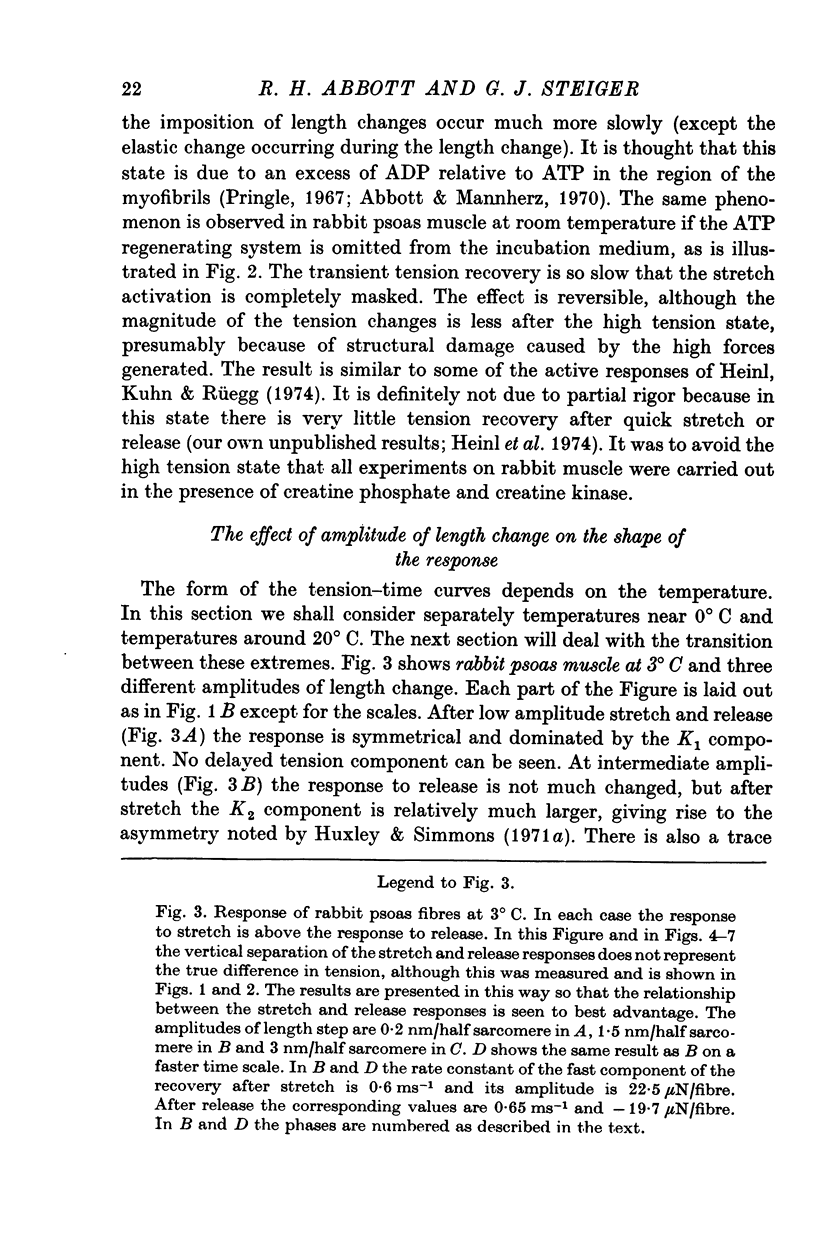
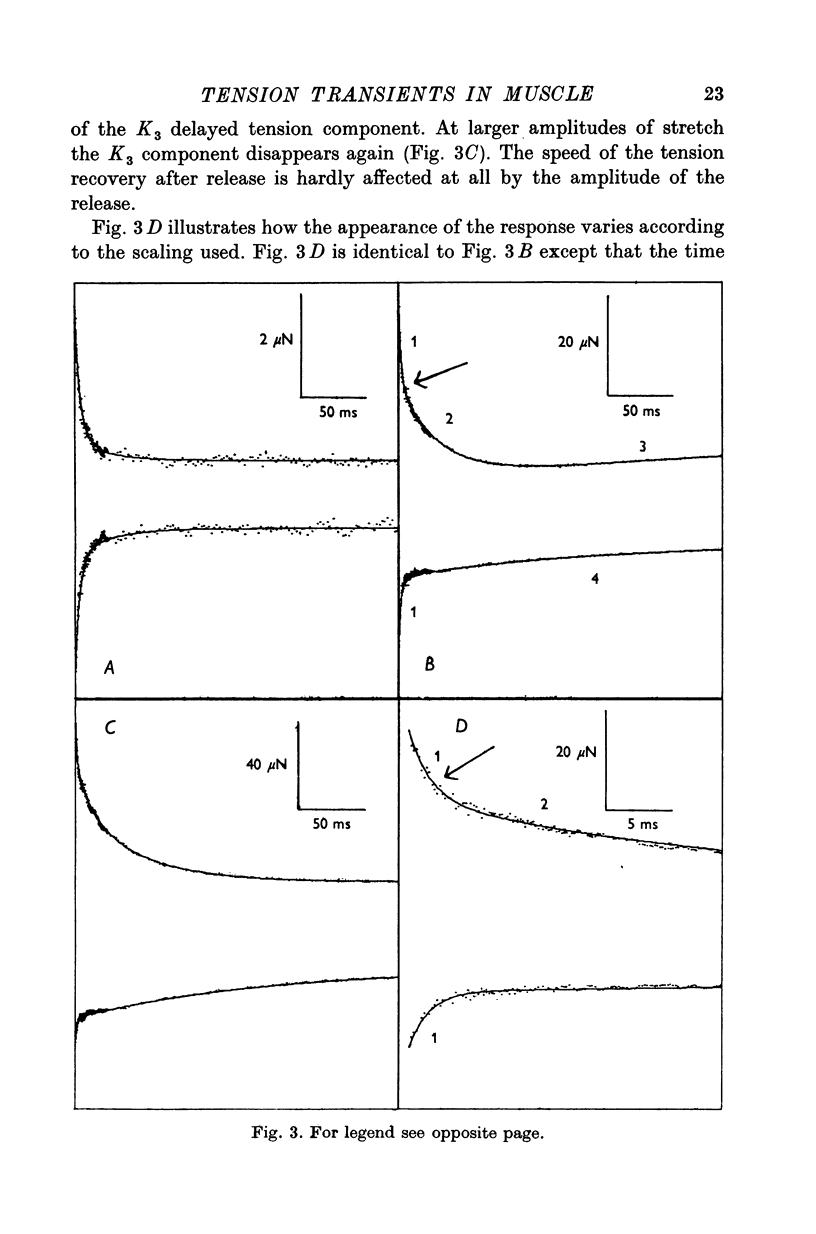
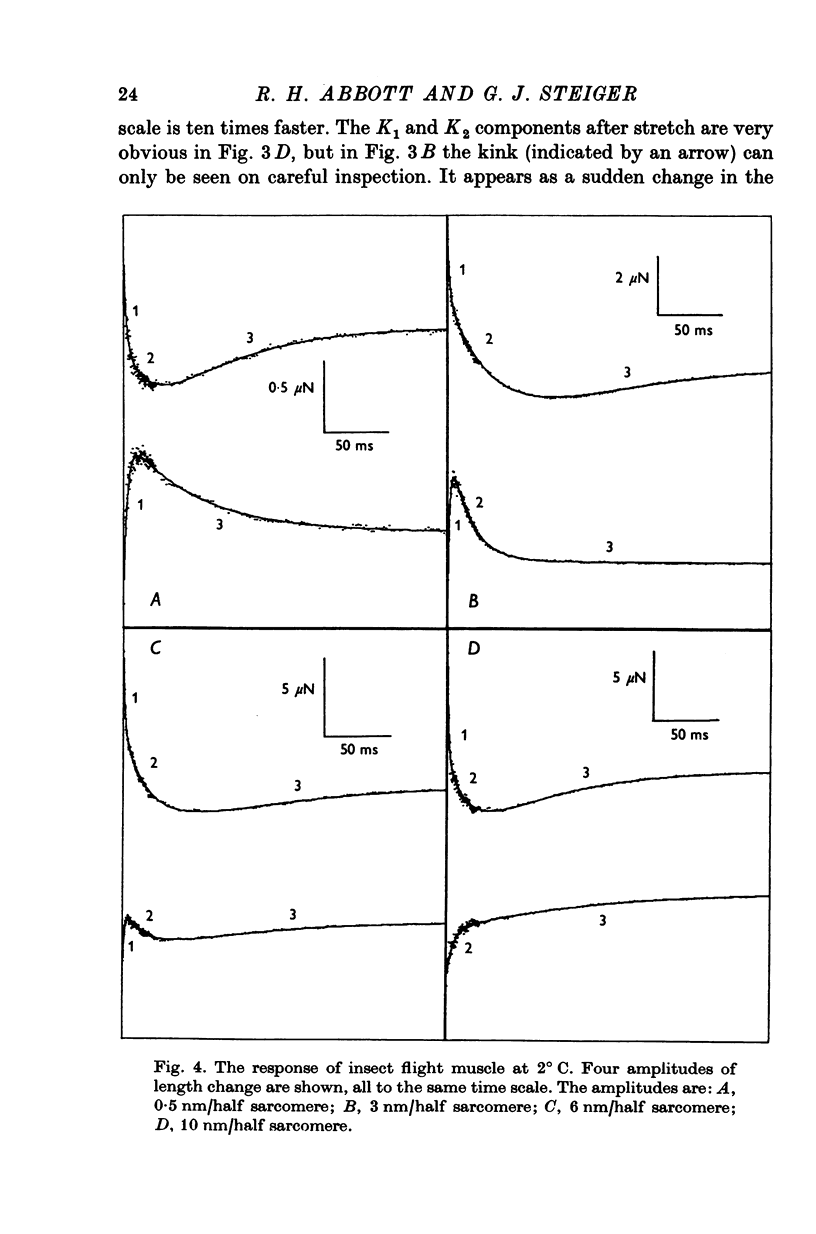
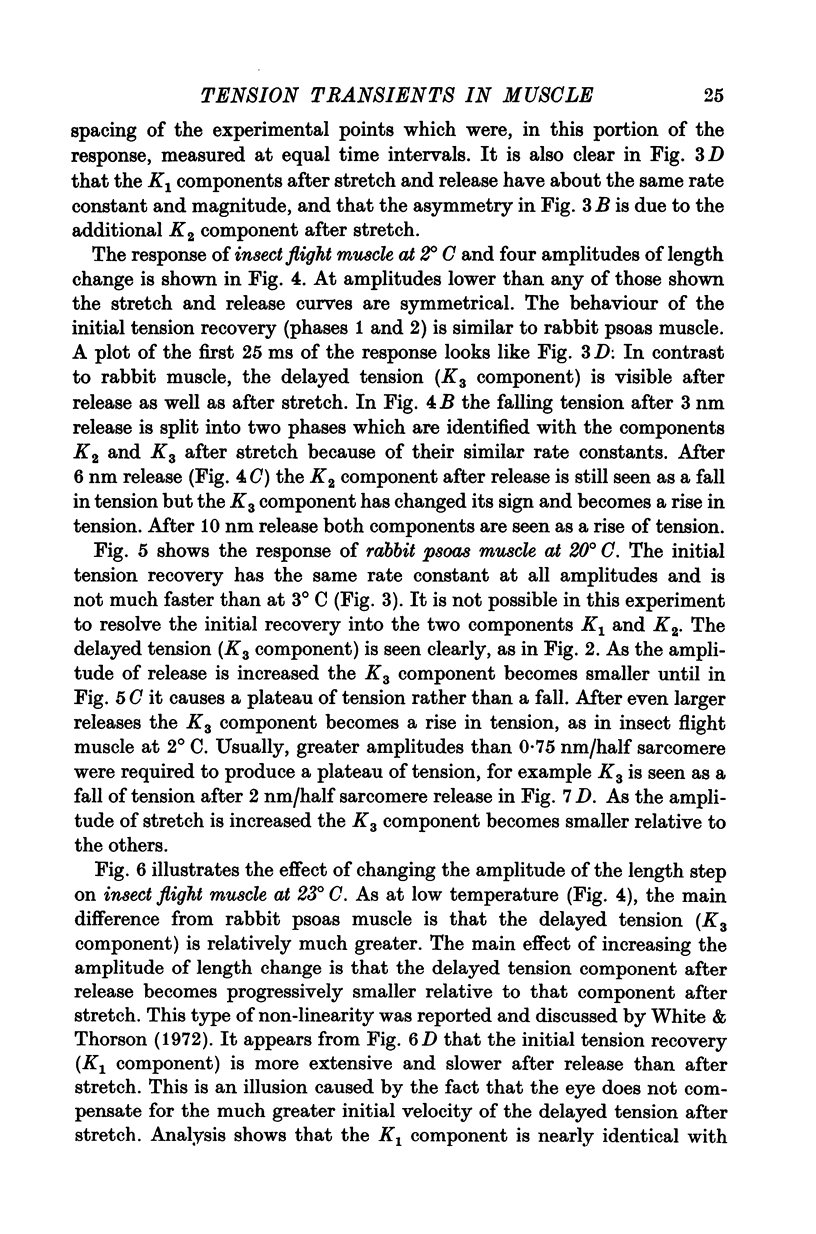
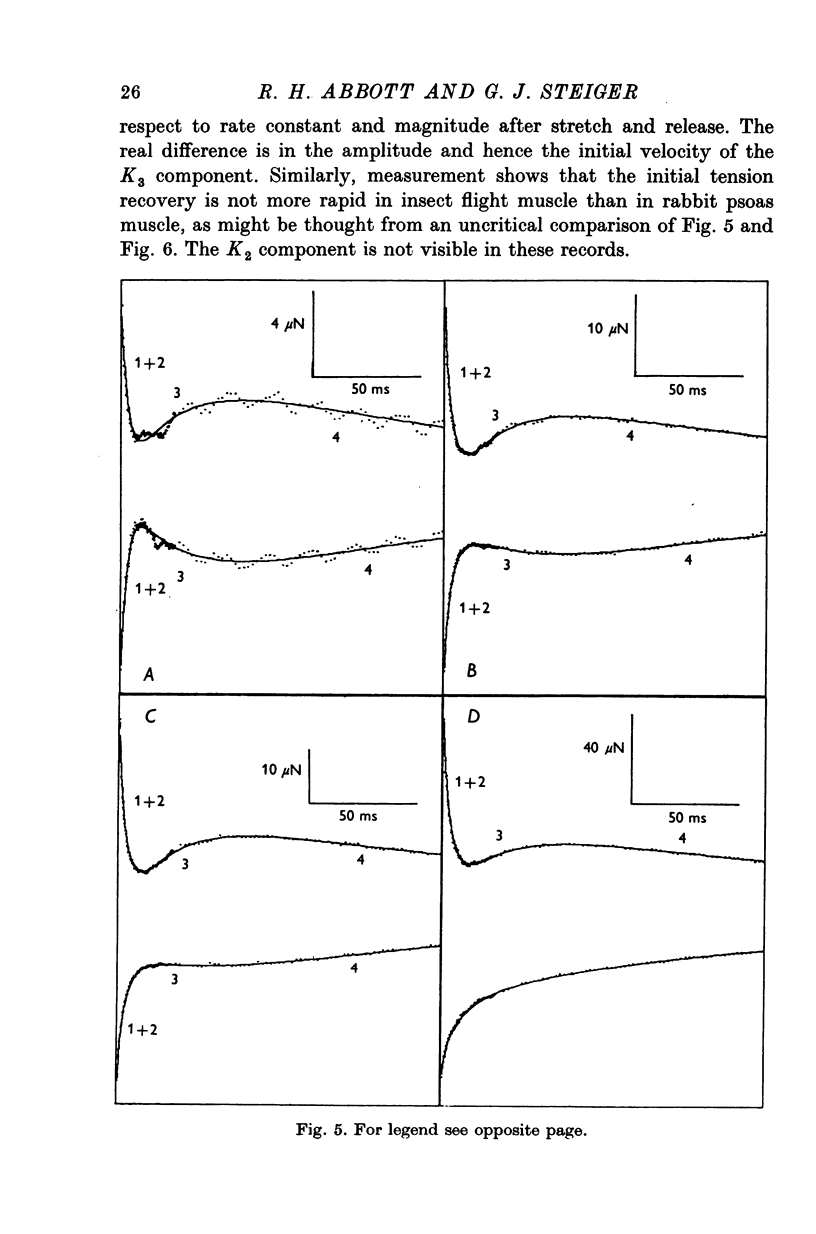
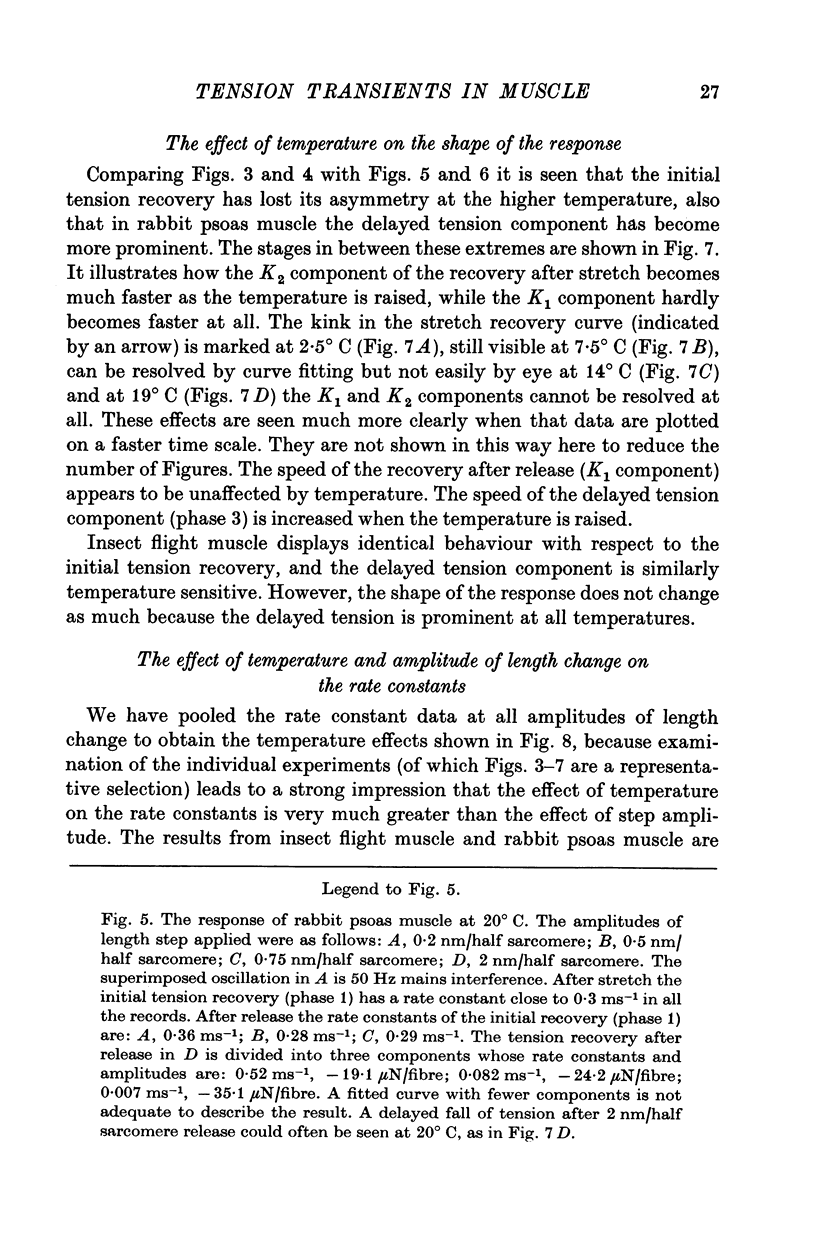
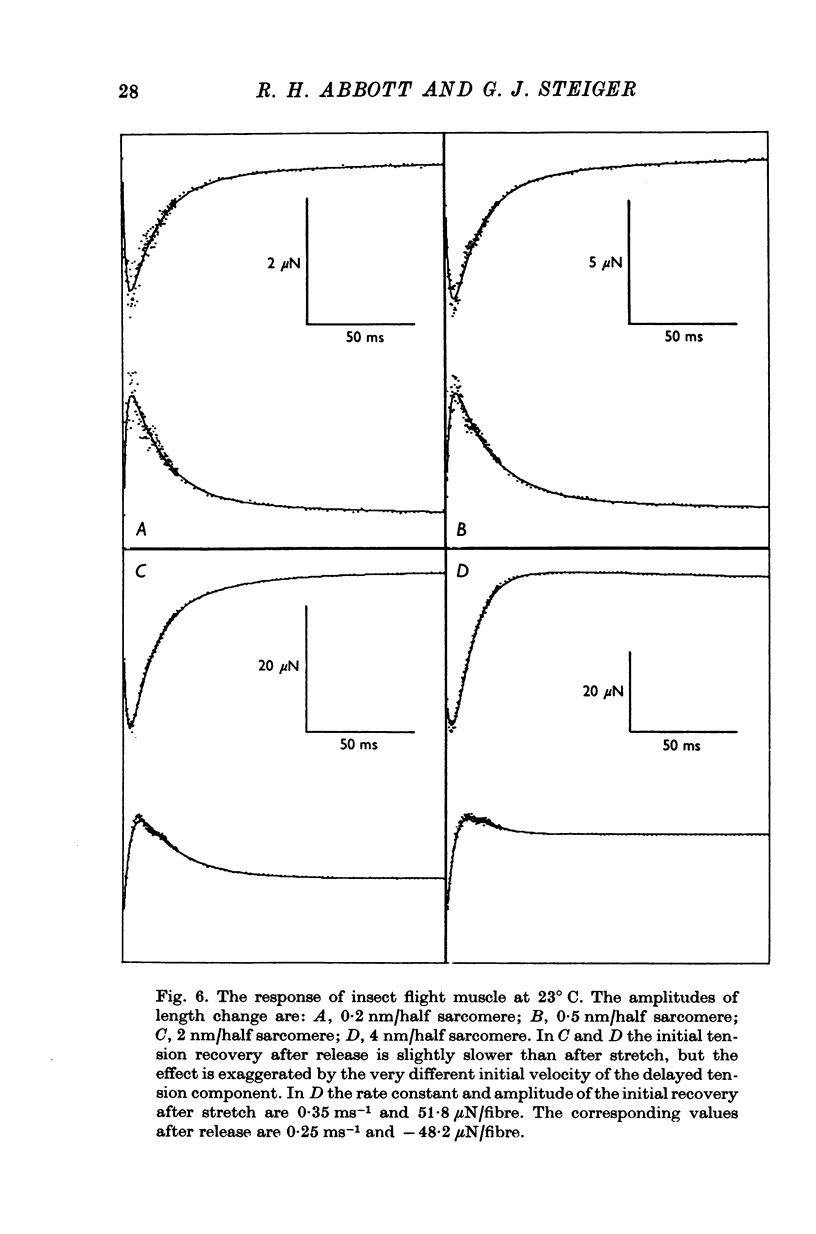
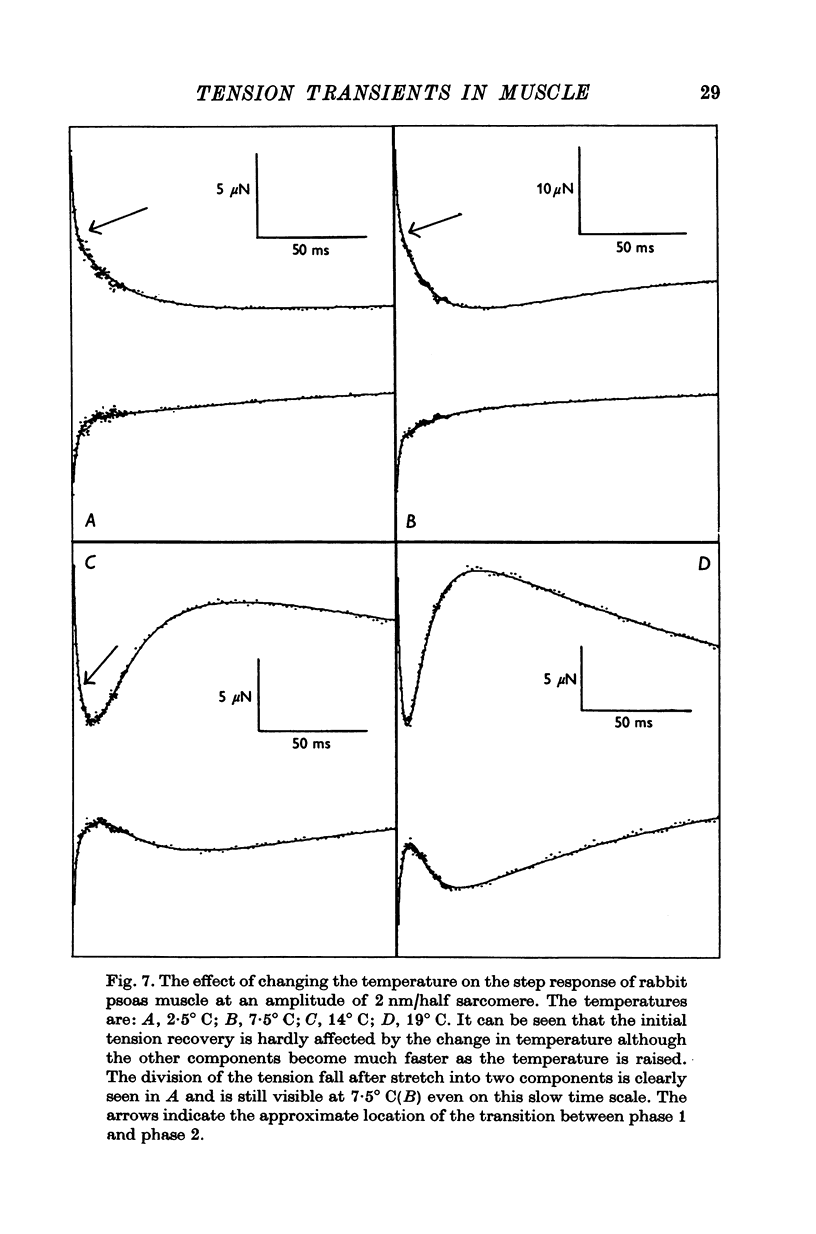
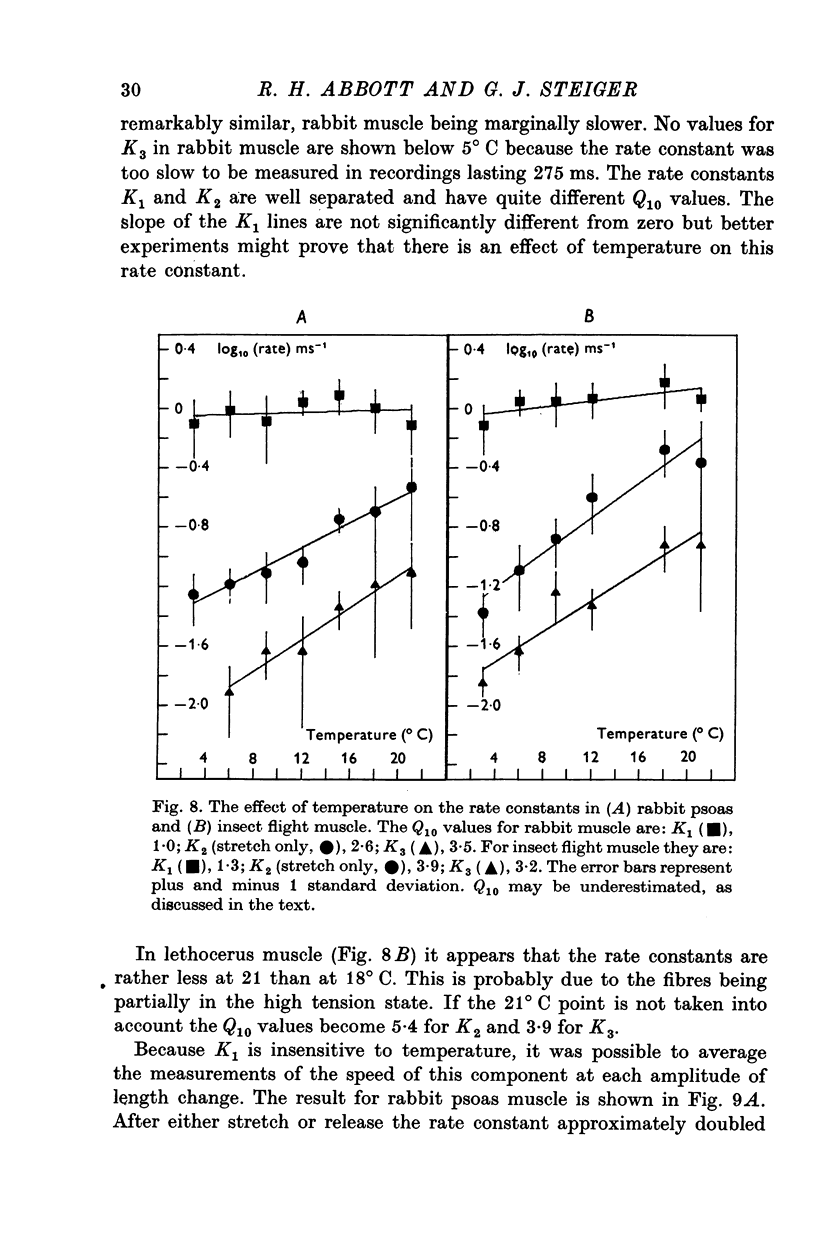
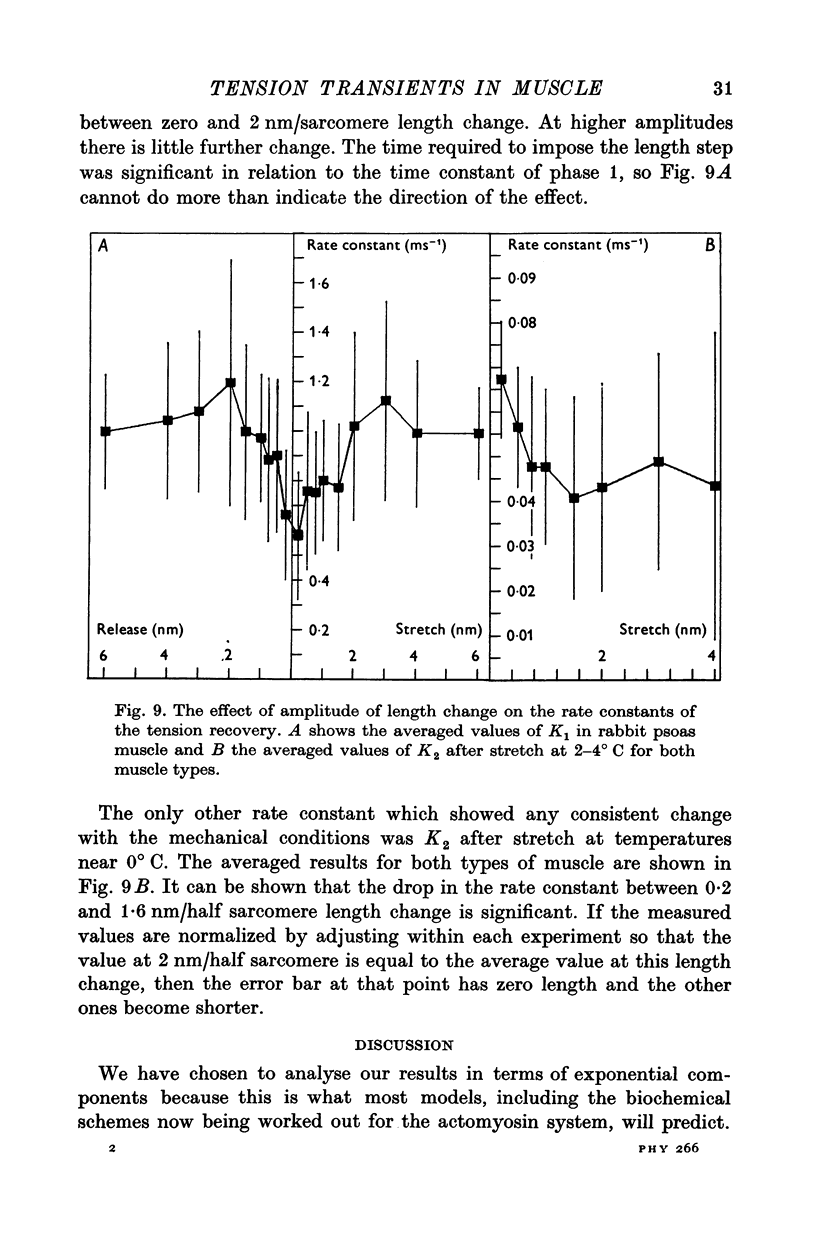
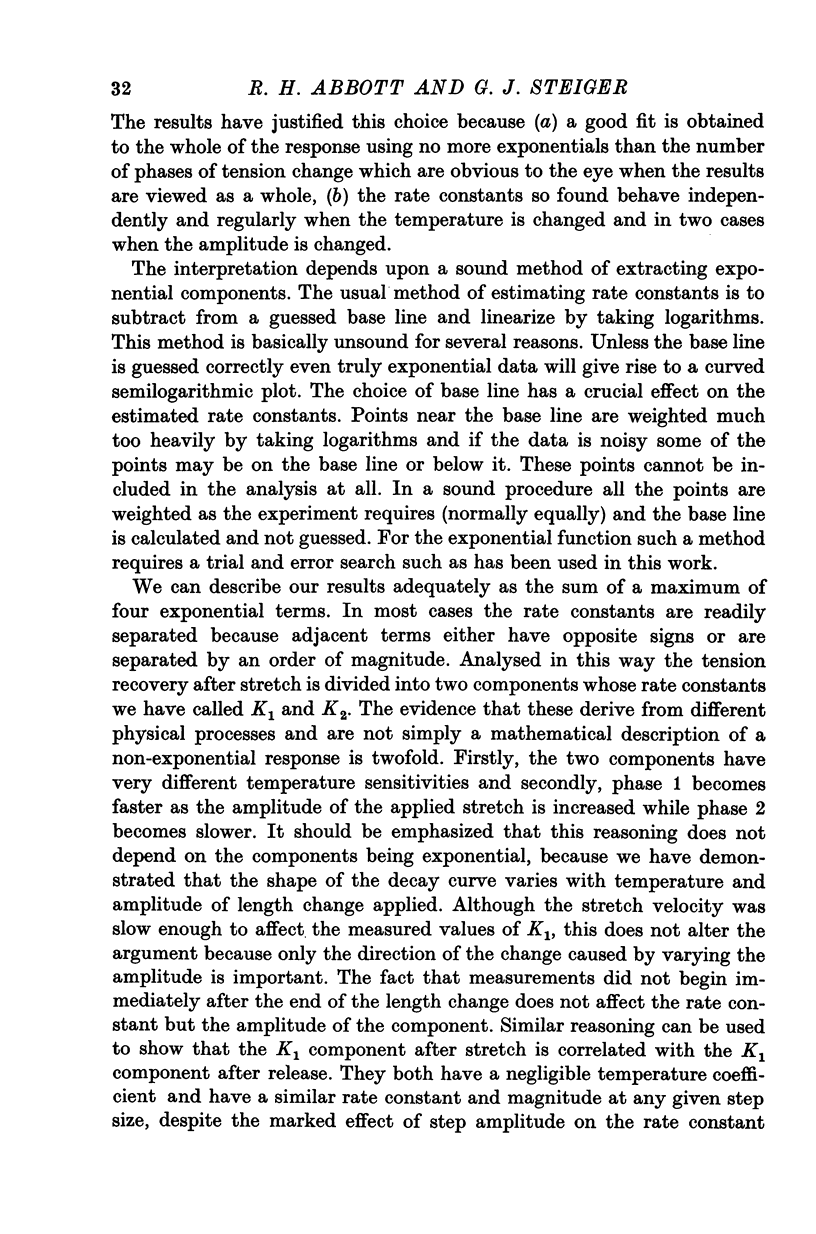
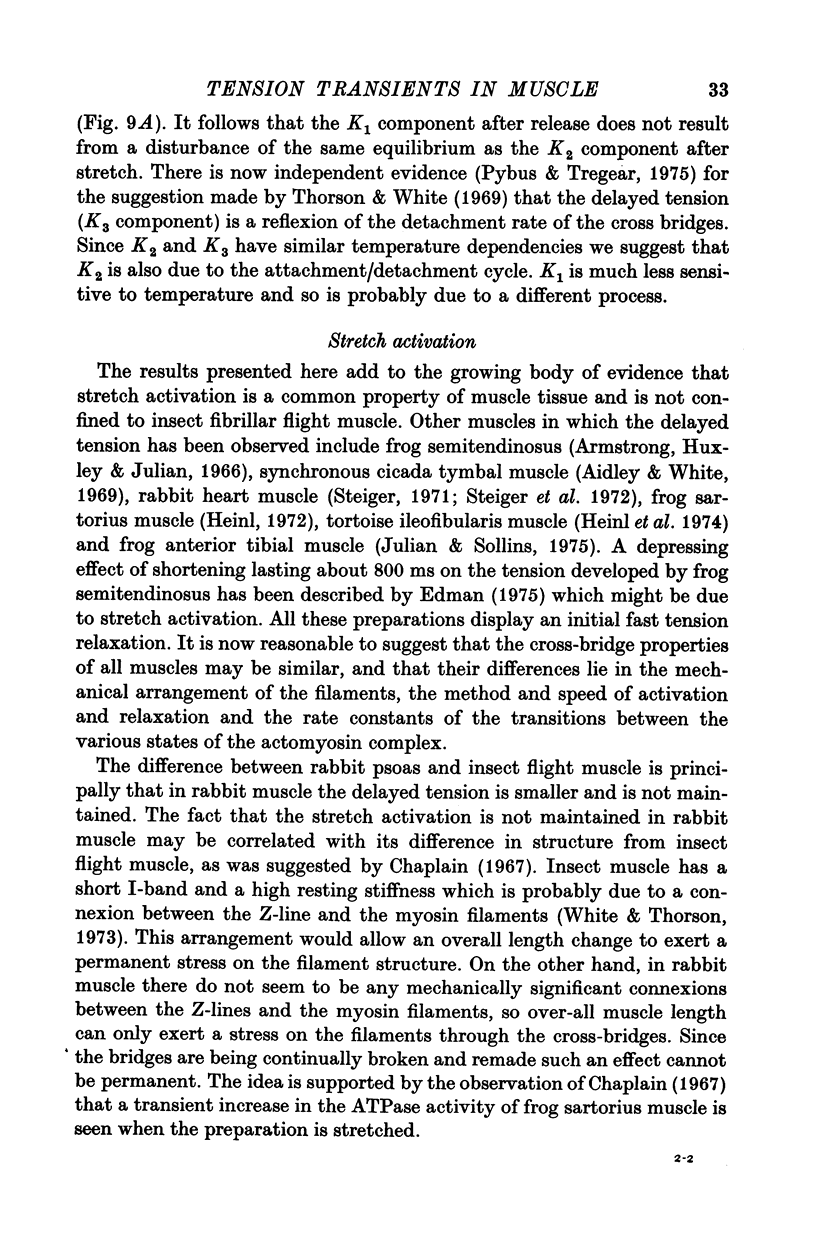
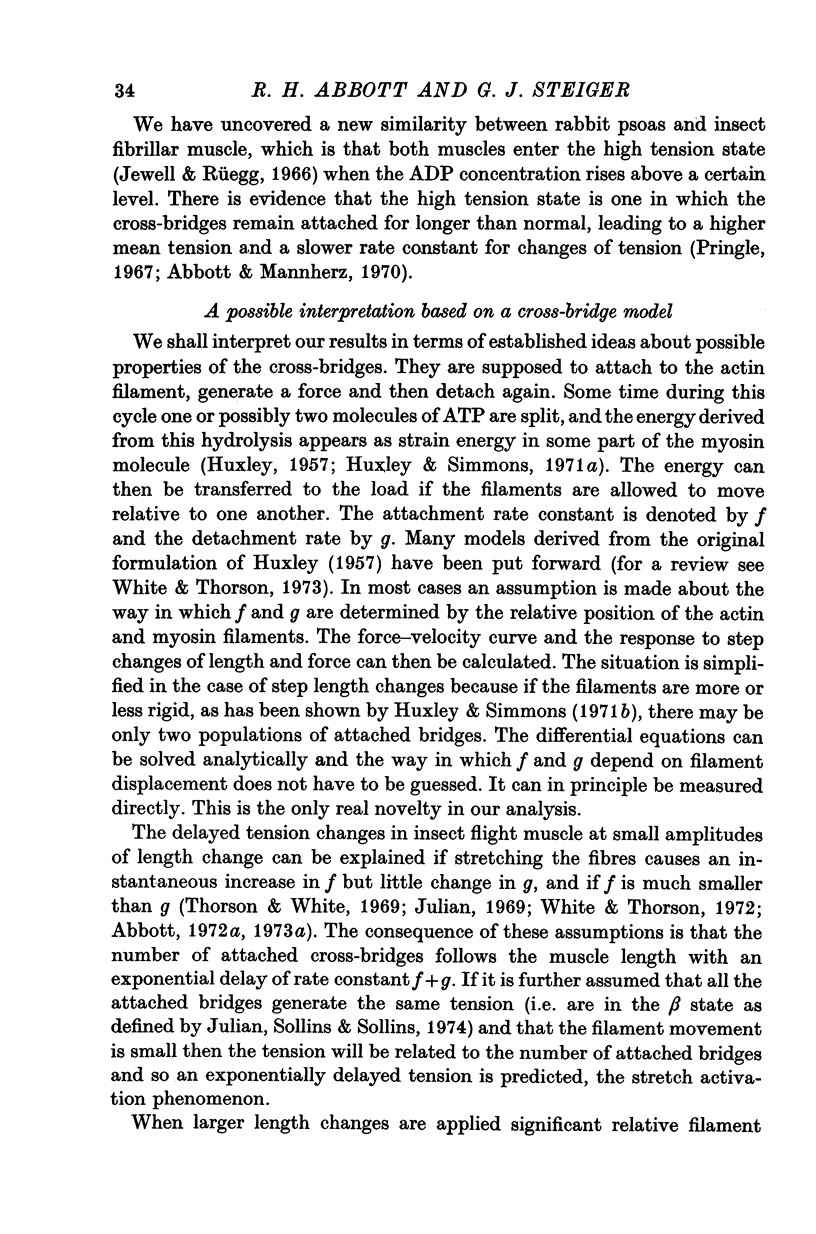
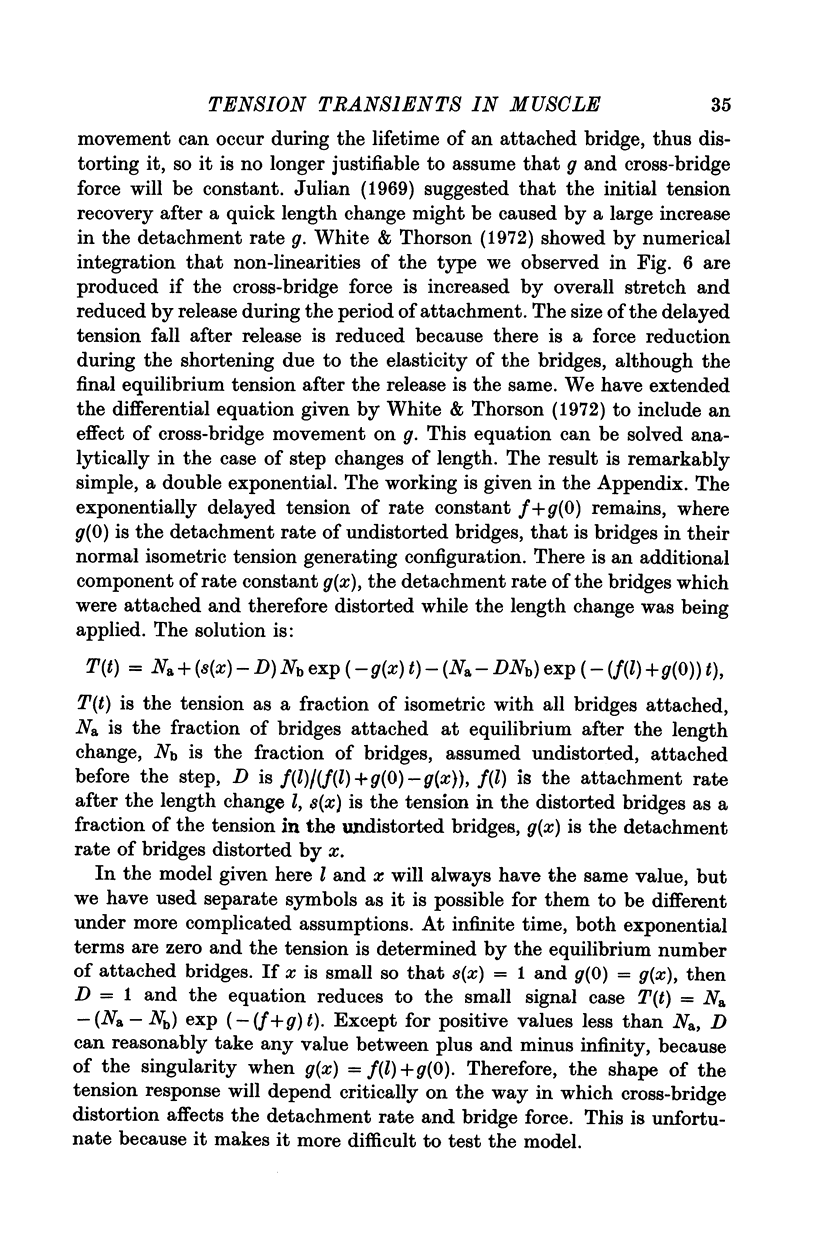
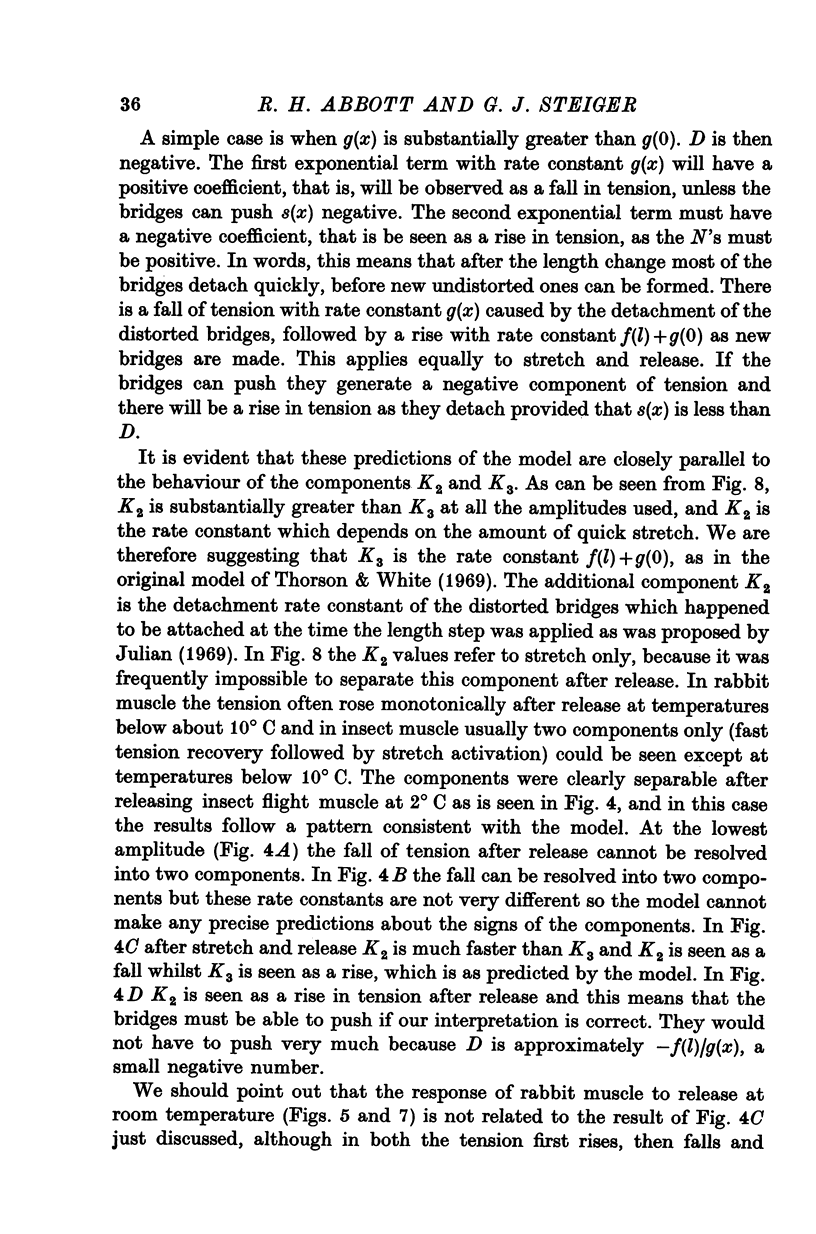
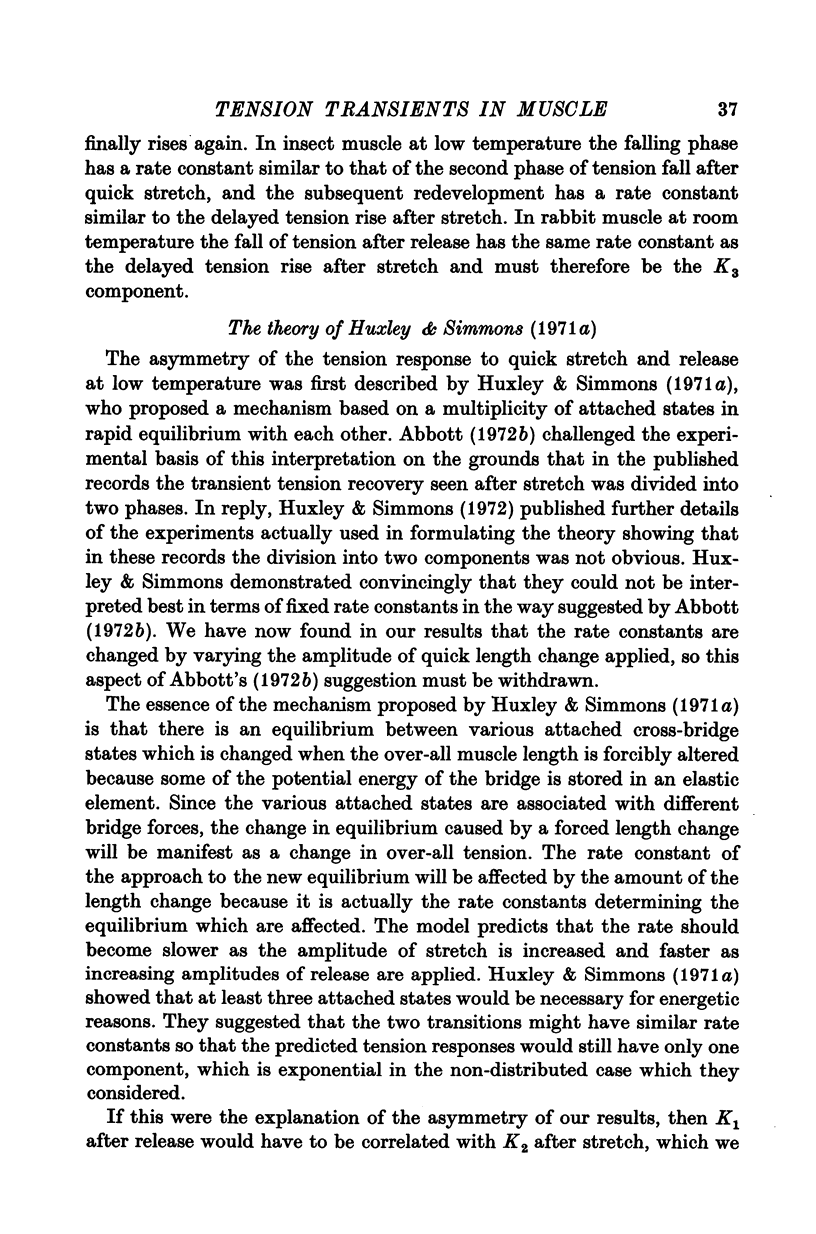
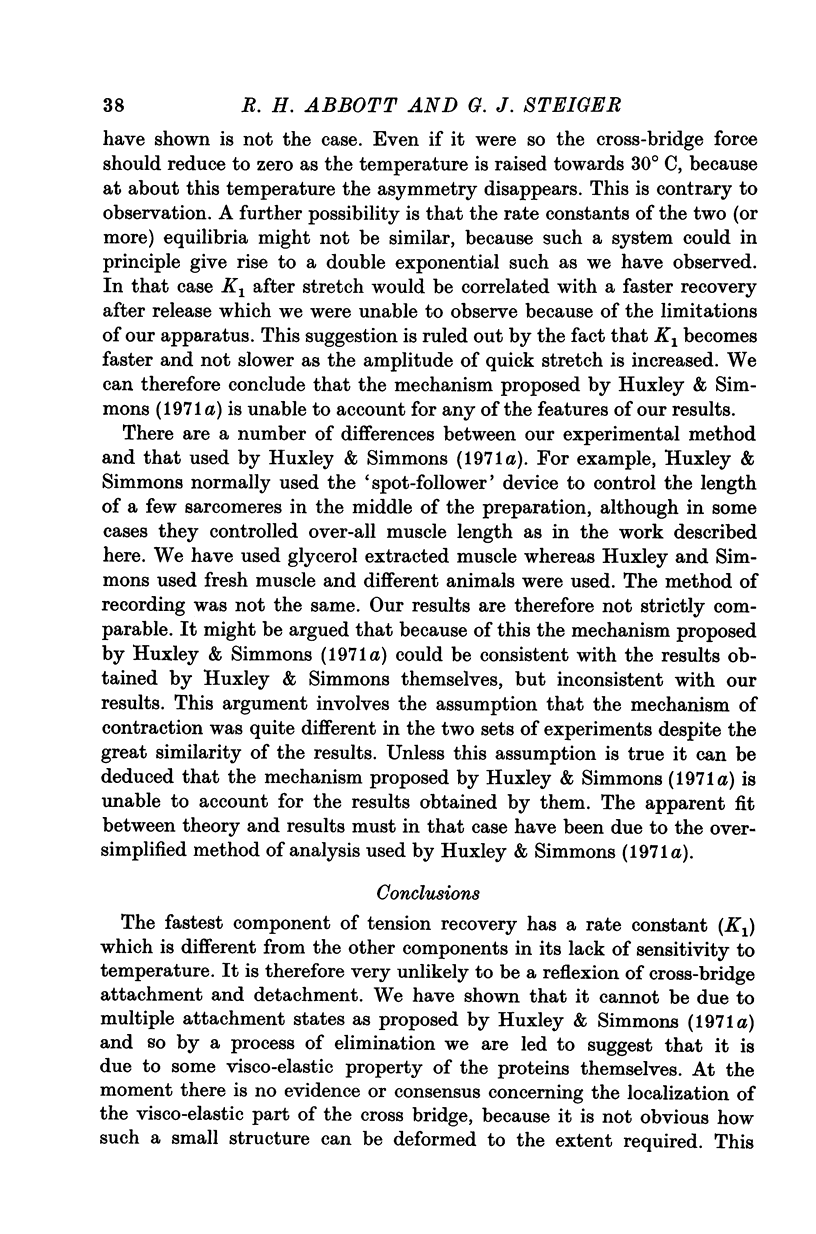
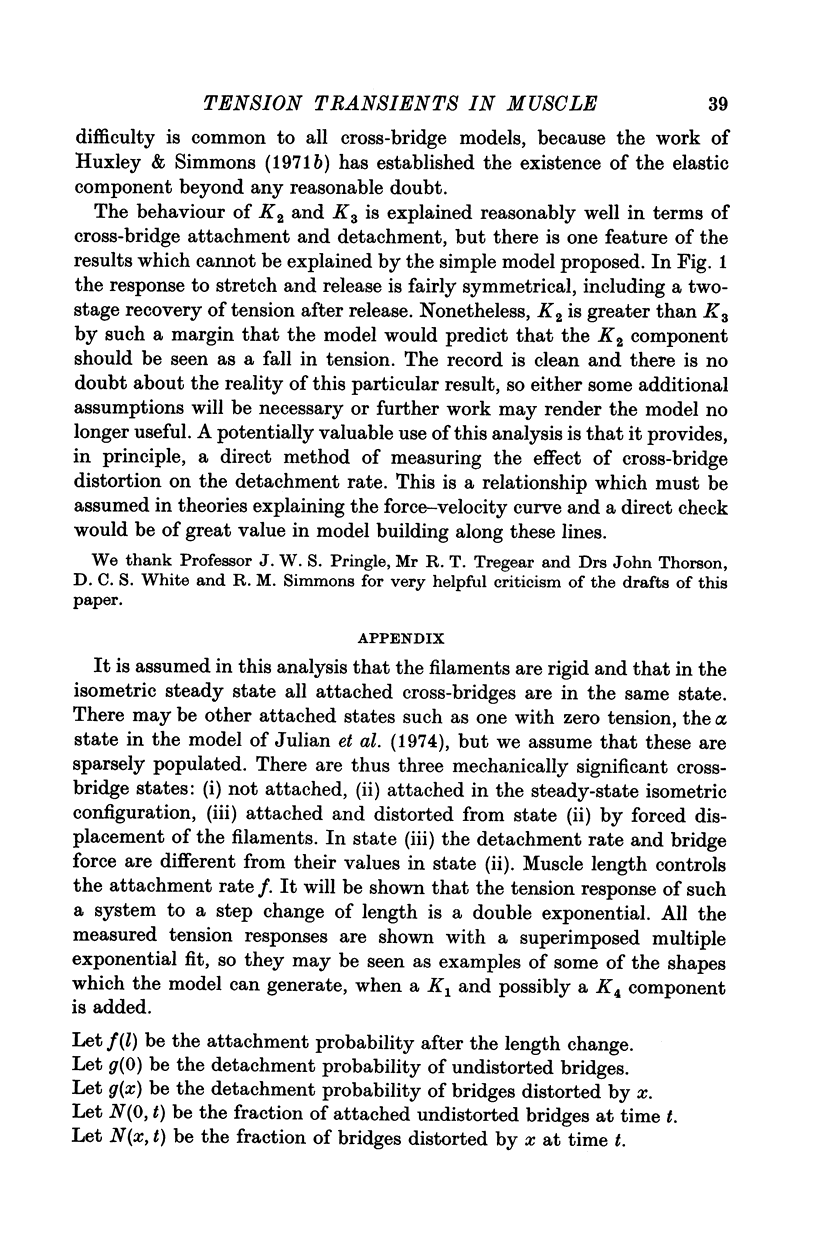
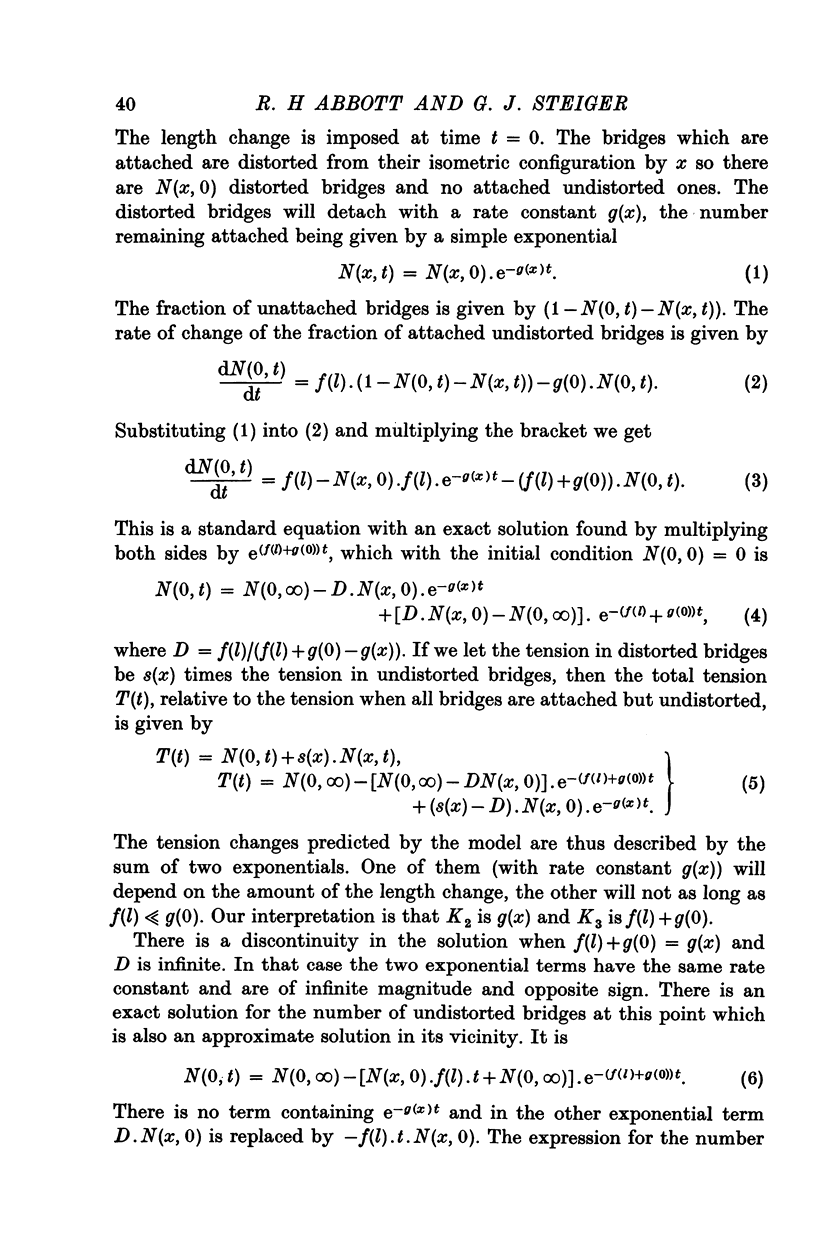
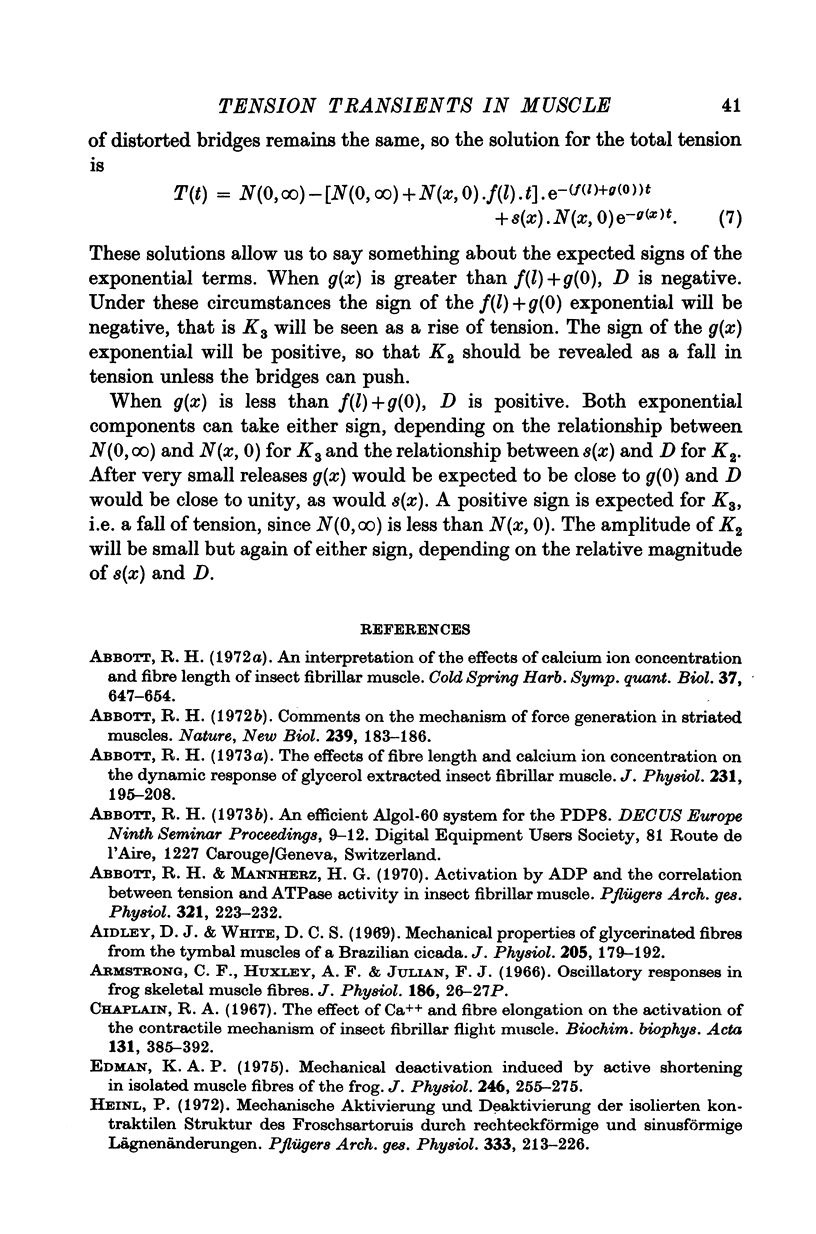
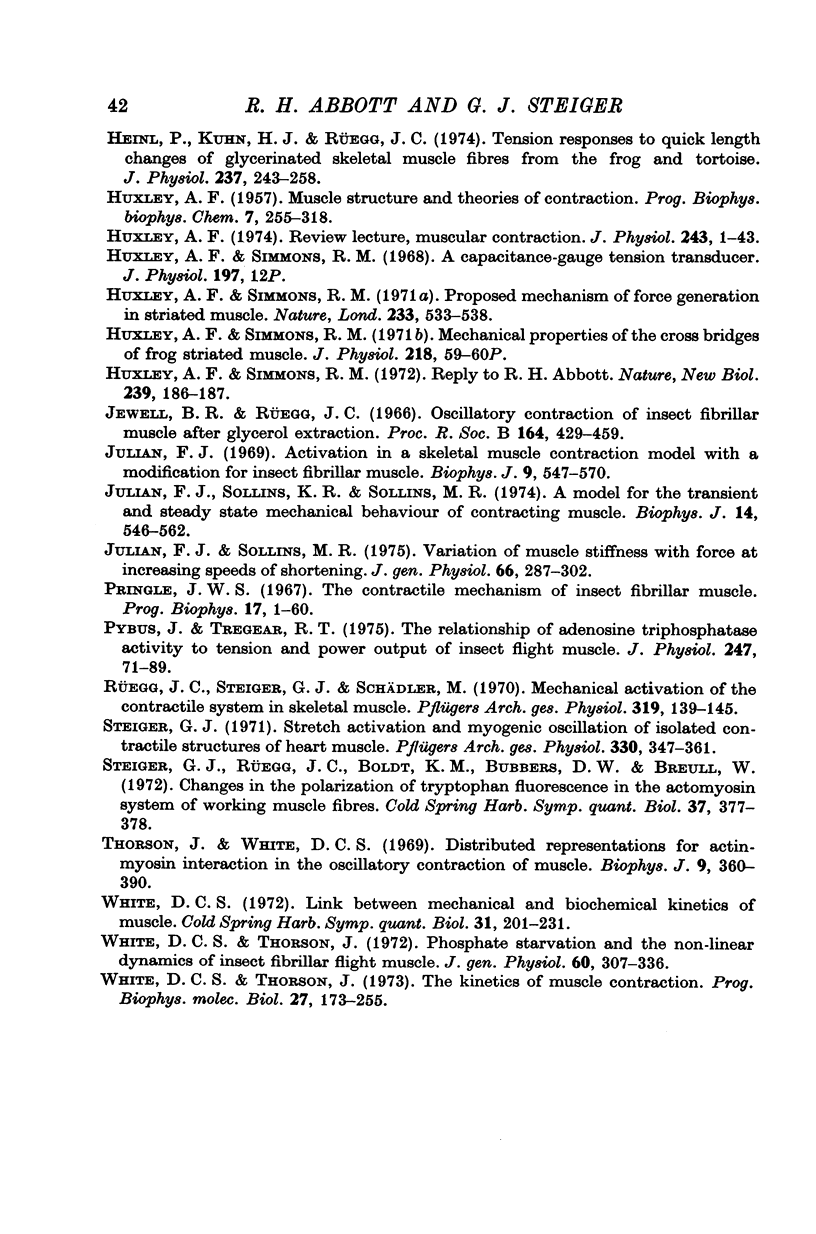
Selected References
These references are in PubMed. This may not be the complete list of references from this article.
- Abbott R. H. Comments on the mechanism of force generation in striated muscles. Nat New Biol. 1972 Oct 11;239(93):183–187. doi: 10.1038/newbio239183a0. [DOI] [PubMed] [Google Scholar]
- Abbott R. H., Mannherz G. H. Activation by ADP and the correlation between tension and ATPase activity in insect fibrillar muscle. Pflugers Arch. 1970;321(3):223–232. doi: 10.1007/BF00588443. [DOI] [PubMed] [Google Scholar]
- Abbott R. H. The effects of fibre length and calcium ion concentration on the dynamic response of glycerol extracted insect fibrillar muscle. J Physiol. 1973 Jun;231(2):195–208. doi: 10.1113/jphysiol.1973.sp010228. [DOI] [PMC free article] [PubMed] [Google Scholar]
- Chaplain R. A. The effect of Ca2+ and fibre elongation on the activation of the contractile mechanism of insect fibrillar flight muscle. Biochim Biophys Acta. 1967 Mar 8;131(2):385–392. doi: 10.1016/0005-2728(67)90152-1. [DOI] [PubMed] [Google Scholar]
- Edman K. A. Mechanical deactivation induced by active shortening in isolated muscle fibres of the frog. J Physiol. 1975 Mar;246(1):255–275. doi: 10.1113/jphysiol.1975.sp010889. [DOI] [PMC free article] [PubMed] [Google Scholar]
- HUXLEY A. F. Muscle structure and theories of contraction. Prog Biophys Biophys Chem. 1957;7:255–318. [PubMed] [Google Scholar]
- Heinl P., Kuhn H. J., Rüegg J. C. Tension responses to quick length changes of glycerinated skeletal muscle fibres from the frog and tortoise. J Physiol. 1974 Mar;237(2):243–258. doi: 10.1113/jphysiol.1974.sp010480. [DOI] [PMC free article] [PubMed] [Google Scholar]
- Huxley A. F., Simmons R. M. A capacitance-gauge tension transducer. J Physiol. 1968 Jul;197(1):12P–12P. [PubMed] [Google Scholar]
- Huxley A. F., Simmons R. M. Proposed mechanism of force generation in striated muscle. Nature. 1971 Oct 22;233(5321):533–538. doi: 10.1038/233533a0. [DOI] [PubMed] [Google Scholar]
- Julian F. J. Activation in a skeletal muscle contraction model with a modification for insect fibrillar muscle. Biophys J. 1969 Apr;9(4):547–570. doi: 10.1016/S0006-3495(69)86403-9. [DOI] [PMC free article] [PubMed] [Google Scholar]
- Julian F. J., Sollins K. R., Sollins M. R. A model for the transient and steady-state mechanical behavior of contracting muscle. Biophys J. 1974 Jul;14(7):546–562. doi: 10.1016/S0006-3495(74)85934-5. [DOI] [PMC free article] [PubMed] [Google Scholar]
- Julian F. J., Sollins M. R. Variation of muscle stiffness with force at increasing speeds of shortening. J Gen Physiol. 1975 Sep;66(3):287–302. doi: 10.1085/jgp.66.3.287. [DOI] [PMC free article] [PubMed] [Google Scholar]
- Pybus J., Tregear R. T. The relationship of adenosine triphosphatase activity to tension and power output of insect flight muscle. J Physiol. 1975 May;247(1):71–89. doi: 10.1113/jphysiol.1975.sp010921. [DOI] [PMC free article] [PubMed] [Google Scholar]
- Rüegg J. C., Steiger G. J., Schädler M. Mechanical activation of the contractile system in skeletal muscle. Pflugers Arch. 1970;319(2):139–145. doi: 10.1007/BF00592492. [DOI] [PubMed] [Google Scholar]
- Steiger G. J. Stretch activation and myogenic oscillation of isolated contractile structures of heart muscle. Pflugers Arch. 1971;330(4):347–361. doi: 10.1007/BF00588586. [DOI] [PubMed] [Google Scholar]
- Thorson J., White D. C. Distributed representations for actin-myosin interaction in the oscillatory contraction of muscle. Biophys J. 1969 Mar;9(3):360–390. doi: 10.1016/S0006-3495(69)86392-7. [DOI] [PMC free article] [PubMed] [Google Scholar]
- White D. C., Thorson J. Phosphate starvation and the nonlinear dynamics of insect fibrillar flight muscle. J Gen Physiol. 1972 Sep;60(3):307–336. doi: 10.1085/jgp.60.3.307. [DOI] [PMC free article] [PubMed] [Google Scholar]