Abstract
1. Threshold changes of Na and Ca currents due to various polyvalent cations (stabilizing cations) or H+ ions were studied in the egg cell membrane of a tunicate, Halocynthia roretzi, by using the voltage-clamp technique.
2. With an increase in [Ca]o or a decrease in pH in the external solution, the current—voltage (I—V) relations for the peak of the Na and Ca currents shifted along the voltage axis in the positive direction. These voltage shifts in the I—V relations, measured at a potential of V½ where inward current attains its half-maximum, were shown to be identical to shifts in voltage-dependence of the time courses of Na and Ca currents, and also identical to shifts in the inactivation curves of Na current along the voltage axis.
3. The shifts in V½ produced by various polyvalent cations or H+ ions were analysed by the Gouy-Chapman equation for the diffuse double layer, by assuming that a change in V½ directly corresponds to a change in the surface double layer potential.
4. The V½-divalent cation concentration relations of Na current were exactly described by the predictions of the theory with a constant value of the surface charge density of 1e-/(9 Å)2. The weak stabilizing effects of Mg2+, Sr2+ and Ba2+ were quite similar to each other and were explained in terms of a `screening' effect. Other divalent cations, such as Ca2+, Mn2+ and Ni2+, showed various different stabilizing effects which were explained in terms of a `binding' effect. The binding constants (K1's) for Ca2+, Mn2+ and Ni2+ were 0·21, 0·45 and 0·94 M-1, respectively.
5. H+ ions showed a powerful stabilizing effect upon the Na current with a KH of 6 × 104 M-1. This value indicates that the acidic sites around Na channels have a pKa of 4·78. La3+ ions also acted as a strong stabilizer upon the Na current with a KLa of 15 M-1. For both H+ and La3+, the V½-concentration relations were also exactly described by the Gouy-Chapman equation with the same charge density of 1e-/(9 Å)2 as estimated by varying divalent cations.
6. The stabilizing effect of permeant cations such as Ca2+, Sr2+ and Ba2+ on Ca channel currents was analysed. The effect of lowering pH was also studied. It was found that the surface charge density of 1e-/(9 Å)2 estimated by Na current is also applicable to the explanation for the V½-divalent cation concentration or — pH relationships. The estimated binding constants for H+, Ca2+ and Sr2+ were 1·2×105, 0·58 and 0·035 M-1, respectively. Ba2+ does not bind to charged sites near to the Ca channels.
7. It was noticed that a considerable reduction in the conductances of Na and Ca currents occurred in parallel with a stabilizing effect. This reduction was ascribed to a decrease in the concentration of permeant cations at the external surface of the cell membrane, as predicted by the theory of the diffuse double layer. The Goldman, Hodgkin—Katz equation for ionic currents was applied to explain the conductance suppression.
8. The conductance suppressions of Na and Ca channel currents due to Ca2+, Sr2+ and Ba2+ were found to be apparent ones, only reflecting decreases in the surface concentration of permeant cations without any changes in the permeability. After correction for the apparent suppression, the real permeability ratio among Ca2+, Sr2+ and Ba2+ for Ca channels was determined as 1·00, 0·56 and 0·21 respectively.
9. The conductance suppression of Na current by lowering pH was explained in terms of a real suppression or blocking which is superimposed on the apparent suppression. Considering the surface [Na]o, the plot of ¯PNa against the surface pH yielded a blocking curve of Na channel by H+ ions, which implies that two H+ ions are necessary to block each Na channel. For Ca channels no real blockage was observed in acidic pH.
10. It was concluded from the present experiment that there exists a surface potential capable of affecting both gating and permeation mechanisms of ionic channels in this tunicate egg cell membrane.
Full text
PDF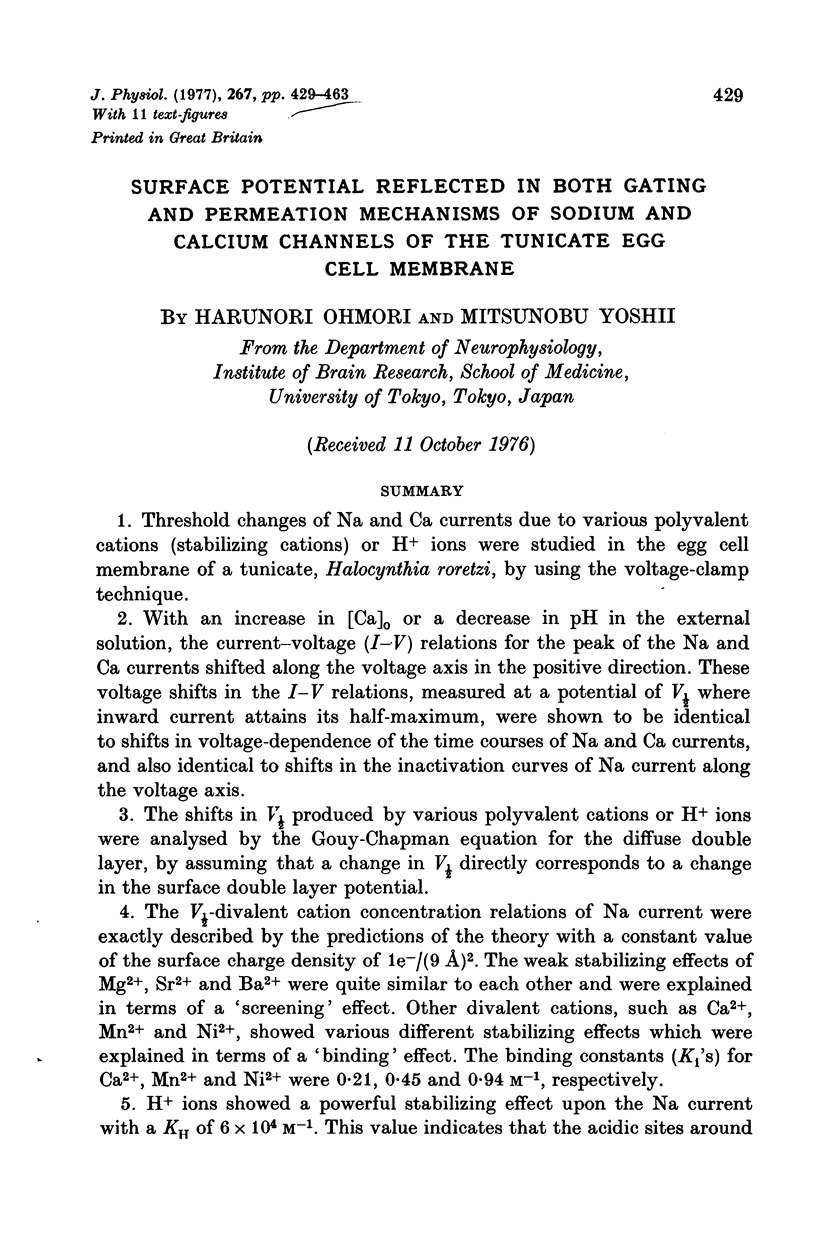
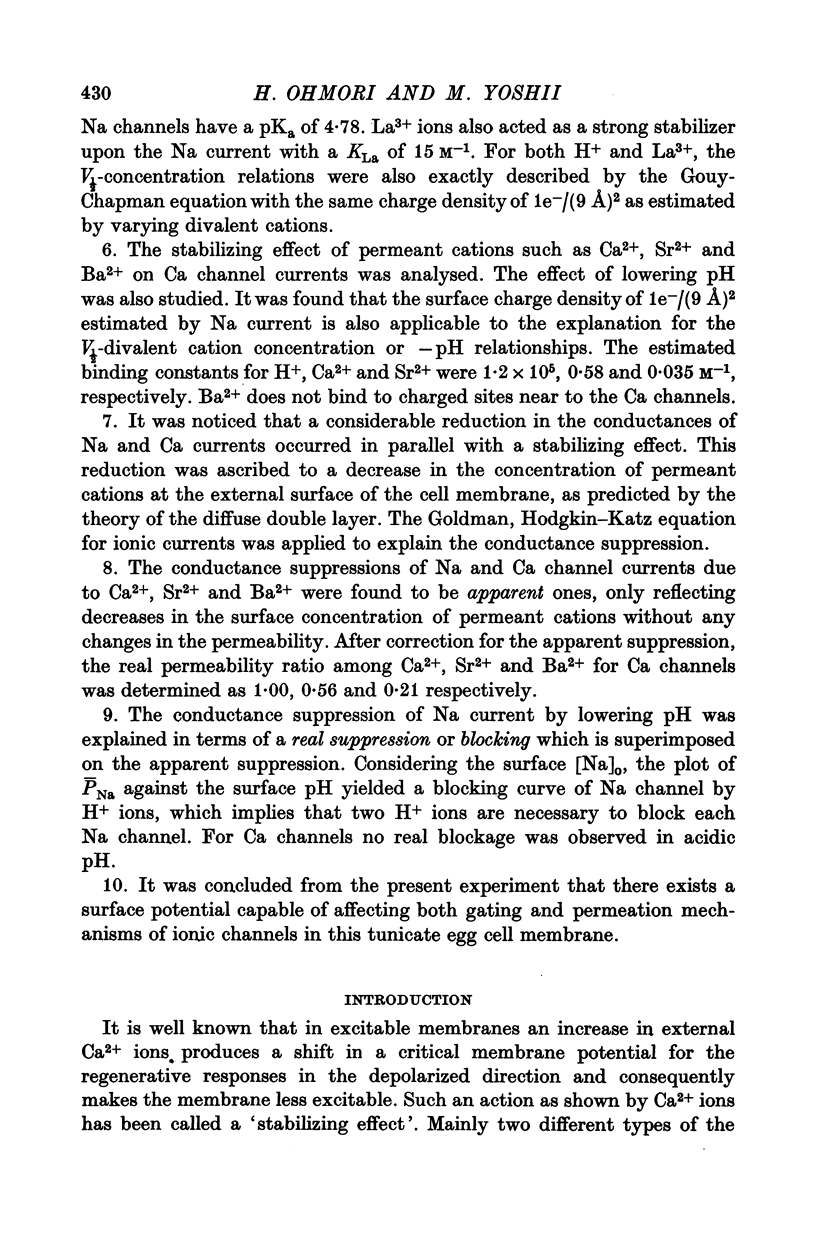
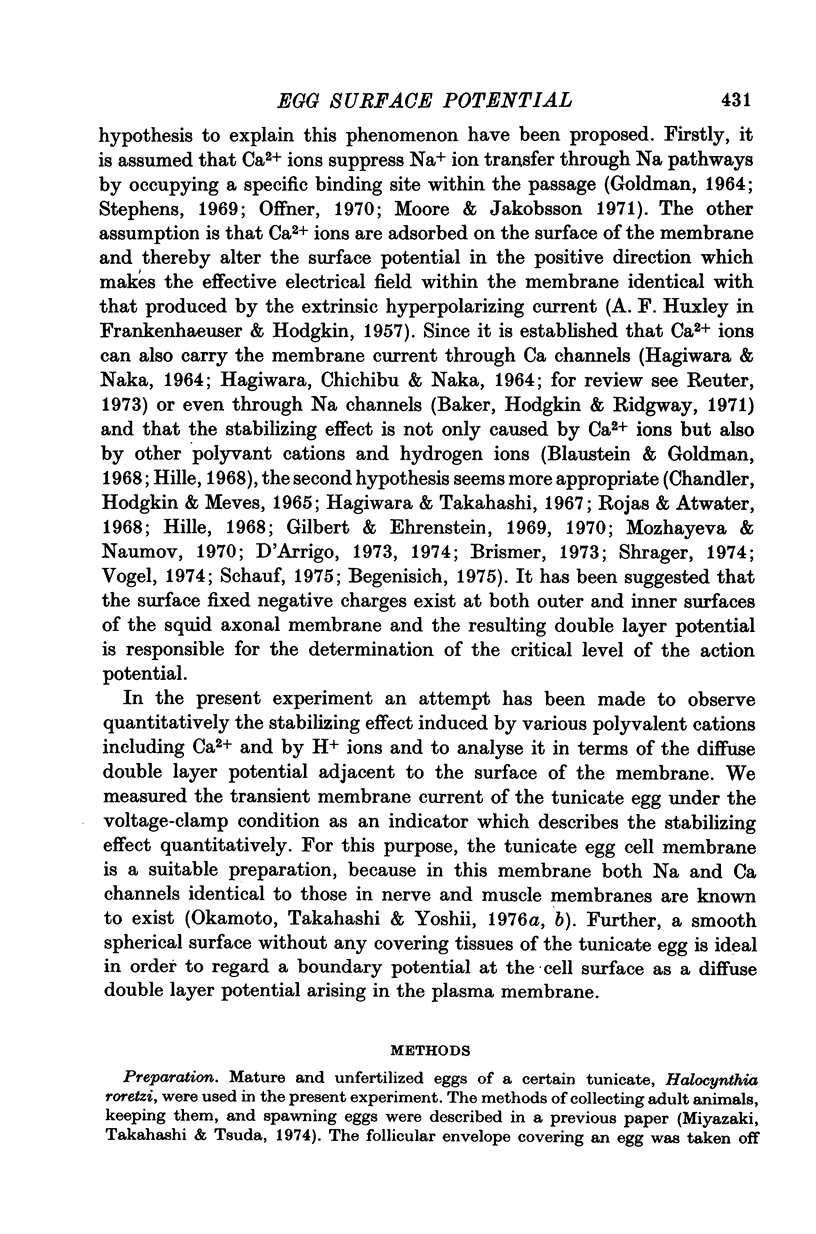
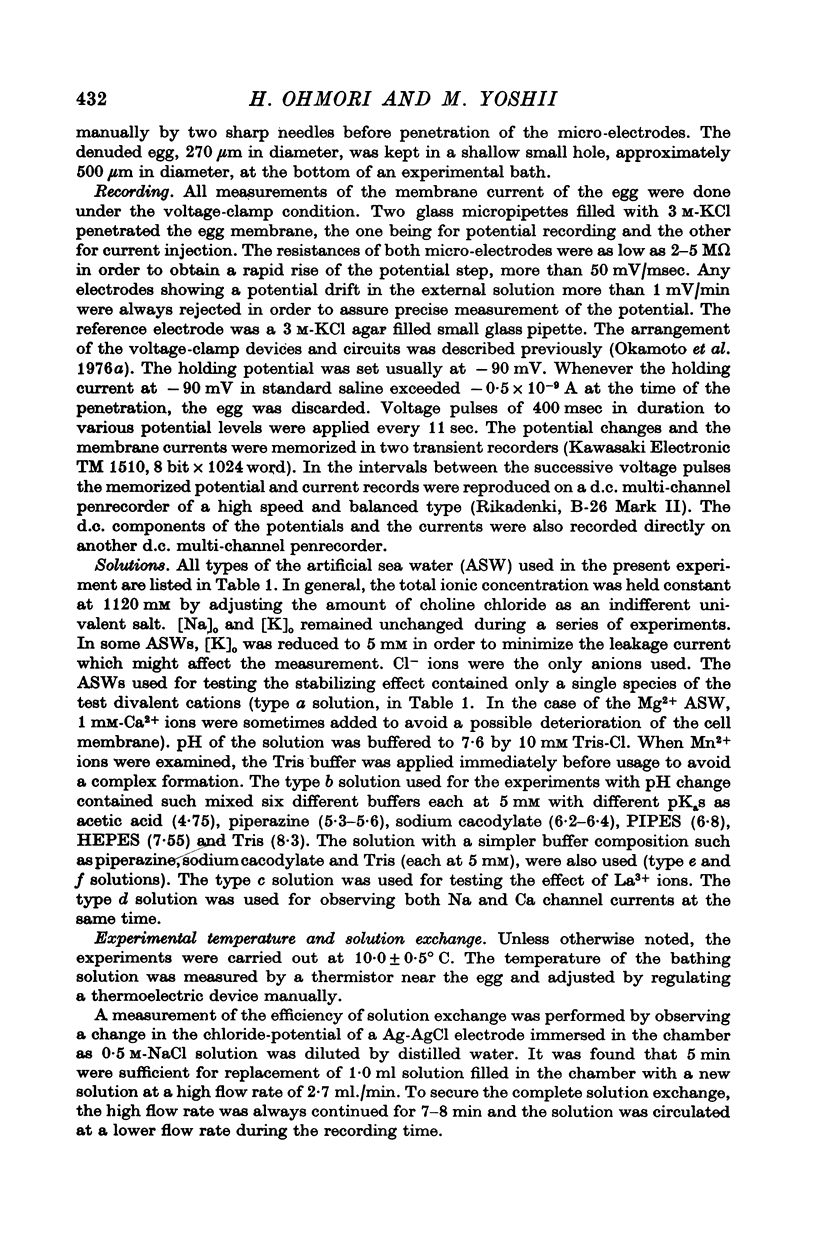
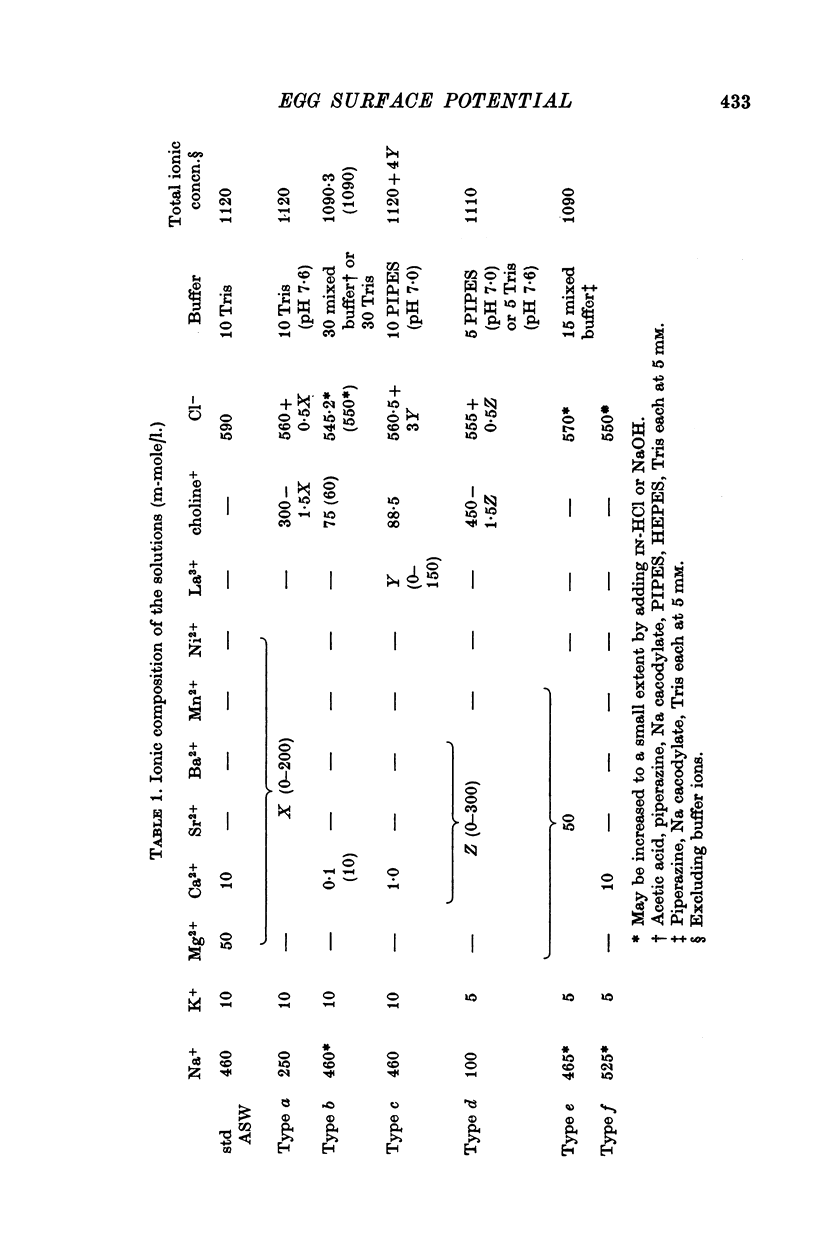
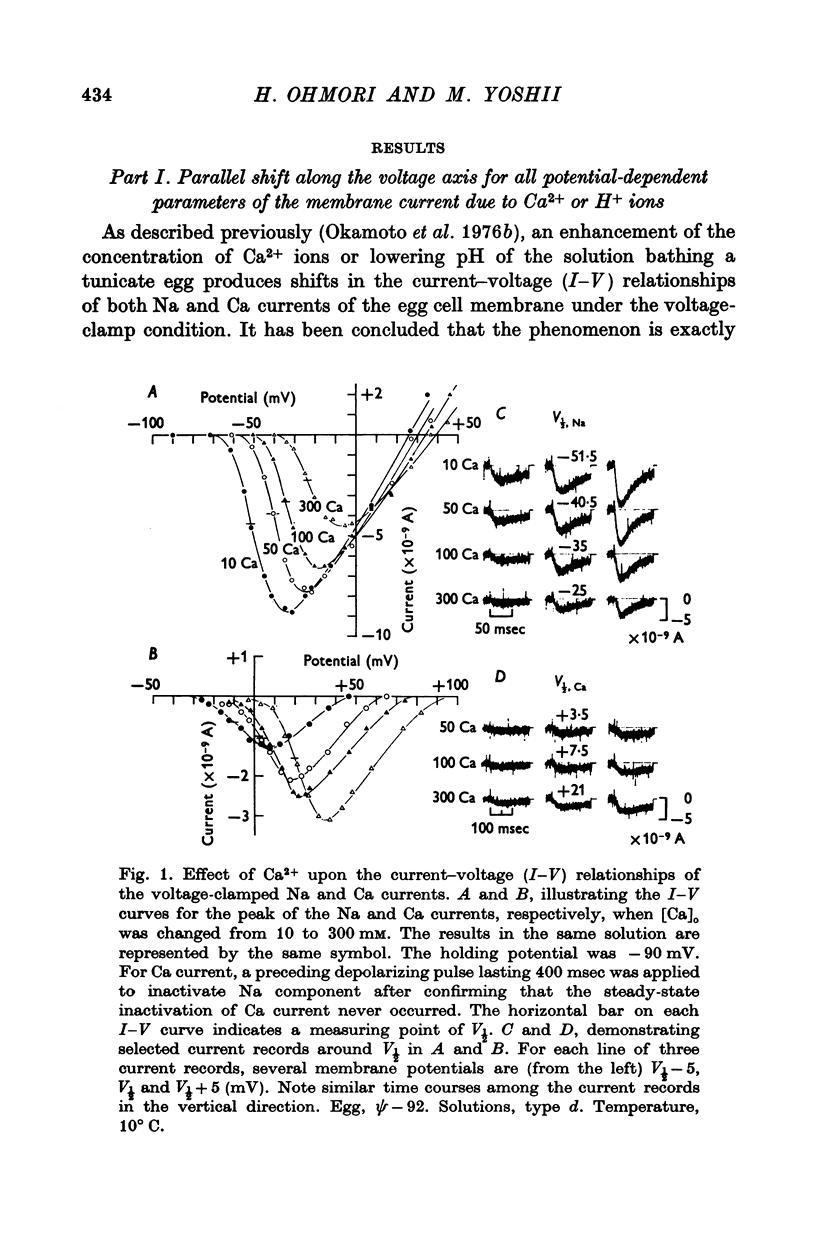
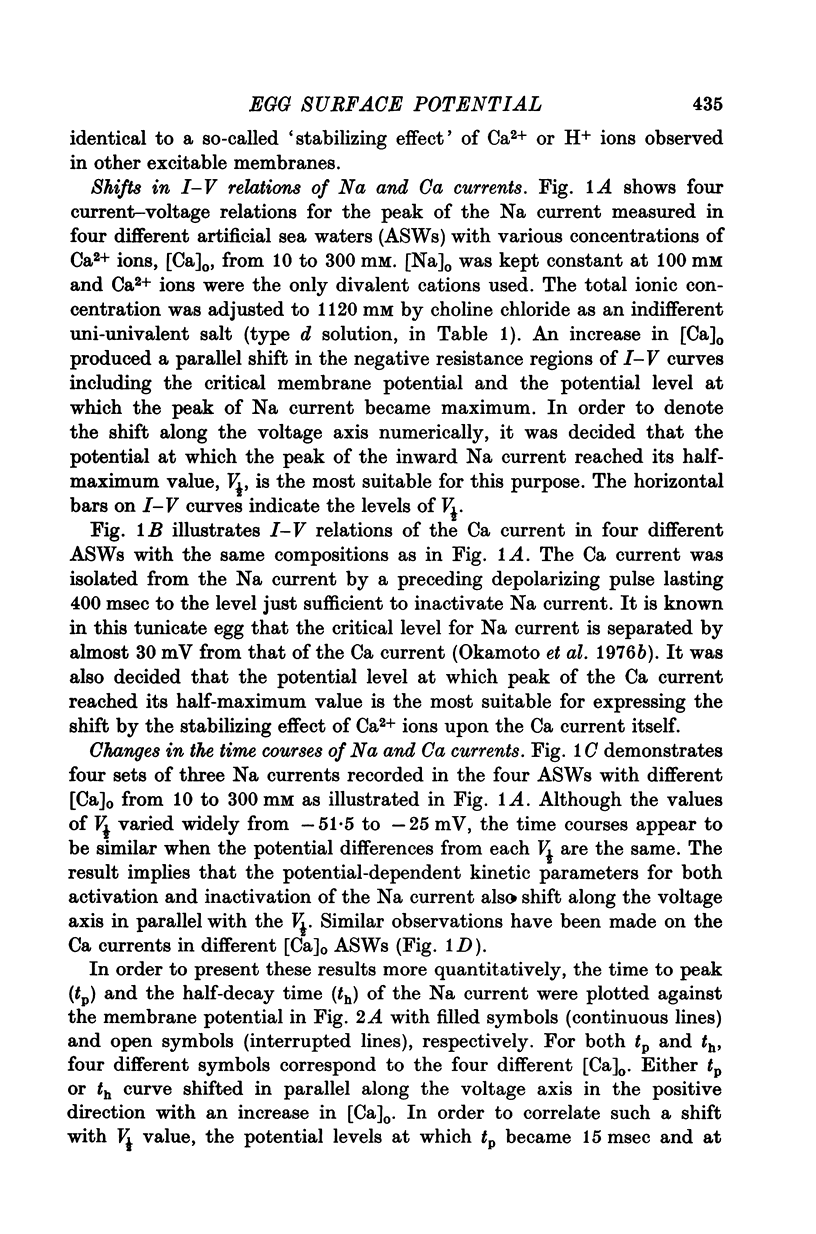
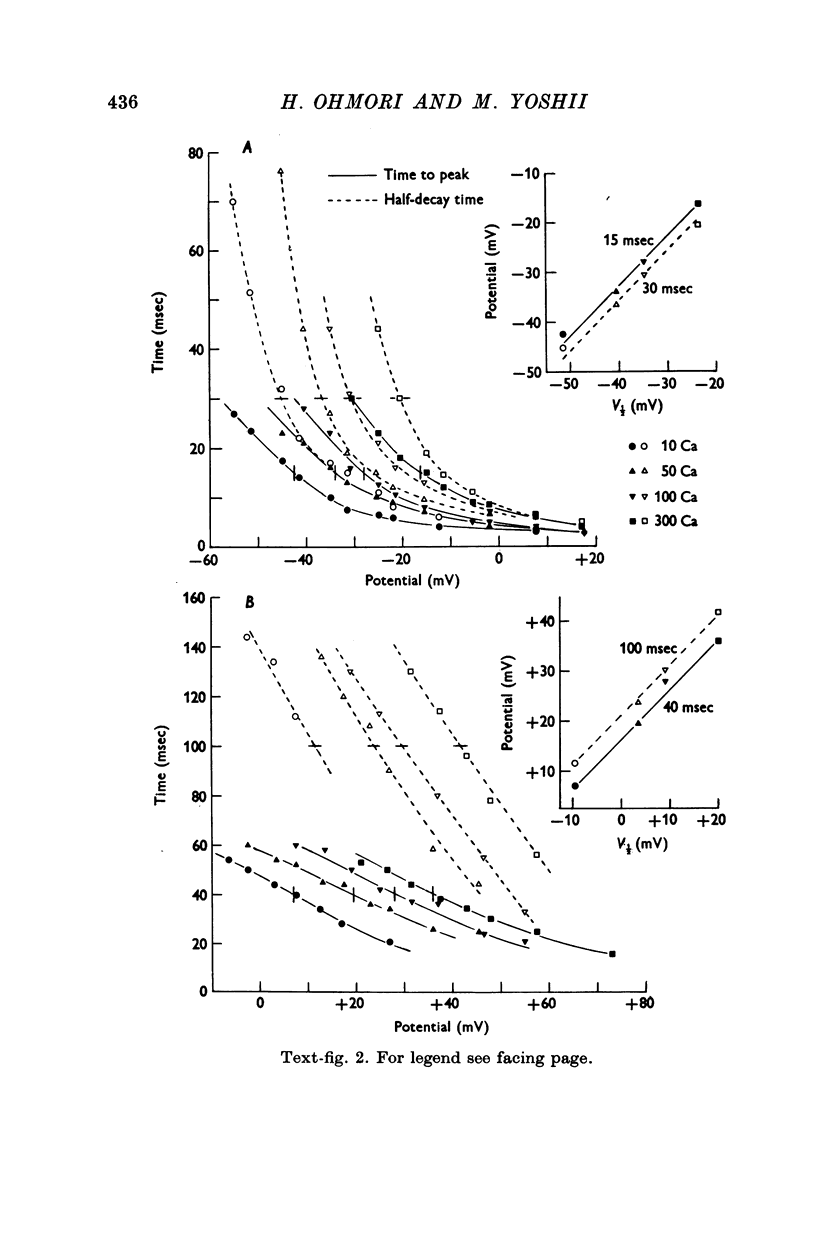
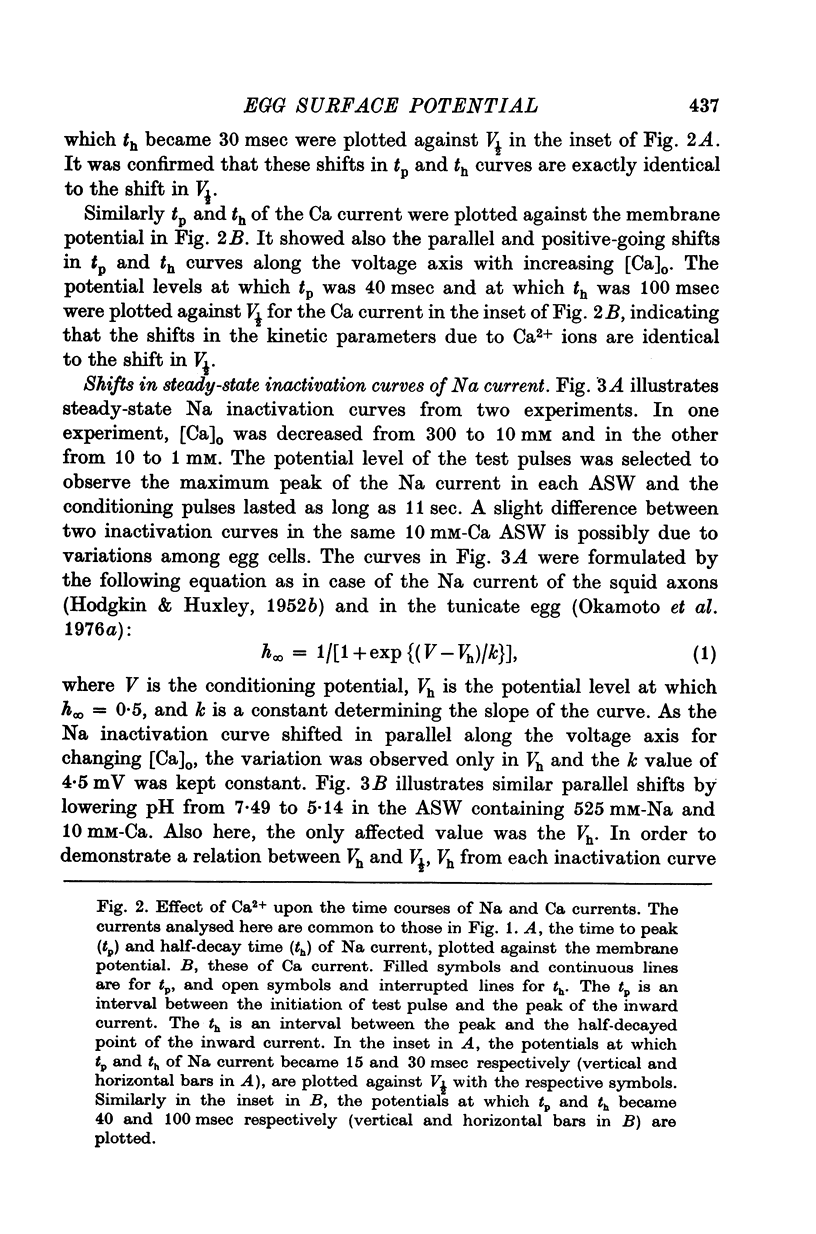
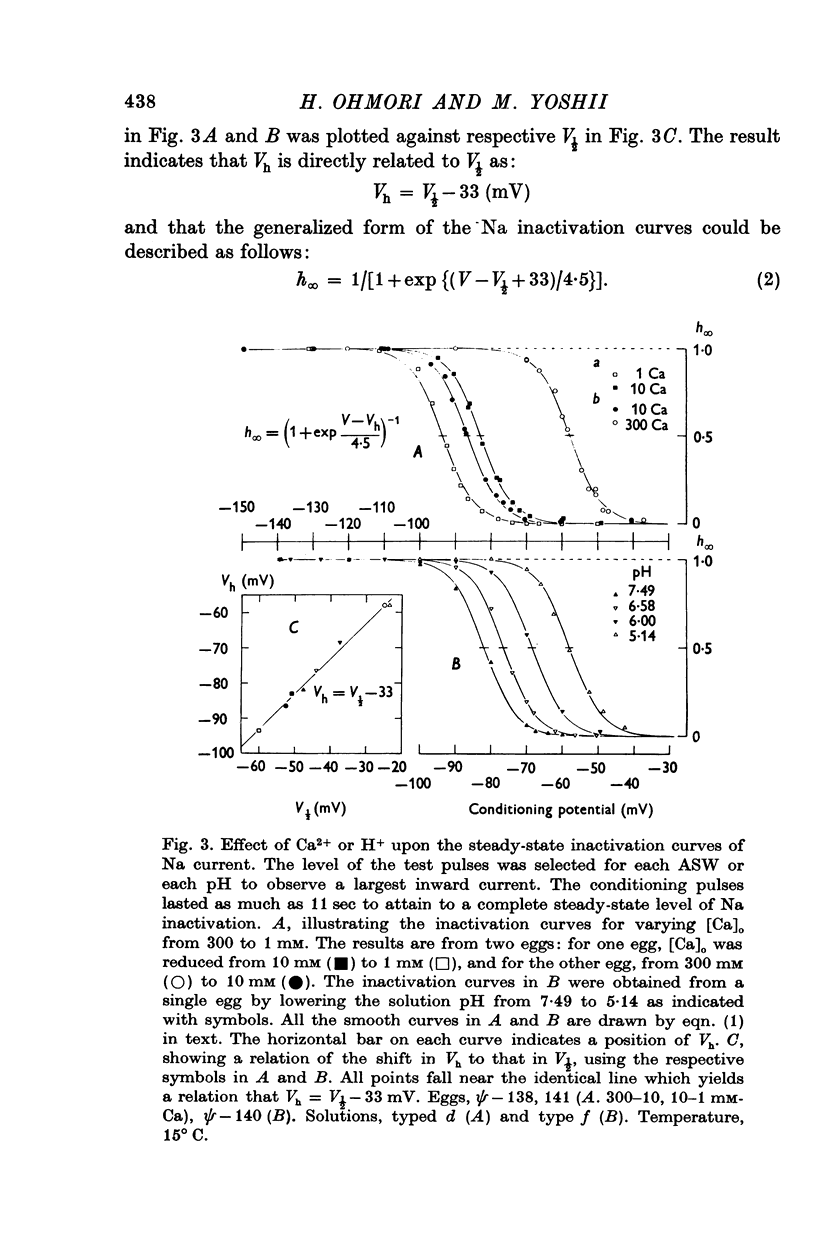
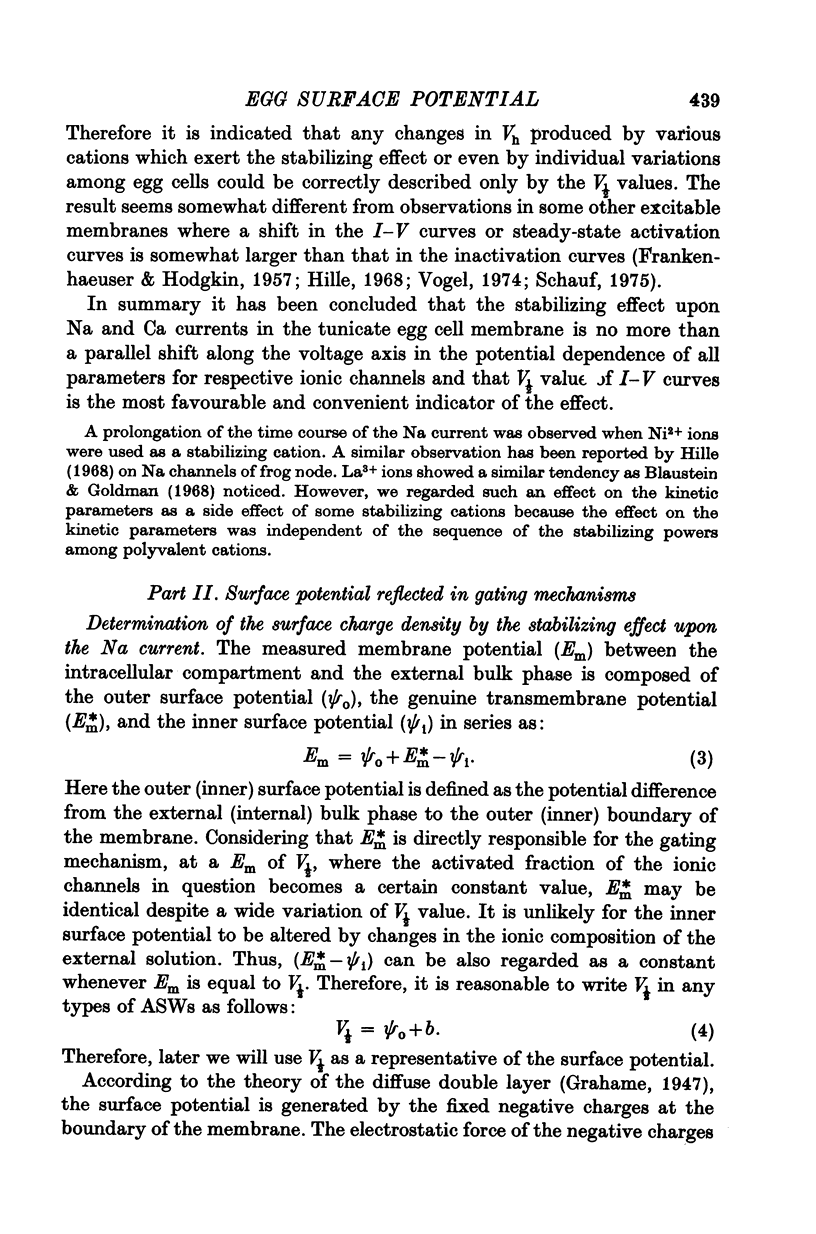
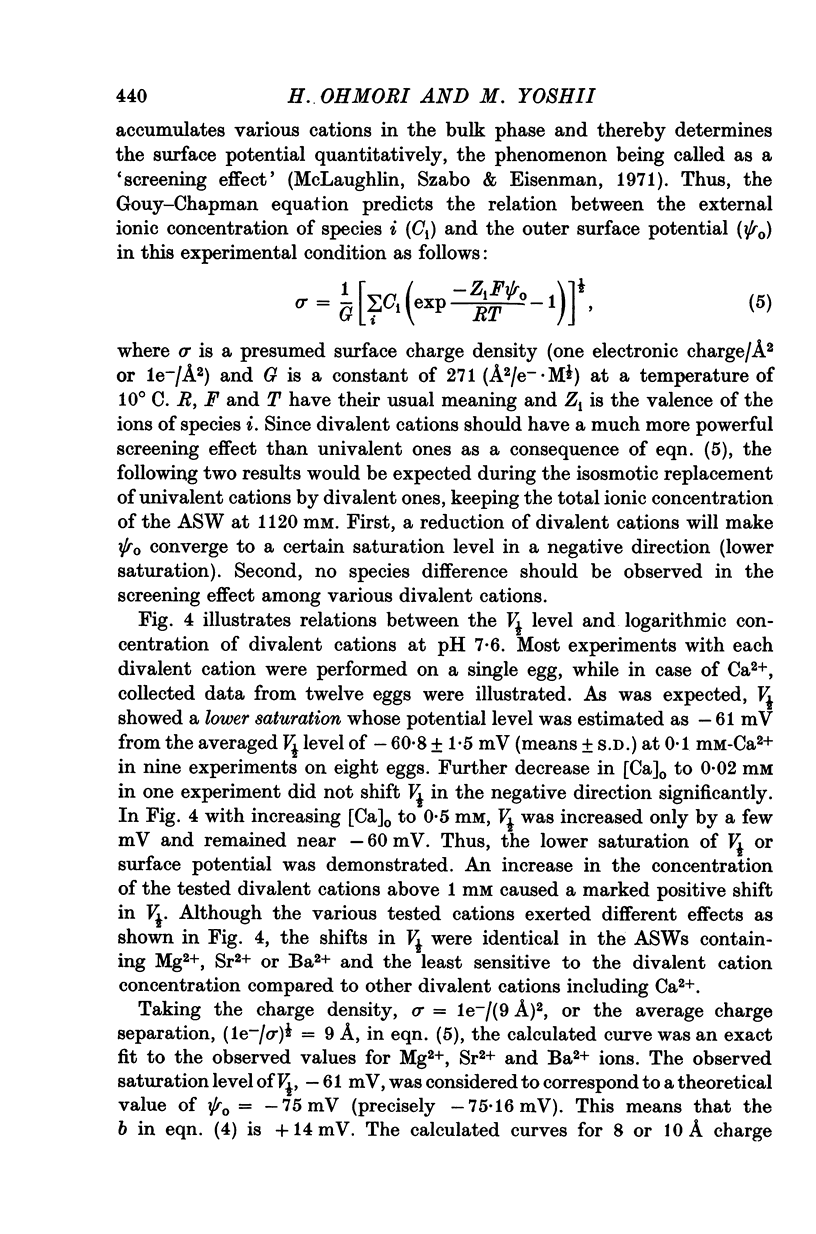
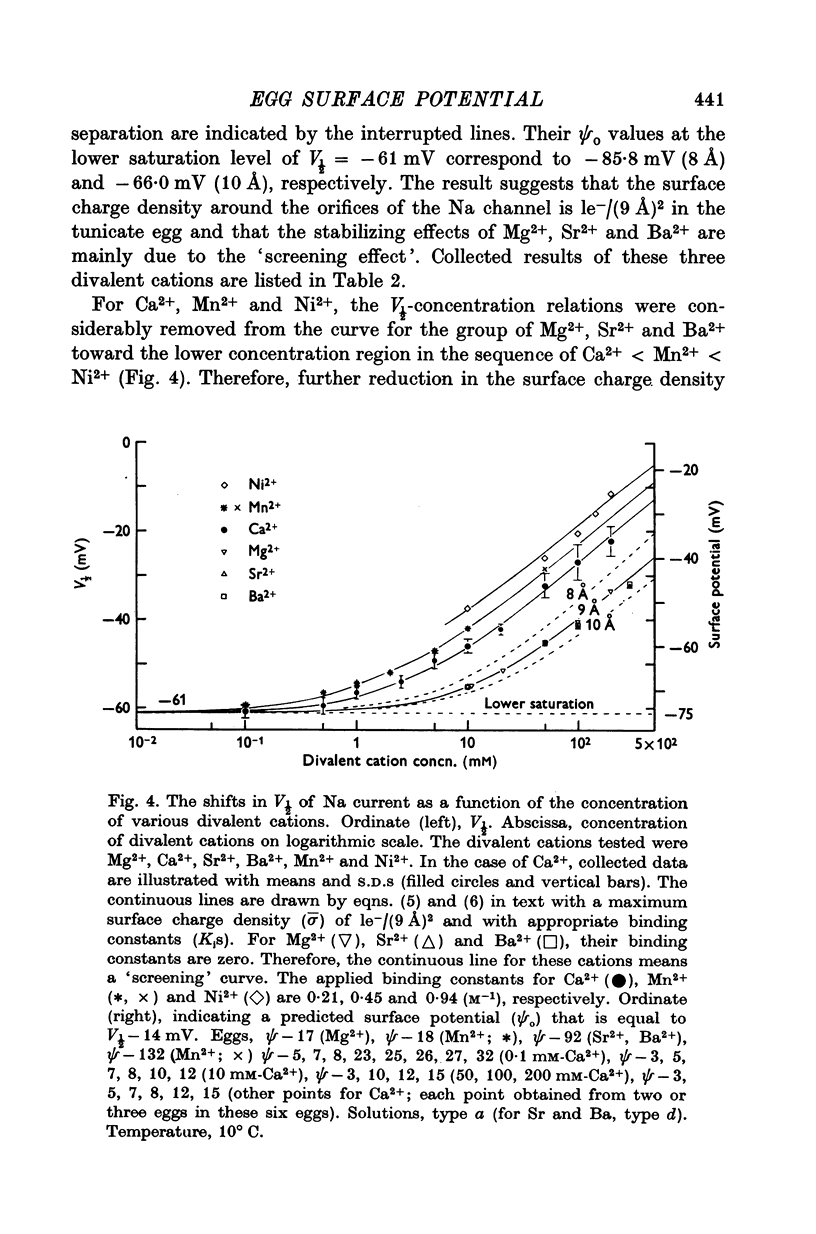
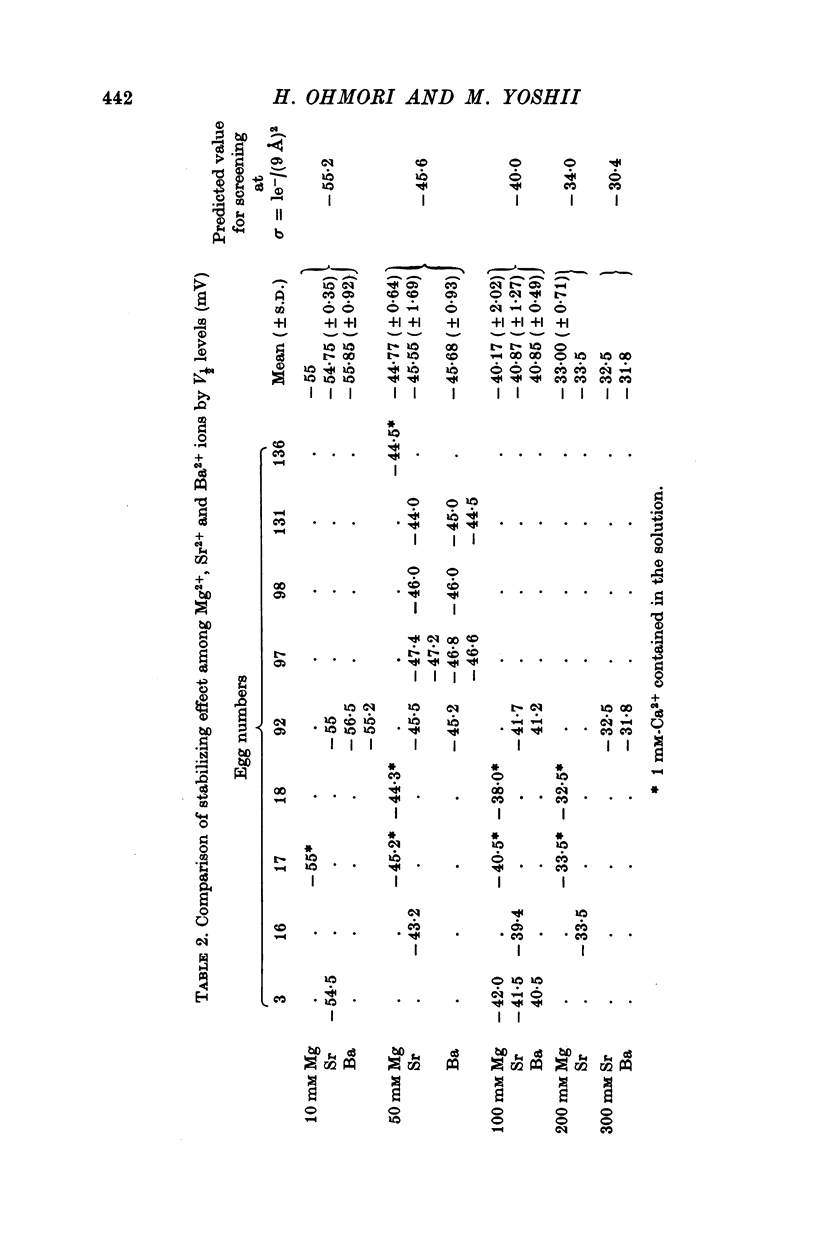
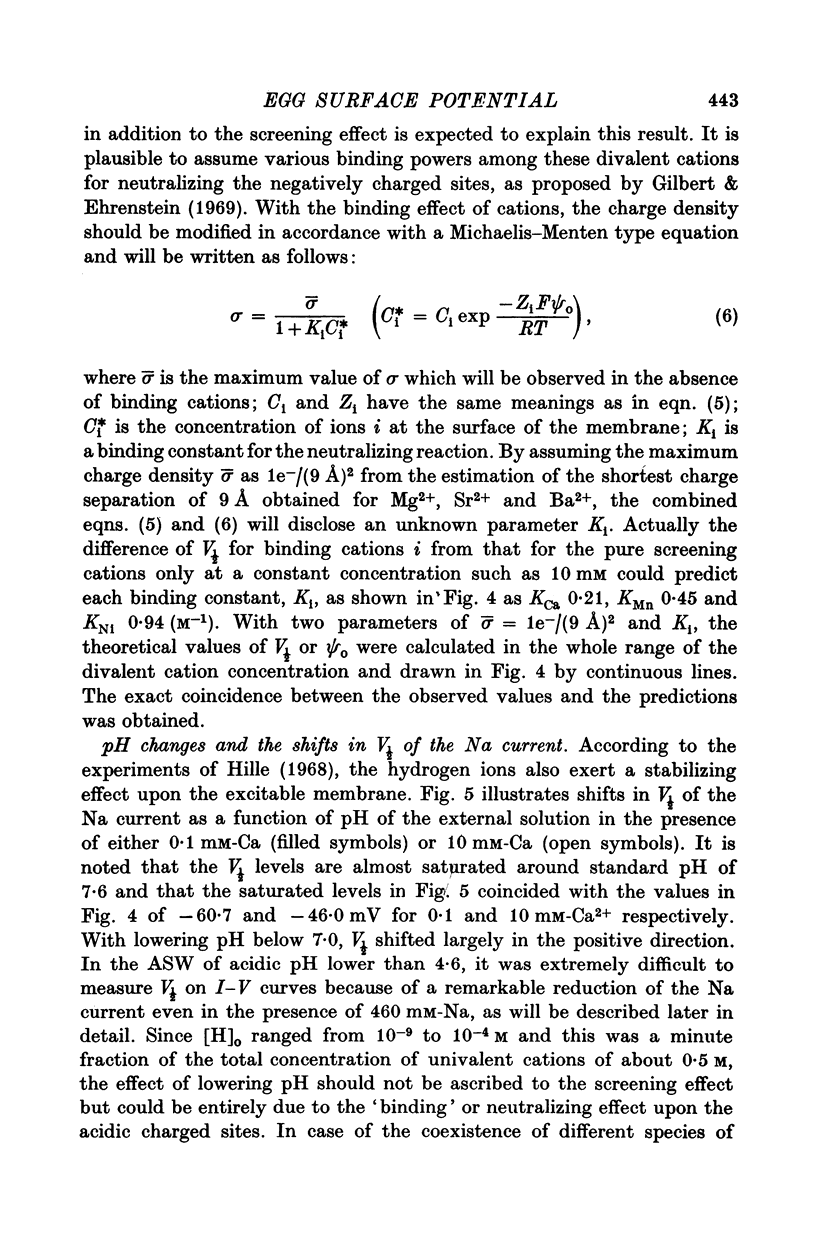
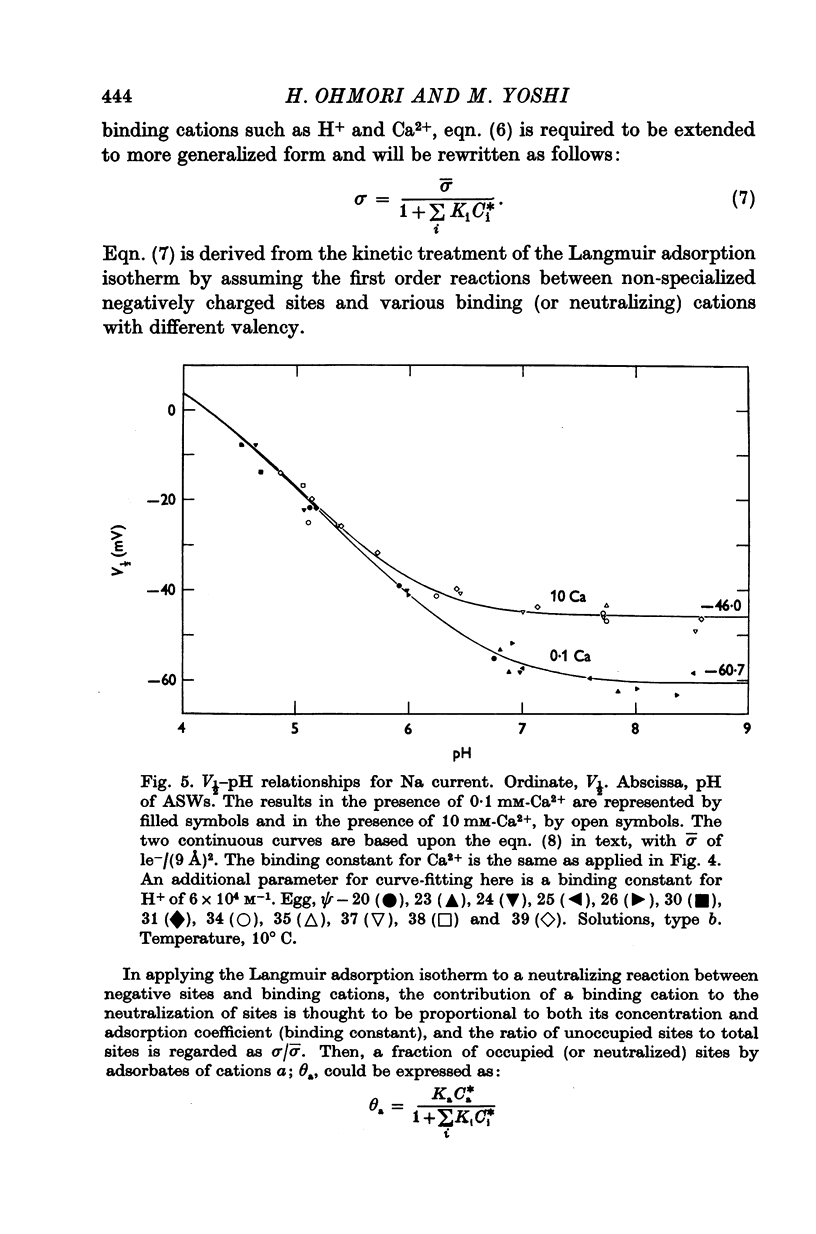
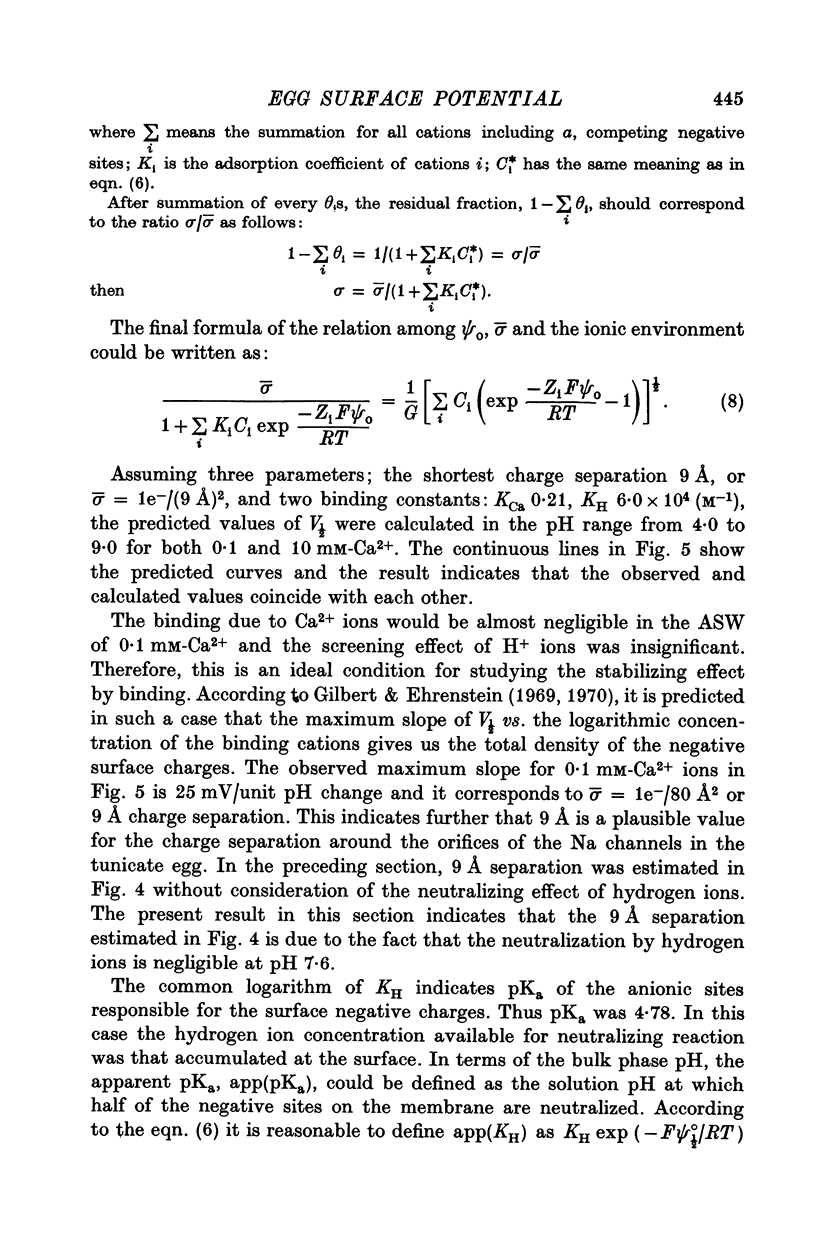
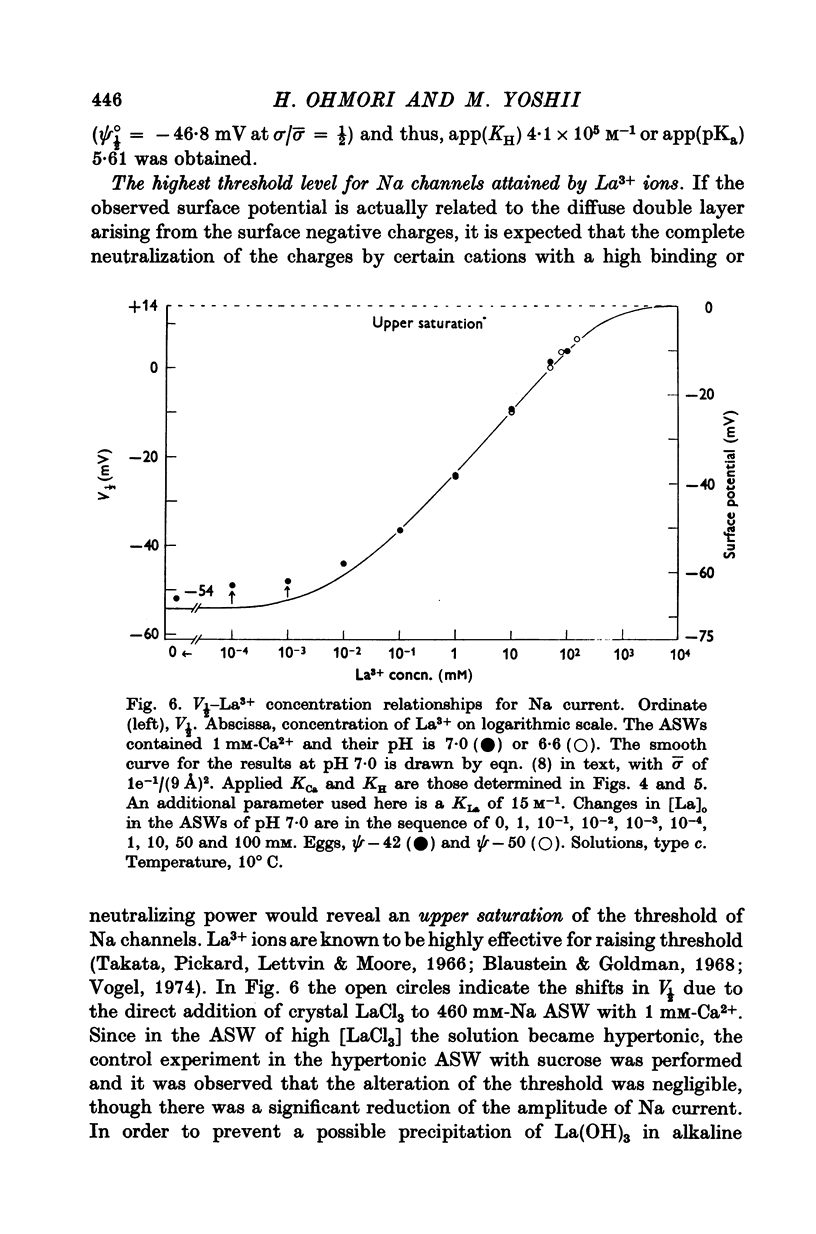
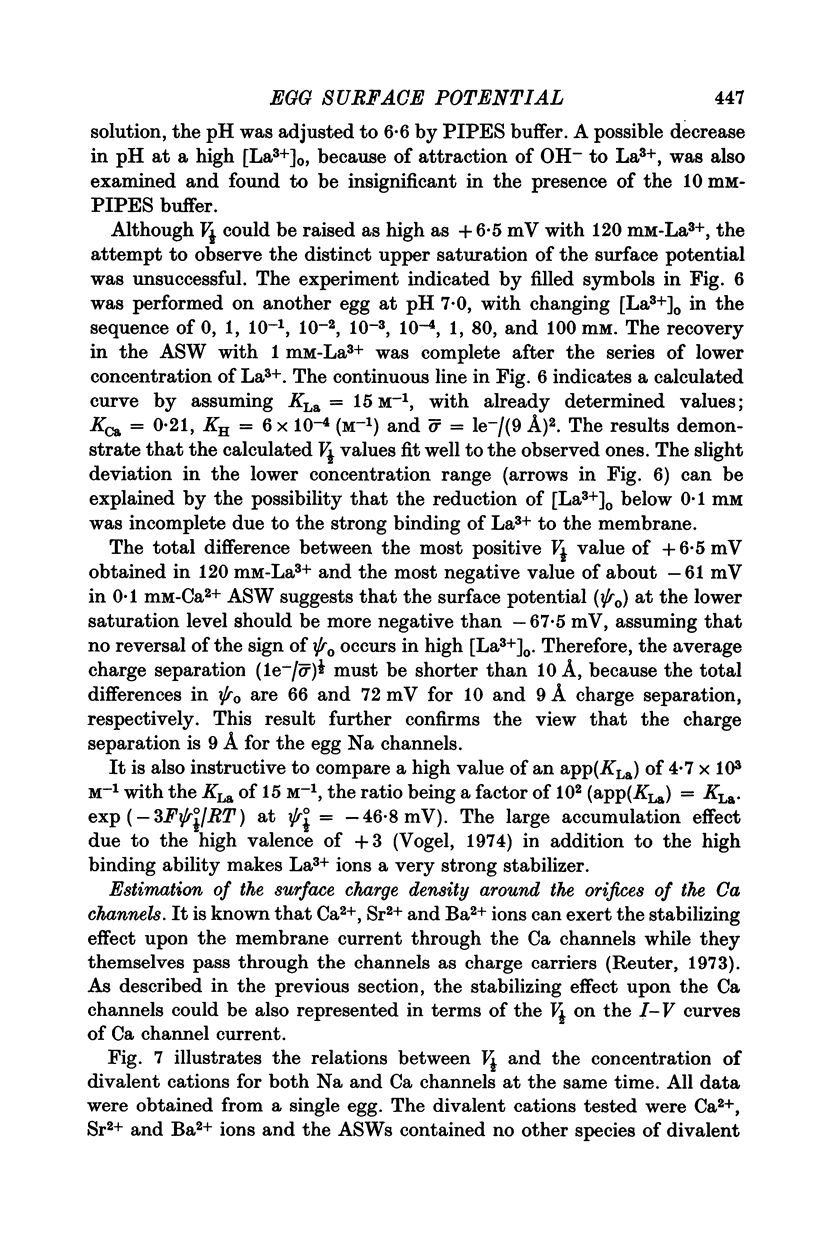
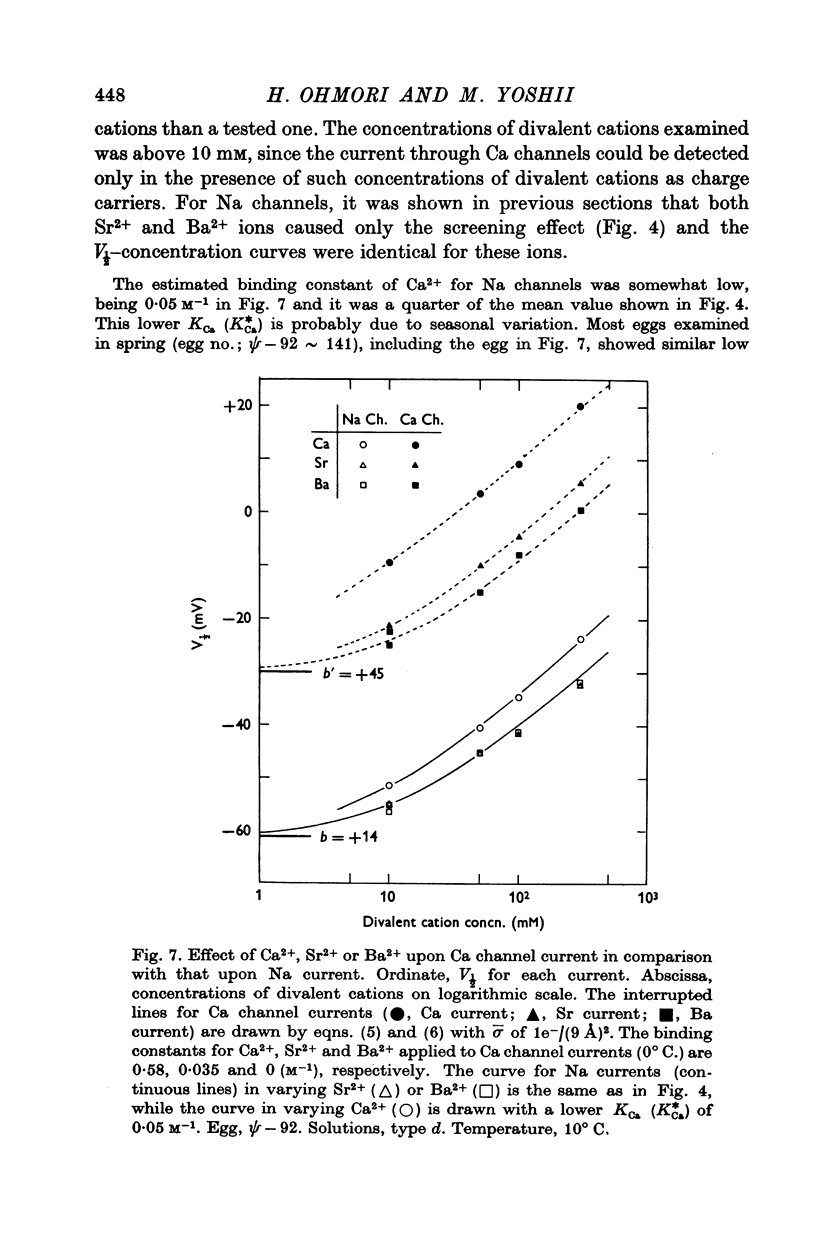
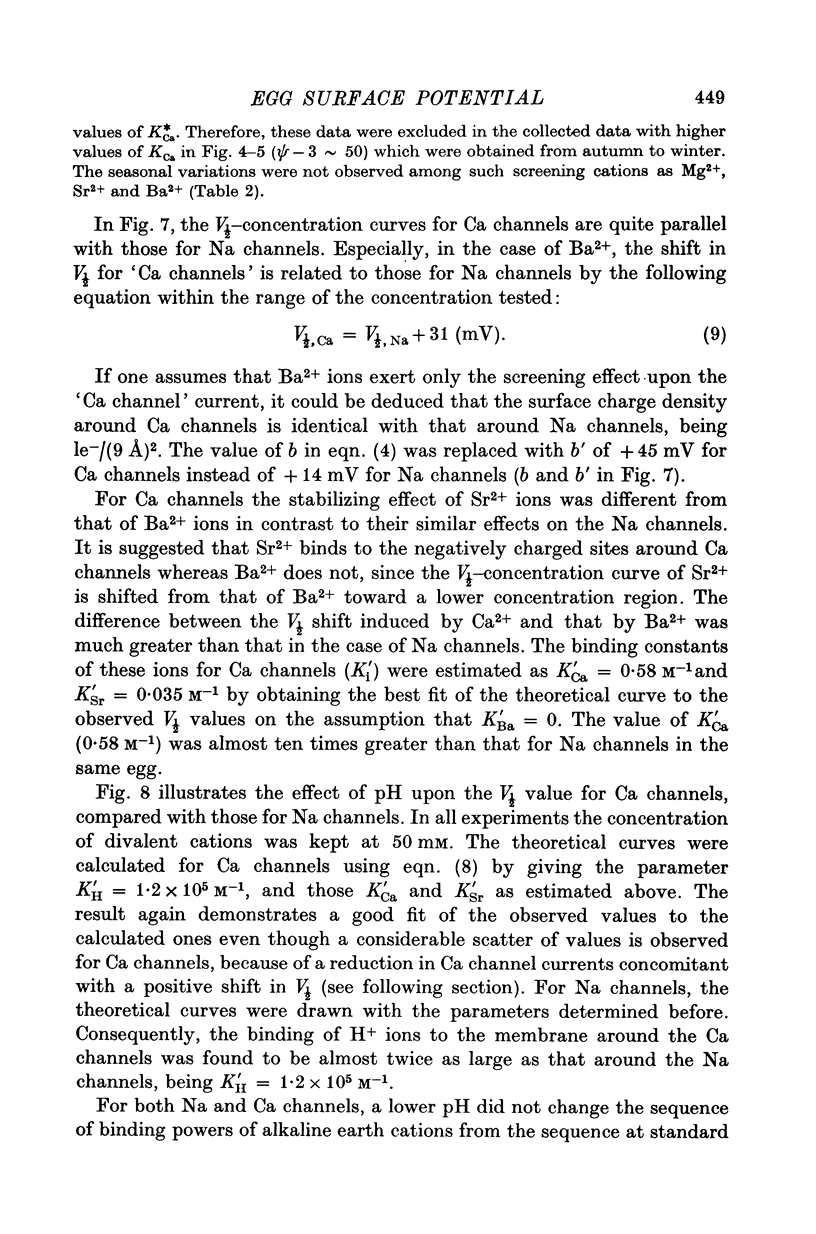
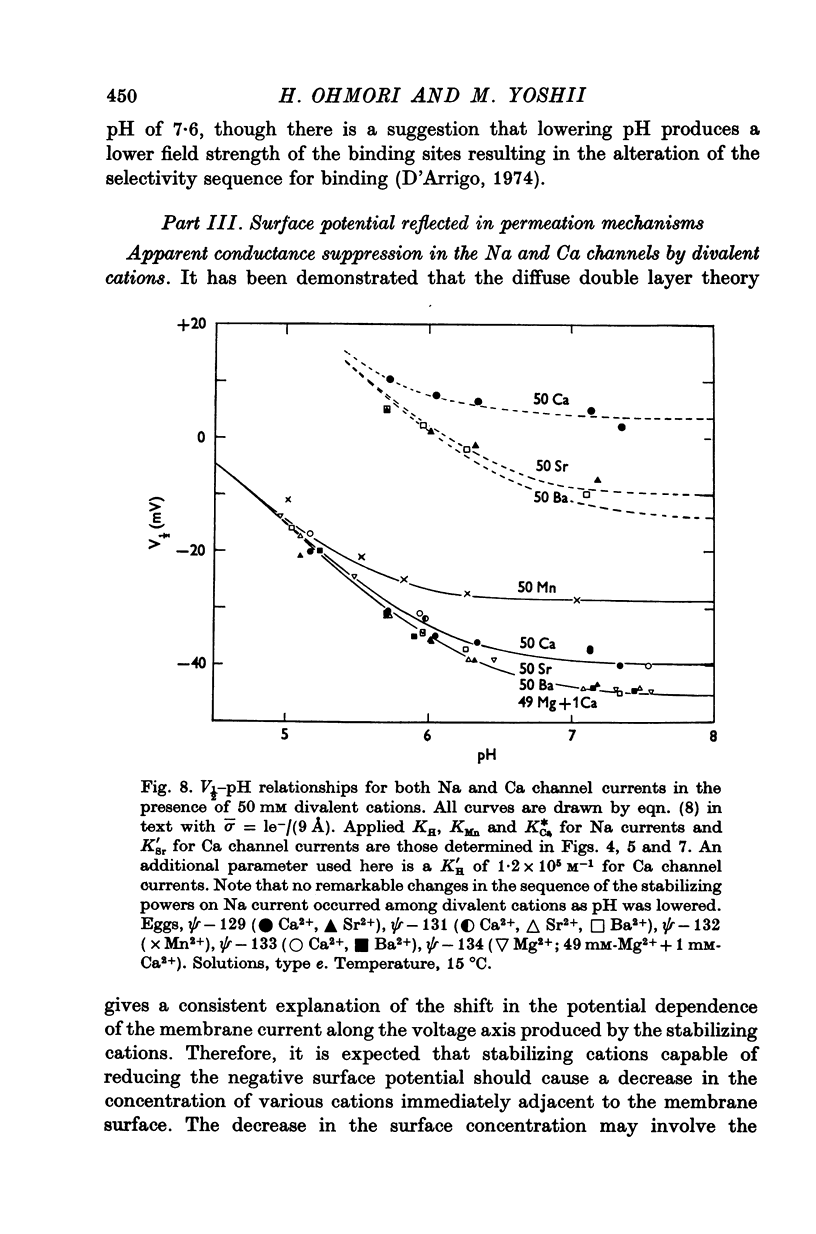
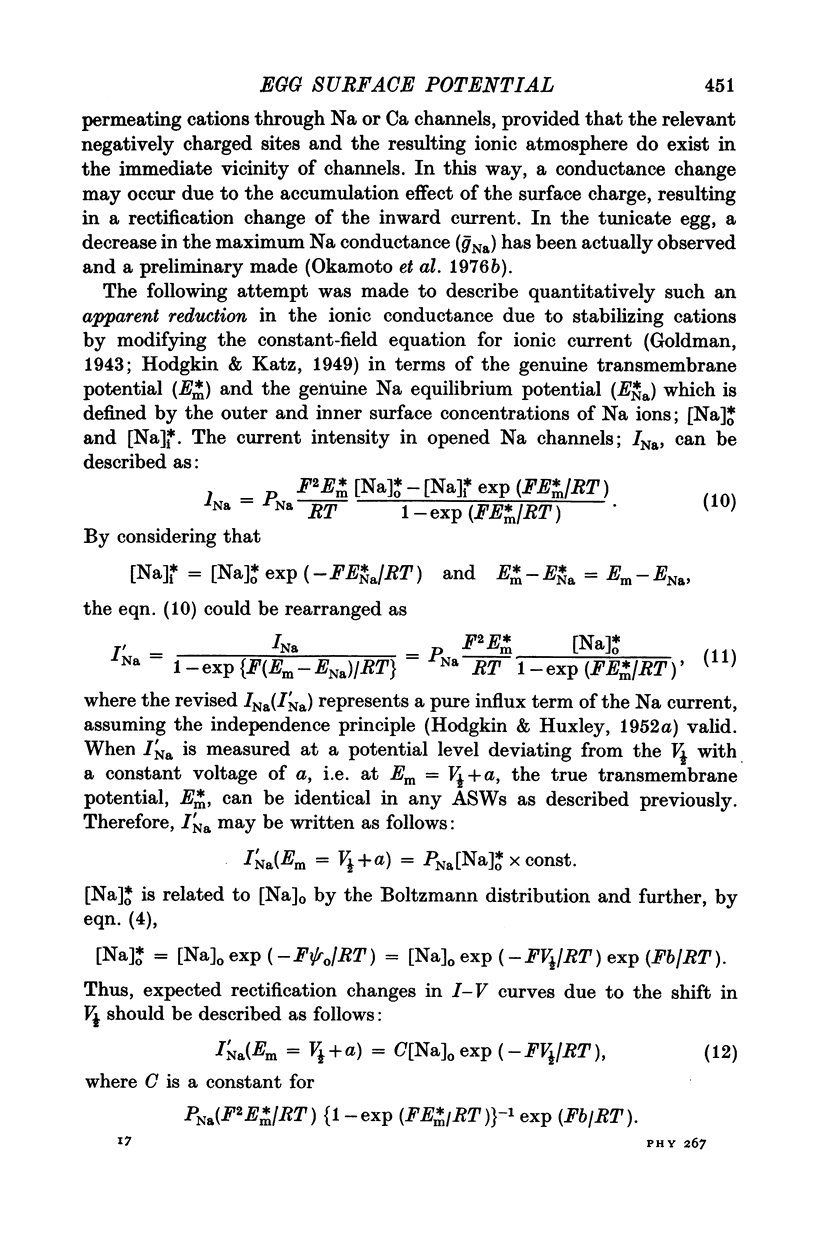
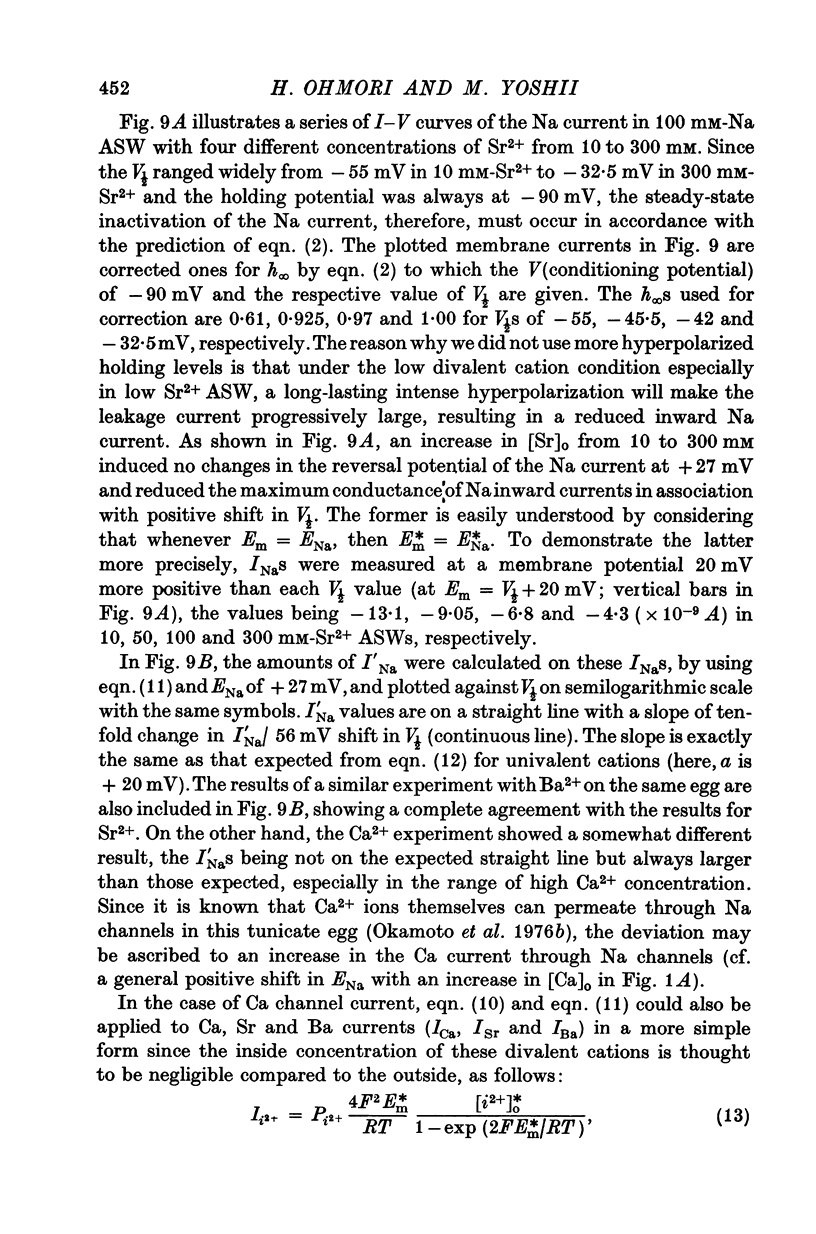
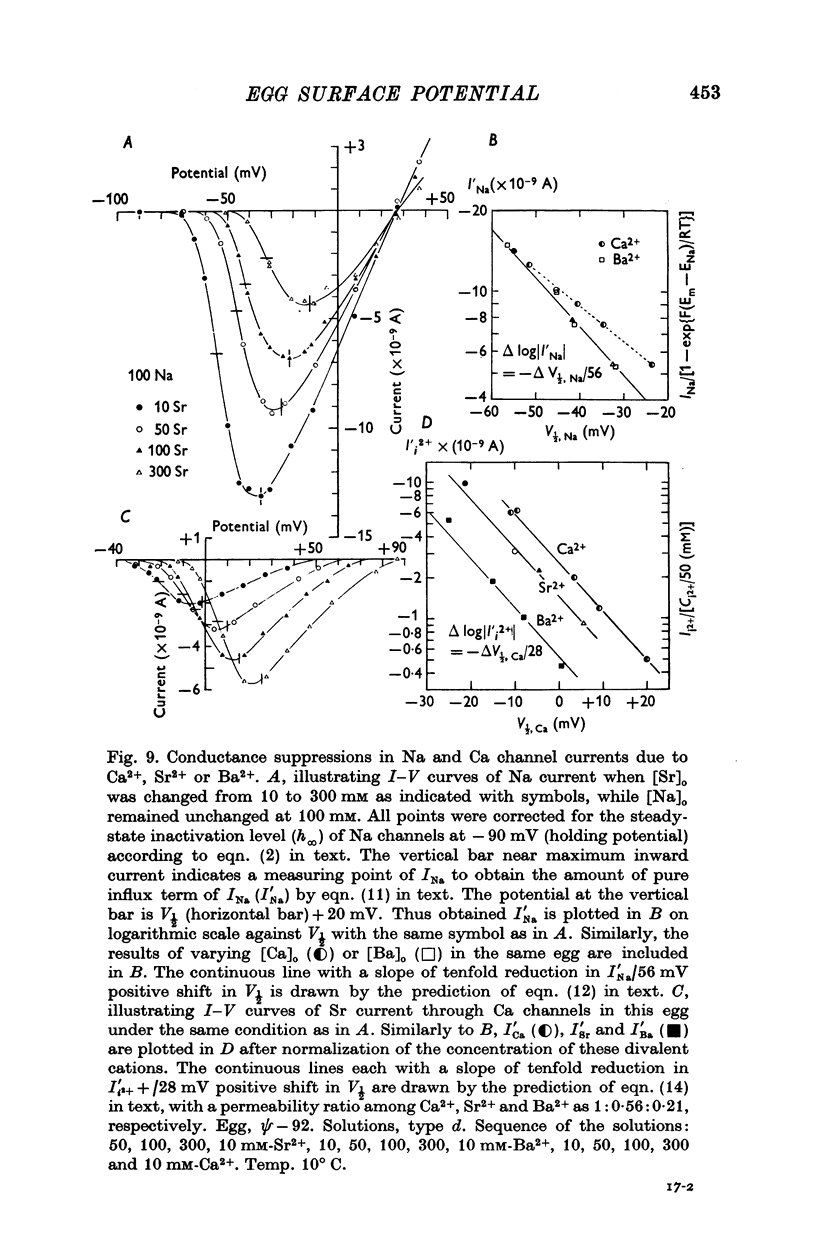
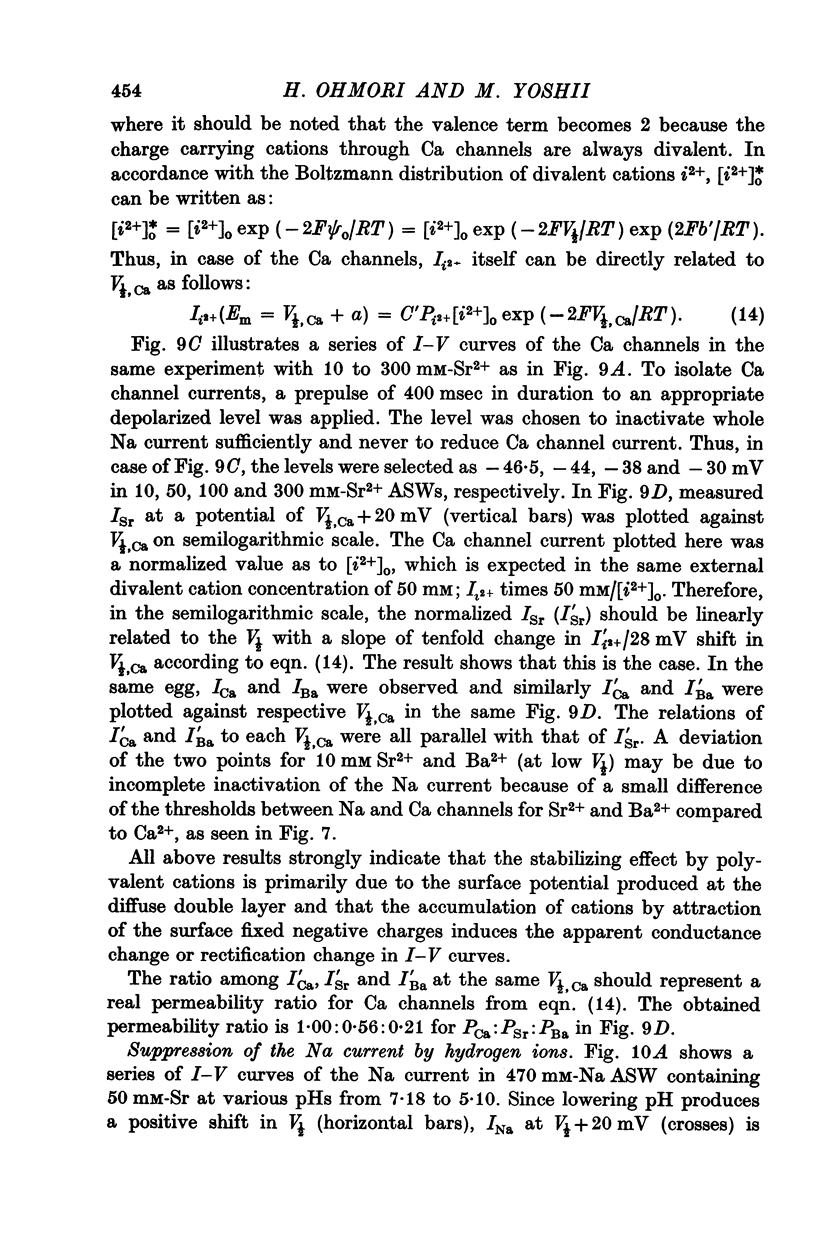
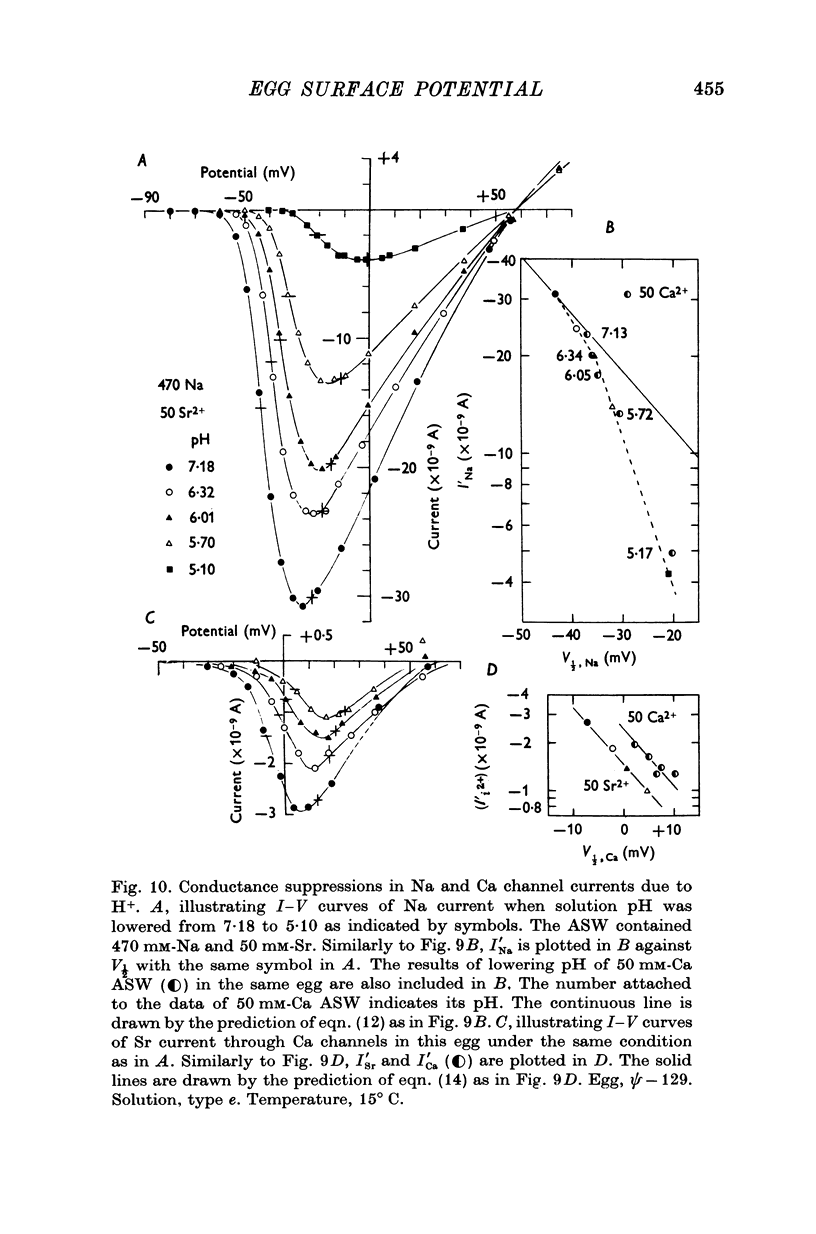
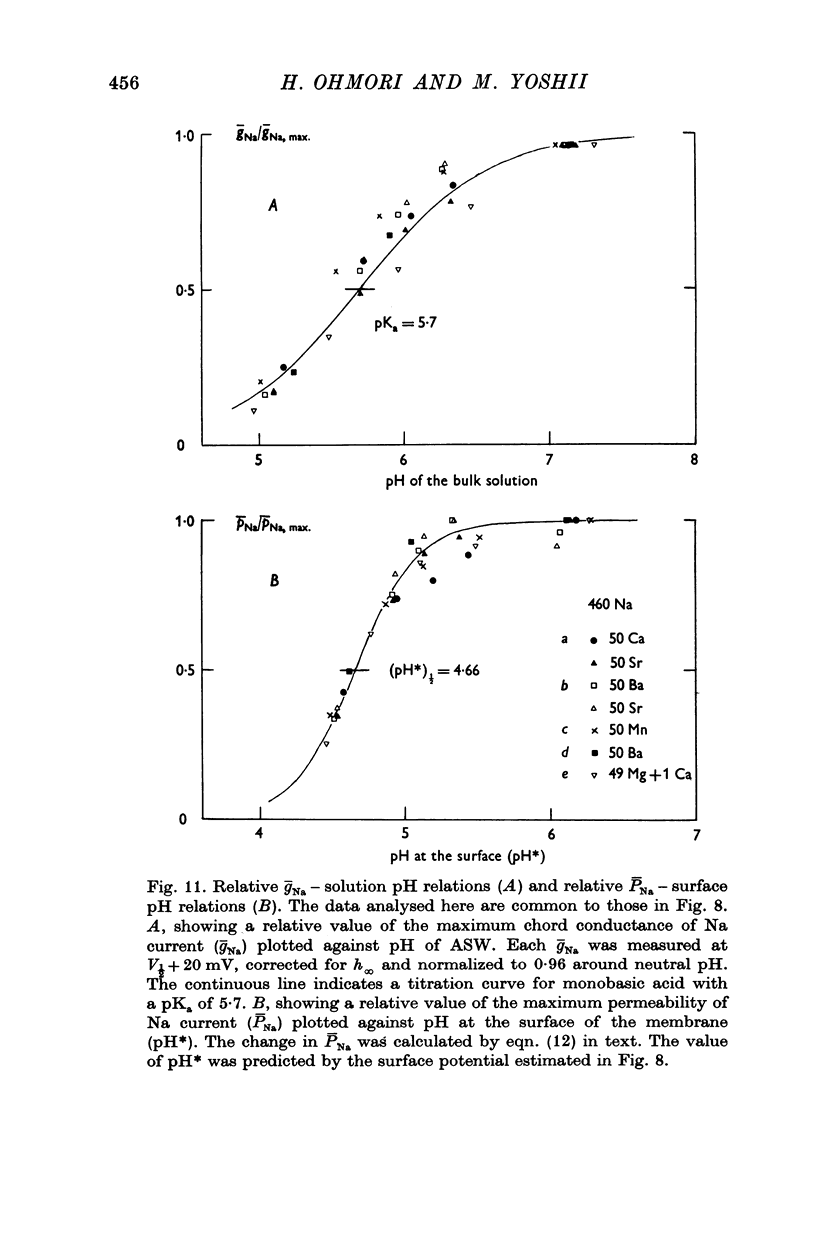
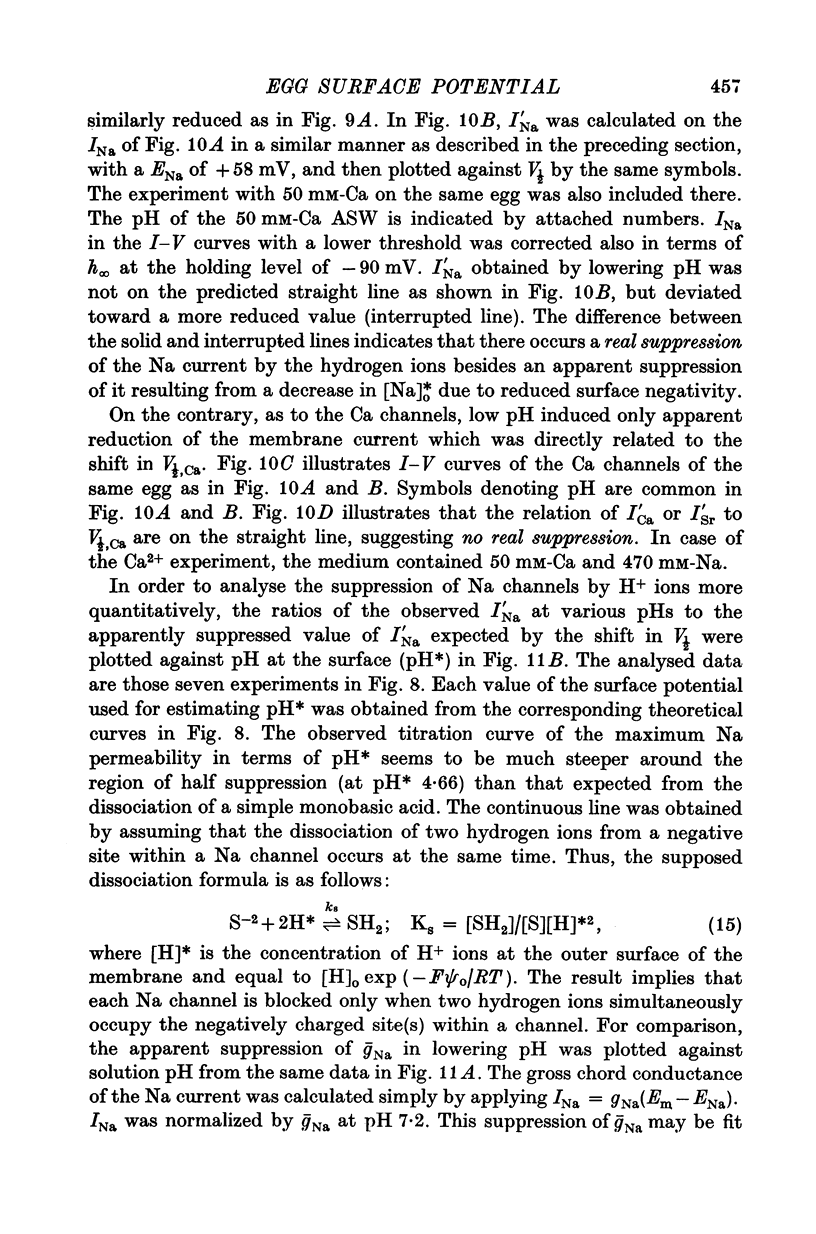
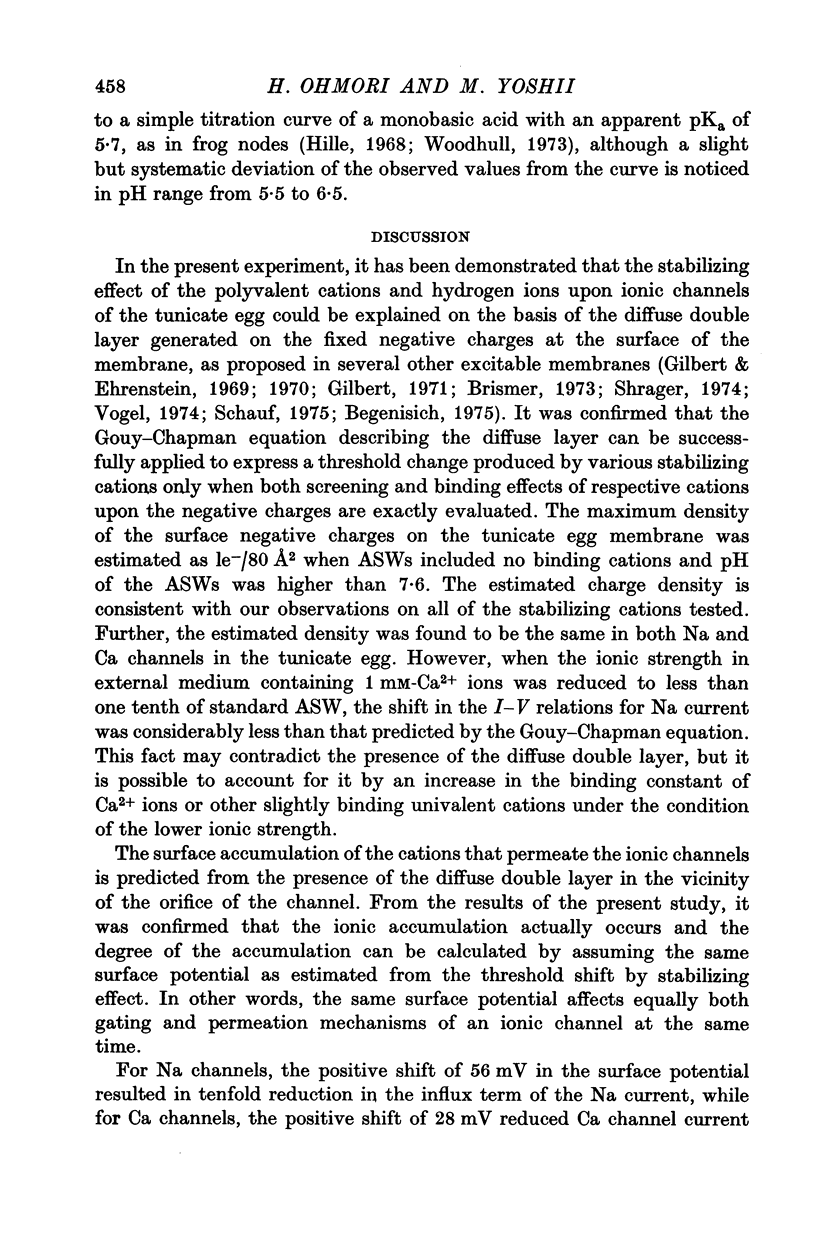
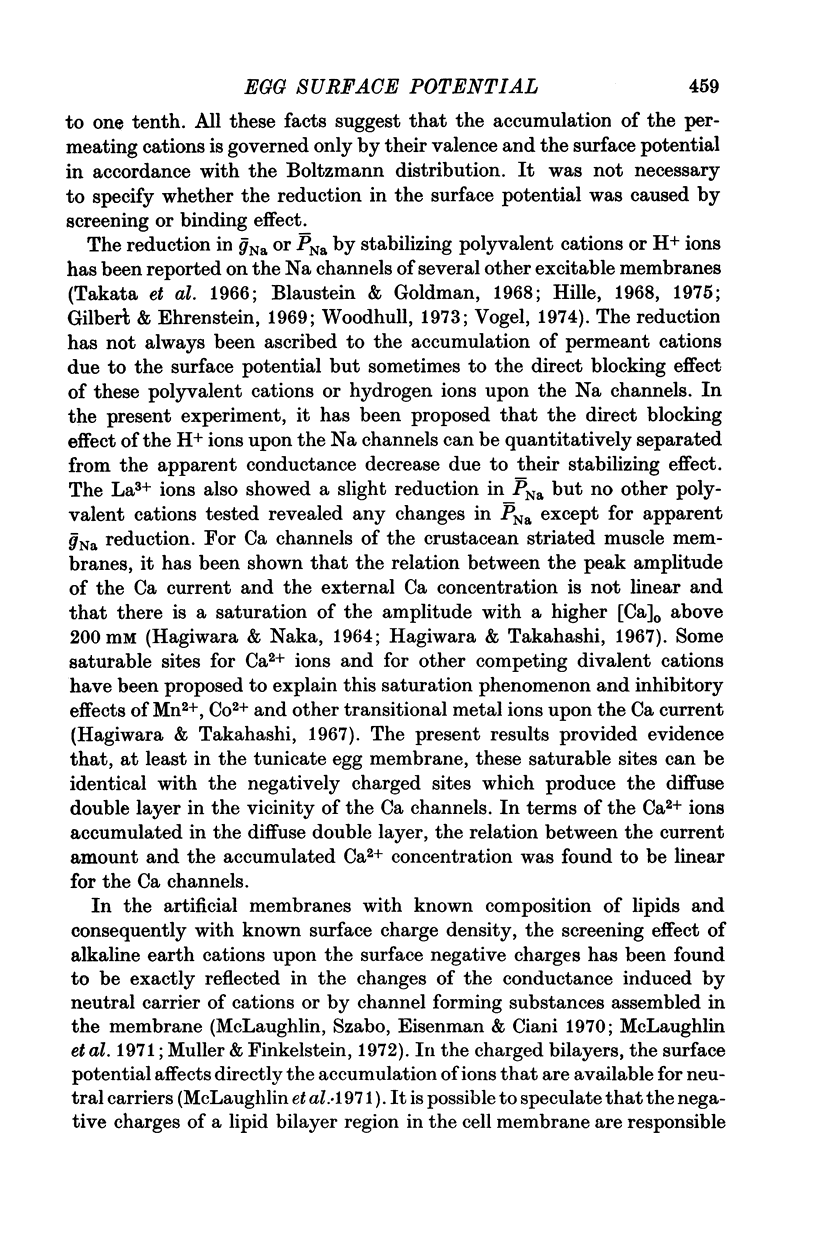
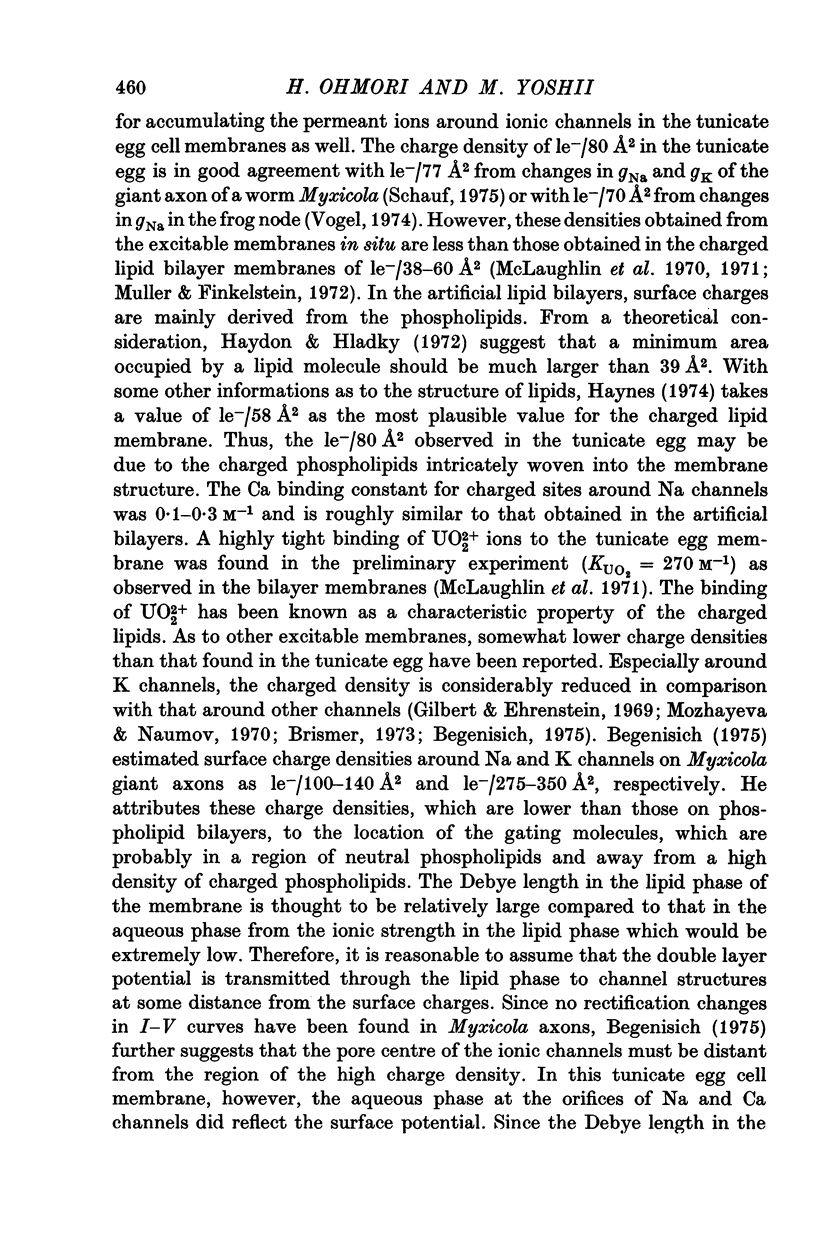
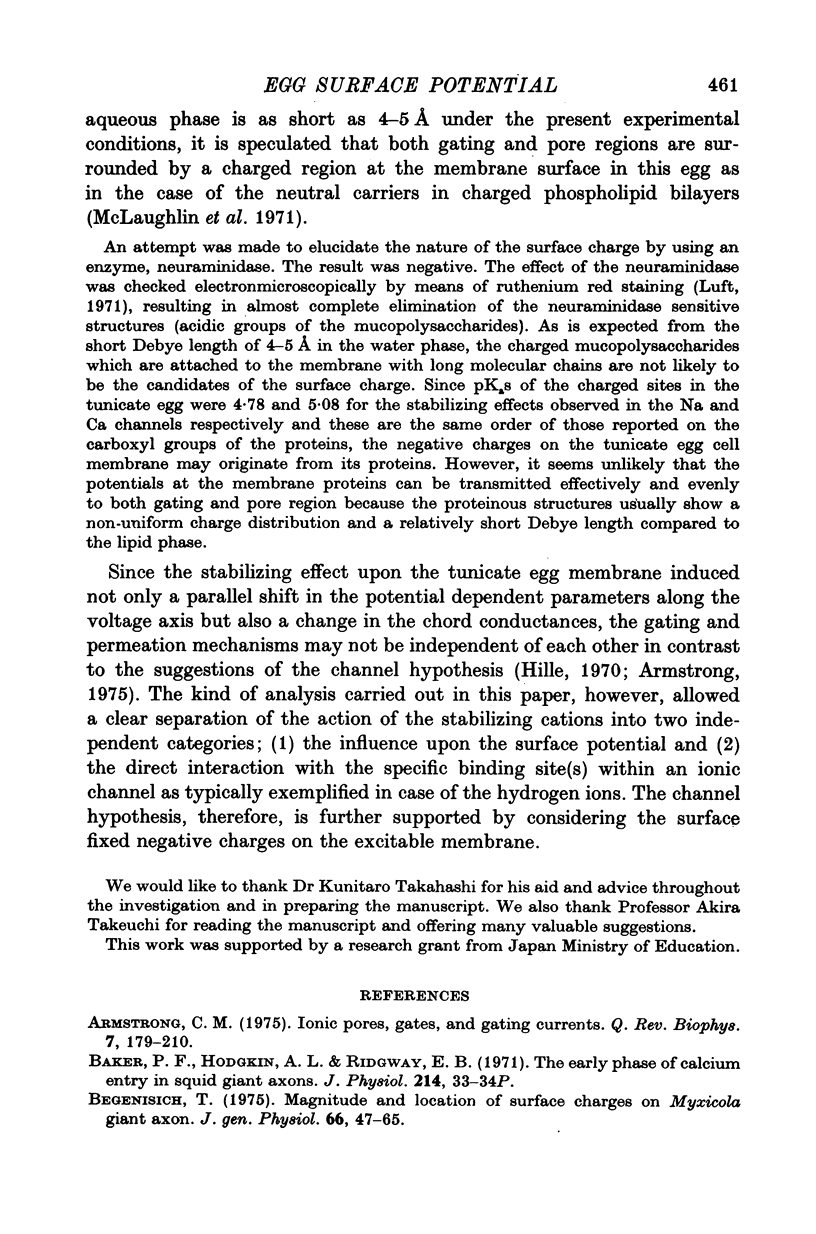
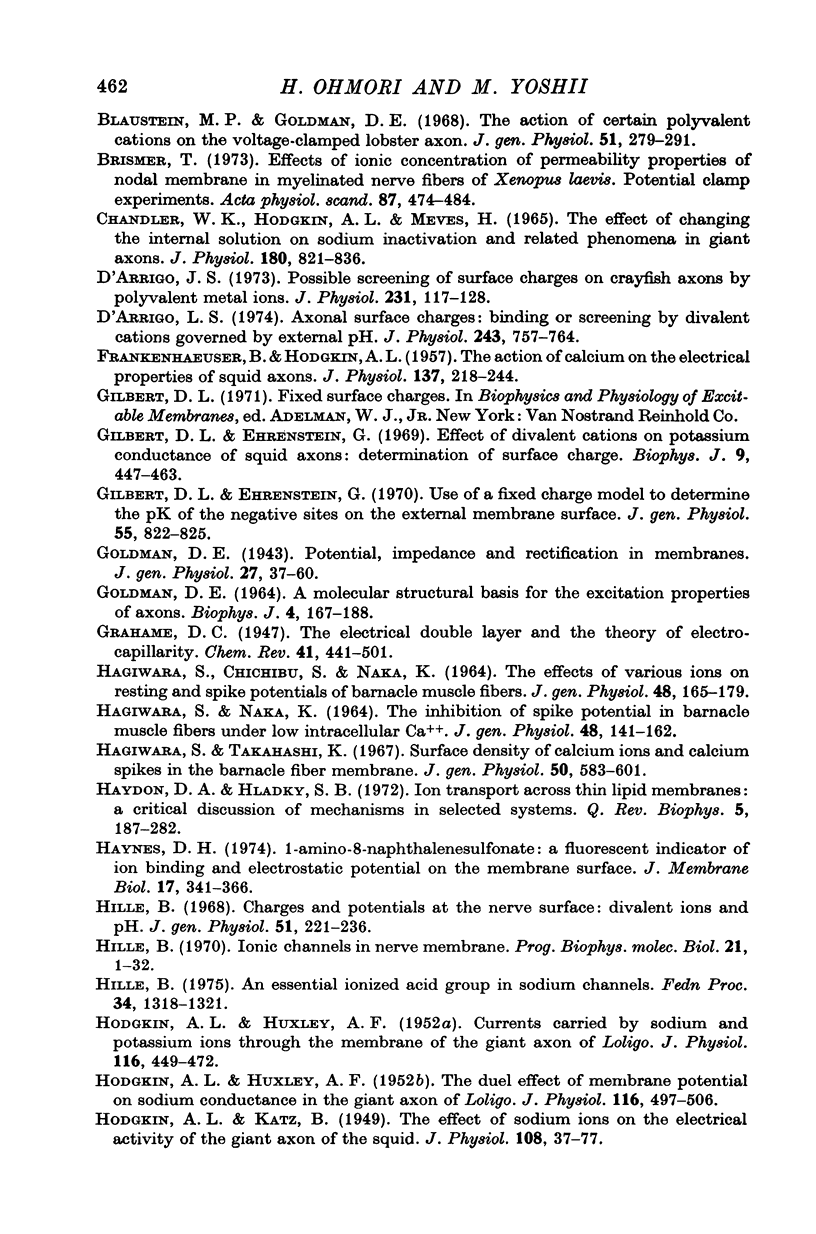
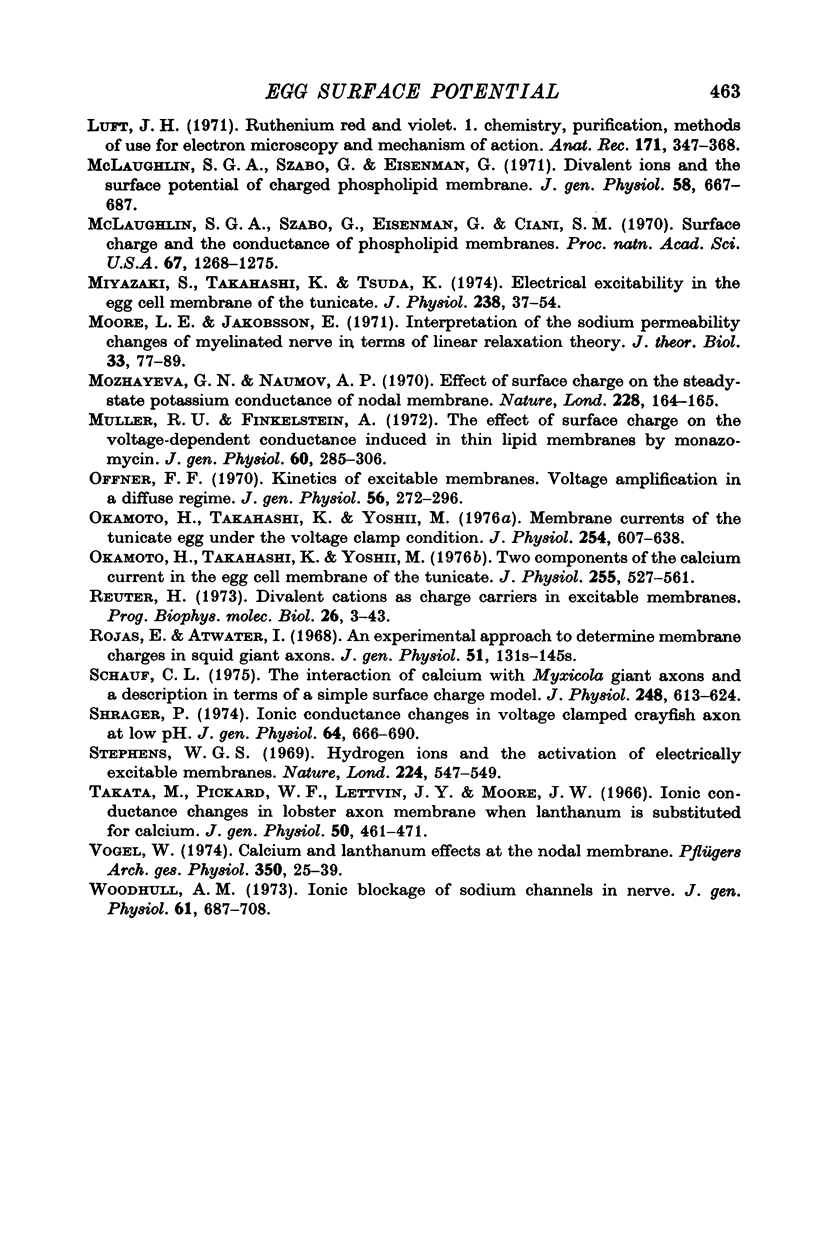
Selected References
These references are in PubMed. This may not be the complete list of references from this article.
- Armstrong C. M. Ionic pores, gates, and gating currents. Q Rev Biophys. 1974 May;7(2):179–210. doi: 10.1017/s0033583500001402. [DOI] [PubMed] [Google Scholar]
- Baker P. F., Hodgkin A. L., Ridgway E. B. The early phase of calcium entry in giant axons of Loligo. J Physiol. 1971;214 (Suppl):33P–34P. [PubMed] [Google Scholar]
- Begenisich T. Magnitude and location of surface charges on Myxicola giant axons. J Gen Physiol. 1975 Jul;66(1):47–65. doi: 10.1085/jgp.66.1.47. [DOI] [PMC free article] [PubMed] [Google Scholar]
- Blaustein M. P., Goldman D. E. The action of certain polyvalent cations on the voltage-clamped lobster axon. J Gen Physiol. 1968 Mar;51(3):279–291. doi: 10.1085/jgp.51.3.279. [DOI] [PMC free article] [PubMed] [Google Scholar]
- Chandler W. K., Hodgkin A. L., Meves H. The effect of changing the internal solution on sodium inactivation and related phenomena in giant axons. J Physiol. 1965 Oct;180(4):821–836. doi: 10.1113/jphysiol.1965.sp007733. [DOI] [PMC free article] [PubMed] [Google Scholar]
- D'Arrigo J. S. Axonal surface charges: binding or screening by divalent cations governed by external pH. J Physiol. 1974 Dec;243(3):757–764. doi: 10.1113/jphysiol.1974.sp010775. [DOI] [PMC free article] [PubMed] [Google Scholar]
- D'Arrigo J. S. Possible screening of surface charges on crayfish axons by polyvalent metal ions. J Physiol. 1973 May;231(1):117–128. doi: 10.1113/jphysiol.1973.sp010223. [DOI] [PMC free article] [PubMed] [Google Scholar]
- FRANKENHAEUSER B., HODGKIN A. L. The action of calcium on the electrical properties of squid axons. J Physiol. 1957 Jul 11;137(2):218–244. doi: 10.1113/jphysiol.1957.sp005808. [DOI] [PMC free article] [PubMed] [Google Scholar]
- GOLDMANN D. E. A MOLECULAR STRUCTURAL BASIS FOR THE EXCITATION PROPERTIES OF AXONS. Biophys J. 1964 May;4:167–188. doi: 10.1016/s0006-3495(64)86776-x. [DOI] [PMC free article] [PubMed] [Google Scholar]
- GRAHAME D. C. The electrical double layer and the theory of electrocapillarity. Chem Rev. 1947 Dec;41(3):441–501. doi: 10.1021/cr60130a002. [DOI] [PubMed] [Google Scholar]
- Gilbert D. L., Ehrenstein G. Effect of divalent cations on potassium conductance of squid axons: determination of surface charge. Biophys J. 1969 Mar;9(3):447–463. doi: 10.1016/S0006-3495(69)86396-4. [DOI] [PMC free article] [PubMed] [Google Scholar]
- Gilbert D. L., Ehrenstein G. Use of a fixed charge model to determine the pK of the negative sites on the external membrane surface. J Gen Physiol. 1970 Jun;55(6):822–825. [PMC free article] [PubMed] [Google Scholar]
- Goldman D. E. POTENTIAL, IMPEDANCE, AND RECTIFICATION IN MEMBRANES. J Gen Physiol. 1943 Sep 20;27(1):37–60. doi: 10.1085/jgp.27.1.37. [DOI] [PMC free article] [PubMed] [Google Scholar]
- HAGIWARA S., CHICHIBU S., NAKA K. I. THE EFFECTS OF VARIOUS IONS ON RESTING AND SPIKE POTENTIALS OF BARNACLE MUSCLE FIBERS. J Gen Physiol. 1964 Sep;48:163–179. doi: 10.1085/jgp.48.1.163. [DOI] [PMC free article] [PubMed] [Google Scholar]
- HAGIWARA S., NAKA K. I. THE INITIATION OF SPIKE POTENTIAL IN BARNACLE MUSCLE FIBERS UNDER LOW INTRACELLULAR CA++. J Gen Physiol. 1964 Sep;48:141–162. doi: 10.1085/jgp.48.1.141. [DOI] [PMC free article] [PubMed] [Google Scholar]
- HODGKIN A. L., HUXLEY A. F. Currents carried by sodium and potassium ions through the membrane of the giant axon of Loligo. J Physiol. 1952 Apr;116(4):449–472. doi: 10.1113/jphysiol.1952.sp004717. [DOI] [PMC free article] [PubMed] [Google Scholar]
- HODGKIN A. L., HUXLEY A. F. The dual effect of membrane potential on sodium conductance in the giant axon of Loligo. J Physiol. 1952 Apr;116(4):497–506. doi: 10.1113/jphysiol.1952.sp004719. [DOI] [PMC free article] [PubMed] [Google Scholar]
- HODGKIN A. L., KATZ B. The effect of sodium ions on the electrical activity of giant axon of the squid. J Physiol. 1949 Mar 1;108(1):37–77. doi: 10.1113/jphysiol.1949.sp004310. [DOI] [PMC free article] [PubMed] [Google Scholar]
- Hagiwara S., Takahashi K. Surface density of calcium ions and calcium spikes in the barnacle muscle fiber membrane. J Gen Physiol. 1967 Jan;50(3):583–601. doi: 10.1085/jgp.50.3.583. [DOI] [PMC free article] [PubMed] [Google Scholar]
- Haydon D. A., Hladky S. B. Ion transport across thin lipid membranes: a critical discussion of mechanisms in selected systems. Q Rev Biophys. 1972 May;5(2):187–282. doi: 10.1017/s0033583500000883. [DOI] [PubMed] [Google Scholar]
- Haynes D. H. 1-Anilino-8-naphthalenesulfonate: a fluorescent indicator of ion binding electrostatic potential on the membrane surface. J Membr Biol. 1974 Jul 12;17(3):341–366. doi: 10.1007/BF01870191. [DOI] [PubMed] [Google Scholar]
- Hille B. An essential ionized acid group in sodium channels. Fed Proc. 1975 Apr;34(5):1318–1321. [PubMed] [Google Scholar]
- Hille B. Charges and potentials at the nerve surface. Divalent ions and pH. J Gen Physiol. 1968 Feb;51(2):221–236. doi: 10.1085/jgp.51.2.221. [DOI] [PMC free article] [PubMed] [Google Scholar]
- Hille B. Ionic channels in nerve membranes. Prog Biophys Mol Biol. 1970;21:1–32. doi: 10.1016/0079-6107(70)90022-2. [DOI] [PubMed] [Google Scholar]
- Luft J. H. Ruthenium red and violet. I. Chemistry, purification, methods of use for electron microscopy and mechanism of action. Anat Rec. 1971 Nov;171(3):347–368. doi: 10.1002/ar.1091710302. [DOI] [PubMed] [Google Scholar]
- McLaughlin S. G., Szabo G., Eisenman G., Ciani S. M. Surface charge and the conductance of phospholipid membranes. Proc Natl Acad Sci U S A. 1970 Nov;67(3):1268–1275. doi: 10.1073/pnas.67.3.1268. [DOI] [PMC free article] [PubMed] [Google Scholar]
- McLaughlin S. G., Szabo G., Eisenman G. Divalent ions and the surface potential of charged phospholipid membranes. J Gen Physiol. 1971 Dec;58(6):667–687. doi: 10.1085/jgp.58.6.667. [DOI] [PMC free article] [PubMed] [Google Scholar]
- Miyazaki S. I., Takahashi K., Tsuda K. Electrical excitability in the egg cell membrane of the tunicate. J Physiol. 1974 Apr;238(1):37–54. doi: 10.1113/jphysiol.1974.sp010509. [DOI] [PMC free article] [PubMed] [Google Scholar]
- Moore L. E., Jakobsson E. Interpretation of the sodium permeability changes of myelinated nerve in terms of linear relaxation theory. J Theor Biol. 1971 Oct;33(1):77–89. doi: 10.1016/0022-5193(71)90217-7. [DOI] [PubMed] [Google Scholar]
- Mozhayeva G. N., Naumov A. P. Effect of surface charge on the steady-state potassium conductance of nodal membrane. Nature. 1970 Oct 10;228(5267):164–165. doi: 10.1038/228164a0. [DOI] [PubMed] [Google Scholar]
- Muller R. U., Finkelstein A. The effect of surface charge on the voltage-dependent conductance induced in thin lipid membranes by monazomycin. J Gen Physiol. 1972 Sep;60(3):285–306. doi: 10.1085/jgp.60.3.285. [DOI] [PMC free article] [PubMed] [Google Scholar]
- Offner F. F. Kinetics of excitable membranes. Voltage amplification in a diffusion regime. J Gen Physiol. 1970 Aug;56(2):272–296. doi: 10.1085/jgp.56.2.272. [DOI] [PMC free article] [PubMed] [Google Scholar]
- Okamoto H., Takahashi K., Yoshii M. Membrane currents of the tunicate egg under the voltage-clamp condition. J Physiol. 1976 Jan;254(3):607–638. doi: 10.1113/jphysiol.1976.sp011249. [DOI] [PMC free article] [PubMed] [Google Scholar]
- Okamoto H., Takahashi K., Yoshii M. Two components of the calcium current in the egg cell membrane of the tunicate. J Physiol. 1976 Feb;255(2):527–561. doi: 10.1113/jphysiol.1976.sp011294. [DOI] [PMC free article] [PubMed] [Google Scholar]
- Reuter H. Divalent cations as charge carriers in excitable membranes. Prog Biophys Mol Biol. 1973;26:1–43. doi: 10.1016/0079-6107(73)90016-3. [DOI] [PubMed] [Google Scholar]
- Rojas E., Atwater I. An experimental approach to determine membrane charges in squid giant axons. J Gen Physiol. 1968 May;51(5 Suppl):131S+–131S+. [PubMed] [Google Scholar]
- Schauf C. L. The interactions of calcium with mpyxicola giant axons and a description in terms of a simple surface charge model. J Physiol. 1975 Jul;248(3):613–624. doi: 10.1113/jphysiol.1975.sp010991. [DOI] [PMC free article] [PubMed] [Google Scholar]
- Shrager P. Ionic conductance changes in voltage clamped crayfish axons at low pH. J Gen Physiol. 1974 Dec;64(6):666–690. doi: 10.1085/jgp.64.6.666. [DOI] [PMC free article] [PubMed] [Google Scholar]
- Stephens W. G. Hydrogen ion and the activation of electrically excitable membranes. Nature. 1969 Nov 8;224(5219):547–549. doi: 10.1038/224547a0. [DOI] [PubMed] [Google Scholar]
- Takata M., Pickard W. F., Lettvin J. Y., Moore J. W. Ionic conductance changes in lobster axon membrane when lanthanum is substituted for calcium. J Gen Physiol. 1966 Nov;50(2):461–471. doi: 10.1085/jgp.50.2.461. [DOI] [PMC free article] [PubMed] [Google Scholar]
- Vogel W. Calcium and lanthanum effects at the nodal membrane. Pflugers Arch. 1974;350(1):25–39. doi: 10.1007/BF00586736. [DOI] [PubMed] [Google Scholar]
- Woodhull A. M. Ionic blockage of sodium channels in nerve. J Gen Physiol. 1973 Jun;61(6):687–708. doi: 10.1085/jgp.61.6.687. [DOI] [PMC free article] [PubMed] [Google Scholar]