Abstract
1. The action potential and the membrane current of the mouse oocyte were analysed by current-clamp and voltage-clamp techniques and they were compared with those of other animal oocytes. 2. The matured and unfertilized oocyte of the mouse in standard medium with 6 mM-K showed the resting potential of -23-1+/-2-9 mV. The resting potential was relatively large in the medium with 20 mM-Ca or 10 mM-Mn, being -35-7+/-2-6 mV and further increased to -46-9+/-4-8 mV with replacement of Na in the medium by choline. 3. At the cessation of large hyperpolarization below -90 mV in standard medium, a regenerative potential was often elicited in the form of an off-response. The off-response depended upon the external concentration of Ca. In 20 mM-Ca medium it was constantly observed with hyperpolarization below -60 mV. Its critical level was -40 mV and its overshoot was +15 mV. 4. The time and potential-dependent inward current was observed both in standard and 20 mM-Ca media under voltage-clamp condition. In 20 mM-Ca medium the inward current was observed by depolarization beyond -40 mV and showed its maximum at -15 mV. It was greatly reduced by replacing the external Ca with Mn but retained by substituting Sr or Ba for Ca. The selectivity ratios among these alkali earth cations were Ca:Sr:Ba=1-0:1-4:0-7. 5. The current-voltage relation in Ca and Na-deficient and 10 mM-Mn medium was linear from -200 to +25 mV. The hyperpolarization below -200 mV revealed an inward-going rectification. The depolarization above +50 mV under voltage-clamp condition induced the outward surge current with activation and inactivation processes. 6. In contrast to the mouse oocyte, the matured and unfertilized oocyte of the sea urchin showed a large resting potential of -70 mV in 30 Ca ASW and the depolarization beyond -40 mV elicited an action potential with an overshoot of 20 mV. The action potential showed a notch in the rising phase and lasted about 1 to 2 sec. 7. Under the voltage-clamp condition both Ca inward current and the outward surge current were observed in the sea urchin oocyte membrane just as in the mouse oocyte membrane. 8. The selectivity ratios among alkali earth cations, Ca:Sr:Ba, for 'Ca channels' of the oocyte membranes were 1-0:1-4:0-7 in the mouse, 1-0:1-7:1-1 in the tunicate and 1-0:0-7:0-5 in the sea urchin. When the current density through Ca channels are revised in terms of the respective critical levels for Ca channels, the revised selectivity sequences become Ca greater than Sr greater than Ba, being common to all three species.
Full text
PDF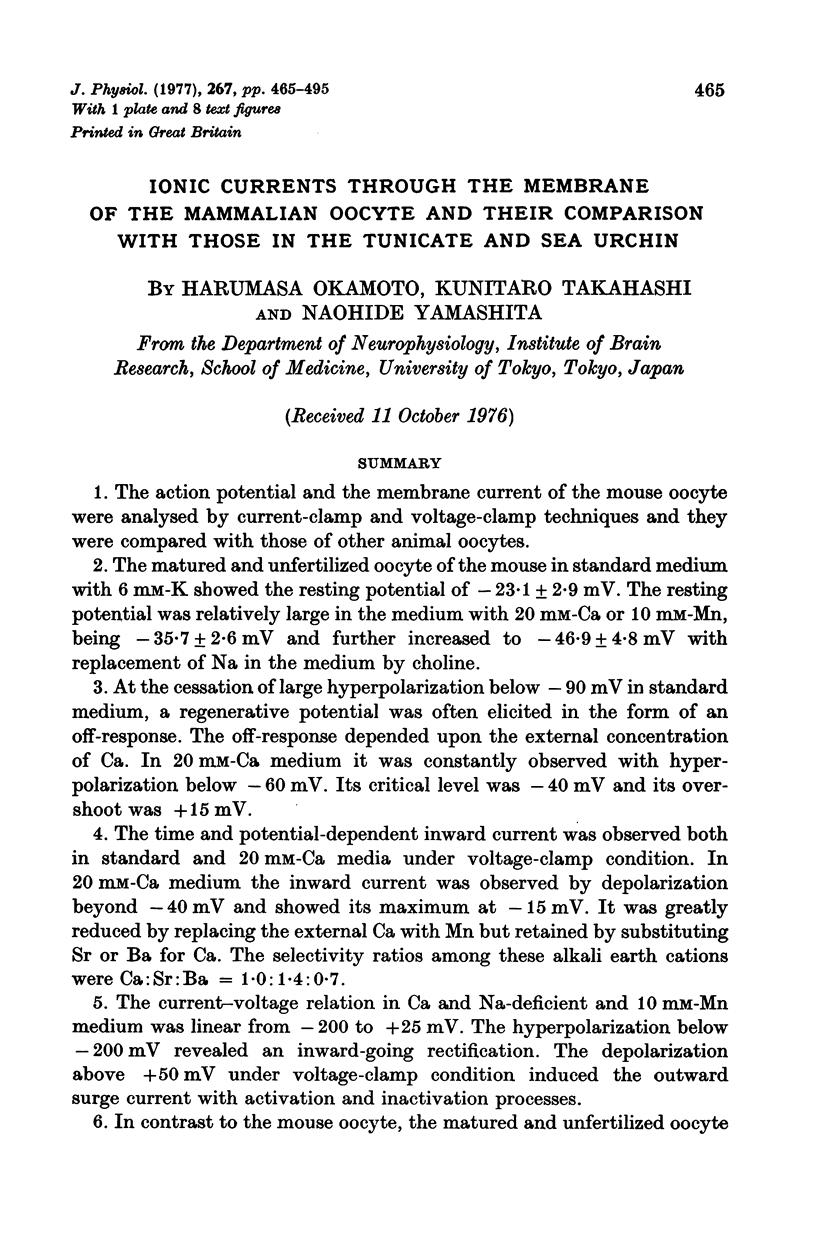
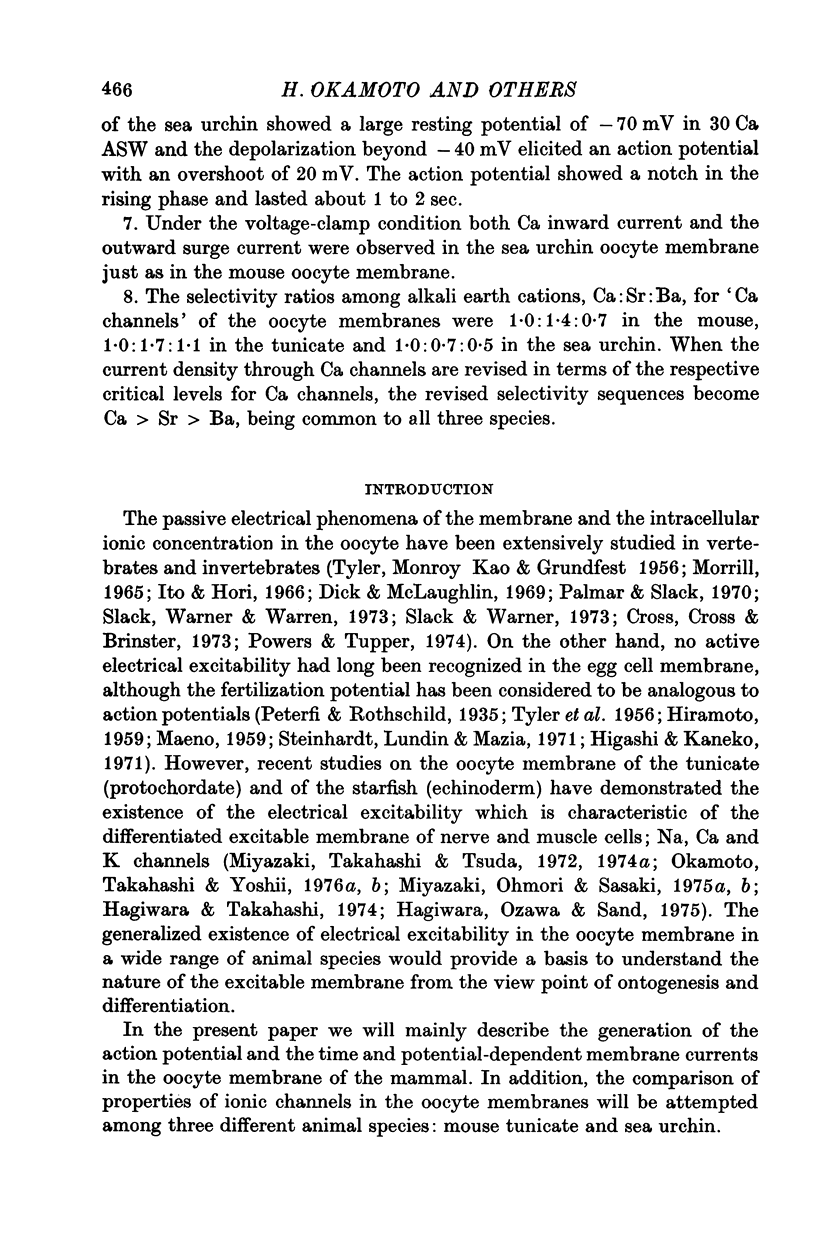
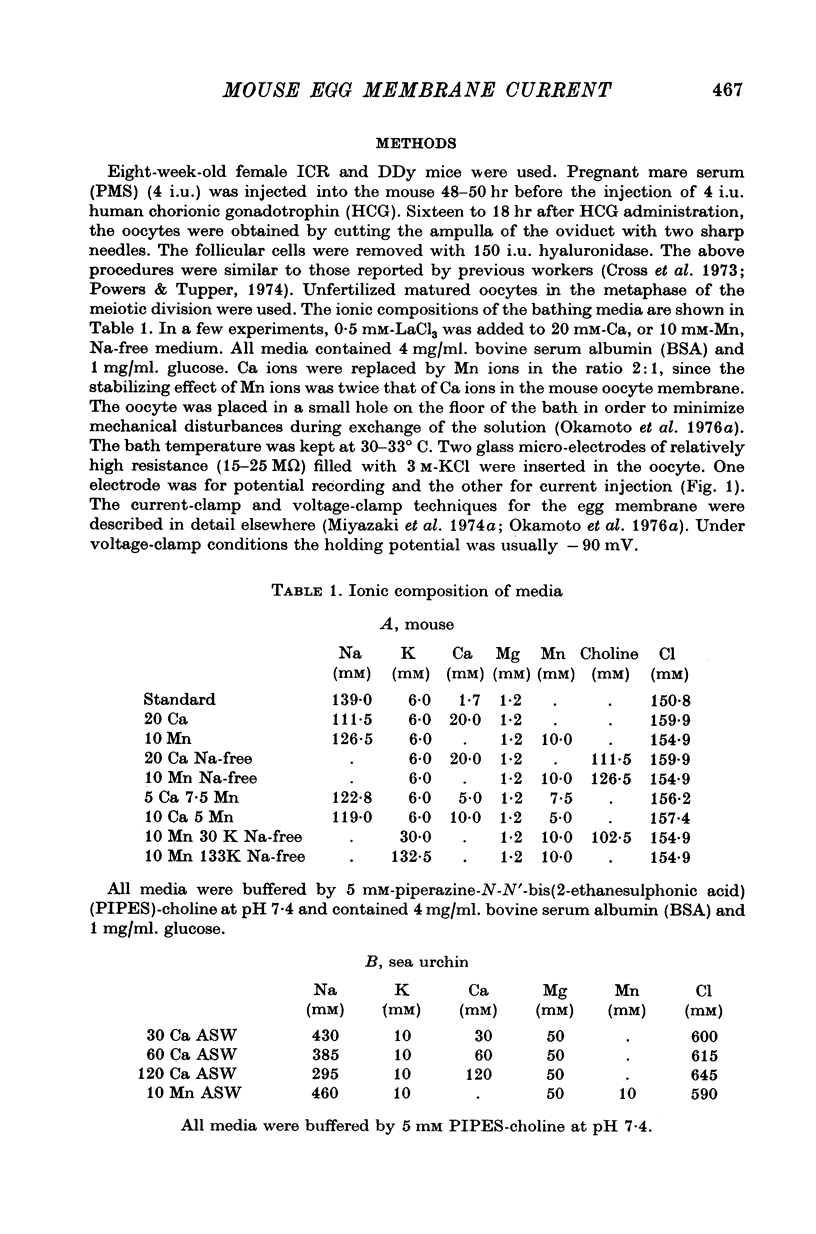
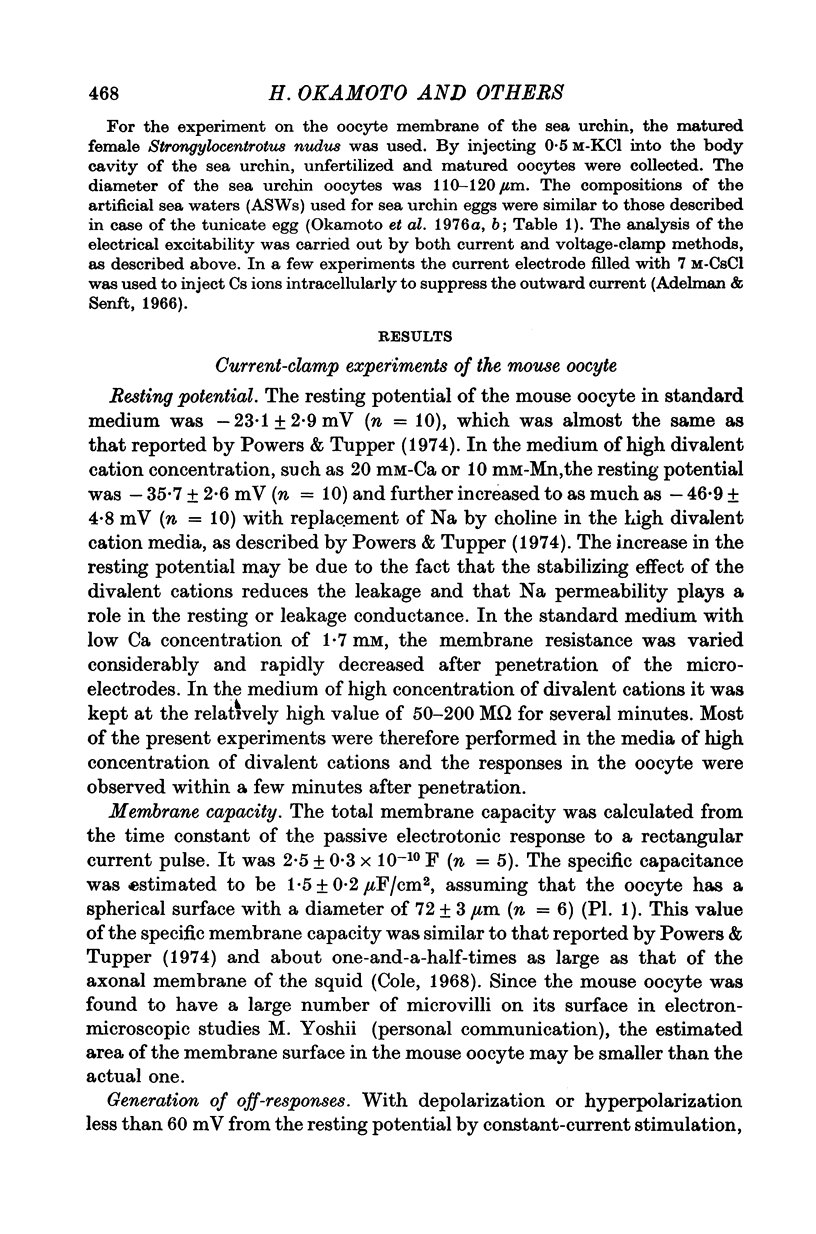
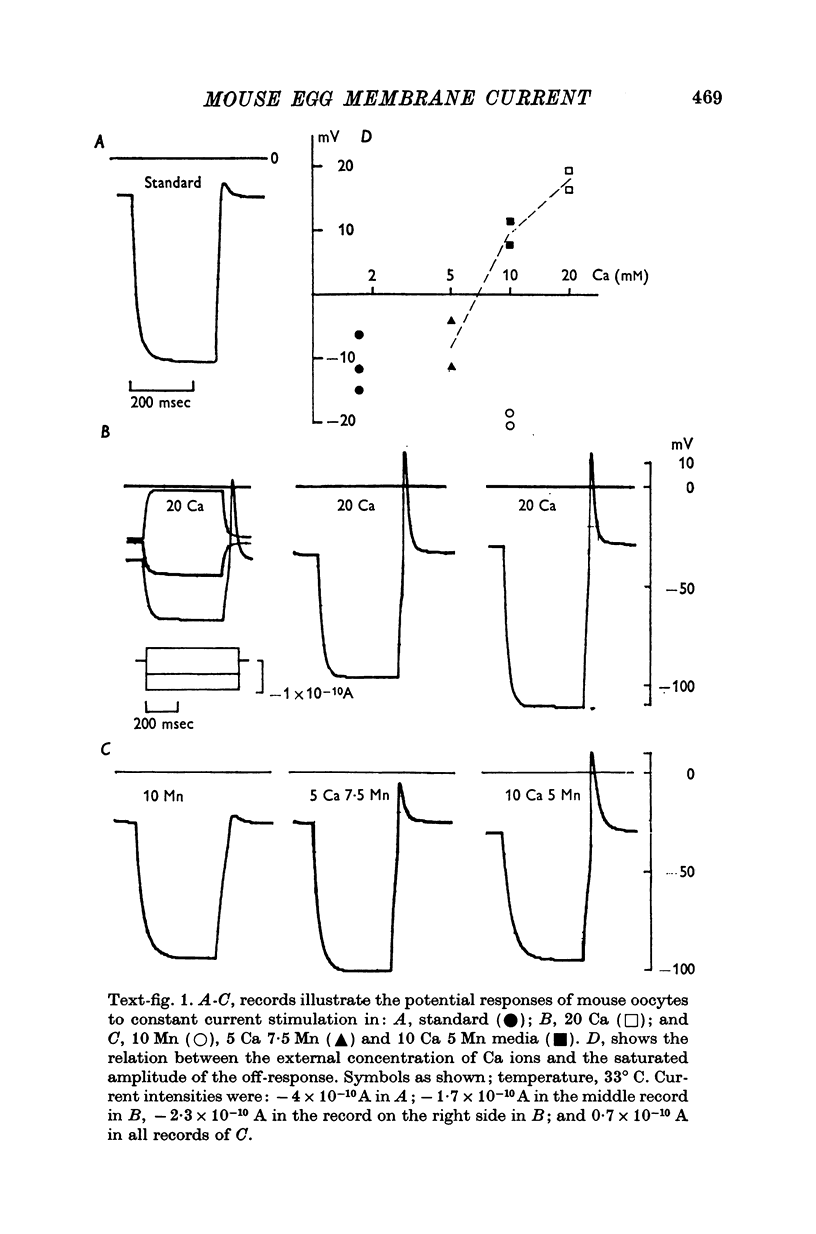
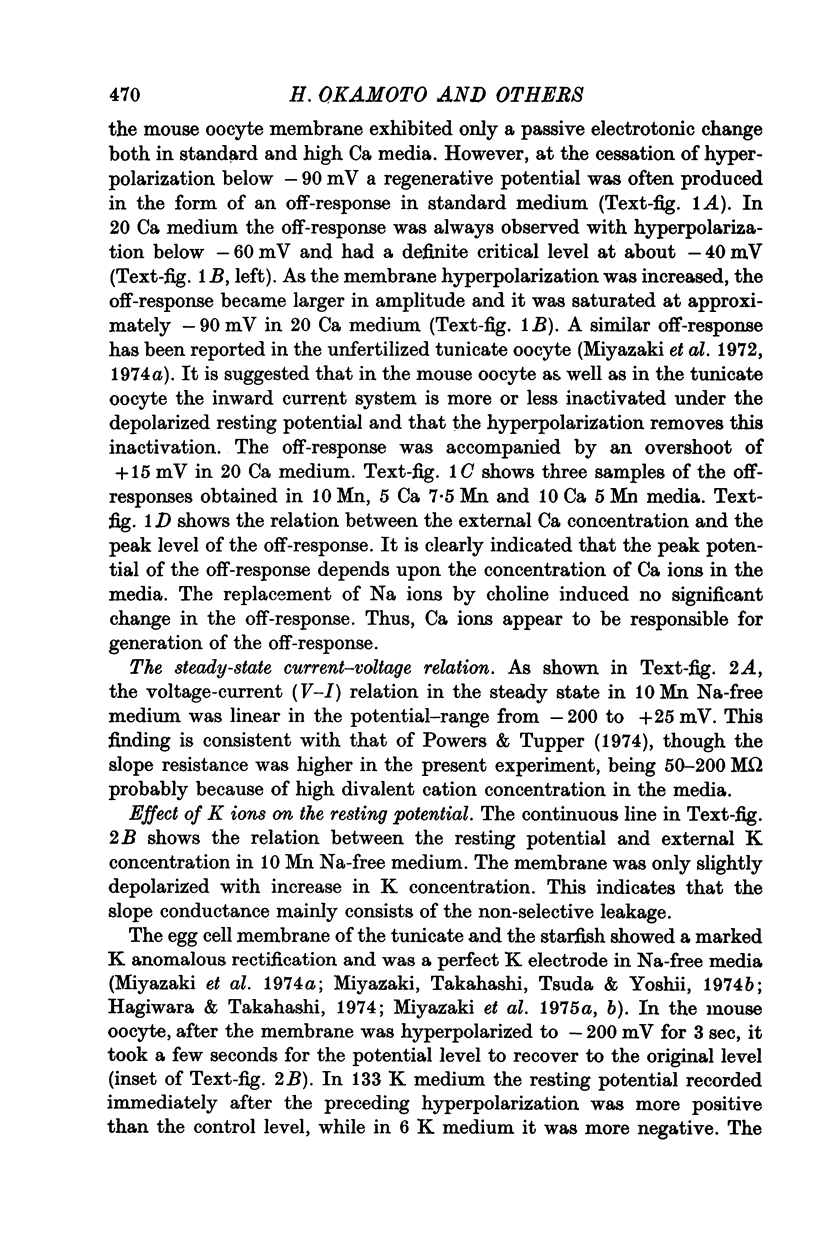
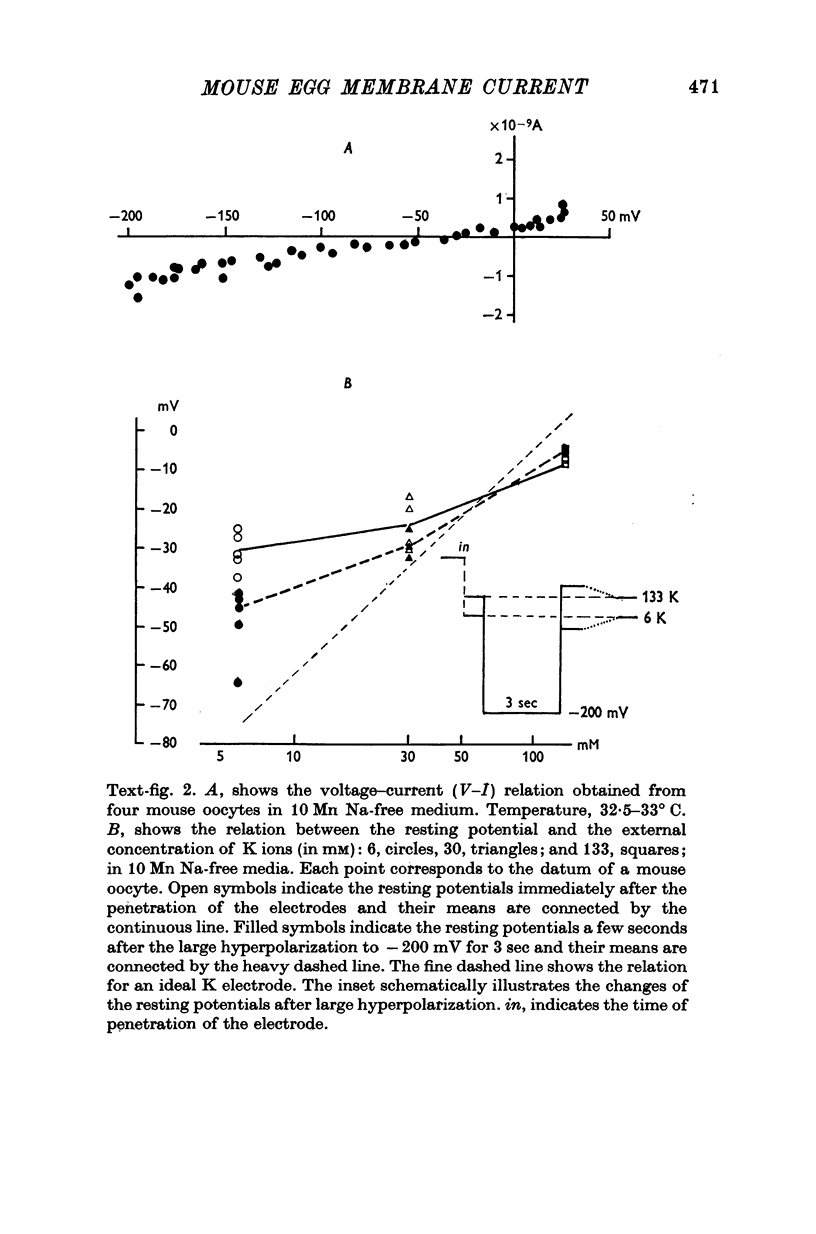
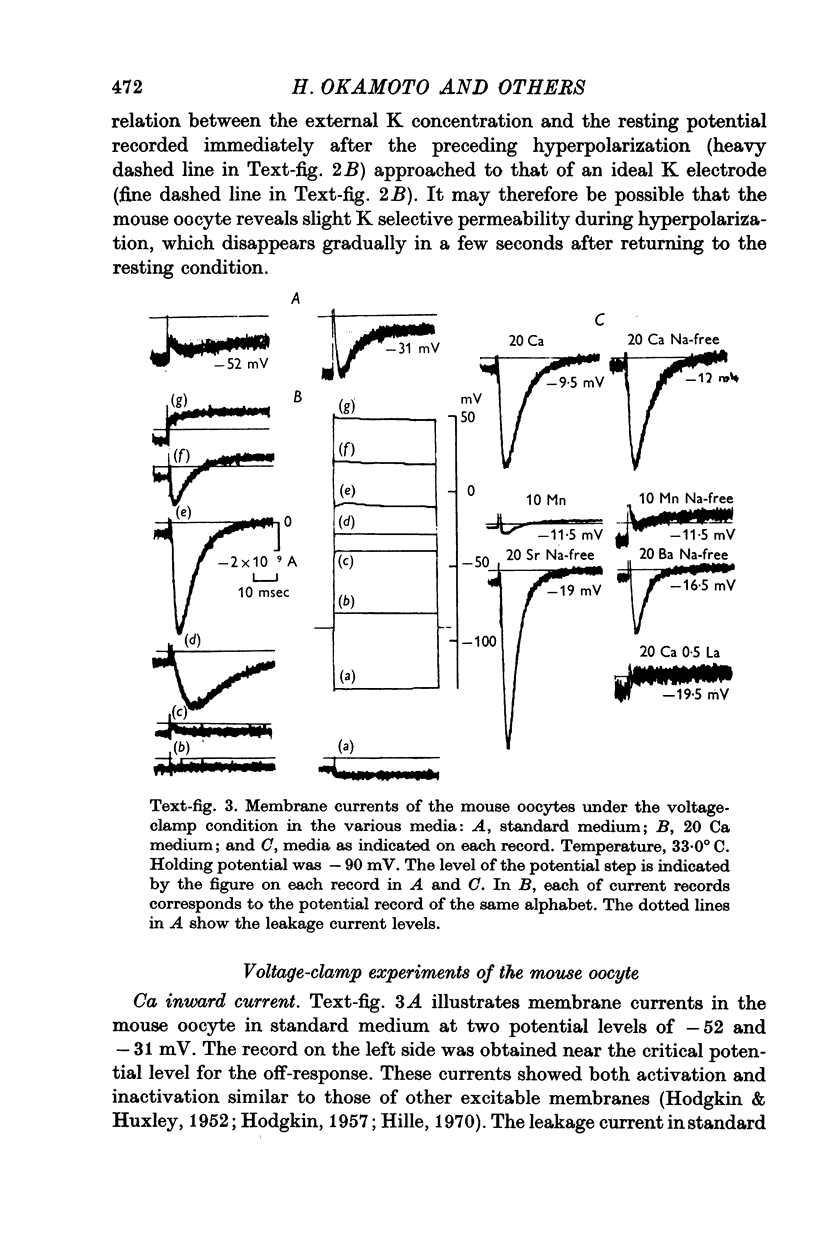
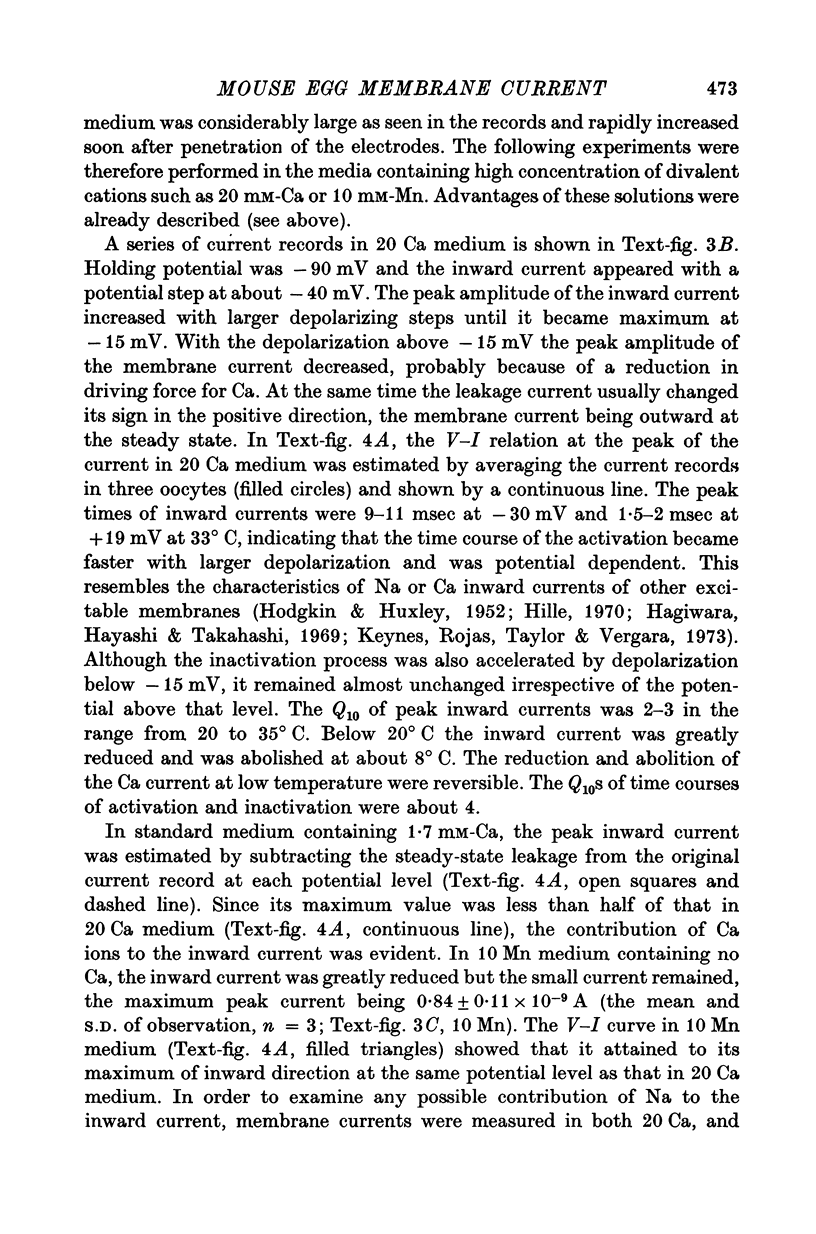
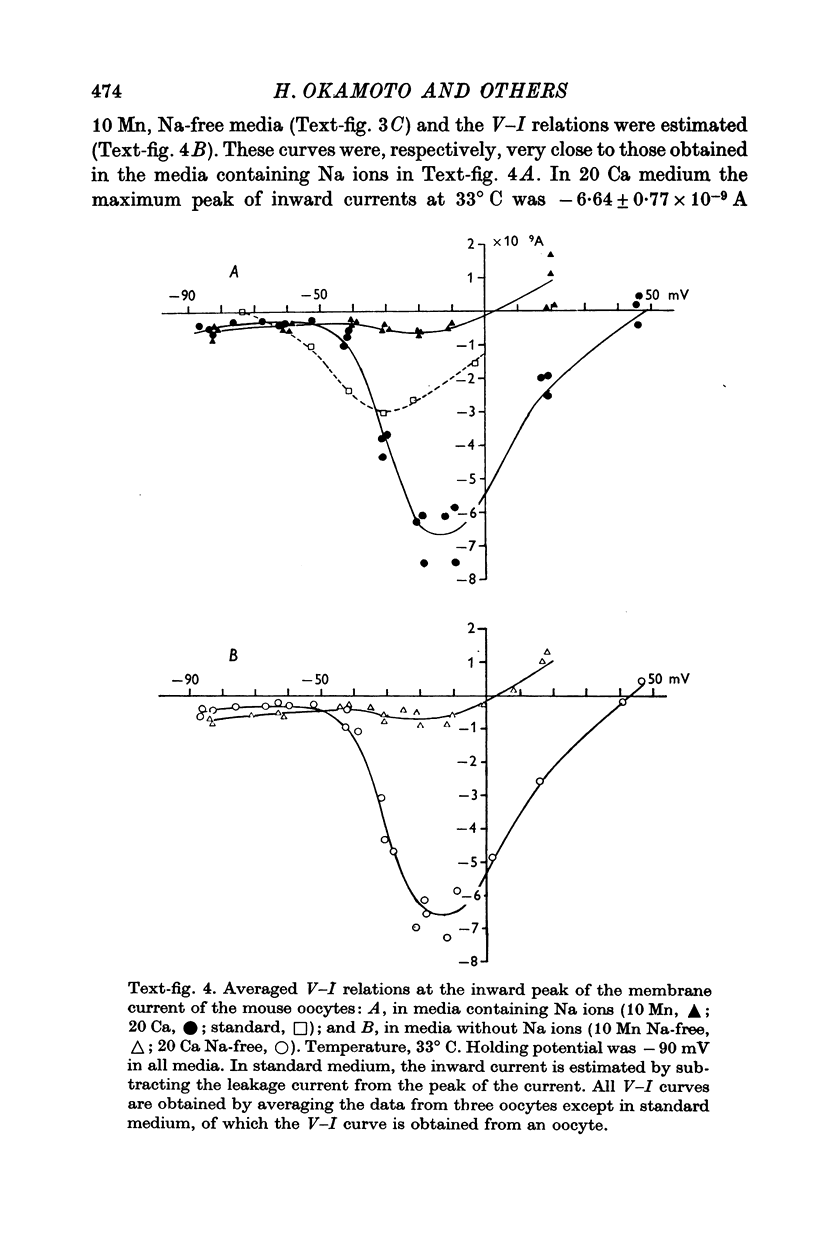
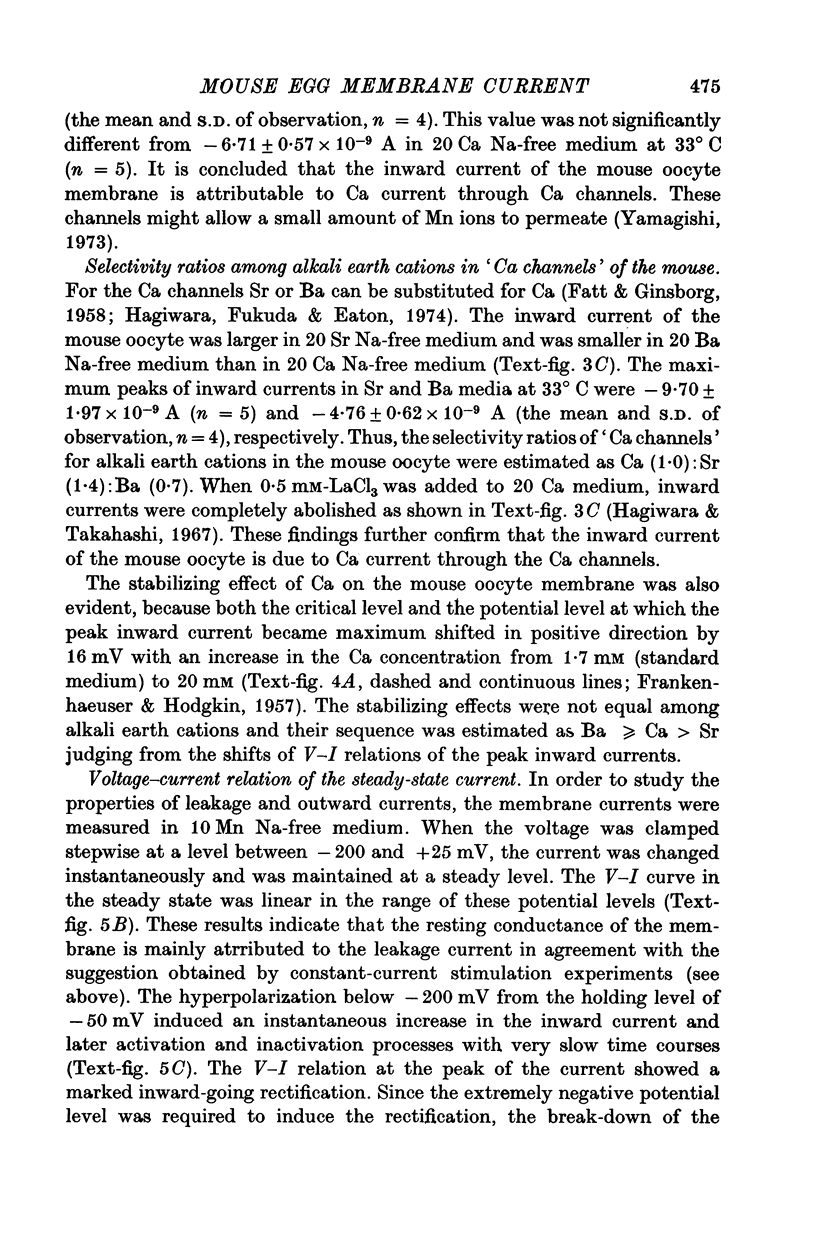
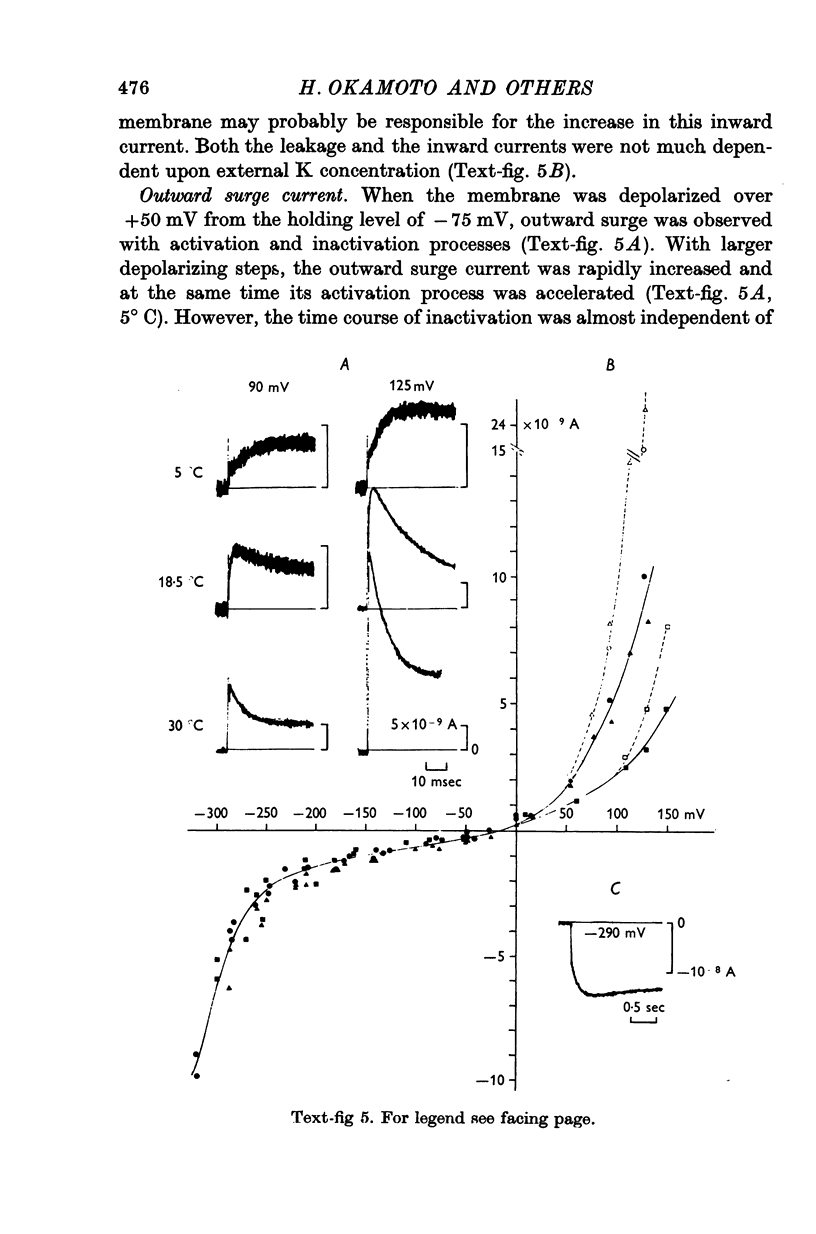
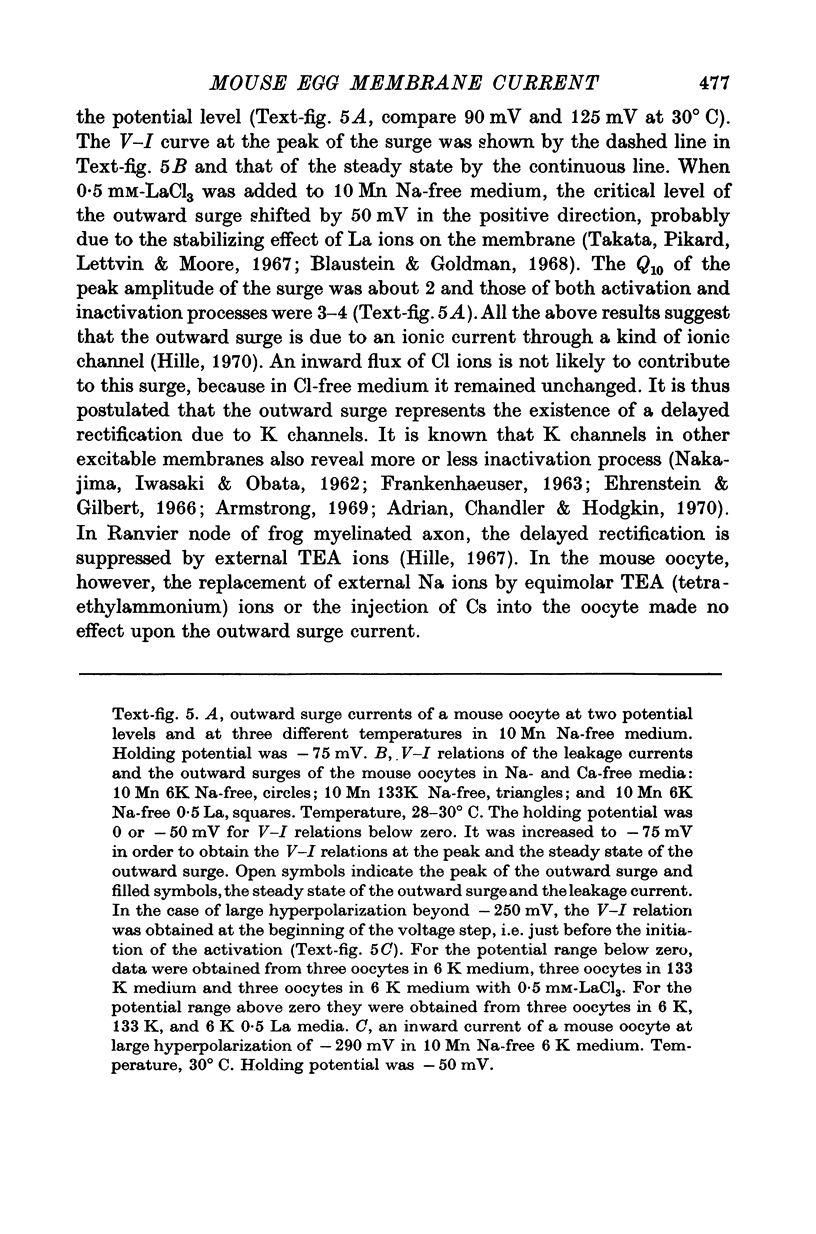
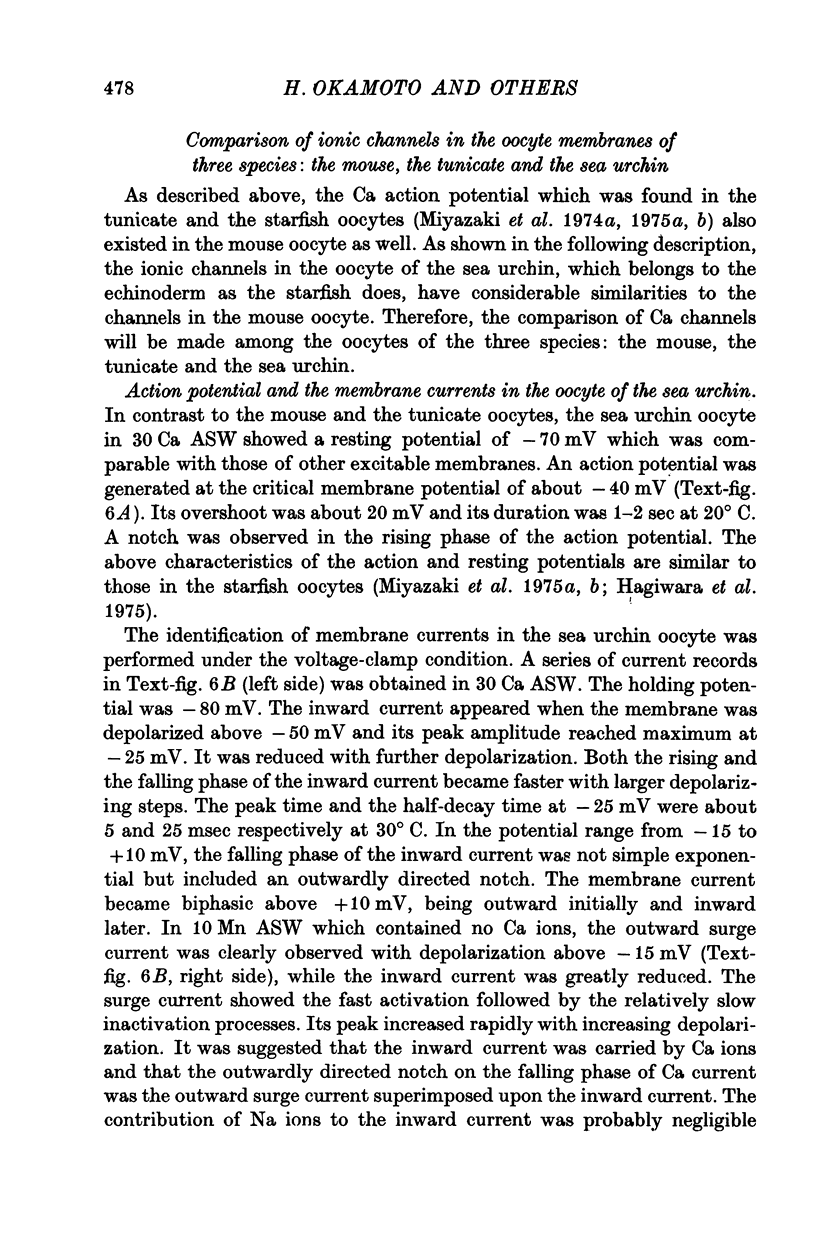
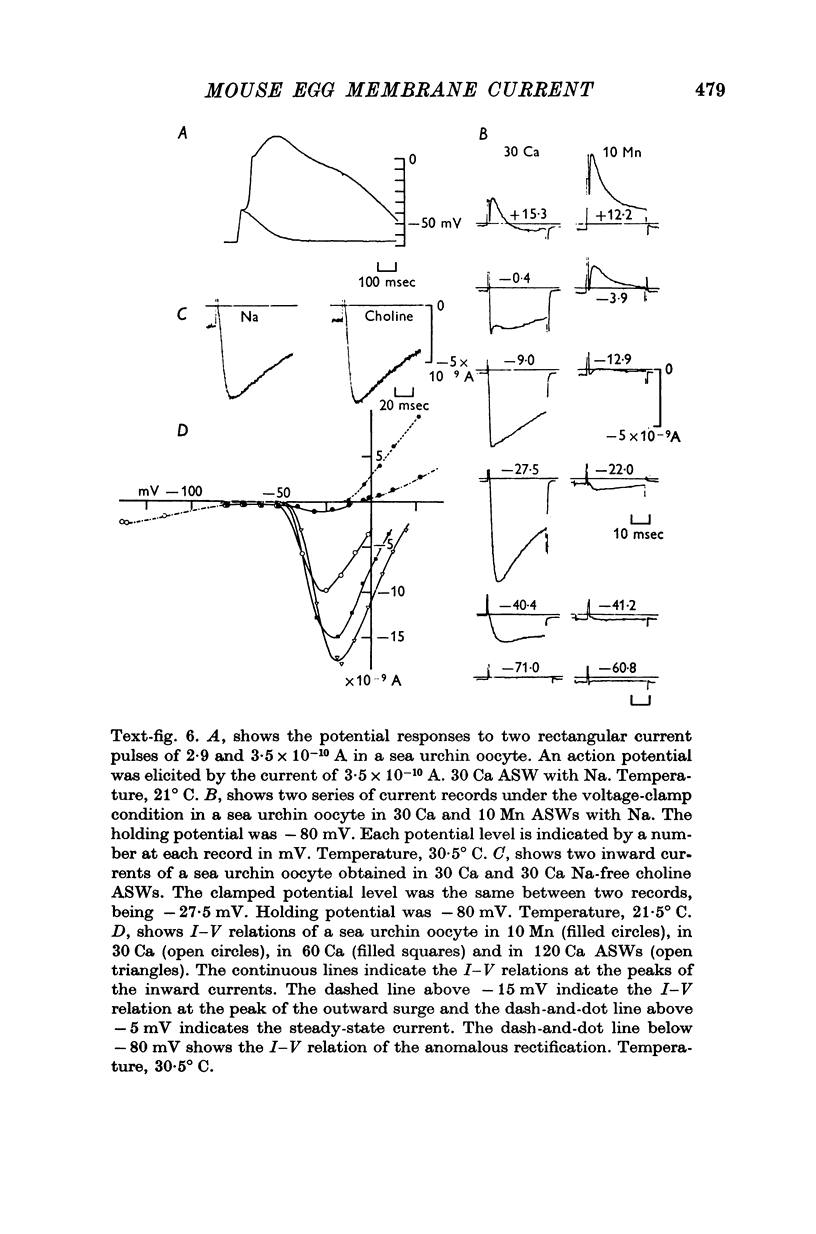
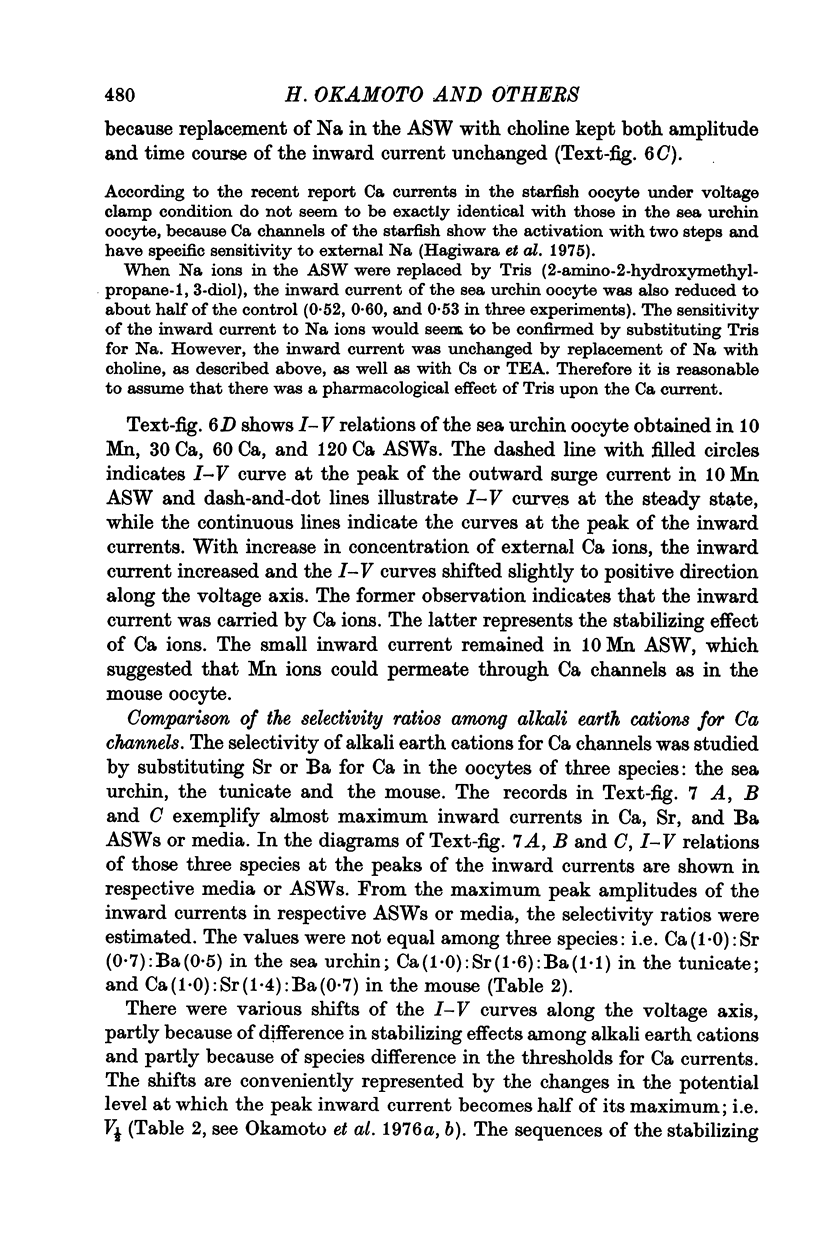
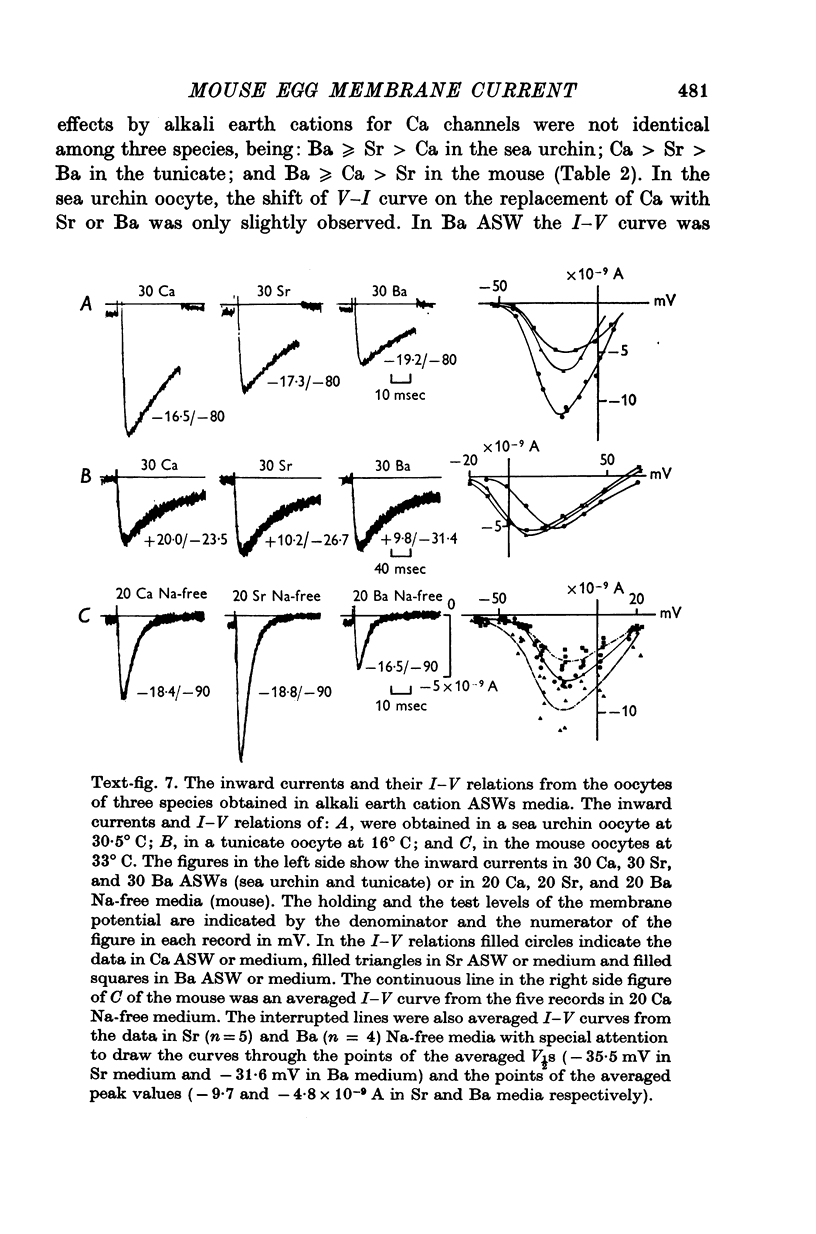
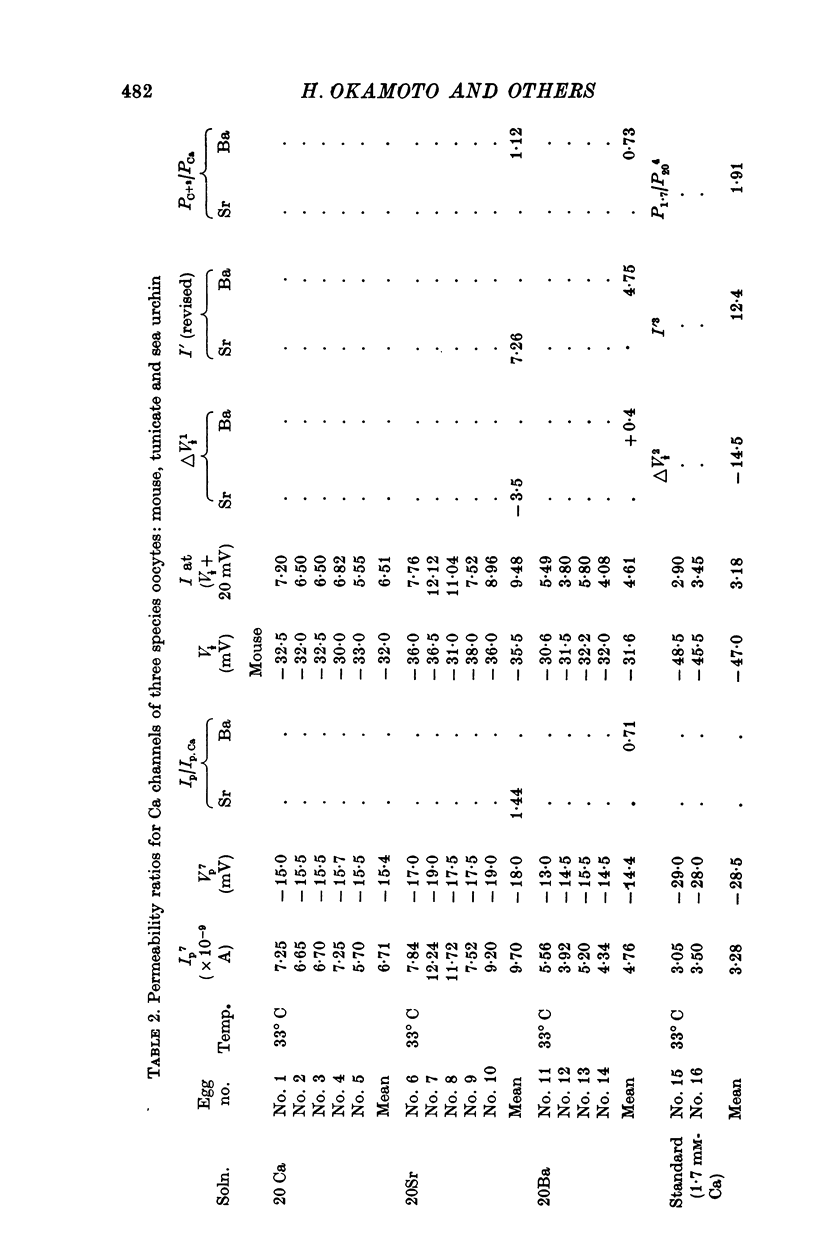
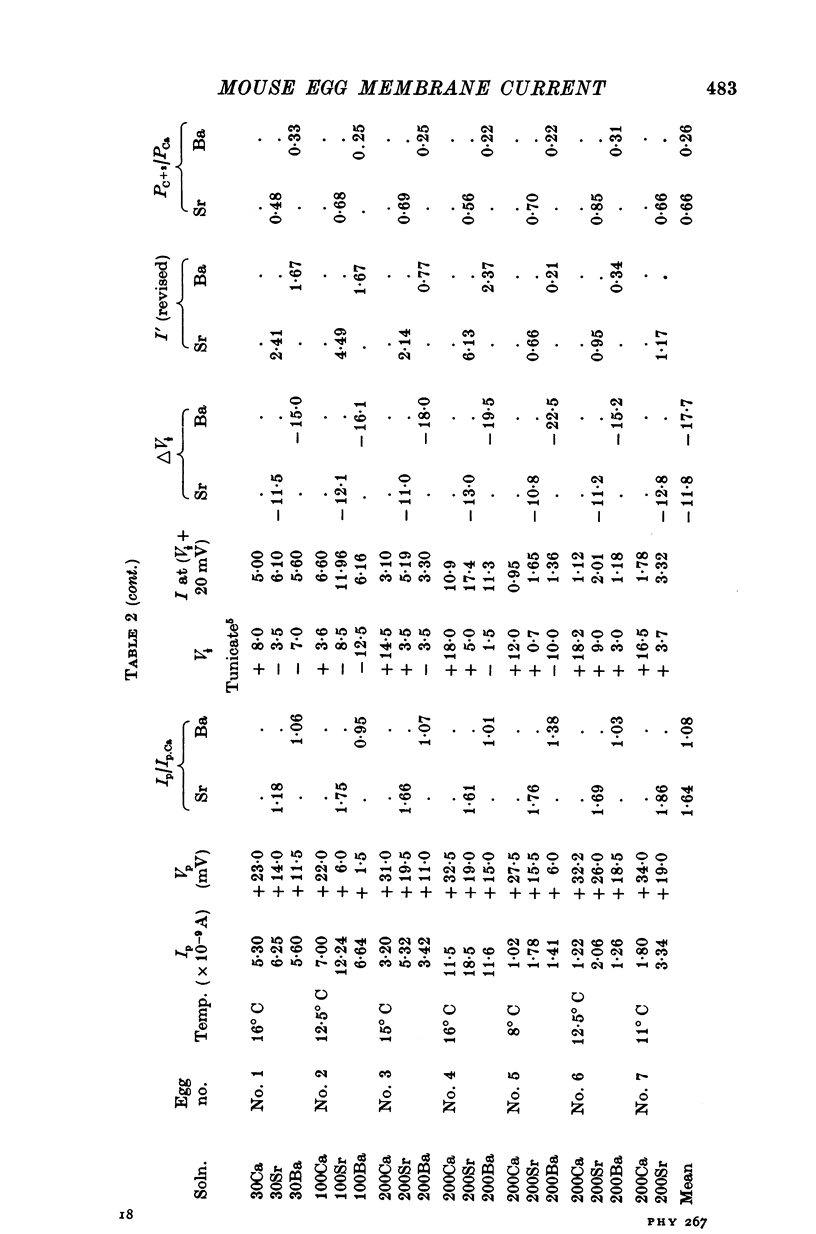
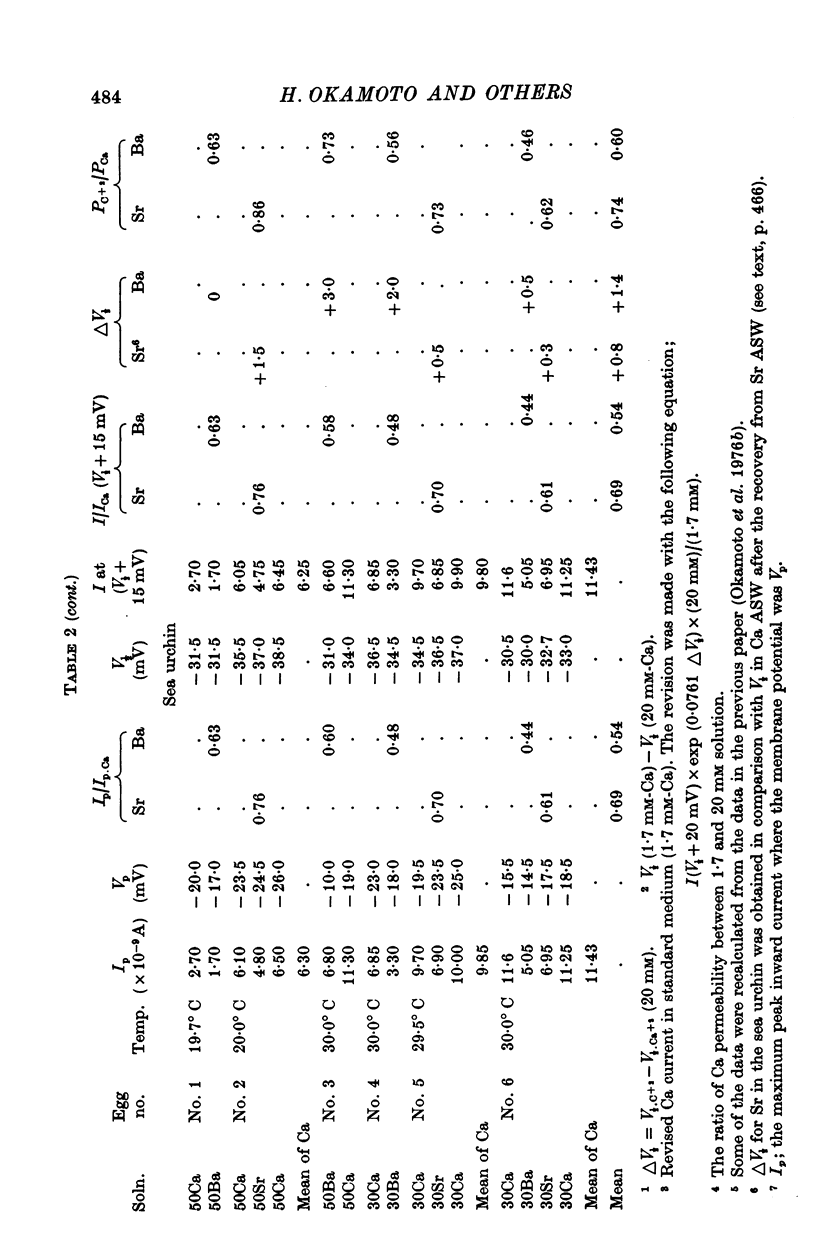
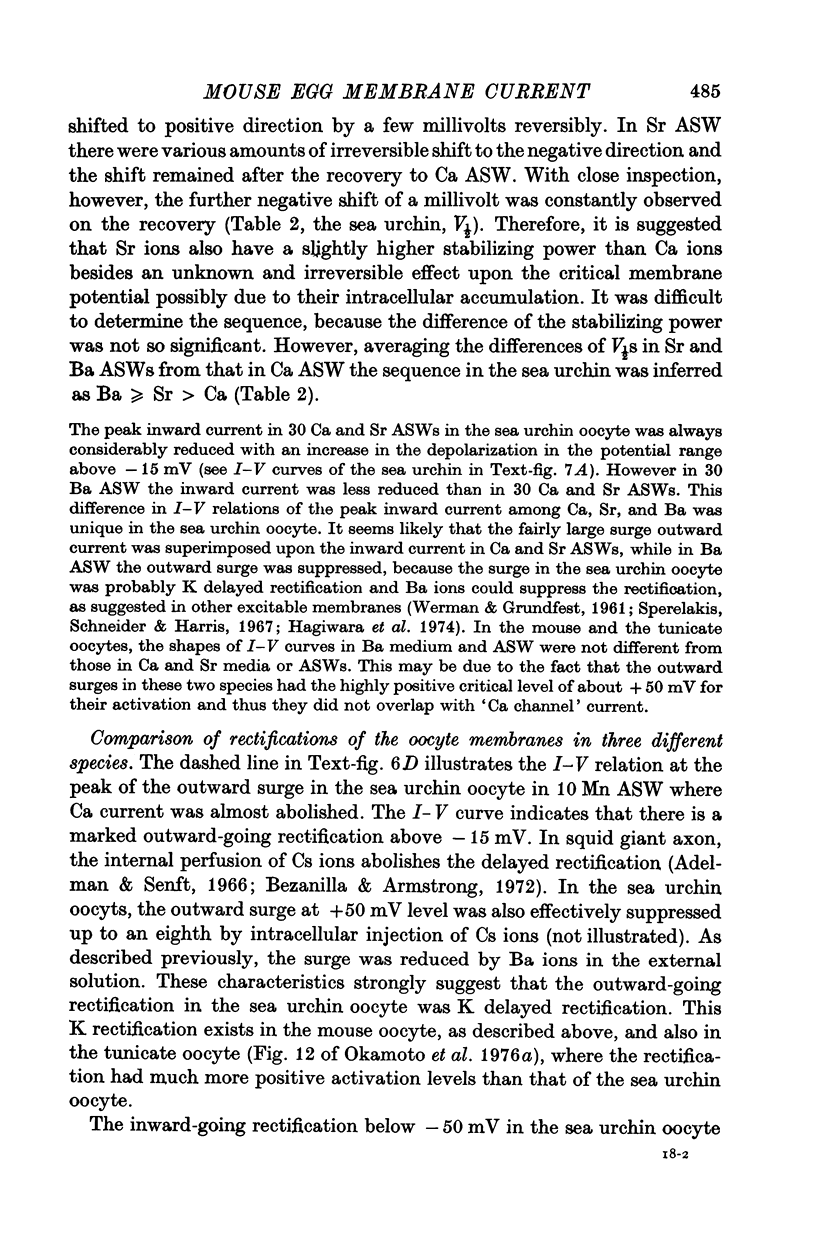
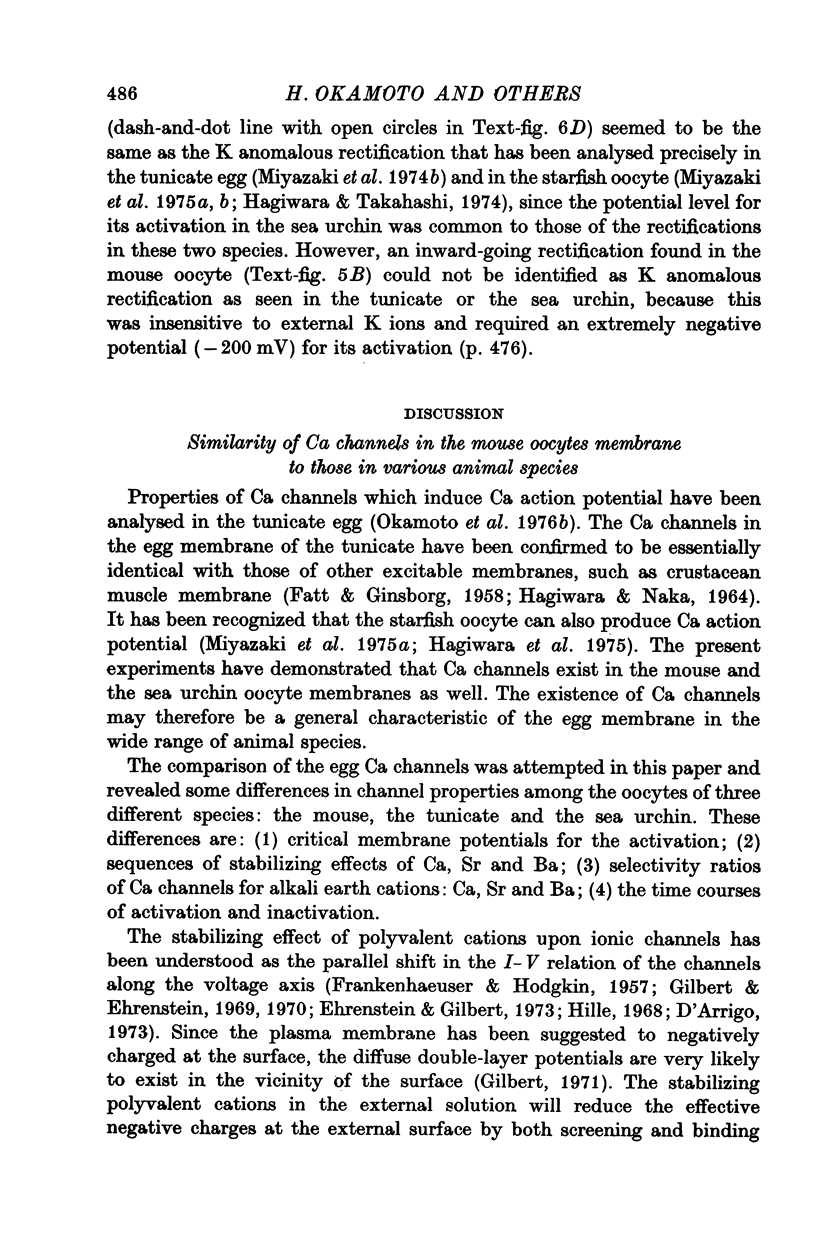
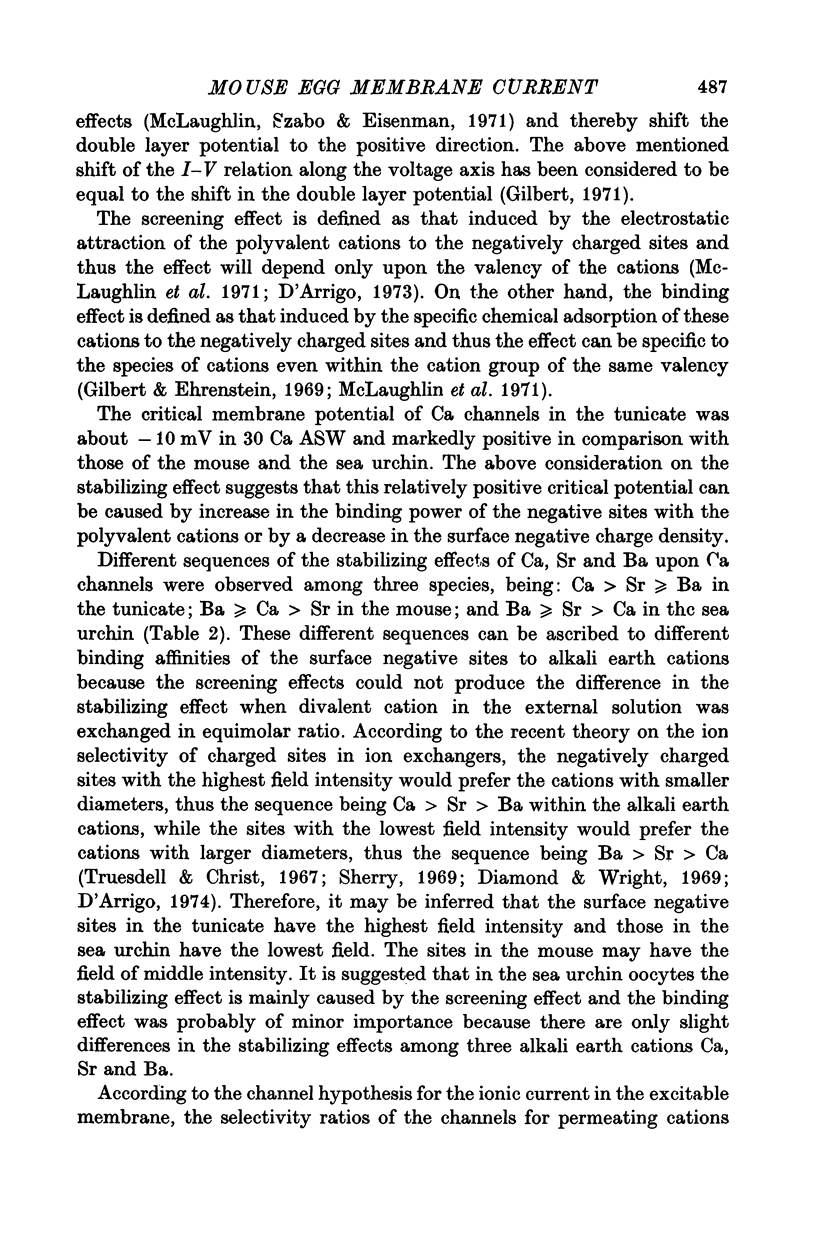
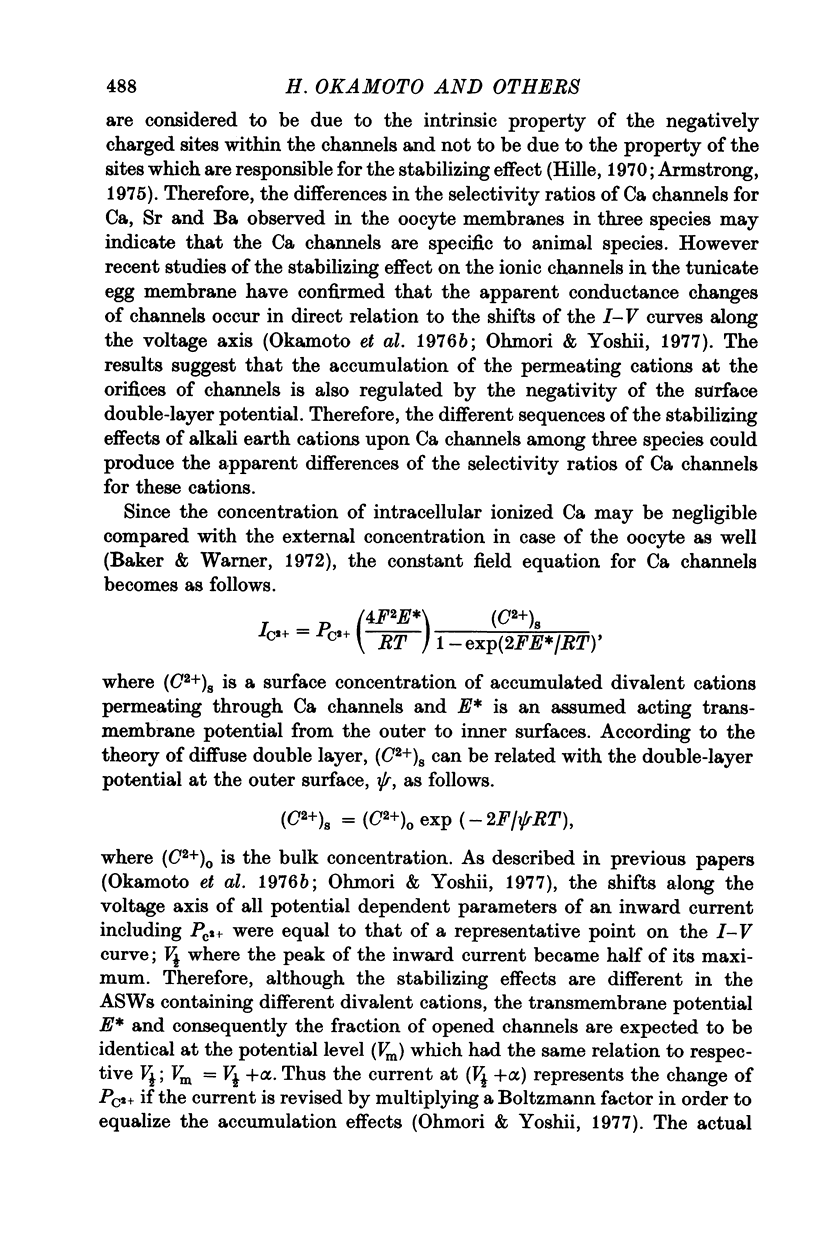
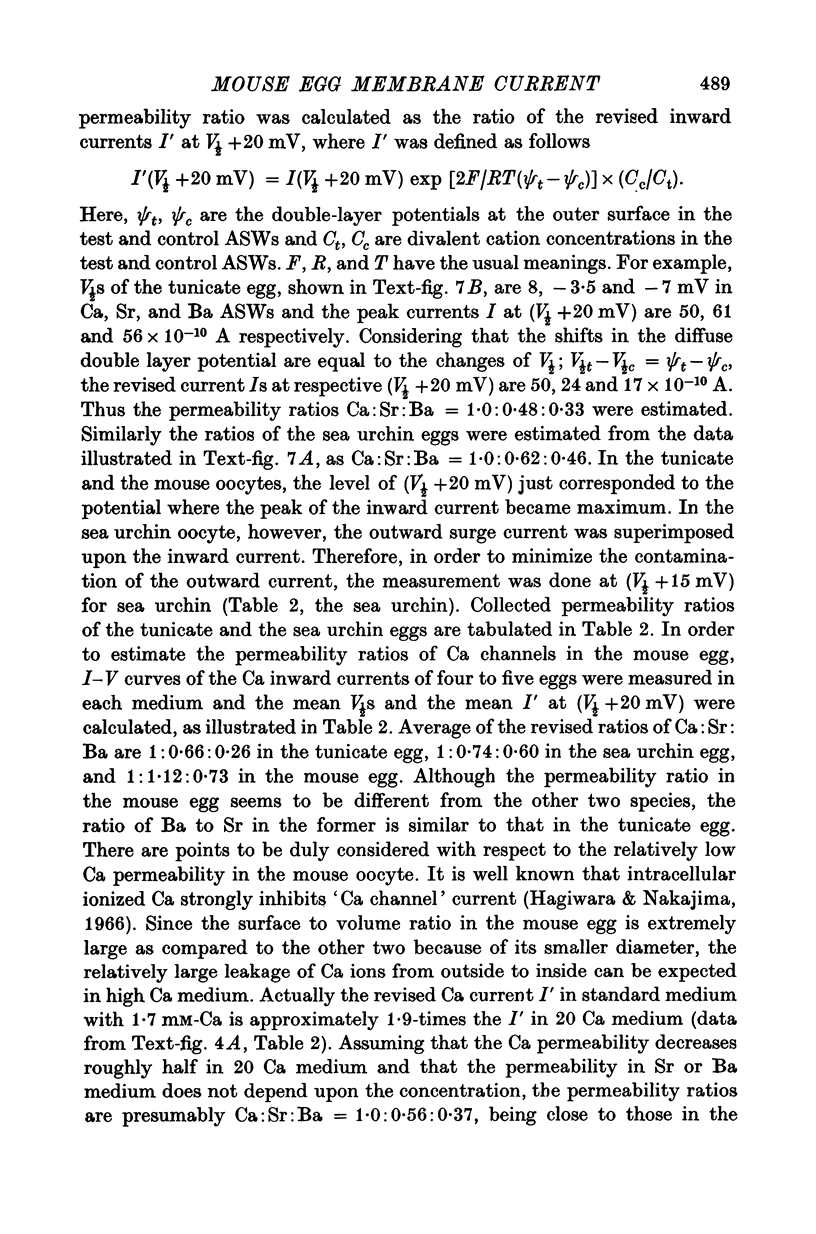
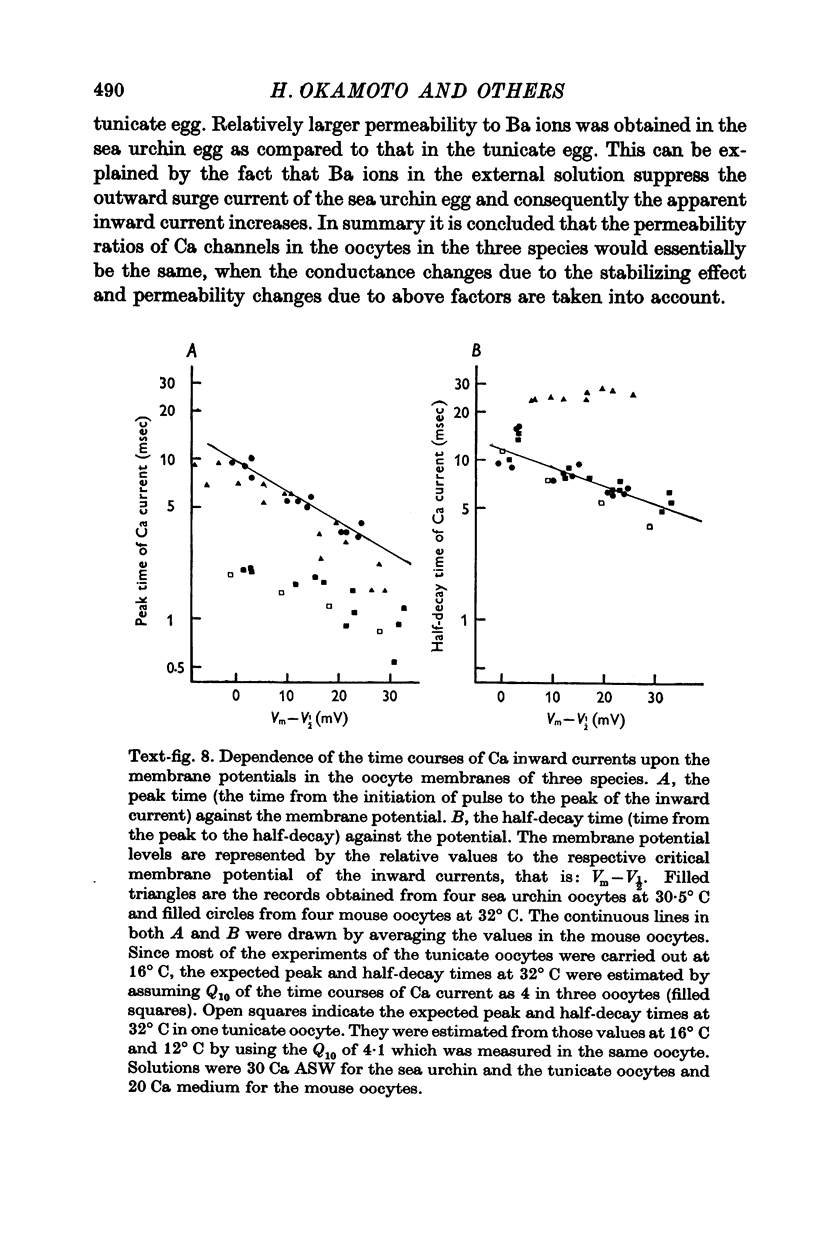
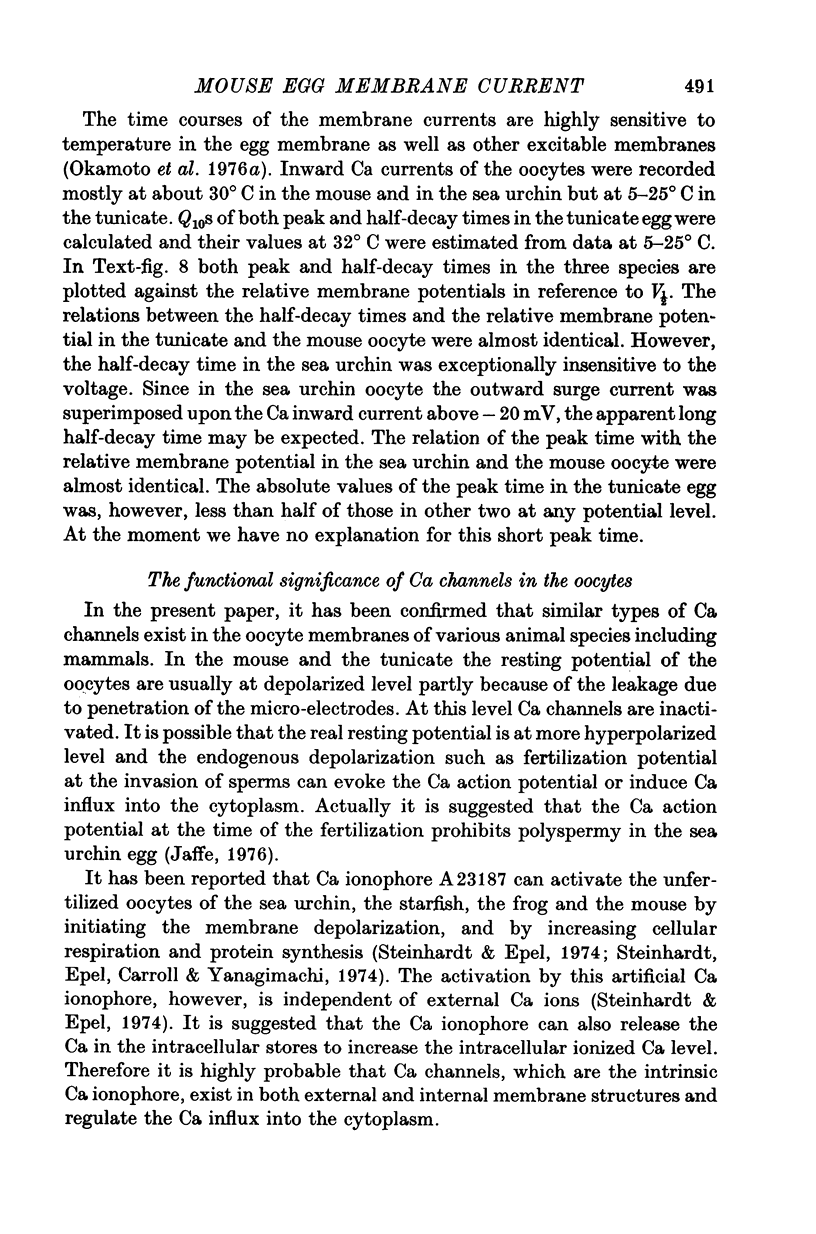
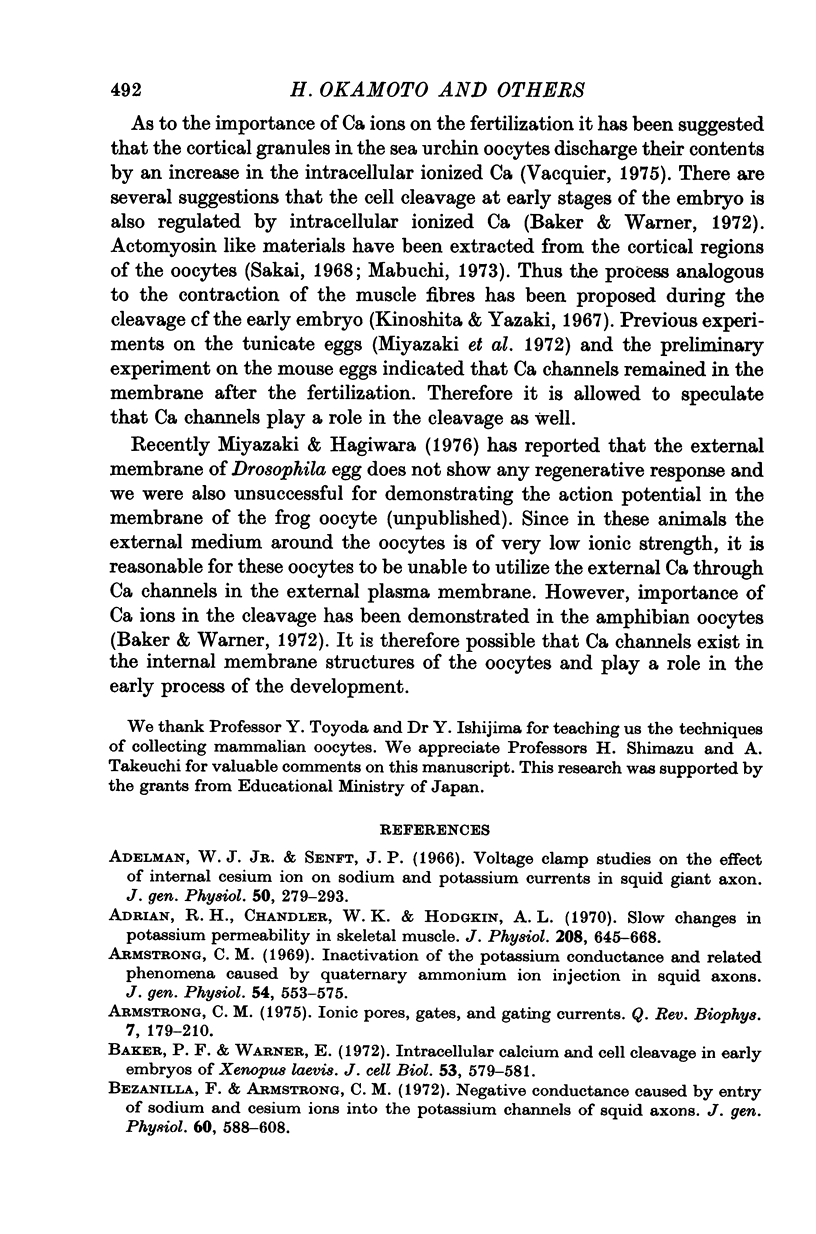
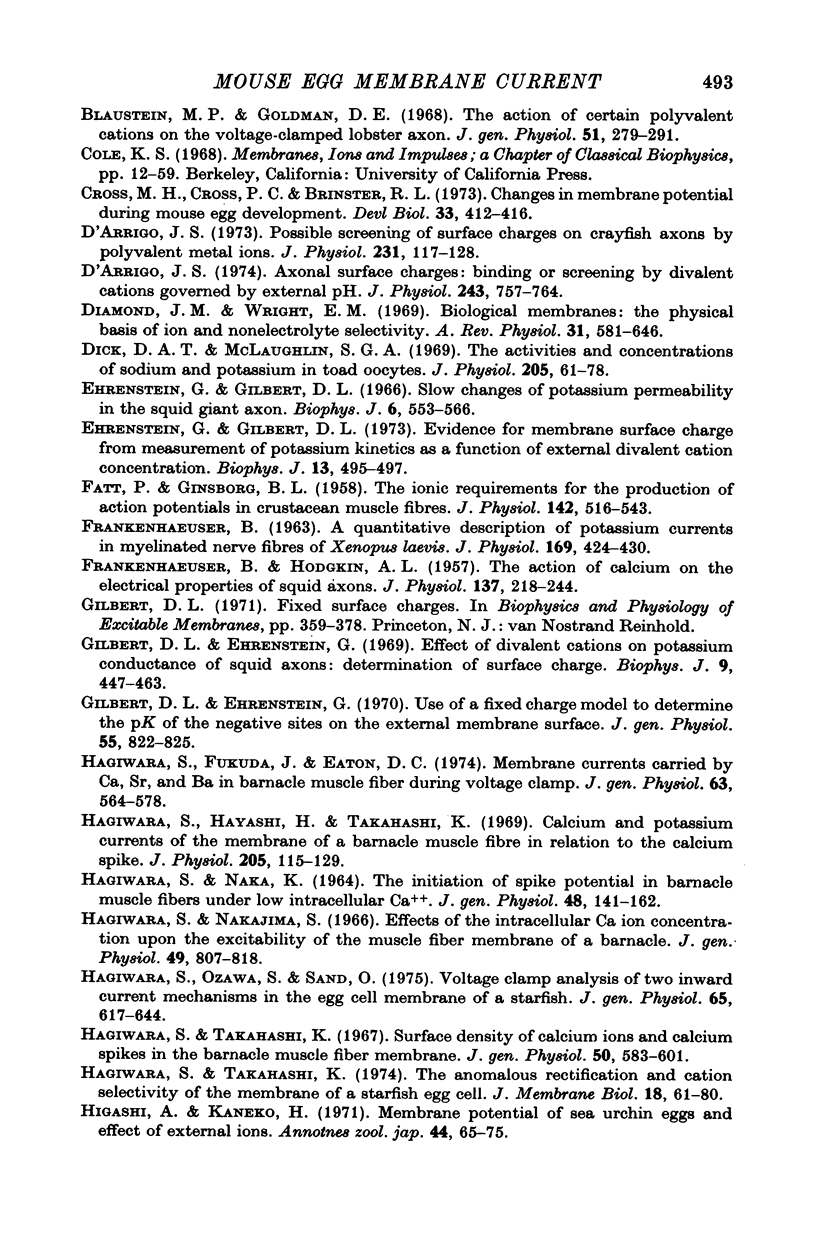
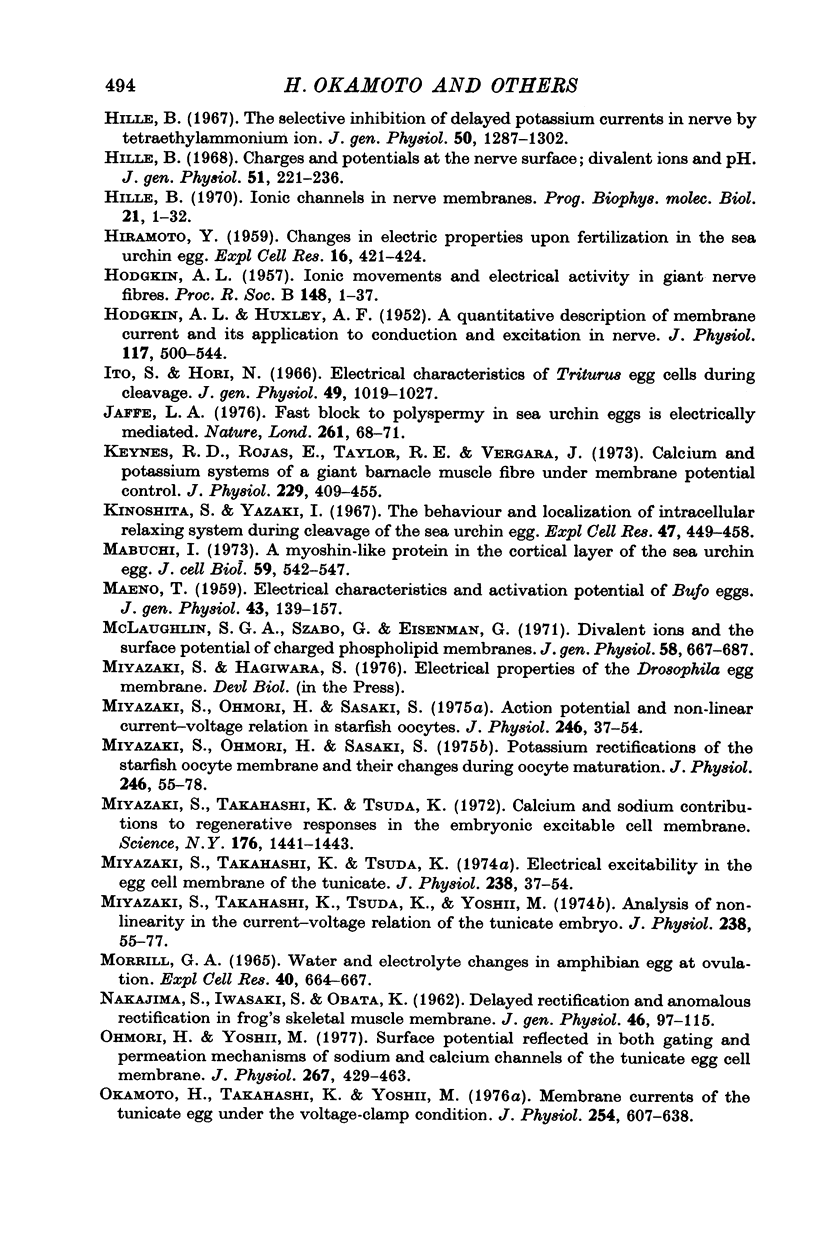
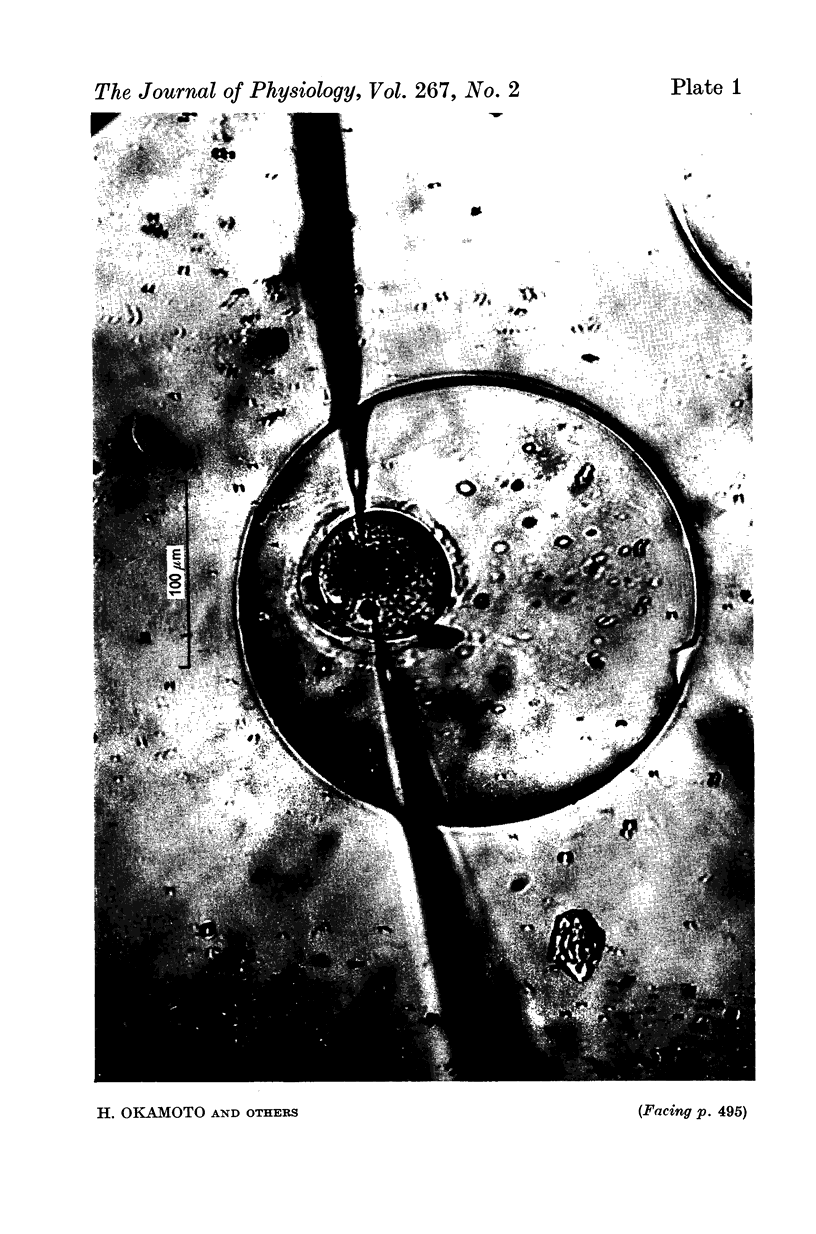
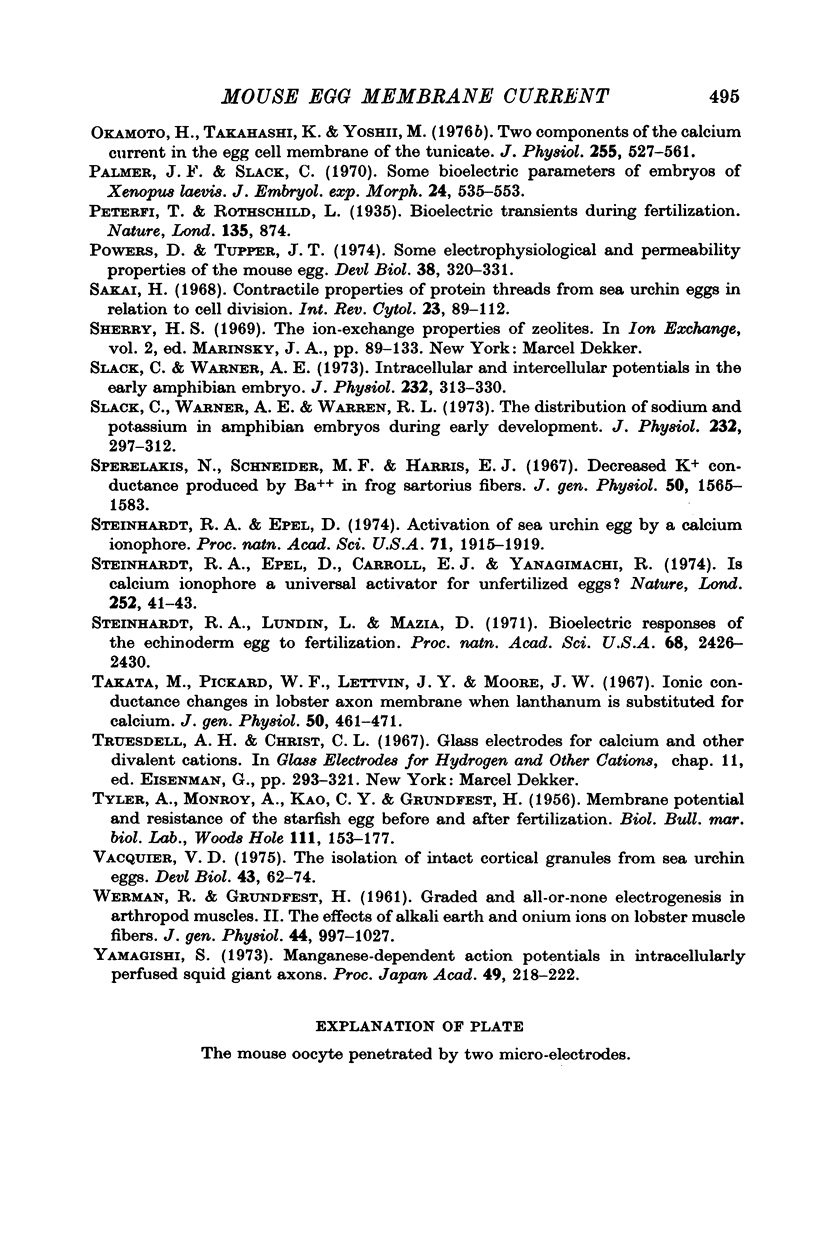
Images in this article
Selected References
These references are in PubMed. This may not be the complete list of references from this article.
- Adelman W. J., Jr, Senft J. P. Voltage clamp studies on the effect of internal cesium ion on sodium and potassium currents in the squid giant axon. J Gen Physiol. 1966 Nov;50(2):279–293. doi: 10.1085/jgp.50.2.279. [DOI] [PMC free article] [PubMed] [Google Scholar]
- Adrian R. H., Chandler W. K., Hodgkin A. L. Slow changes in potassium permeability in skeletal muscle. J Physiol. 1970 Jul;208(3):645–668. doi: 10.1113/jphysiol.1970.sp009140. [DOI] [PMC free article] [PubMed] [Google Scholar]
- Armstrong C. M. Inactivation of the potassium conductance and related phenomena caused by quaternary ammonium ion injection in squid axons. J Gen Physiol. 1969 Nov;54(5):553–575. doi: 10.1085/jgp.54.5.553. [DOI] [PMC free article] [PubMed] [Google Scholar]
- Armstrong C. M. Ionic pores, gates, and gating currents. Q Rev Biophys. 1974 May;7(2):179–210. doi: 10.1017/s0033583500001402. [DOI] [PubMed] [Google Scholar]
- Baker P. F., Warner A. E. Intracellular calcium and cell cleavage in early embryos of Xenopus laevis. J Cell Biol. 1972 May;53(2):579–581. doi: 10.1083/jcb.53.2.579. [DOI] [PMC free article] [PubMed] [Google Scholar]
- Bezanilla F., Armstrong C. M. Negative conductance caused by entry of sodium and cesium ions into the potassium channels of squid axons. J Gen Physiol. 1972 Nov;60(5):588–608. doi: 10.1085/jgp.60.5.588. [DOI] [PMC free article] [PubMed] [Google Scholar]
- Blaustein M. P., Goldman D. E. The action of certain polyvalent cations on the voltage-clamped lobster axon. J Gen Physiol. 1968 Mar;51(3):279–291. doi: 10.1085/jgp.51.3.279. [DOI] [PMC free article] [PubMed] [Google Scholar]
- Cross M. H., Cross P. C., Brinster R. L. Changes in membrane potential during mouse egg development. Dev Biol. 1973 Aug;33(2):412–416. doi: 10.1016/0012-1606(73)90146-2. [DOI] [PubMed] [Google Scholar]
- D'Arrigo J. S. Axonal surface charges: binding or screening by divalent cations governed by external pH. J Physiol. 1974 Dec;243(3):757–764. doi: 10.1113/jphysiol.1974.sp010775. [DOI] [PMC free article] [PubMed] [Google Scholar]
- D'Arrigo J. S. Possible screening of surface charges on crayfish axons by polyvalent metal ions. J Physiol. 1973 May;231(1):117–128. doi: 10.1113/jphysiol.1973.sp010223. [DOI] [PMC free article] [PubMed] [Google Scholar]
- Diamond J. M., Wright E. M. Biological membranes: the physical basis of ion and nonelectrolyte selectivity. Annu Rev Physiol. 1969;31:581–646. doi: 10.1146/annurev.ph.31.030169.003053. [DOI] [PubMed] [Google Scholar]
- Dick D. A., McLaughlin S. G. The activities and concentrations of sodium and potassium in toad oocytes. J Physiol. 1969 Nov;205(1):61–78. doi: 10.1113/jphysiol.1969.sp008951. [DOI] [PMC free article] [PubMed] [Google Scholar]
- Ehrenstein G., Gilbert D. L. Evidence for membrane surface from measurement of potassium kinetics as a function of external divalent cation concentration. Biophys J. 1973 May;13(5):495–497. doi: 10.1016/S0006-3495(73)86002-3. [DOI] [PMC free article] [PubMed] [Google Scholar]
- Ehrenstein G., Gilbert D. L. Slow changes of potassium permeability in the squid giant axon. Biophys J. 1966 Sep;6(5):553–566. doi: 10.1016/S0006-3495(66)86677-8. [DOI] [PMC free article] [PubMed] [Google Scholar]
- FATT P., GINSBORG B. L. The ionic requirements for the production of action potentials in crustacean muscle fibres. J Physiol. 1958 Aug 6;142(3):516–543. doi: 10.1113/jphysiol.1958.sp006034. [DOI] [PMC free article] [PubMed] [Google Scholar]
- FRANKENHAEUSER B. A QUANTITATIVE DESCRIPTION OF POTASSIUM CURRENTS IN MYELINATED NERVE FIBRES OF XENOPUS LAEVIS. J Physiol. 1963 Nov;169:424–430. doi: 10.1113/jphysiol.1963.sp007268. [DOI] [PMC free article] [PubMed] [Google Scholar]
- FRANKENHAEUSER B., HODGKIN A. L. The action of calcium on the electrical properties of squid axons. J Physiol. 1957 Jul 11;137(2):218–244. doi: 10.1113/jphysiol.1957.sp005808. [DOI] [PMC free article] [PubMed] [Google Scholar]
- Gilbert D. L., Ehrenstein G. Effect of divalent cations on potassium conductance of squid axons: determination of surface charge. Biophys J. 1969 Mar;9(3):447–463. doi: 10.1016/S0006-3495(69)86396-4. [DOI] [PMC free article] [PubMed] [Google Scholar]
- Gilbert D. L., Ehrenstein G. Use of a fixed charge model to determine the pK of the negative sites on the external membrane surface. J Gen Physiol. 1970 Jun;55(6):822–825. [PMC free article] [PubMed] [Google Scholar]
- HAGIWARA S., NAKA K. I. THE INITIATION OF SPIKE POTENTIAL IN BARNACLE MUSCLE FIBERS UNDER LOW INTRACELLULAR CA++. J Gen Physiol. 1964 Sep;48:141–162. doi: 10.1085/jgp.48.1.141. [DOI] [PMC free article] [PubMed] [Google Scholar]
- HIRAMOTO Y. Changes in electric properties upon fertilization in the sea urchin egg. Exp Cell Res. 1959 Feb;16(2):421–424. doi: 10.1016/0014-4827(59)90272-1. [DOI] [PubMed] [Google Scholar]
- HODGKIN A. L., HUXLEY A. F. A quantitative description of membrane current and its application to conduction and excitation in nerve. J Physiol. 1952 Aug;117(4):500–544. doi: 10.1113/jphysiol.1952.sp004764. [DOI] [PMC free article] [PubMed] [Google Scholar]
- HODGKIN A. L. Ionic movements and electrical activity in giant nerve fibres. Proc R Soc Lond B Biol Sci. 1958 Jan 1;148(930):1–37. doi: 10.1098/rspb.1958.0001. [DOI] [PubMed] [Google Scholar]
- Hagiwara S., Fukuda J., Eaton D. C. Membrane currents carried by Ca, Sr, and Ba in barnacle muscle fiber during voltage clamp. J Gen Physiol. 1974 May;63(5):564–578. doi: 10.1085/jgp.63.5.564. [DOI] [PMC free article] [PubMed] [Google Scholar]
- Hagiwara S., Hayashi H., Takahashi K. Calcium and potassium currents of the membrane of a barnacle muscle fibre in relation to the calcium spike. J Physiol. 1969 Nov;205(1):115–129. doi: 10.1113/jphysiol.1969.sp008955. [DOI] [PMC free article] [PubMed] [Google Scholar]
- Hagiwara S., Nakajima S. Effects of the intracellular Ca ion concentration upon the excitability of the muscle fiber membrane of a barnacle. J Gen Physiol. 1966 Mar;49(4):807–818. doi: 10.1085/jgp.49.4.807. [DOI] [PMC free article] [PubMed] [Google Scholar]
- Hagiwara S., Ozawa S., Sand O. Voltage clamp analysis of two inward current mechanisms in the egg cell membrane of a starfish. J Gen Physiol. 1975 May;65(5):617–644. doi: 10.1085/jgp.65.5.617. [DOI] [PMC free article] [PubMed] [Google Scholar]
- Hagiwara S., Takahashi K. Surface density of calcium ions and calcium spikes in the barnacle muscle fiber membrane. J Gen Physiol. 1967 Jan;50(3):583–601. doi: 10.1085/jgp.50.3.583. [DOI] [PMC free article] [PubMed] [Google Scholar]
- Hagiwara S., Takahashi K. The anomalous rectification and cation selectivity of the membrane of a starfish egg cell. J Membr Biol. 1974;18(1):61–80. doi: 10.1007/BF01870103. [DOI] [PubMed] [Google Scholar]
- Hille B. Charges and potentials at the nerve surface. Divalent ions and pH. J Gen Physiol. 1968 Feb;51(2):221–236. doi: 10.1085/jgp.51.2.221. [DOI] [PMC free article] [PubMed] [Google Scholar]
- Hille B. The selective inhibition of delayed potassium currents in nerve by tetraethylammonium ion. J Gen Physiol. 1967 May;50(5):1287–1302. doi: 10.1085/jgp.50.5.1287. [DOI] [PMC free article] [PubMed] [Google Scholar]
- Ito S., Hori N. Electrical characteristics of Triturus egg cells during cleavage. J Gen Physiol. 1966 May;49(5):1019–1027. doi: 10.1085/jgp.49.5.1019. [DOI] [PMC free article] [PubMed] [Google Scholar]
- Jaffe L. A. Fast block to polyspermy in sea urchin eggs is electrically mediated. Nature. 1976 May 6;261(5555):68–71. doi: 10.1038/261068a0. [DOI] [PubMed] [Google Scholar]
- Keynes R. D., Rojas E., Taylor R. E., Vergara J. Calcium and potassium systems of a giant barnacle muscle fibre under membrane potential control. J Physiol. 1973 Mar;229(2):409–455. doi: 10.1113/jphysiol.1973.sp010146. [DOI] [PMC free article] [PubMed] [Google Scholar]
- Kinoshita S., Yazaki I. The behaviour and localization of intracellular relaxing system during cleavage in the sea urchin egg. Exp Cell Res. 1967 Sep;47(3):449–458. doi: 10.1016/0014-4827(67)90003-1. [DOI] [PubMed] [Google Scholar]
- MAENO T. Electrical characteristics and activation potential of Bufo eggs. J Gen Physiol. 1959 Sep;43:139–157. doi: 10.1085/jgp.43.1.139. [DOI] [PMC free article] [PubMed] [Google Scholar]
- Mabuchi I. A myosin-like protein in the cortical layer of the sea urchin egg. J Cell Biol. 1973 Nov;59(2 Pt 1):542–547. doi: 10.1083/jcb.59.2.542. [DOI] [PMC free article] [PubMed] [Google Scholar]
- McLaughlin S. G., Szabo G., Eisenman G. Divalent ions and the surface potential of charged phospholipid membranes. J Gen Physiol. 1971 Dec;58(6):667–687. doi: 10.1085/jgp.58.6.667. [DOI] [PMC free article] [PubMed] [Google Scholar]
- Miyazaki S. I., Ohmori H., Sasaki S. Action potential and non-linear current-voltage relation in starfish oocytes. J Physiol. 1975 Mar;246(1):37–54. doi: 10.1113/jphysiol.1975.sp010879. [DOI] [PMC free article] [PubMed] [Google Scholar]
- Miyazaki S. I., Ohmori H., Sasaki S. Potassium rectifications of the starfish oocyte membrane and their changes during oocyte maturation. J Physiol. 1975 Mar;246(1):55–78. doi: 10.1113/jphysiol.1975.sp010880. [DOI] [PMC free article] [PubMed] [Google Scholar]
- Miyazaki S. I., Takahashi K., Tsuda K. Electrical excitability in the egg cell membrane of the tunicate. J Physiol. 1974 Apr;238(1):37–54. doi: 10.1113/jphysiol.1974.sp010509. [DOI] [PMC free article] [PubMed] [Google Scholar]
- Miyazaki S. I., Takahashi K., Tsuda K., Yoshii M. Analysis of non-linearity observed in the current-voltage relation of the tunicate embryo. J Physiol. 1974 Apr;238(1):55–77. doi: 10.1113/jphysiol.1974.sp010510. [DOI] [PMC free article] [PubMed] [Google Scholar]
- Miyazaki S., Takahashi K., Tsuda K. Calcium and sodium contributions to regenerative responses in the embryonic excitable cell membrane. Science. 1972 Jun 30;176(4042):1441–1443. doi: 10.1126/science.176.4042.1441. [DOI] [PubMed] [Google Scholar]
- Morrill G. A. Water and electrolyte changes in amphibian eggs at ovulation. Exp Cell Res. 1965 Dec;40(3):664–667. doi: 10.1016/0014-4827(65)90245-4. [DOI] [PubMed] [Google Scholar]
- NAKAJIMA S., IWASAKI S., OBATA K. Delayed rectification and anomalous rectification in frog's skeletal muscle membrane. J Gen Physiol. 1962 Sep;46:97–115. doi: 10.1085/jgp.46.1.97. [DOI] [PMC free article] [PubMed] [Google Scholar]
- Ohmori H., Yoshii M. Surface potential reflected in both gating and permeation mechanisms of sodium and calcium channels of the tunicate egg cell membrane. J Physiol. 1977 May;267(2):429–463. doi: 10.1113/jphysiol.1977.sp011821. [DOI] [PMC free article] [PubMed] [Google Scholar]
- Okamoto H., Takahashi K., Yoshii M. Membrane currents of the tunicate egg under the voltage-clamp condition. J Physiol. 1976 Jan;254(3):607–638. doi: 10.1113/jphysiol.1976.sp011249. [DOI] [PMC free article] [PubMed] [Google Scholar]
- Okamoto H., Takahashi K., Yoshii M. Two components of the calcium current in the egg cell membrane of the tunicate. J Physiol. 1976 Feb;255(2):527–561. doi: 10.1113/jphysiol.1976.sp011294. [DOI] [PMC free article] [PubMed] [Google Scholar]
- Palmer J. F., Slack C. Some bio-electric parameters of early Xenopus embryos. J Embryol Exp Morphol. 1970 Nov;24(3):535–553. [PubMed] [Google Scholar]
- Powers R. D., Tupper J. T. Some electrophysiological and permeability properties of the mouse egg. Dev Biol. 1974 Jun;38(2):320–331. doi: 10.1016/0012-1606(74)90010-4. [DOI] [PubMed] [Google Scholar]
- Sakai H. Contractile properties of protein threads from sea urchin eggs in relation to cell division. Int Rev Cytol. 1968;23:89–112. doi: 10.1016/s0074-7696(08)60270-3. [DOI] [PubMed] [Google Scholar]
- Slack C., Warner A. E. Intracellular and intercellular potentials in the early amphibian embryo. J Physiol. 1973 Jul;232(2):313–330. doi: 10.1113/jphysiol.1973.sp010272. [DOI] [PMC free article] [PubMed] [Google Scholar]
- Slack C., Warner A. E., Warren R. L. The distribution of sodium and potassium in amphibian embryos during early development. J Physiol. 1973 Jul;232(2):297–312. doi: 10.1113/jphysiol.1973.sp010271. [DOI] [PMC free article] [PubMed] [Google Scholar]
- Sperelakis N., Schneider M. F., Harris E. J. Decreased K+ conductance produced by Ba++ in frog sartorius fibers. J Gen Physiol. 1967 Jul;50(6):1565–1583. doi: 10.1085/jgp.50.6.1565. [DOI] [PMC free article] [PubMed] [Google Scholar]
- Steinhardt R. A., Epel D. Activation of sea-urchin eggs by a calcium ionophore. Proc Natl Acad Sci U S A. 1974 May;71(5):1915–1919. doi: 10.1073/pnas.71.5.1915. [DOI] [PMC free article] [PubMed] [Google Scholar]
- Steinhardt R. A., Epel D., Carroll E. J., Jr, Yanagimachi R. Is calcium ionophore a universal activator for unfertilised eggs? Nature. 1974 Nov 1;252(5478):41–43. doi: 10.1038/252041a0. [DOI] [PubMed] [Google Scholar]
- Steinhardt R. A., Lundin L., Mazia D. Bioelectric responses of the echinoderm egg to fertilization. Proc Natl Acad Sci U S A. 1971 Oct;68(10):2426–2430. doi: 10.1073/pnas.68.10.2426. [DOI] [PMC free article] [PubMed] [Google Scholar]
- Takata M., Pickard W. F., Lettvin J. Y., Moore J. W. Ionic conductance changes in lobster axon membrane when lanthanum is substituted for calcium. J Gen Physiol. 1966 Nov;50(2):461–471. doi: 10.1085/jgp.50.2.461. [DOI] [PMC free article] [PubMed] [Google Scholar]
- Vacquier V. D. The isolation of intact cortical granules from sea urchin eggs: calcium lons trigger granule discharge. Dev Biol. 1975 Mar;43(1):62–74. doi: 10.1016/0012-1606(75)90131-1. [DOI] [PubMed] [Google Scholar]
- WERMAN R., GRUNDFEST H. Graded and all-or-none electrogenesis in arthropod muscle. II. The effects of alkali-earth and onium ions on lobster muscle fibers. J Gen Physiol. 1961 May;44:997–1027. doi: 10.1085/jgp.44.5.997. [DOI] [PMC free article] [PubMed] [Google Scholar]