Abstract
1. The relationship between active force and velocity of shortening was studied during tetanic contraction of isolated semitendinosus muscle fibres of the frog (0·5-2·0° C). Measurements were carried out with the fibre immersed in isotonic (1·00R) Ringer solution and in solutions that were made hypotonic by reduction of NaCl (osmolality 0·62 and 0·81 of normal Ringer) and hypertonic by addition of sucrose (osmolality 1·22 and 1·44 of normal Ringer).
2. The force—velocity relation was hyperbolic at loads lower than 80% of measured isometric force (P0) but exhibited a reversed curvature between 0·8P0 and P0. The maximum velocity of shortening was determined in two different ways: (i) by extrapolation to zero load from force—velocity data truncated at 0·8P0 (computer fitting of hyperbola, leastsquares method) and (ii) by recording the time required to take up the slack of the fibre after a quick release during tetanus.
3. Isometric force and maximum speed of shortening both changed inversely with the tonicity of the extracellular medium. Immersion of the fibre in 0·81R hypotonic solution caused active tension and shortening velocity to increase by 10 ± 1% (mean ± S.E. of mean, n = 14) and 12 ± 1%, respectively. Conversely, force and shortening velocity decreased by 12 ± 1% (n = 13) and 22 ± 2% when normal Ringer was replaced by 1·22R hypertonic solution. These changes doubled when the tonicity was altered from normal Ringer to 0·62R and 1·44R, respectively.
4. Changes in fibre cross-sectional area equivalent to those obtained in the 0·81R and 1·22R solutions (+11% and -13%, respectively) were produced by varying the sarcomere length within the range 2·0-2·5 μm in the normal Ringer solution. Maximum velocity of shortening remained very nearly constant under these conditions, indicating that the shortening velocity, like the isometric force, is not critically dependent on changes in myofilament lattice width over the range considered.
5. The results support the view that both shortening velocity and active force are modulated by changes of the intracellular ionic strength above and below the level that normally exists in the intact muscle fibre.
Full text
PDF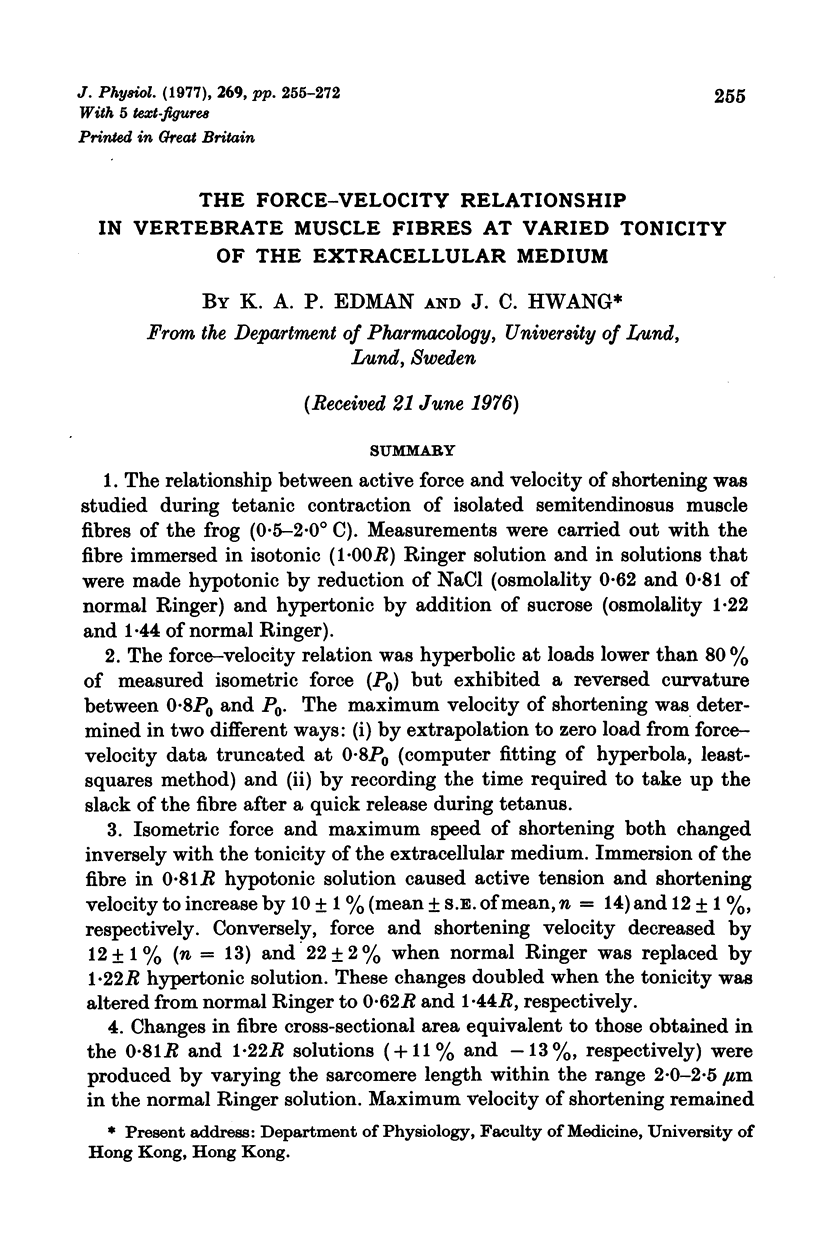
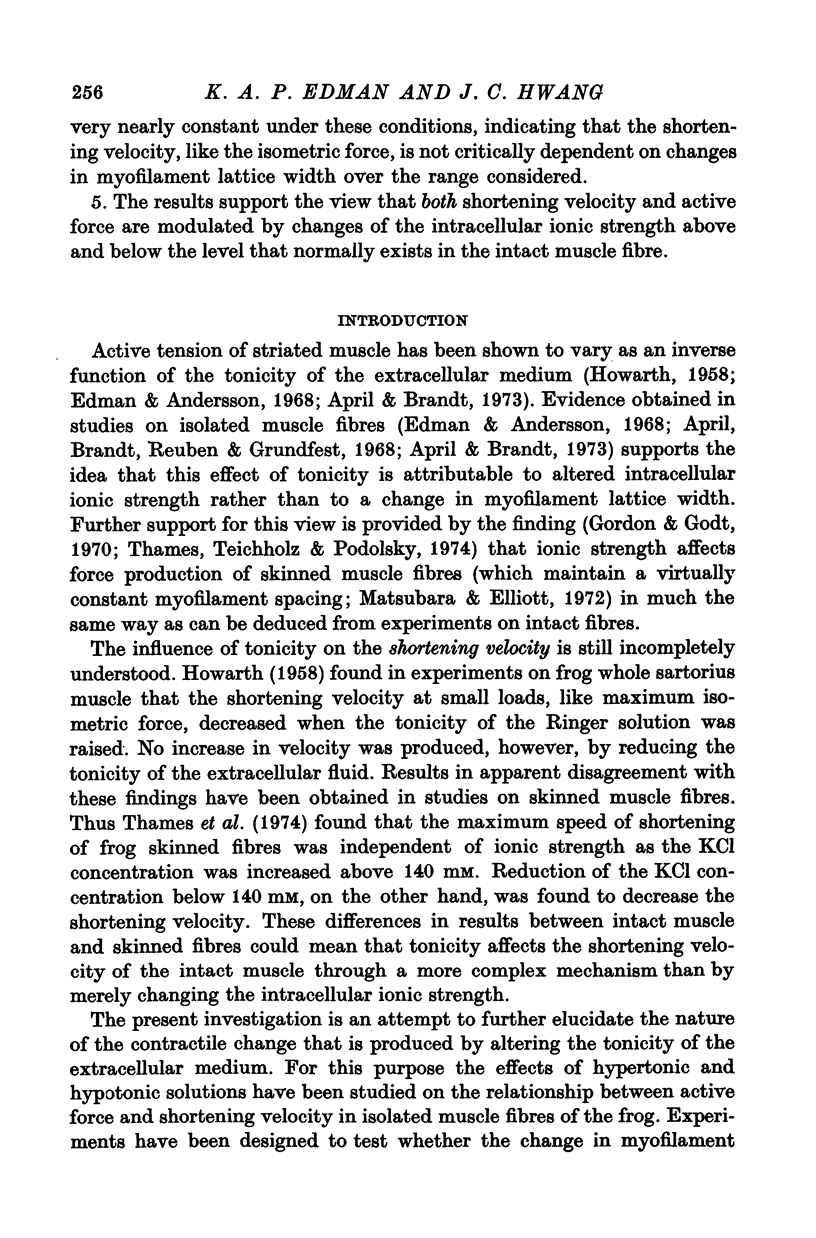
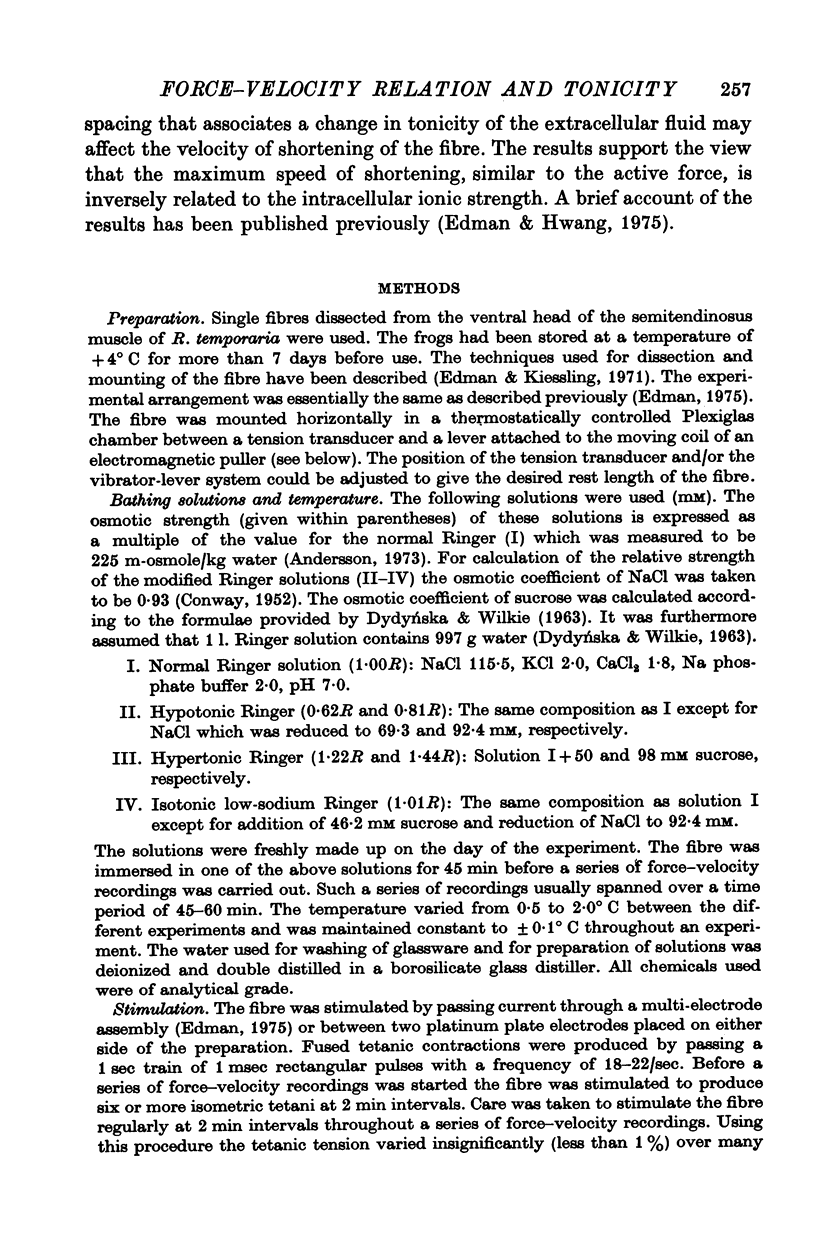
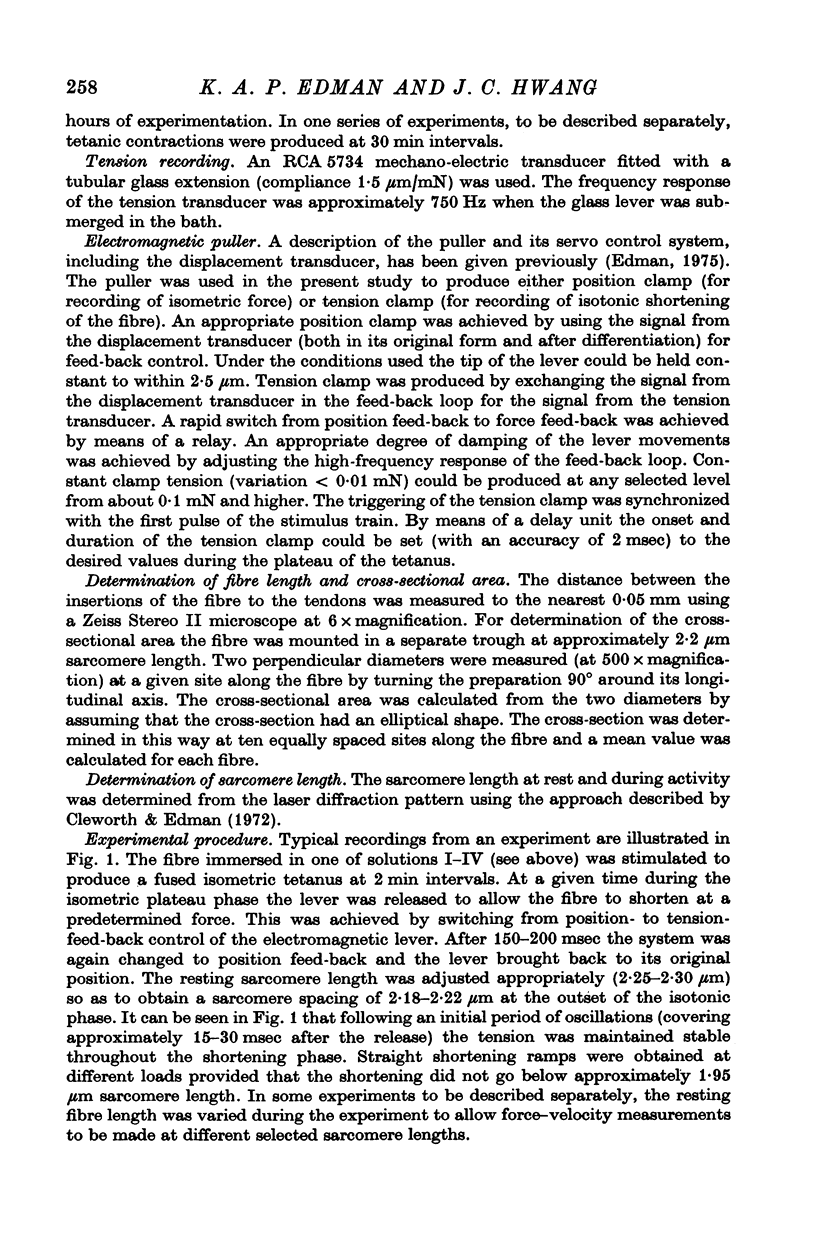
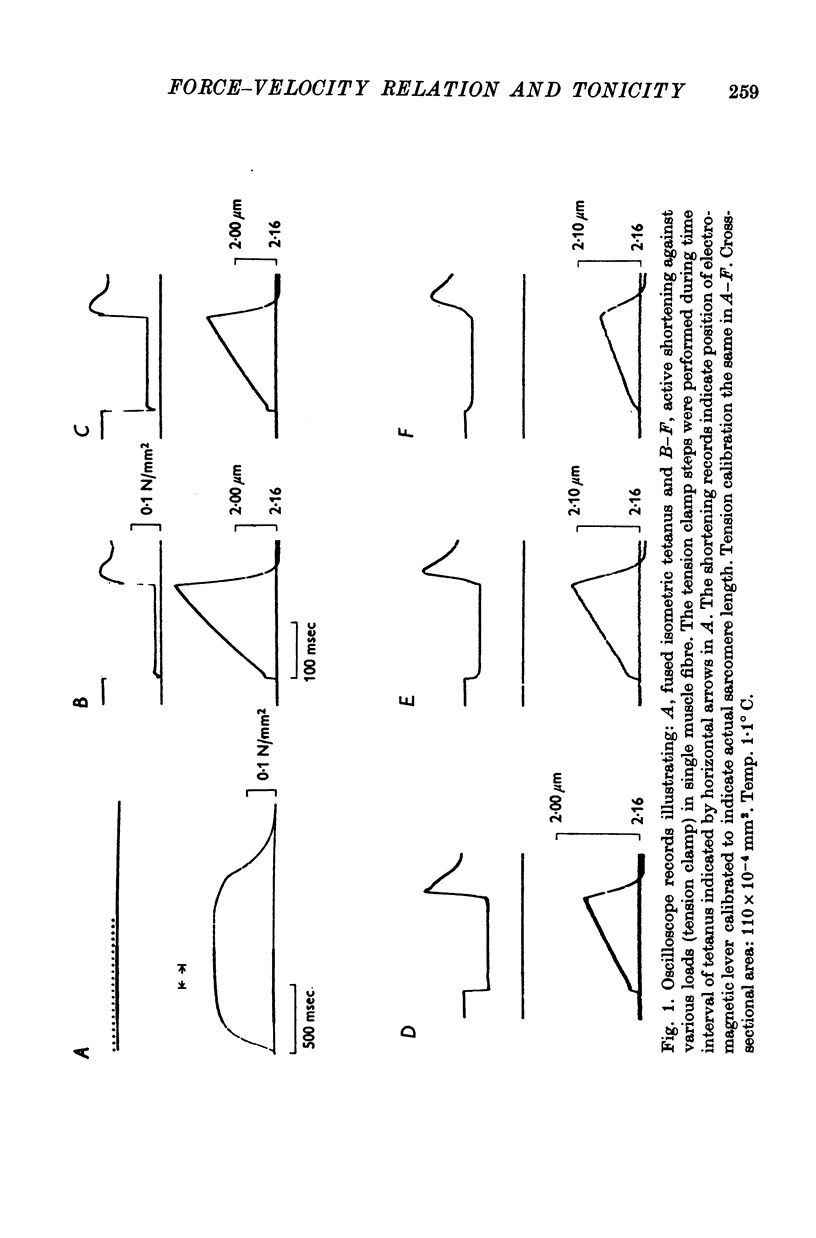
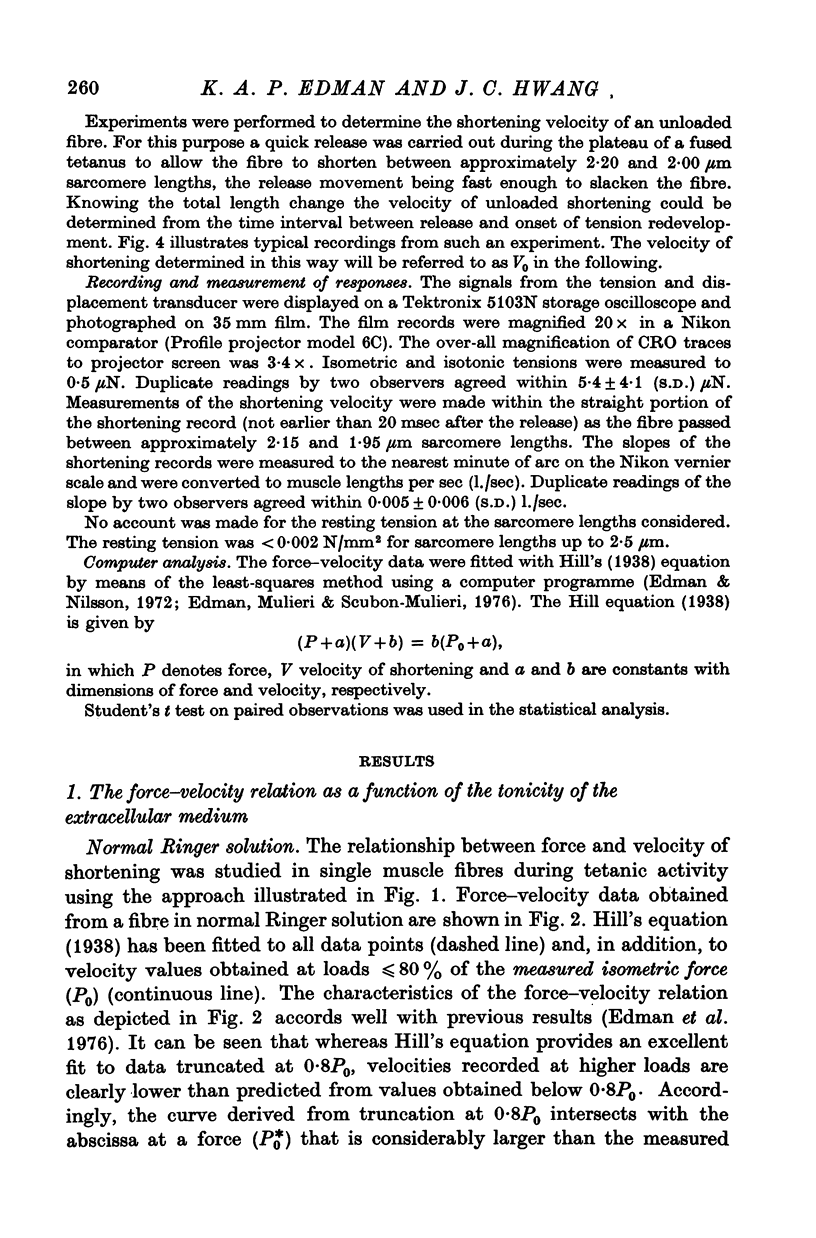
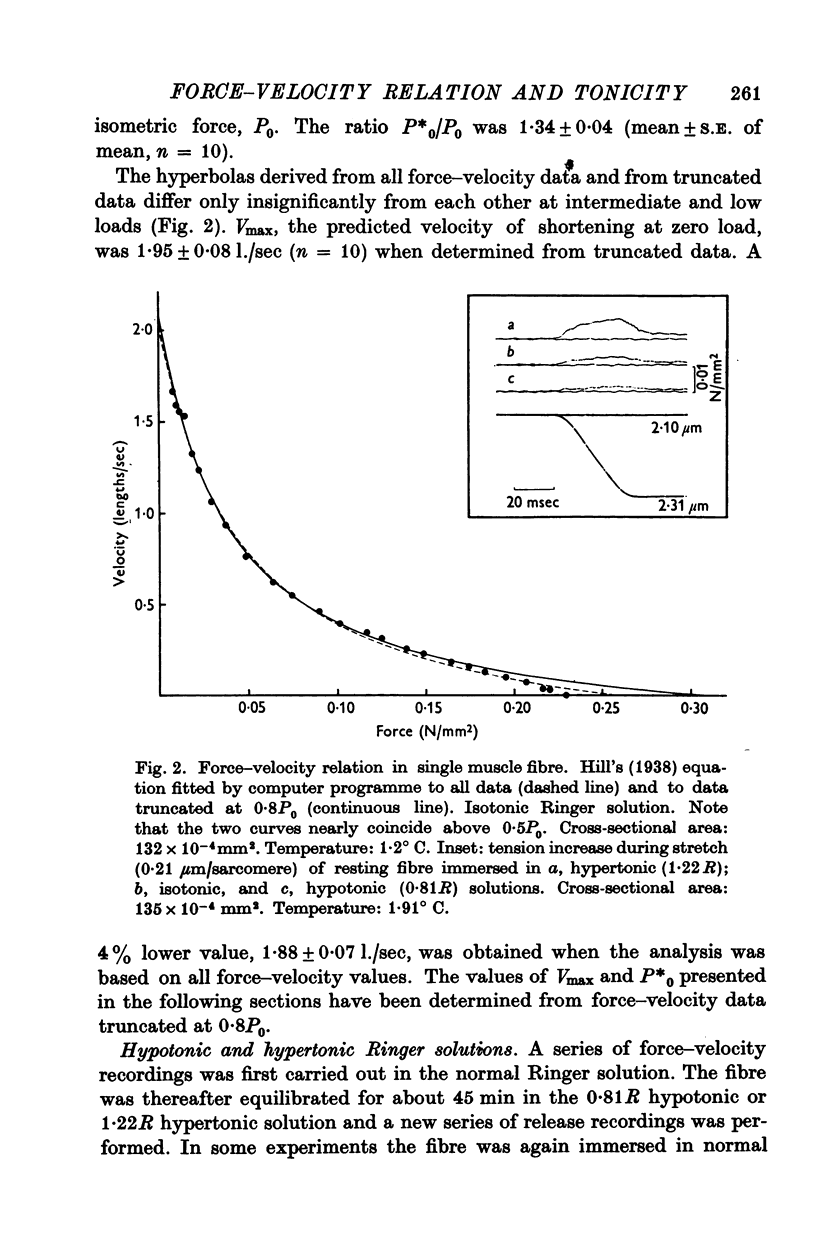
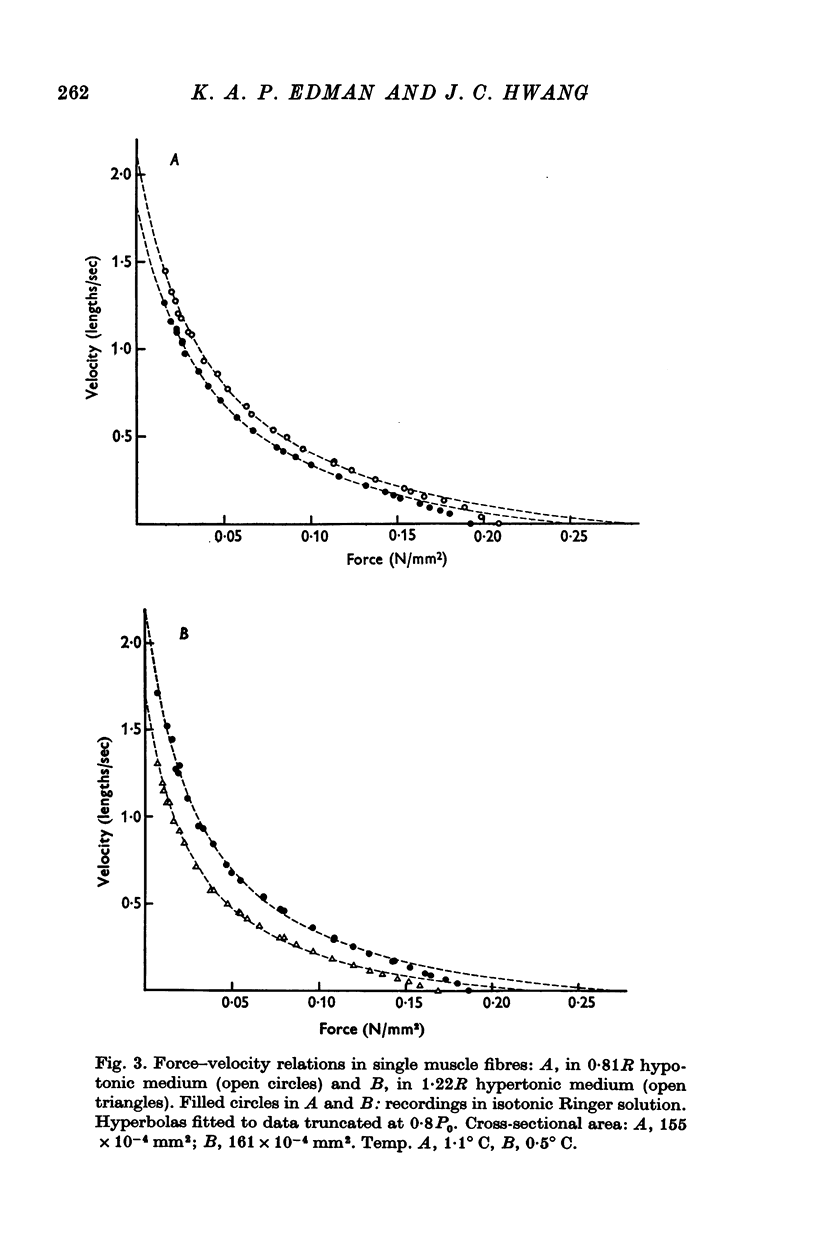
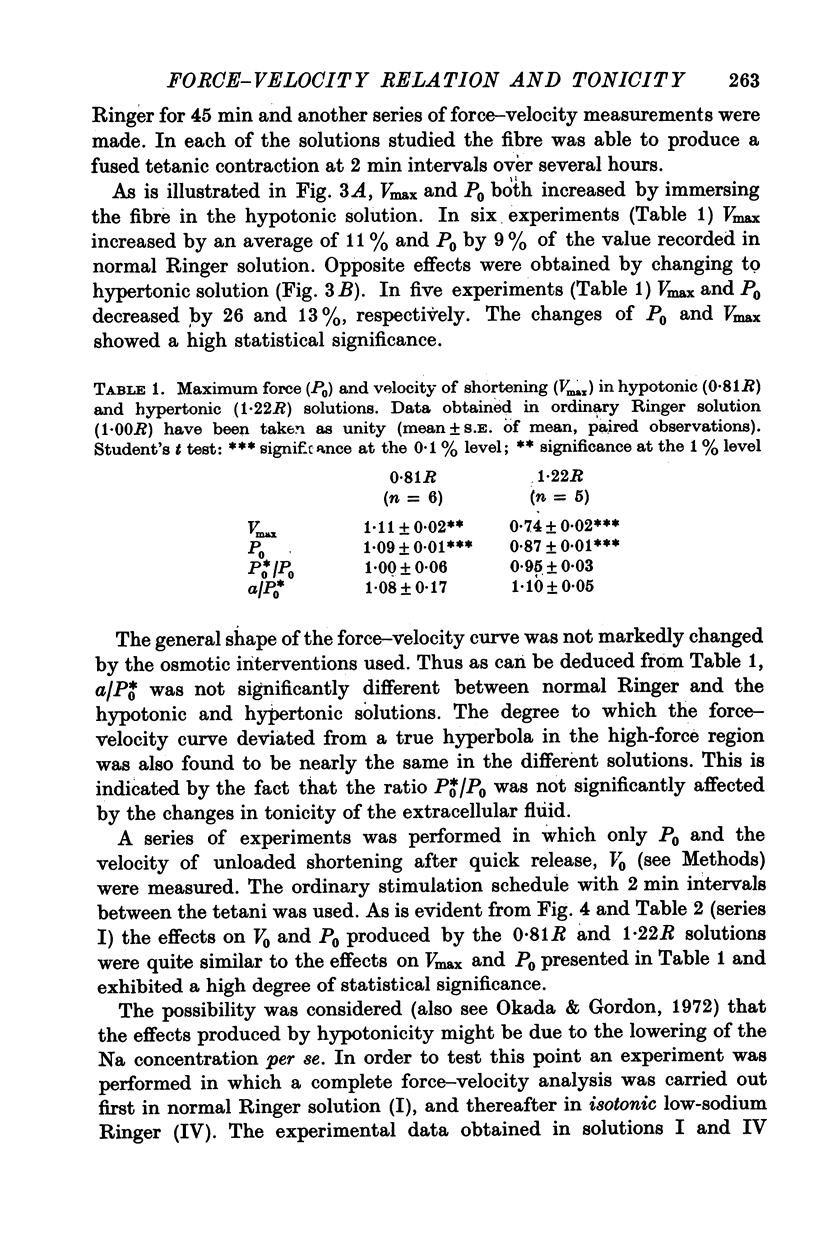
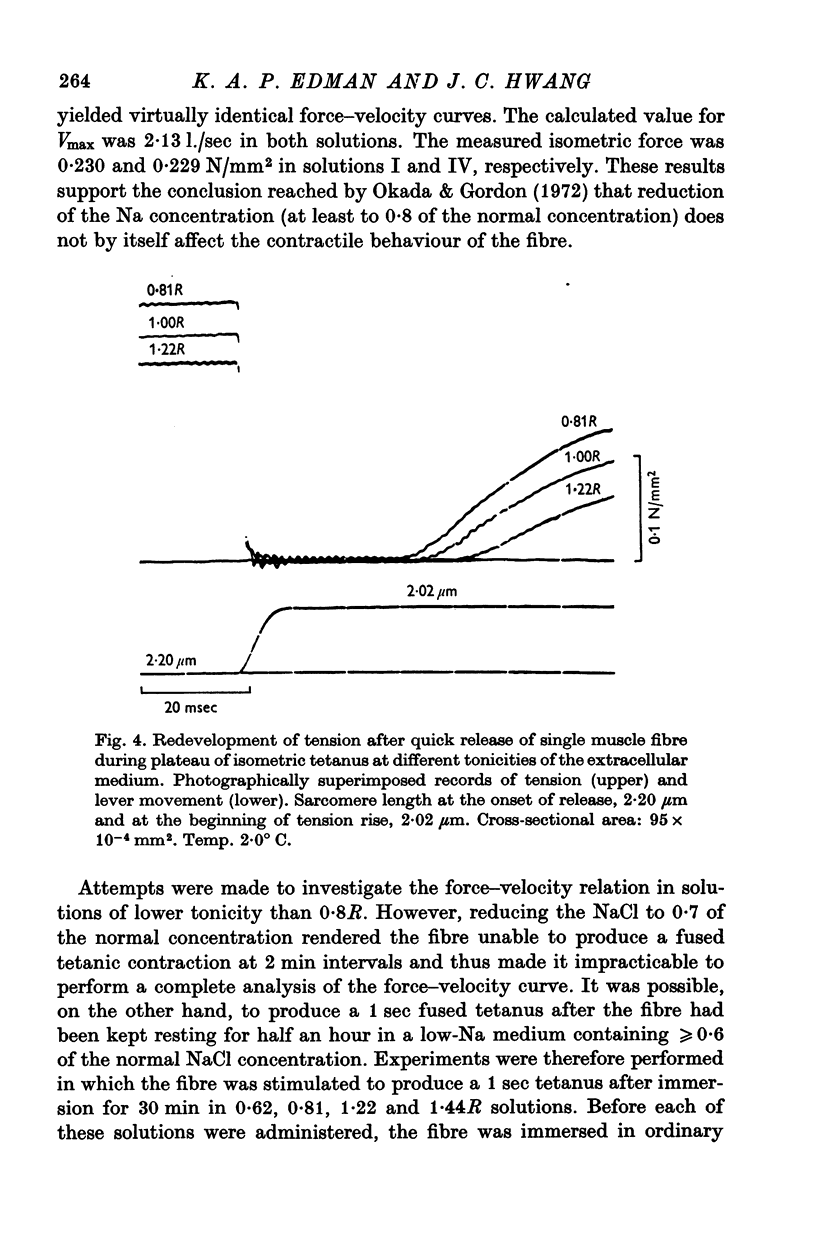
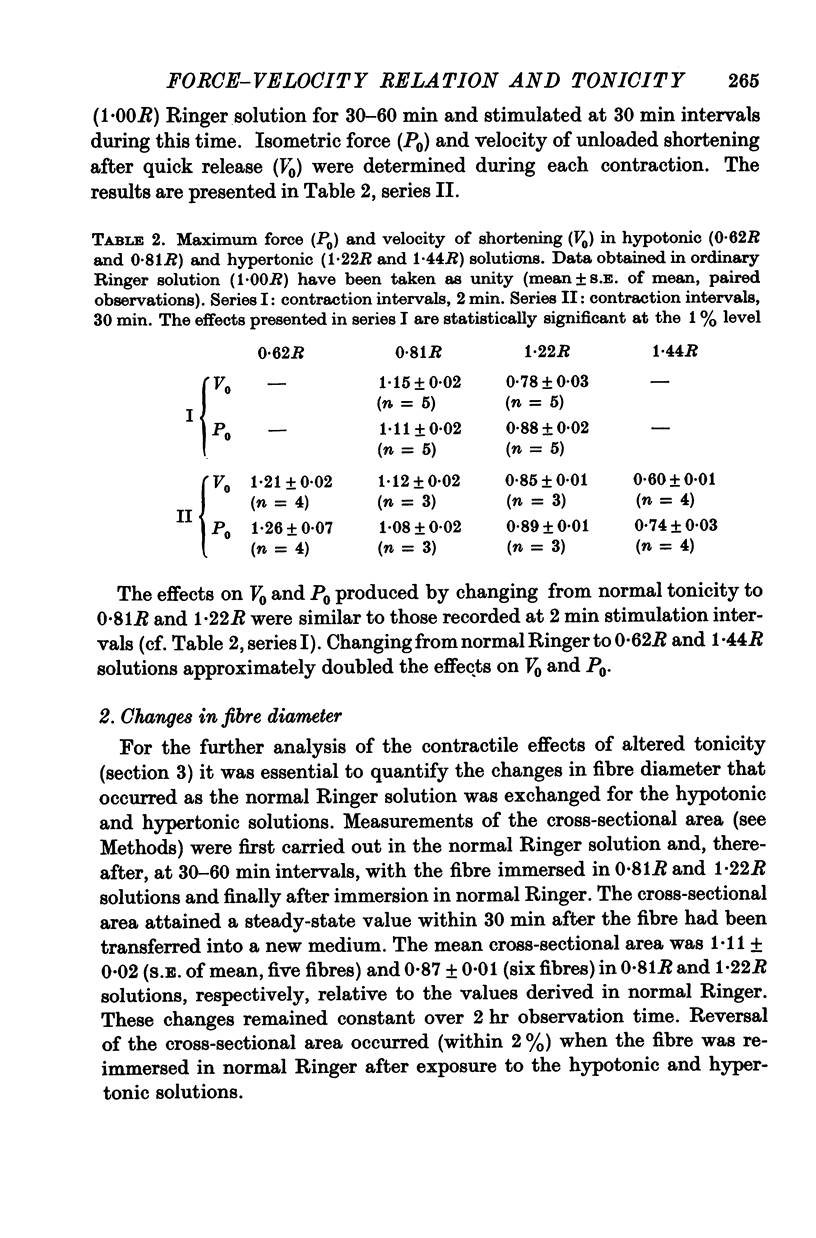
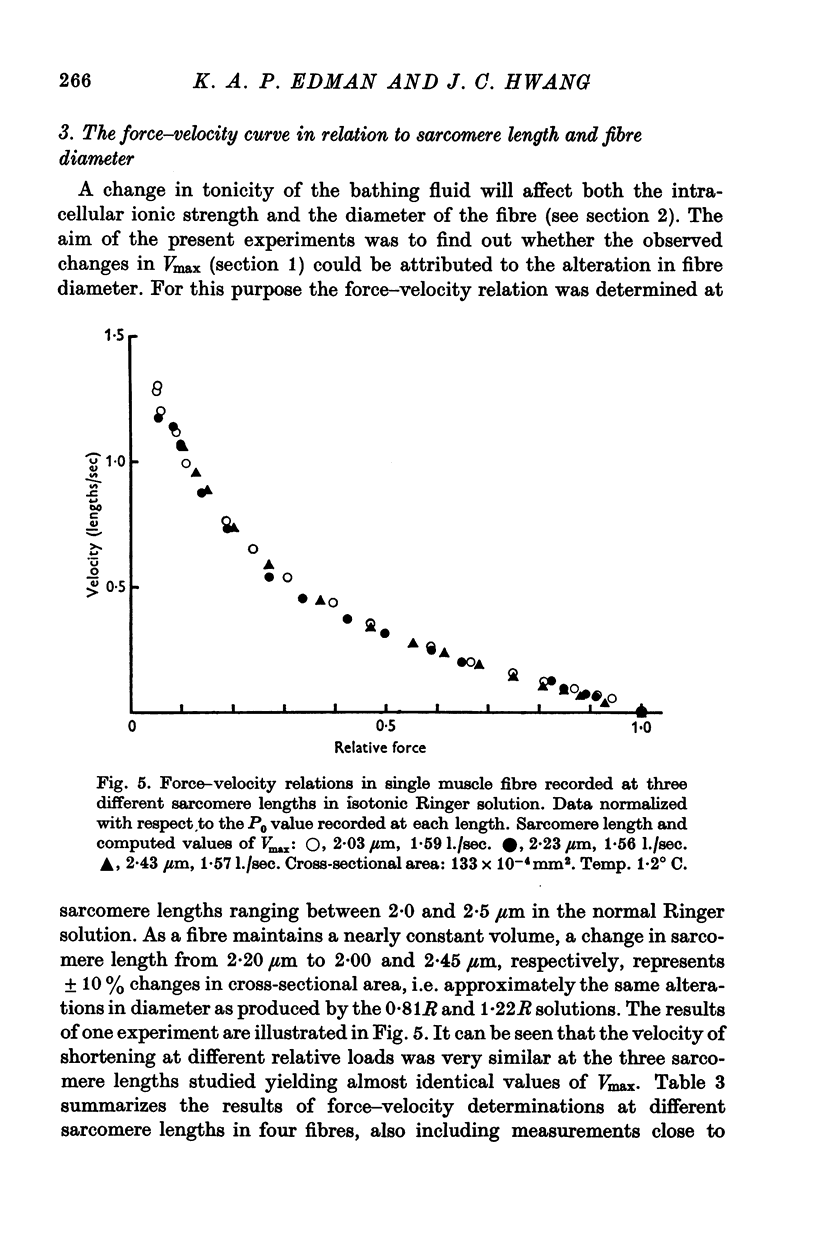
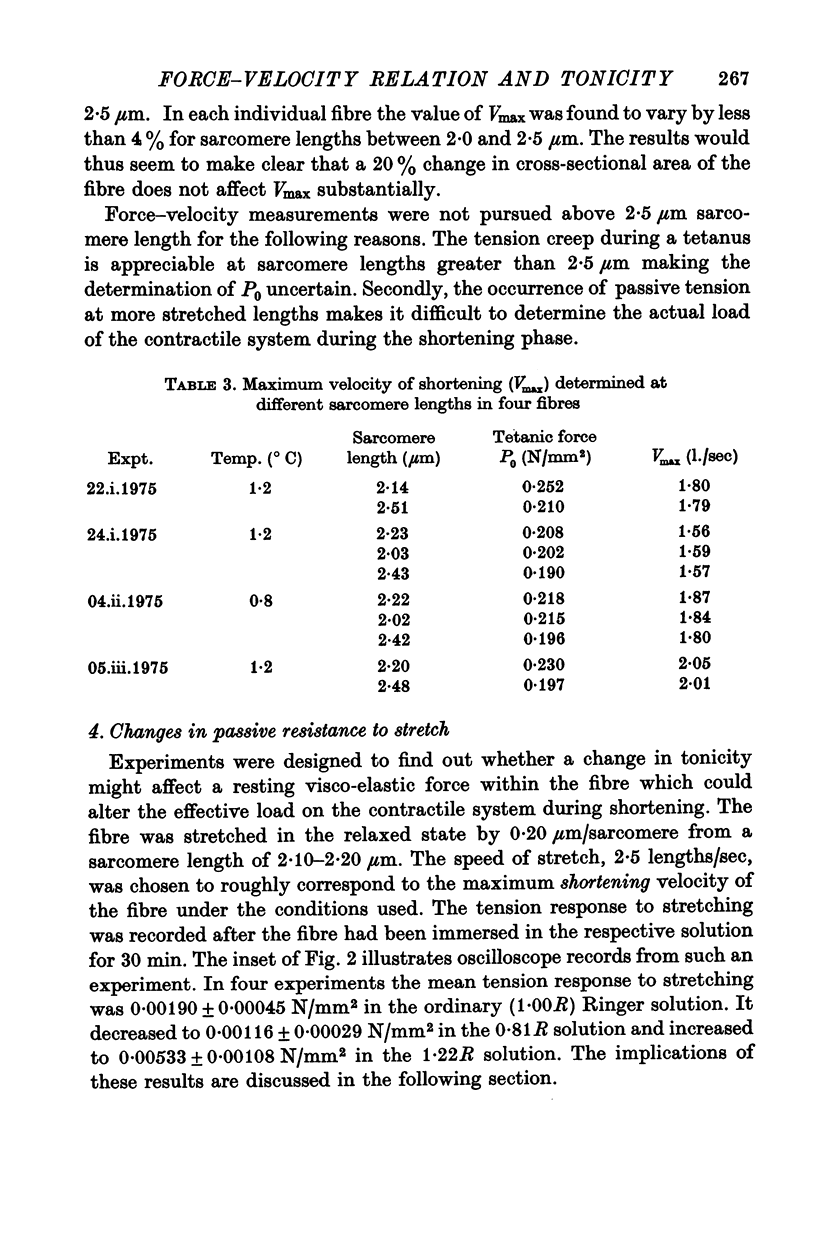
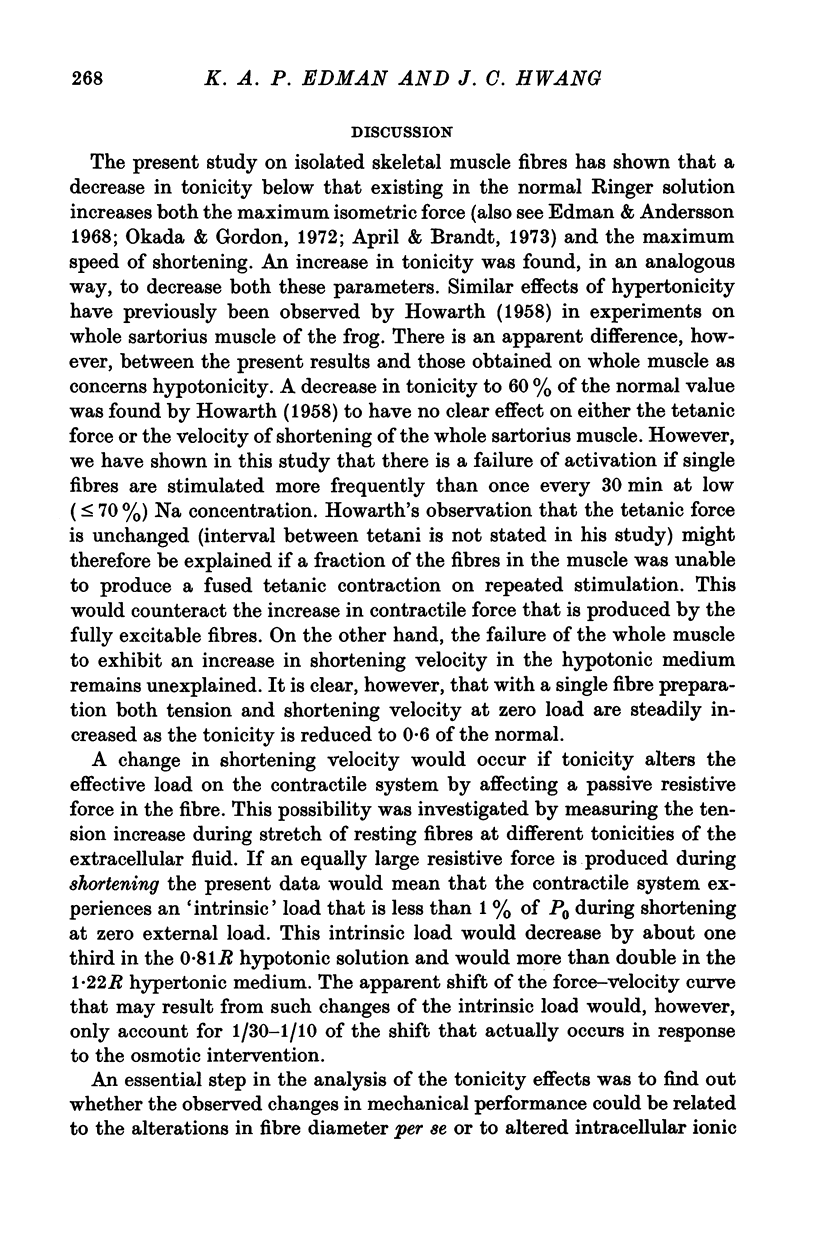
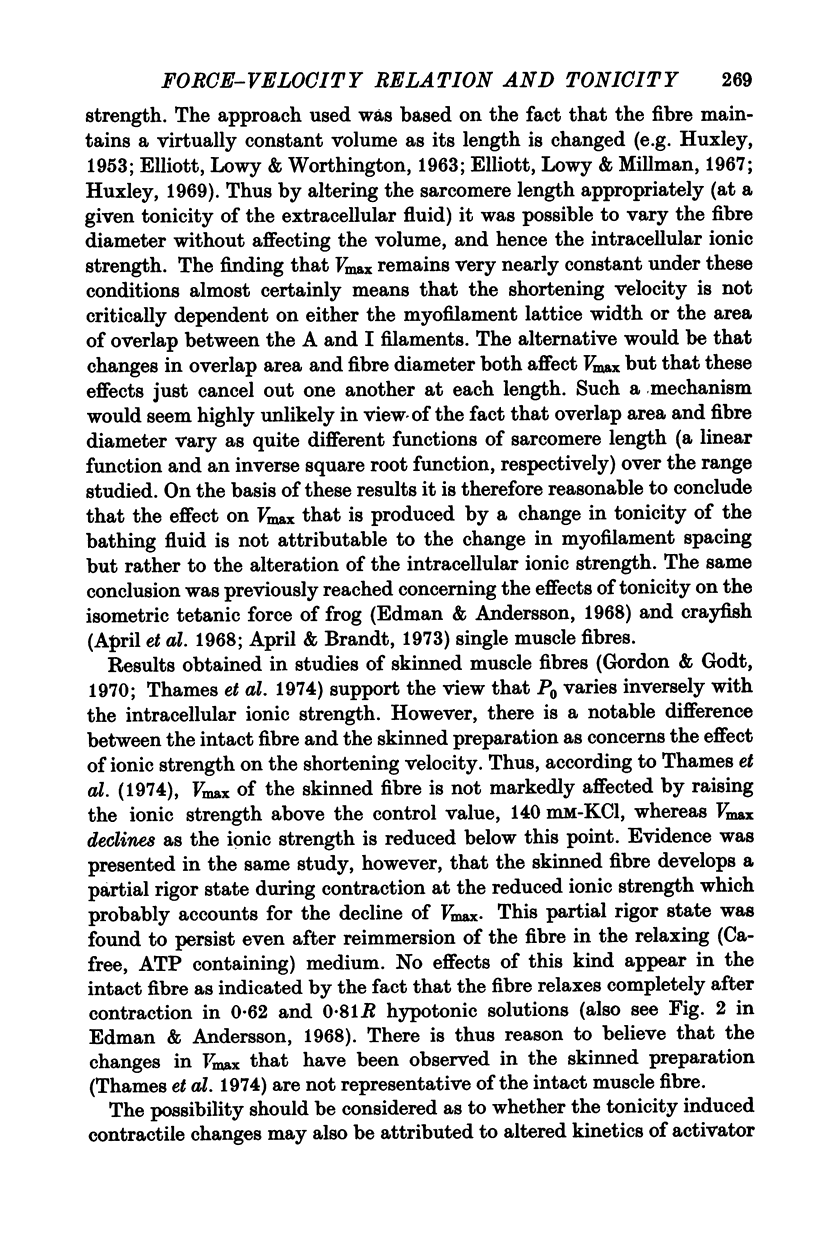
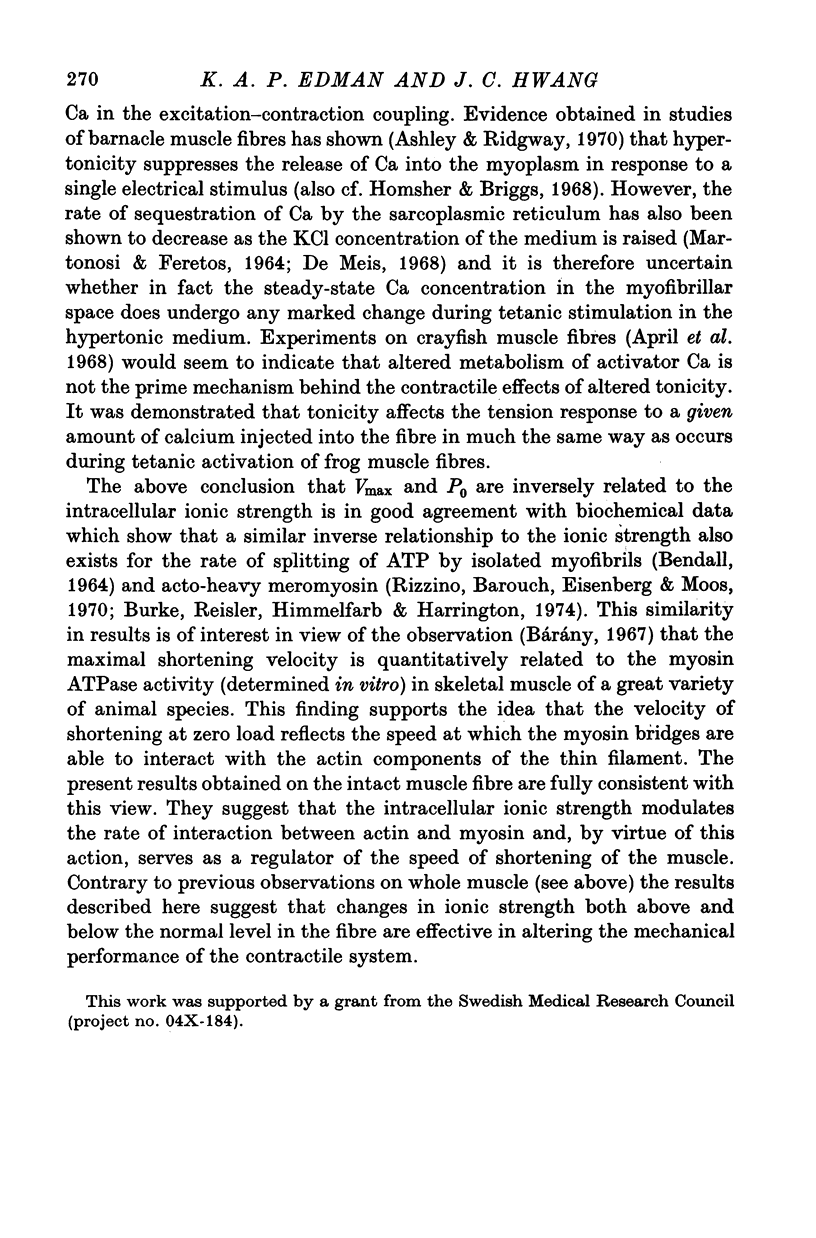
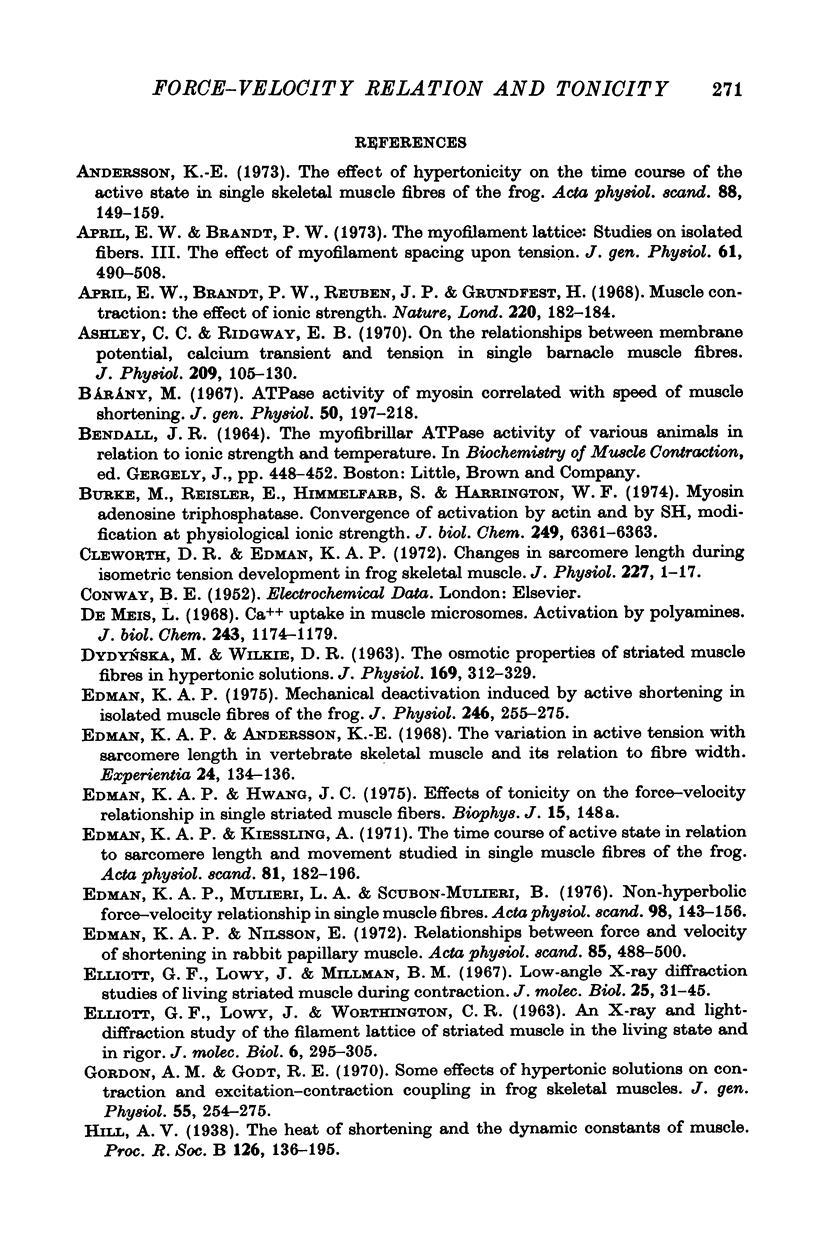
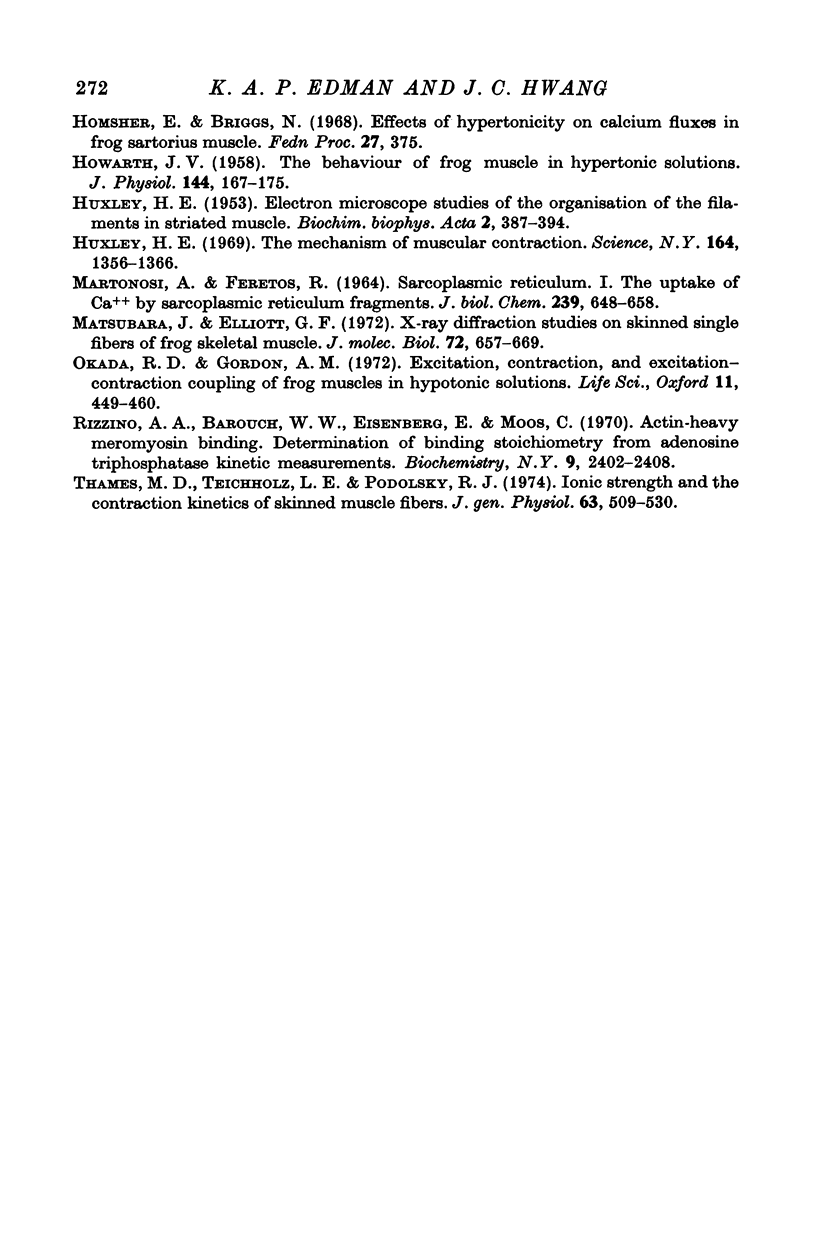
Selected References
These references are in PubMed. This may not be the complete list of references from this article.
- Andersson K. E. The effect of hypertonicity on the time course of the active state in single skeletal muscle fibres of the frog. Acta Physiol Scand. 1973 Jun;88(2):149–159. doi: 10.1111/j.1748-1716.1973.tb05442.x. [DOI] [PubMed] [Google Scholar]
- April E. W., Brandt P. W. The myofilament lattice: studies on isolated fibers. 3. The effect of myofilament spacing upon tension. J Gen Physiol. 1973 Apr;61(4):490–508. doi: 10.1085/jgp.61.4.490. [DOI] [PMC free article] [PubMed] [Google Scholar]
- April E., Brandt P. W., Reuben J. P., Grundfest H. Muscle contraction: the effect of ionic strength. Nature. 1968 Oct 12;220(5163):182–184. doi: 10.1038/220182a0. [DOI] [PubMed] [Google Scholar]
- Ashley C. C., Ridgway E. B. On the relationships between membrane potential, calcium transient and tension in single barnacle muscle fibres. J Physiol. 1970 Jul;209(1):105–130. doi: 10.1113/jphysiol.1970.sp009158. [DOI] [PMC free article] [PubMed] [Google Scholar]
- Burke M., Reisler E., Himmelfarb S., Harrington W. F. Myosin adenosine triphosphatase. Convergence of activation by actin and by SH1 modification at physiological ionic strength. J Biol Chem. 1974 Oct 10;249(19):6361–6363. [PubMed] [Google Scholar]
- Bárány M. ATPase activity of myosin correlated with speed of muscle shortening. J Gen Physiol. 1967 Jul;50(6 Suppl):197–218. doi: 10.1085/jgp.50.6.197. [DOI] [PMC free article] [PubMed] [Google Scholar]
- Cleworth D. R., Edman K. A. Changes in sarcomere length during isometric tension development in frog skeletal muscle. J Physiol. 1972 Dec;227(1):1–17. doi: 10.1113/jphysiol.1972.sp010016. [DOI] [PMC free article] [PubMed] [Google Scholar]
- De Meis L. Ca++ uptake in muscle microsomes. Activation by polyamines. J Biol Chem. 1968 Mar 25;243(6):1174–1179. [PubMed] [Google Scholar]
- Edman K. A., Andersson K. E. The variation in active tension with sarcomere length in vertebrate skeletal muscle and its relation to fibre width. Experientia. 1968 Feb 15;24(2):134–136. doi: 10.1007/BF02146942. [DOI] [PubMed] [Google Scholar]
- Edman K. A., Kiessling A. The time course of the active state in relation to sarcomere length and movement studied in single skeletal muscle fibres of the frog. Acta Physiol Scand. 1971 Feb;81(2):182–196. doi: 10.1111/j.1748-1716.1971.tb04891.x. [DOI] [PubMed] [Google Scholar]
- Edman K. A. Mechanical deactivation induced by active shortening in isolated muscle fibres of the frog. J Physiol. 1975 Mar;246(1):255–275. doi: 10.1113/jphysiol.1975.sp010889. [DOI] [PMC free article] [PubMed] [Google Scholar]
- Edman K. A., Mulieri L. A., Scubon-Mulieri B. Non-hyperbolic force-velocity relationship in single muscle fibres. Acta Physiol Scand. 1976 Oct;98(2):143–156. doi: 10.1111/j.1748-1716.1976.tb00234.x. [DOI] [PubMed] [Google Scholar]
- Edman K. A., Nilsson E. Relationships between force and velocity of shortening in rabbit papillary muscle. Acta Physiol Scand. 1972 Aug;85(4):488–500. doi: 10.1111/j.1748-1716.1971.tb05286.x. [DOI] [PubMed] [Google Scholar]
- Elliott G. F., Lowy J., Millman B. M. Low-angle x-ray diffraction studies of living striated muscle during contraction. J Mol Biol. 1967 Apr 14;25(1):31–45. doi: 10.1016/0022-2836(67)90277-x. [DOI] [PubMed] [Google Scholar]
- Gordon A. M., Godt R. E. Some effects of hypertonic solutions on contraction and excitation-contraction coupling in frog skeletal muscles. J Gen Physiol. 1970 Feb;55(2):254–275. doi: 10.1085/jgp.55.2.254. [DOI] [PMC free article] [PubMed] [Google Scholar]
- HOWARTH J. V. The behaviour of frog muscle in hypertonic solutions. J Physiol. 1958 Nov 10;144(1):167–175. doi: 10.1113/jphysiol.1958.sp006093. [DOI] [PMC free article] [PubMed] [Google Scholar]
- HUXLEY H. E. Electron microscope studies of the organisation of the filaments in striated muscle. Biochim Biophys Acta. 1953 Nov;12(3):387–394. doi: 10.1016/0006-3002(53)90156-5. [DOI] [PubMed] [Google Scholar]
- MARTONOSI A., FERETOS R. SARCOPLASMIC RETICULUM. I. THE UPTAKE OF CA++ BY SARCOPLASMIC RETICULUM FRAGMENTS. J Biol Chem. 1964 Feb;239:648–658. [PubMed] [Google Scholar]
- Matsubara I., Elliott G. F. X-ray diffraction studies on skinned single fibres of frog skeletal muscle. J Mol Biol. 1972 Dec 30;72(3):657–669. doi: 10.1016/0022-2836(72)90183-0. [DOI] [PubMed] [Google Scholar]
- Okada R. D., Gordon A. M. Excitation, contraction, and excitation-contraction coupling of frog muscles in hypotonic solutions. Life Sci I. 1972 May 1;11(9):449–460. doi: 10.1016/0024-3205(72)90117-8. [DOI] [PubMed] [Google Scholar]
- Rizzino A. A., Barouch W. W., Eisenberg E., Moos C. Actin-heavy meromyosin biding. Determination of binding stoichiometry from adenosine triphosphatase kinetic measurements. Biochemistry. 1970 Jun 9;9(12):2402–2408. doi: 10.1021/bi00814a003. [DOI] [PubMed] [Google Scholar]
- Thames M. D., Teichholz L. E., Podolsky R. J. Ionic strength and the contraction kinetics of skinned muscle fibers. J Gen Physiol. 1974 Apr;63(4):509–530. doi: 10.1085/jgp.63.4.509. [DOI] [PMC free article] [PubMed] [Google Scholar]