Abstract
1. The mechanism by which the fluorescent, cationic dye diS-C3-(5) responds to the membrane potential of red blood cells has been investigated. 2. The dye in aqueous solution absorbs most strongly at 650 nm. Addition of white, haemoglobin-free membranes red shifts the absorption maximum ca. 20 nm, while addition of membrane-free cell lysate results in the appearance of a new dye absorption peak at 590 nm. Thus the dye binds both to cell membranes and to cell contents. The component of the cytoplasm which binds the dye is non-dialysable, presumably haemoglobin. 3. Dye added to a suspension of intact cells shows a strong absorption at 590 nm indicating that the dye has bound to the cell contents and that the membrane is permeable to the dye. 4. The amount of dye which partitions into (and on to) the cells can be determined, as reported by Sims, Waggoner, Wang & Hoffman (1974), from the fluorescence of the dye remaining in the supernatant after the cells are centrifuged to the bottom of the suspension. In most conditions the proportion of the cell associated dye which is either free inside the cell or bound to the outside face of the membrane is negligible compared to the proportion bound to the cell contents. 5. On the assumption that the dye is not actively transported, the ratio of the equilibrium dye activities inside and outside the cell, ai/ao, is determined by the membrane potential according to the Nernst relation. Driving the membrane potenial negative then increases the cell associated dye by increasing the activity ratio and hence ai and the amount of dye bound to cell contents. 6. At the known Donnan equilibrium potential the internal dye activity can be calculated from the external activity. An empirical relation between cell associated dye and internal activity has been determined by measuring the dye partition between cells and medium at different external activities. 7. Using this empirial relation, and providing that any changes in cell composition do not affect the dye binding, the internal activity at any potential can be calculated from the measured amount of cell associated dye. The external activity can be estimated fluorimetrically. The membrane potential is then calculated from the activity ratio. 8. The membrane potenial of cells has been altered by adding valinomycin in the presence of different K gradients. Under the conditions used, the 'constant field' permeability for K-Val is 15-20 times that of Cl. 9. Dye binding to haemoglobin is influenced by pH and thus dye partitioning into cells changes with intracellular pH. Increasing intracellular pH increases the amount of dye partitioned, while decreasing pH decreases this amount. 10. When large potentials are produced with valinomycin there is no change in intracellular pH. This result indicates that in red blood cells intracellular pH is determined by the external pH and the Cl concentration ratio and not by the membrane potentials. 11. DiS-C3-(5) can be used to estimate potentials across resealed ghost membranes...
Full text
PDF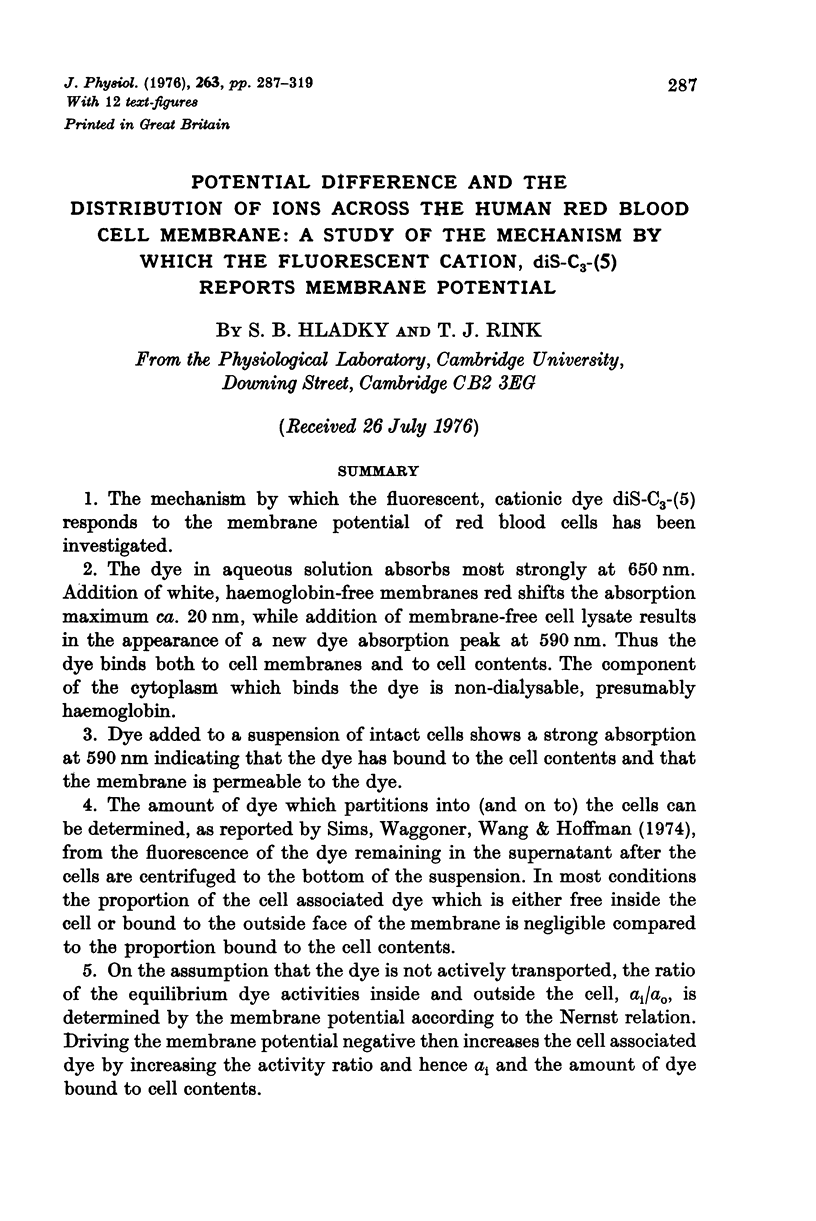
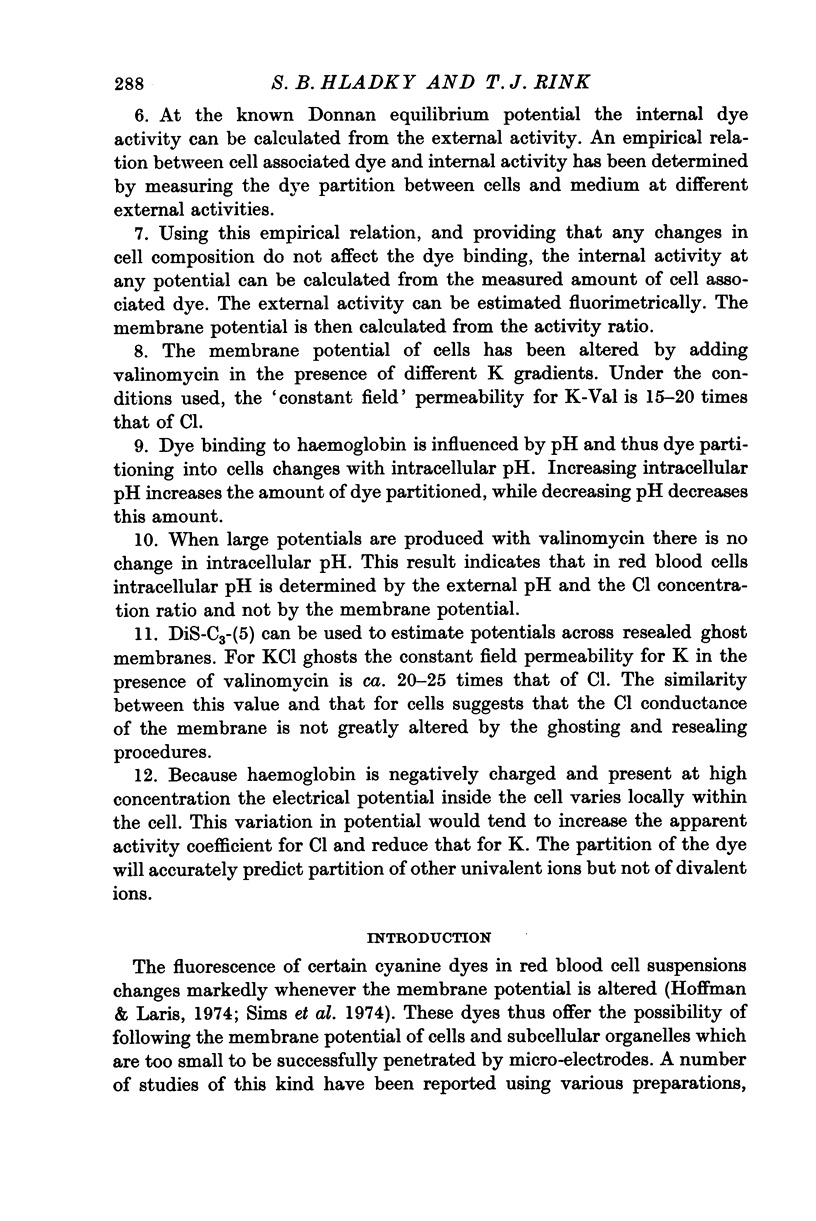
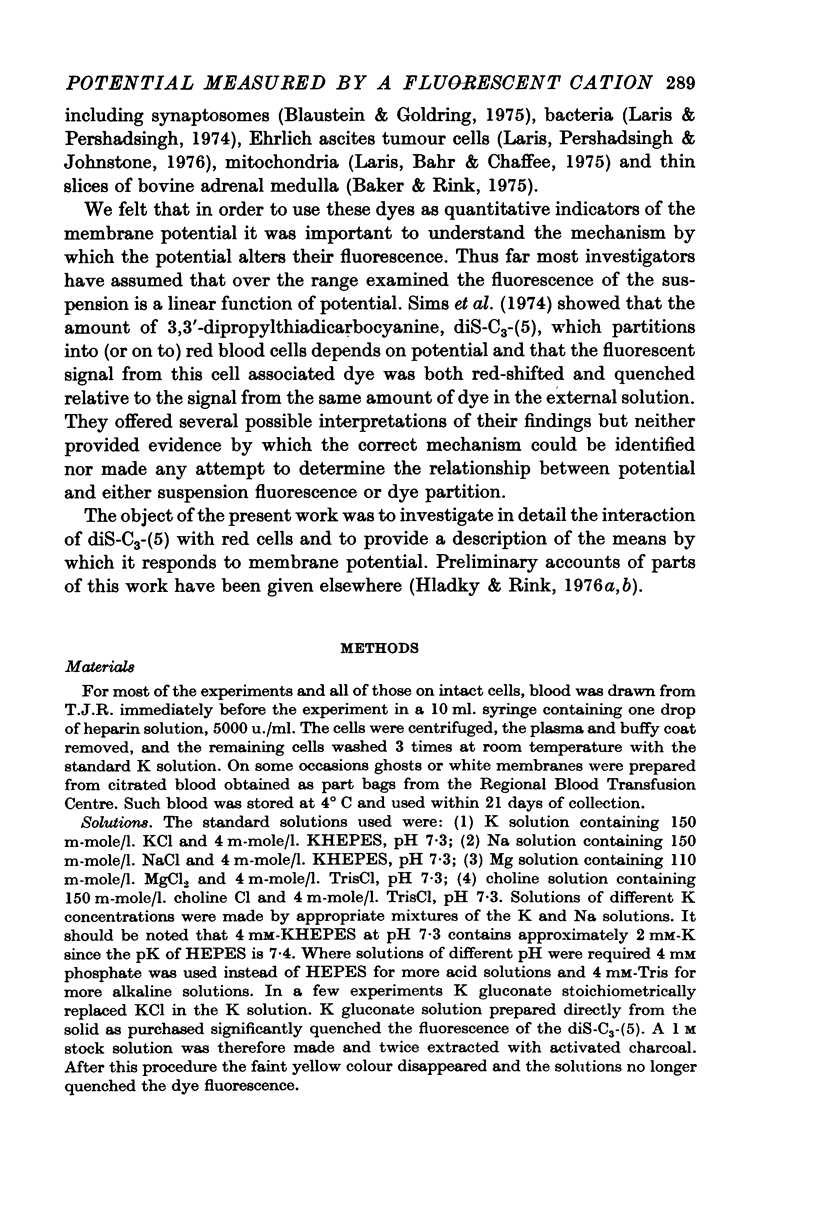
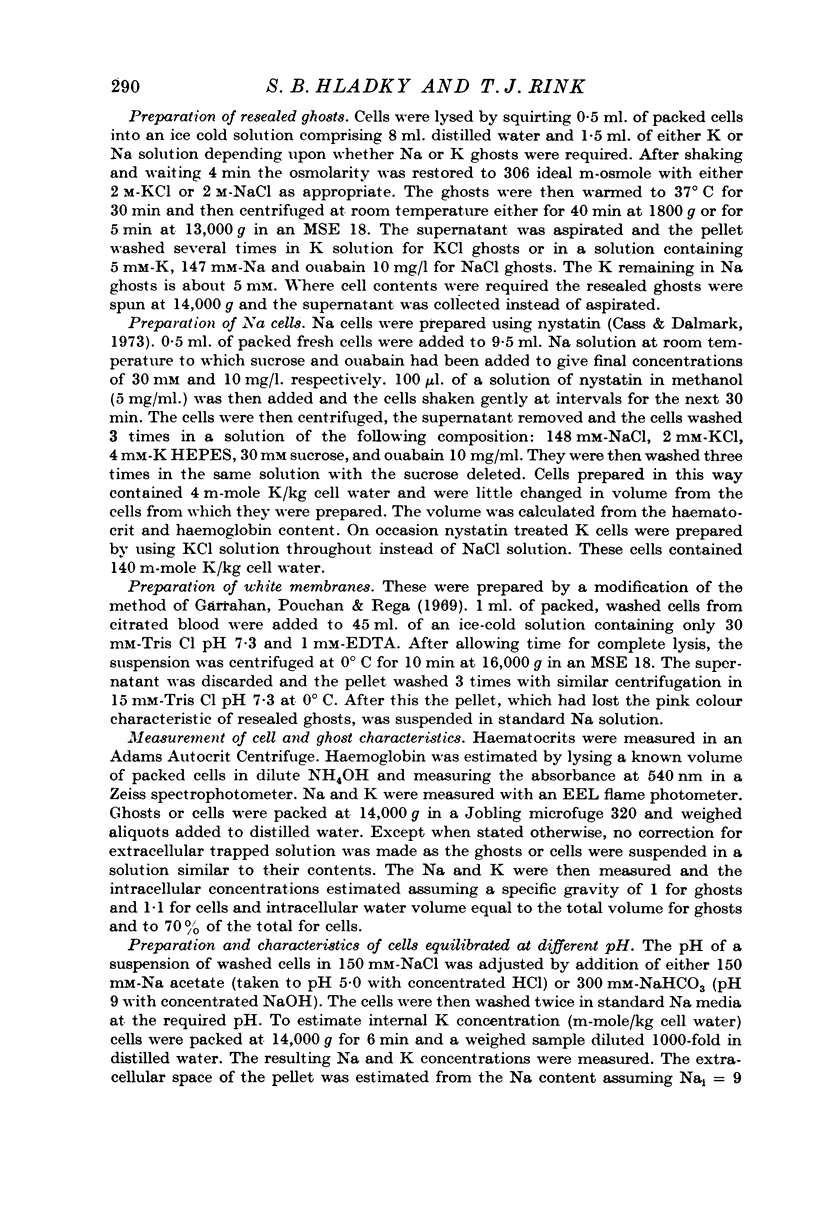
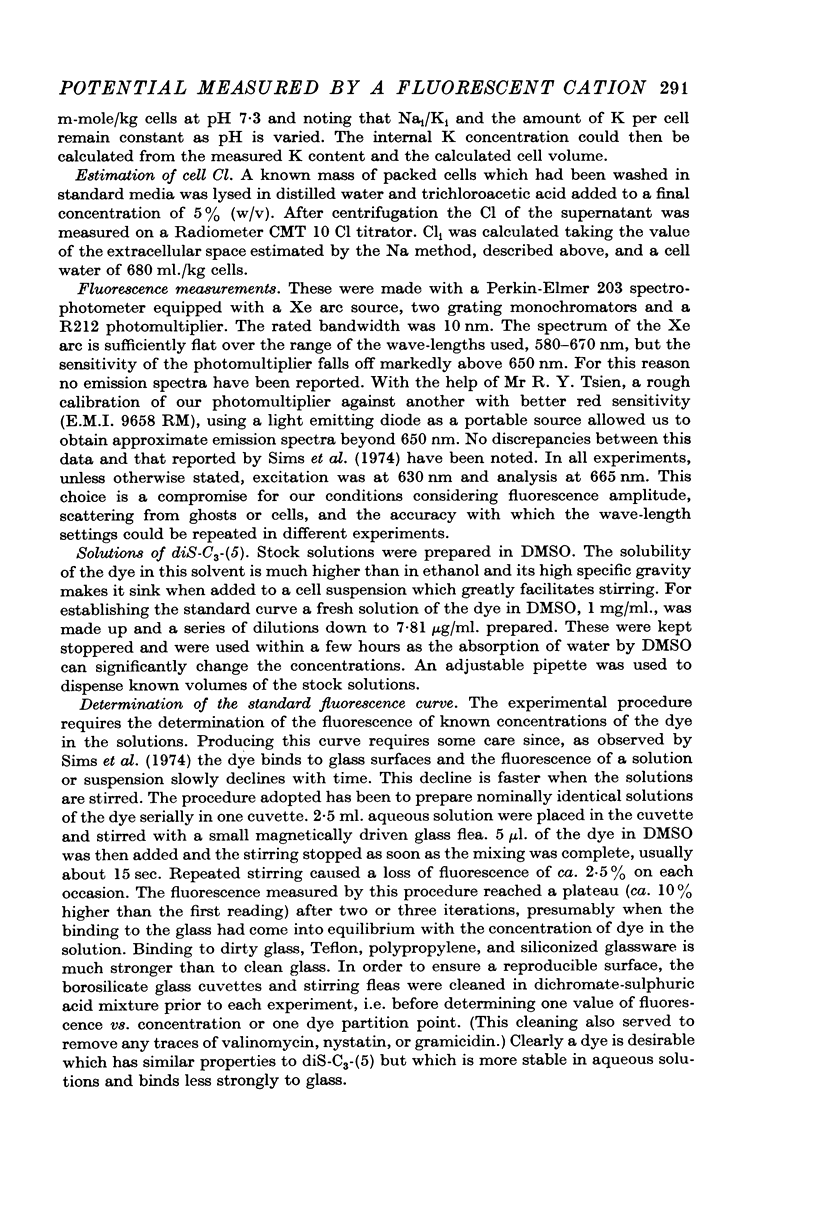
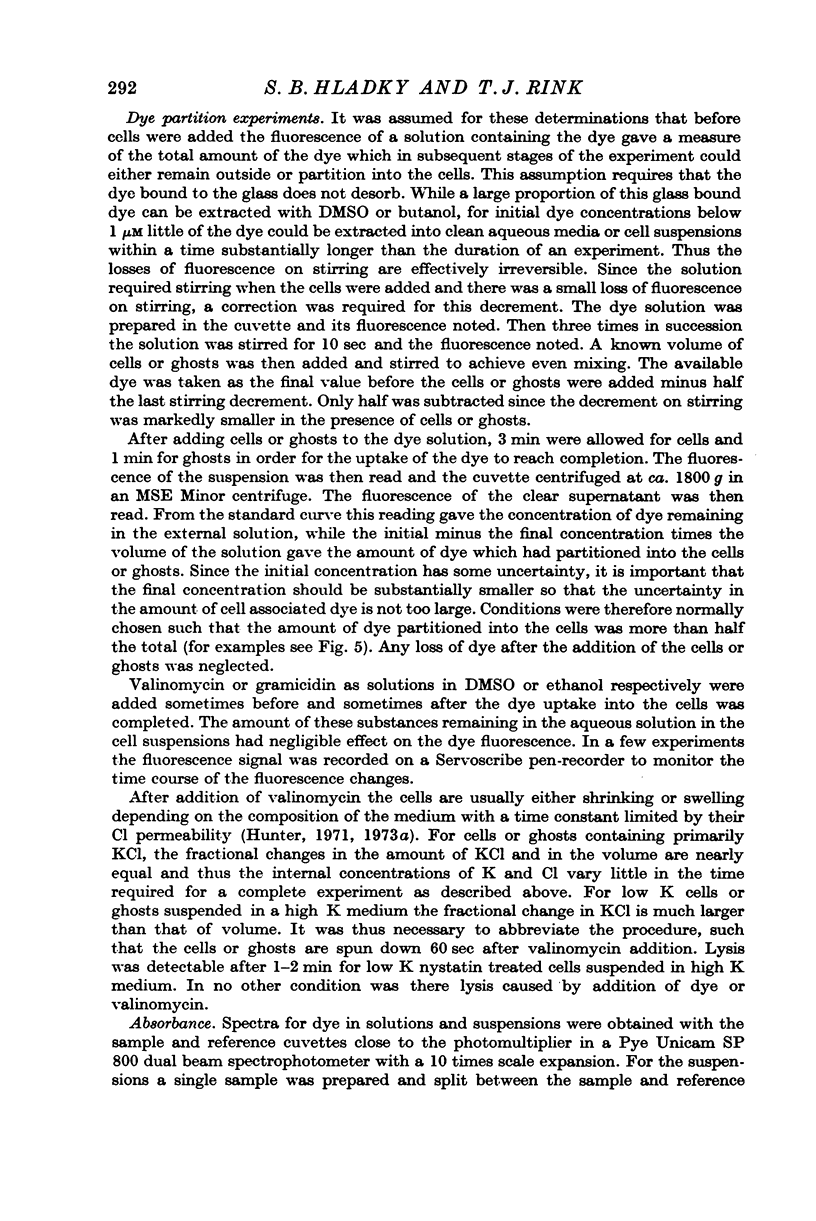
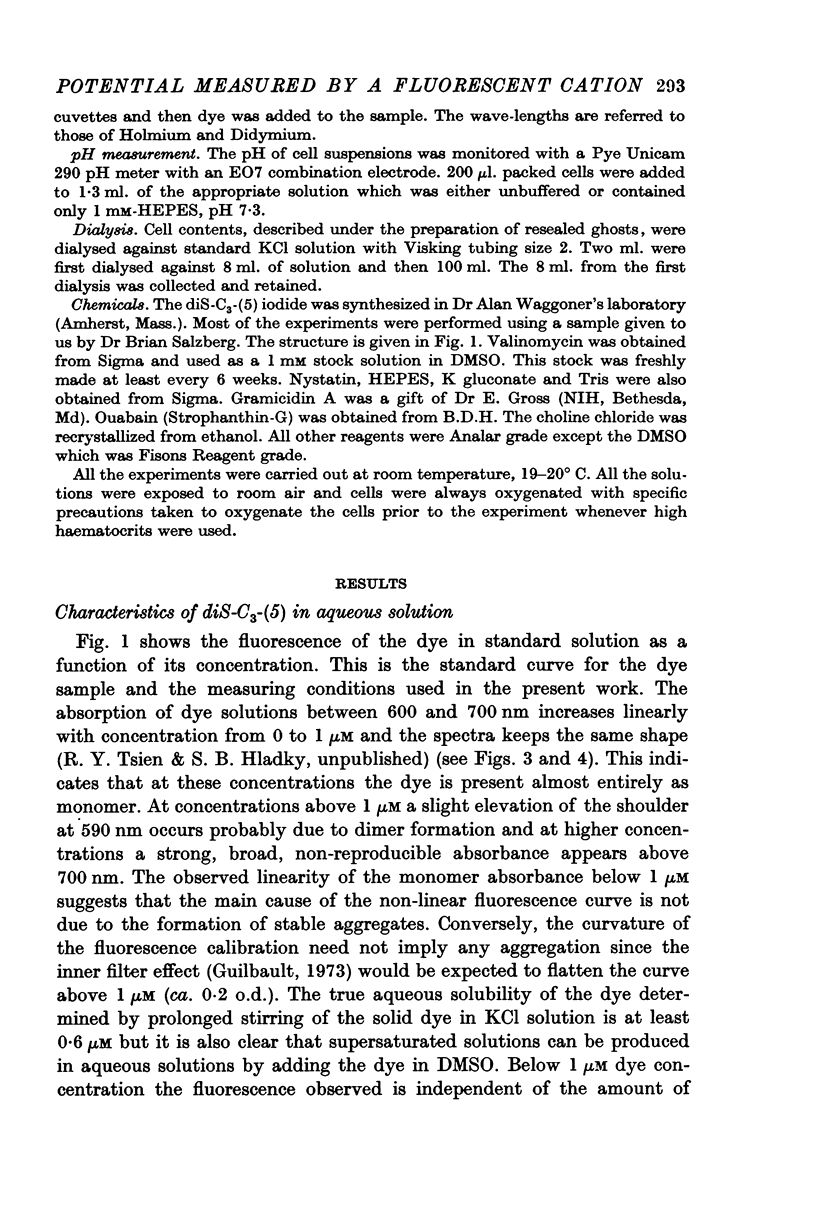
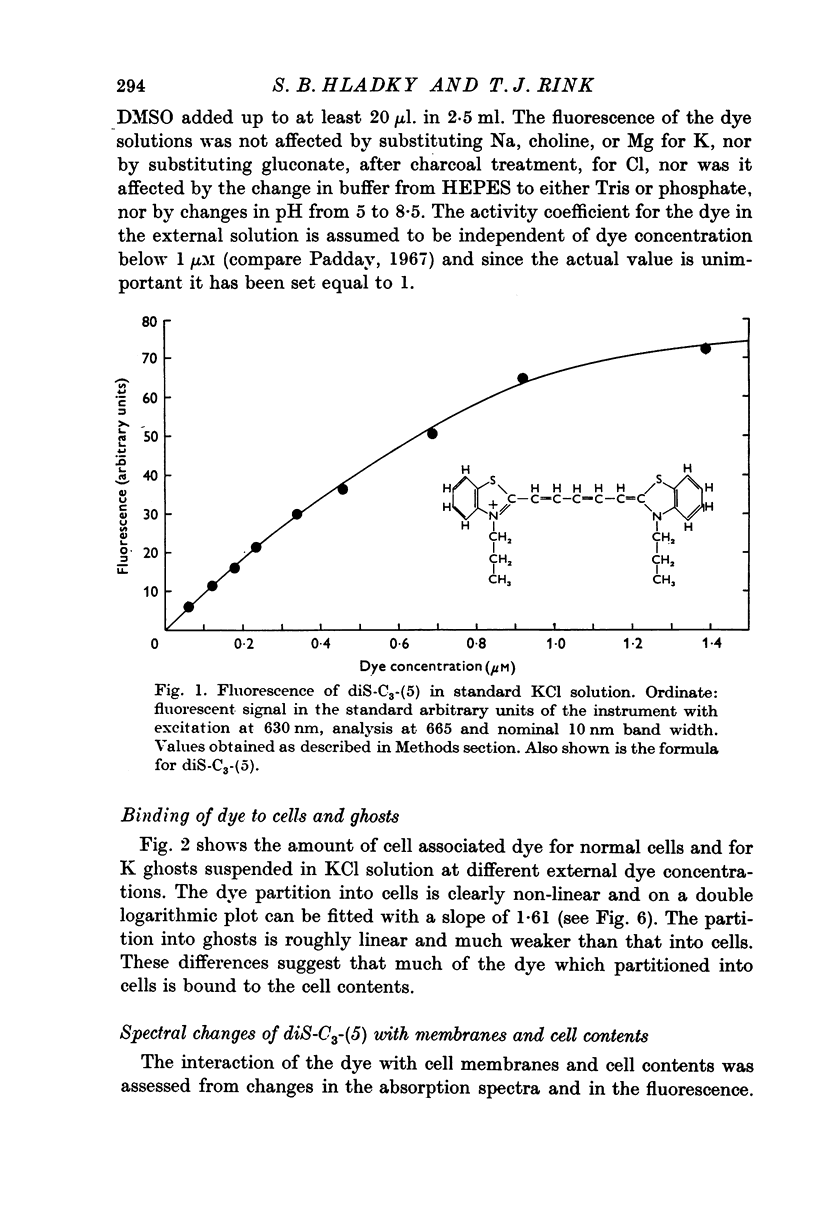
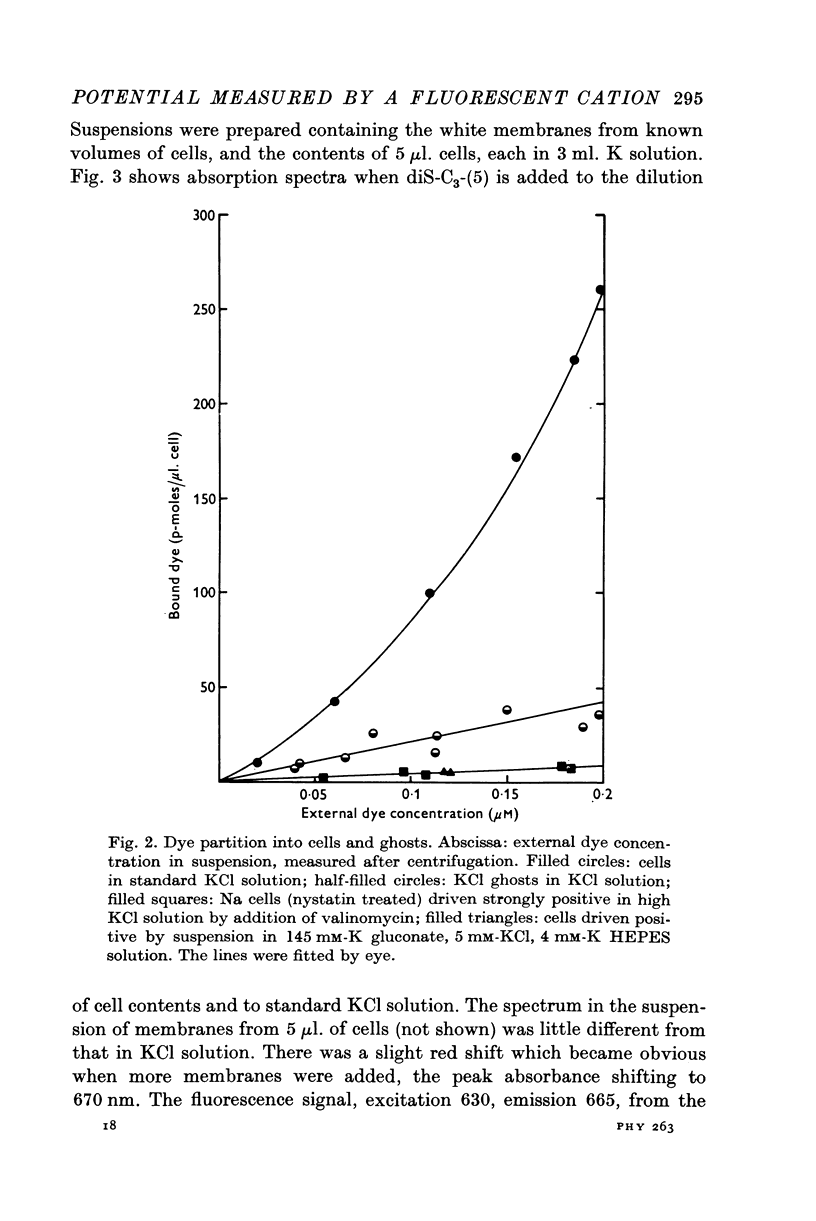
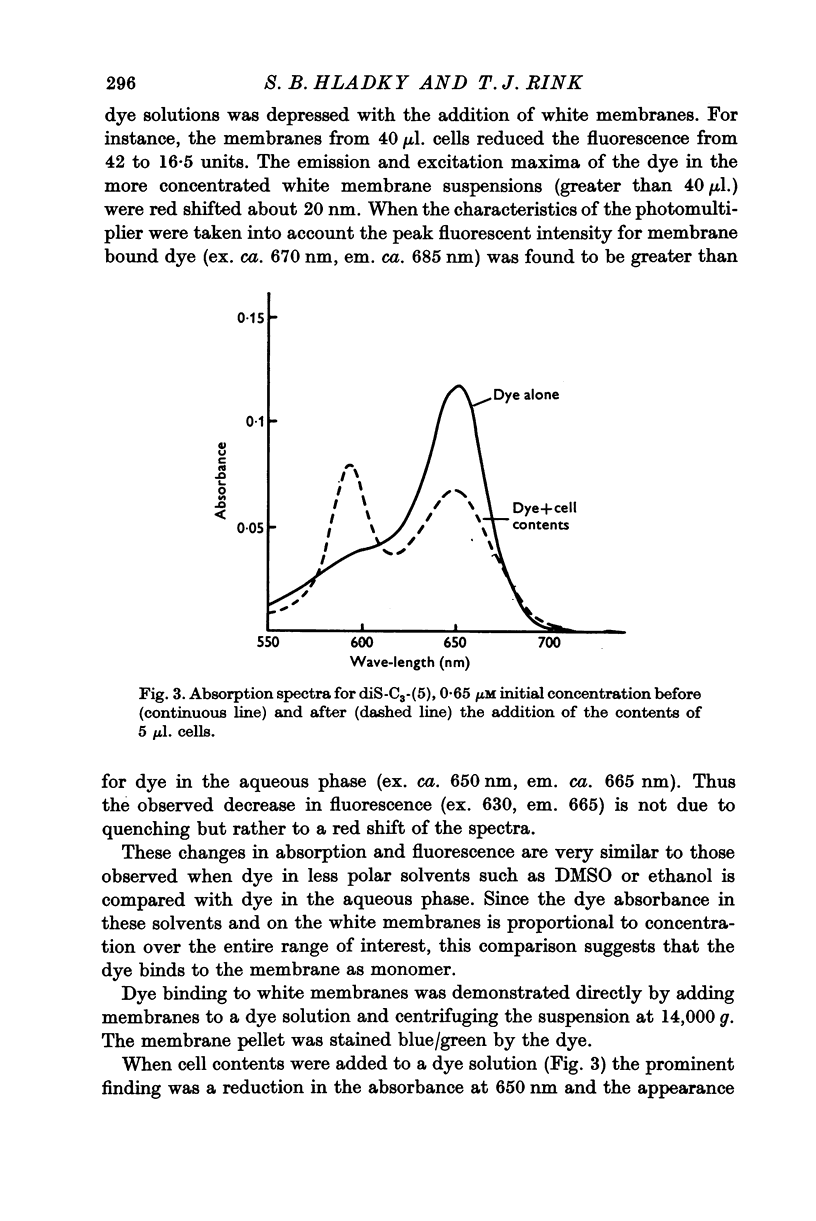
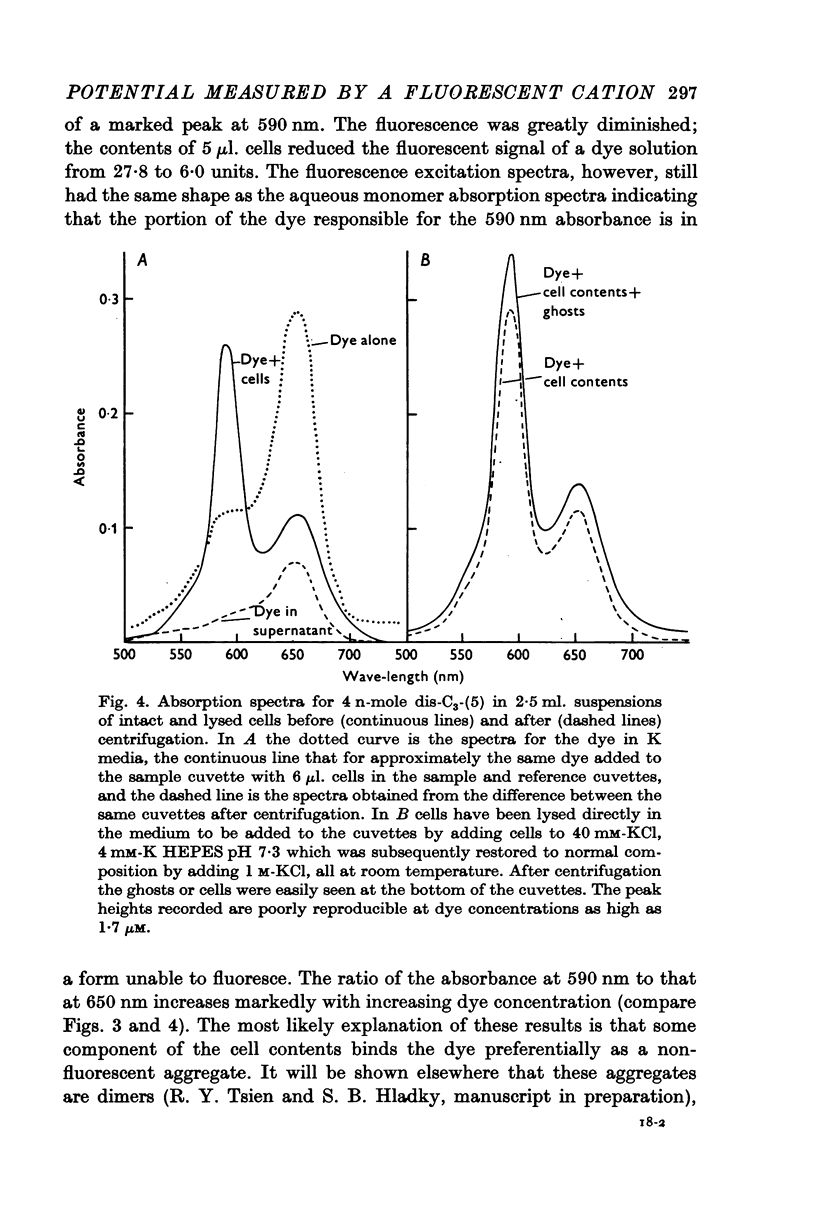
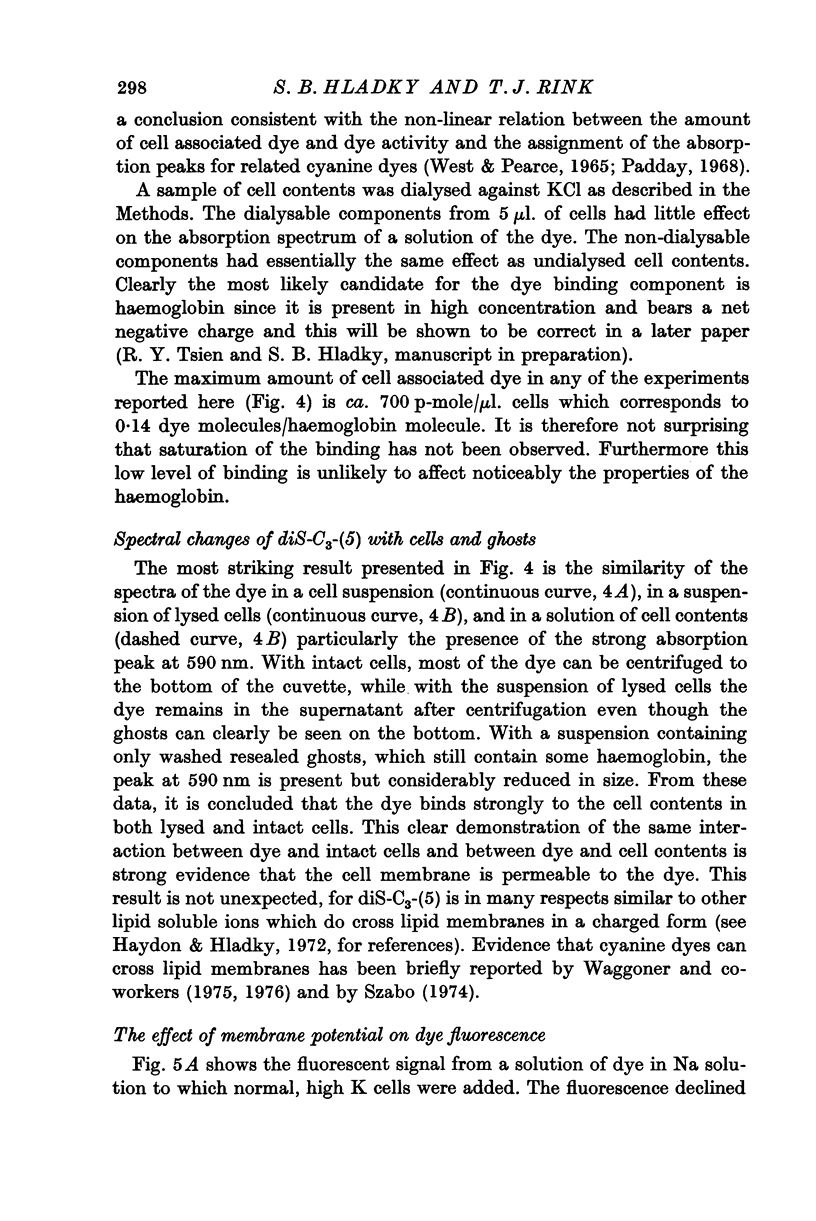
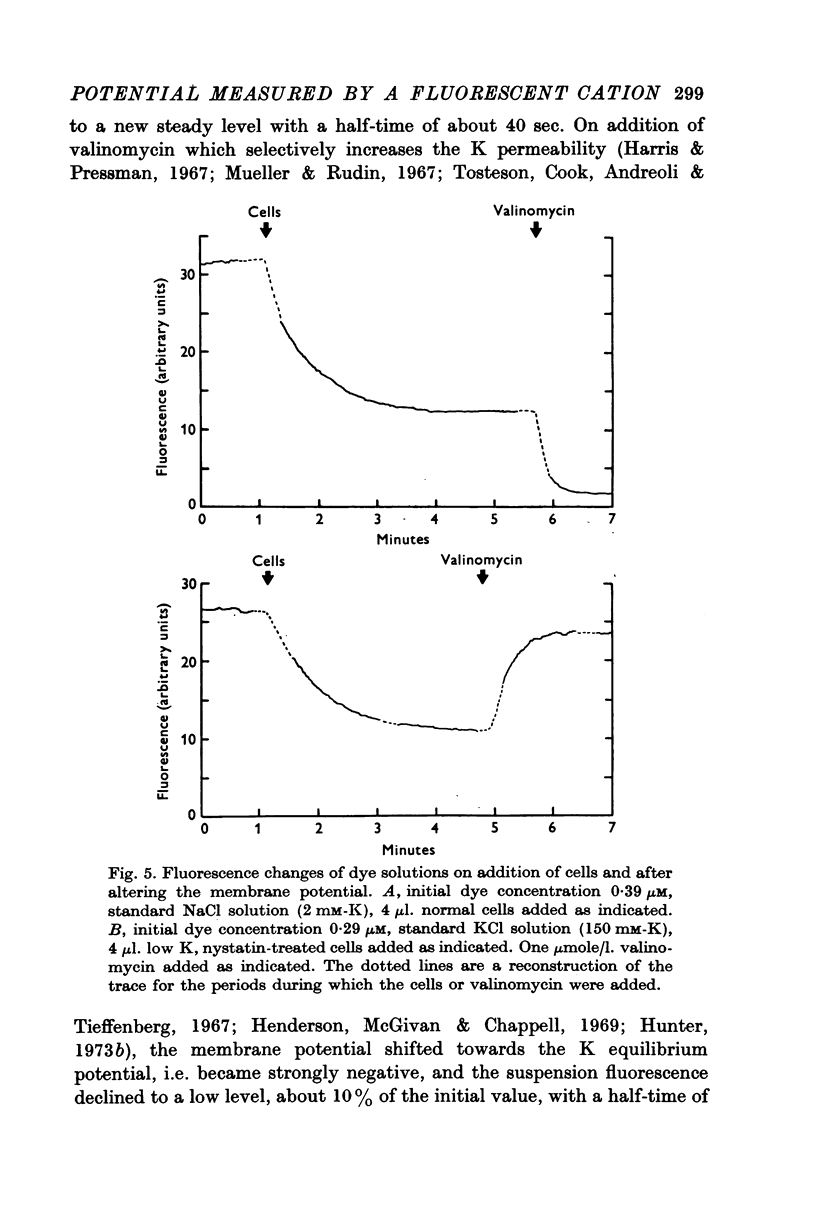
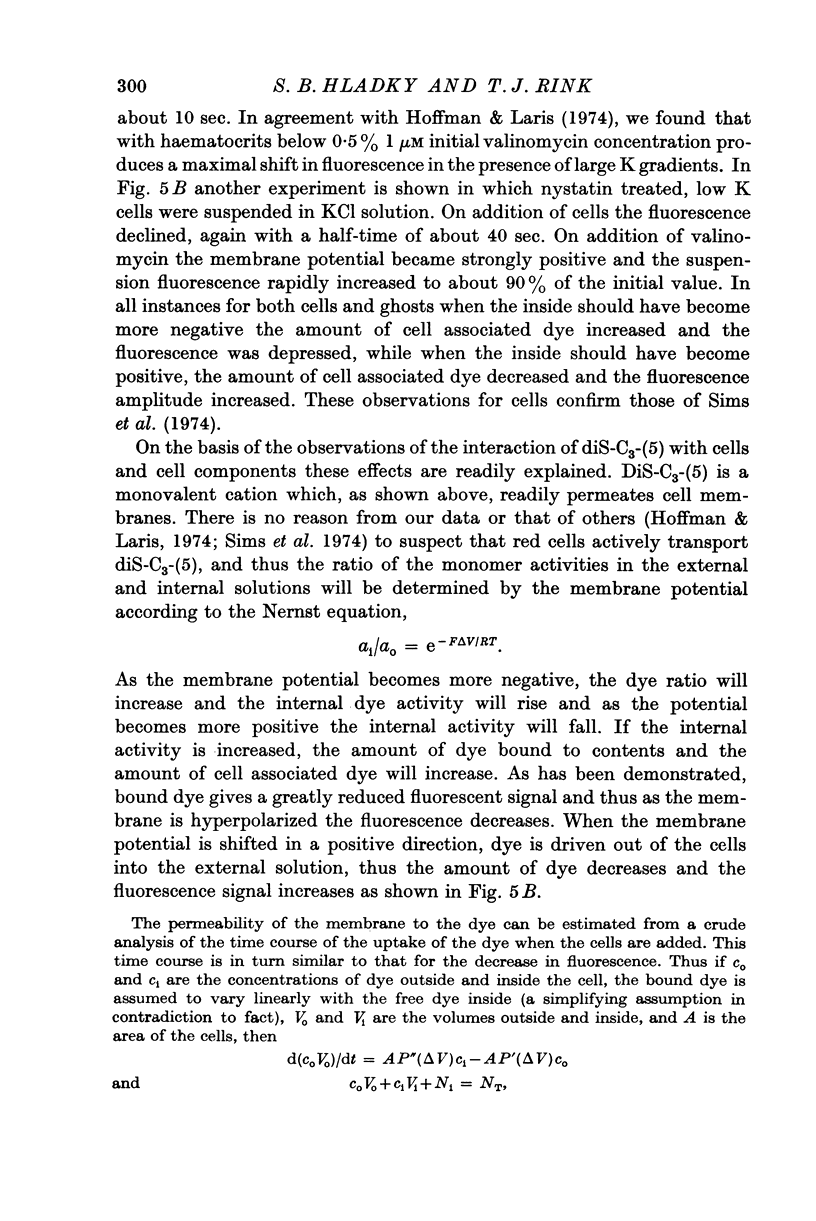
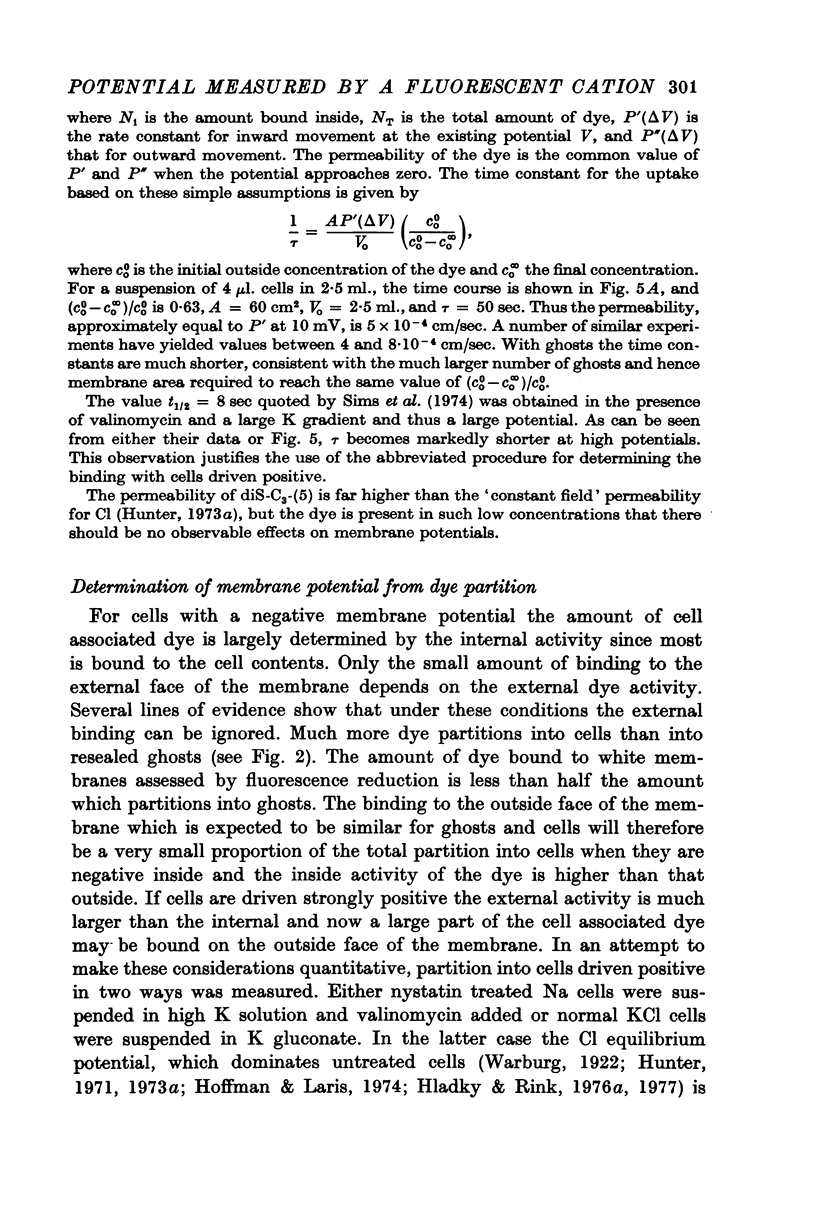
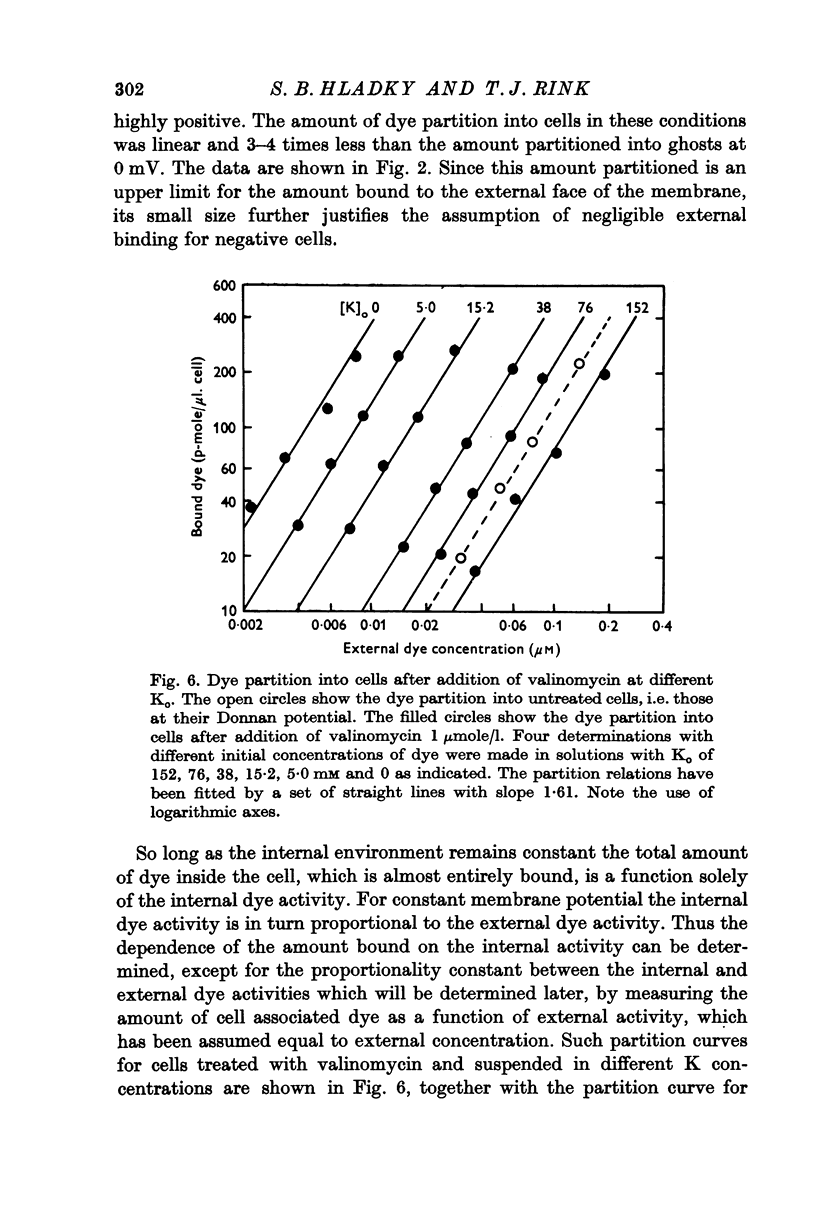
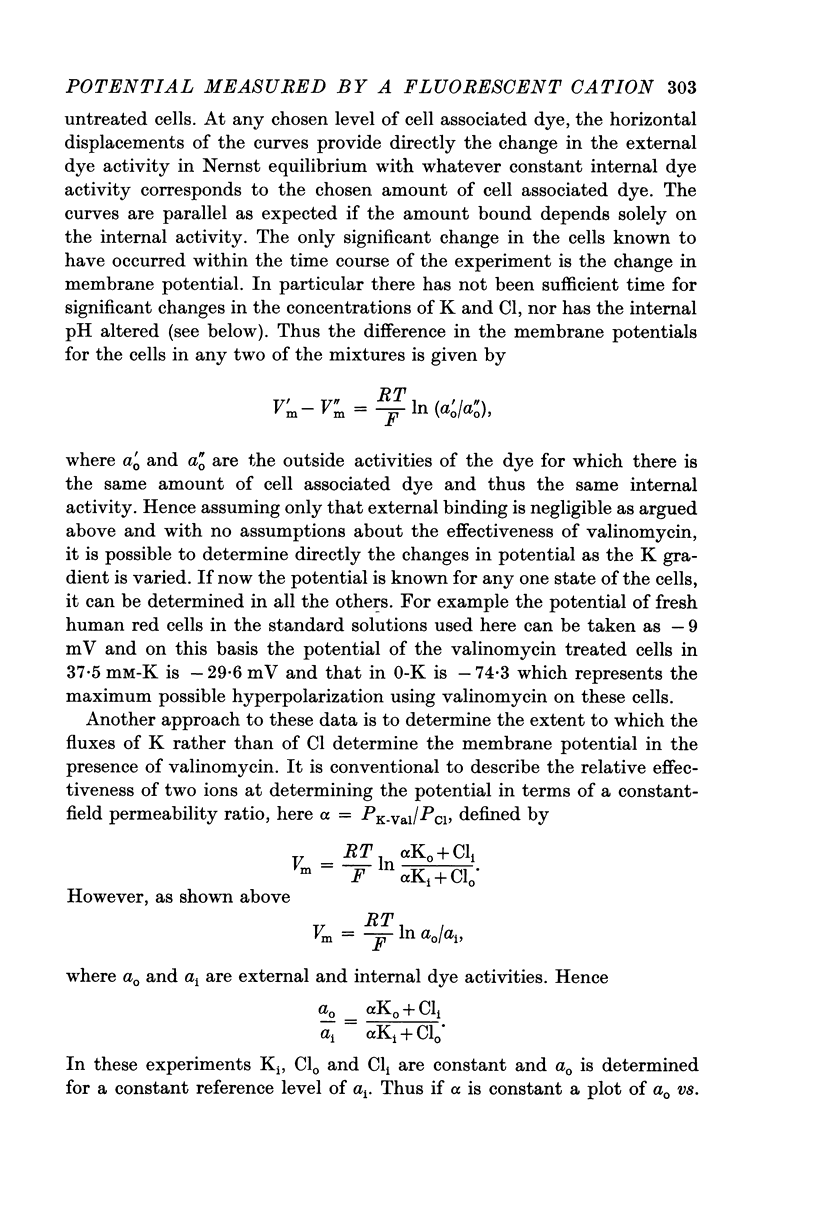
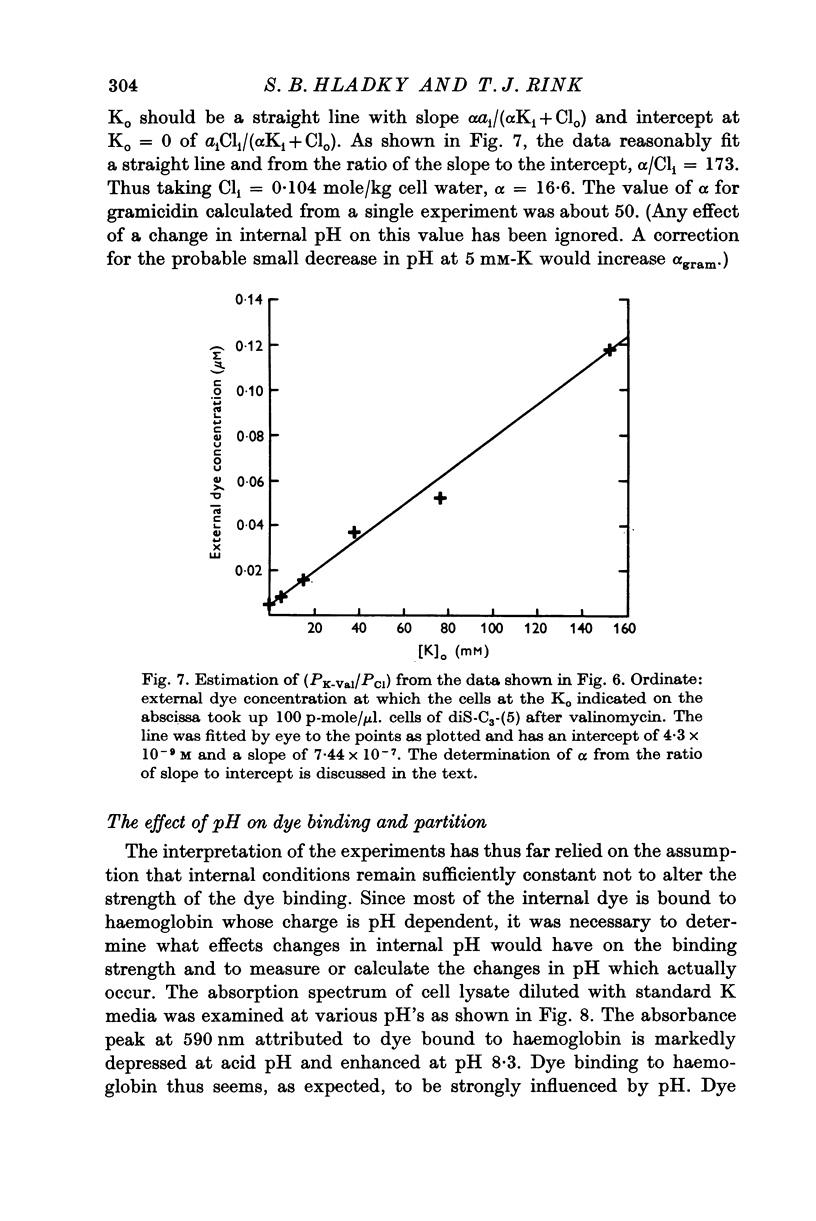
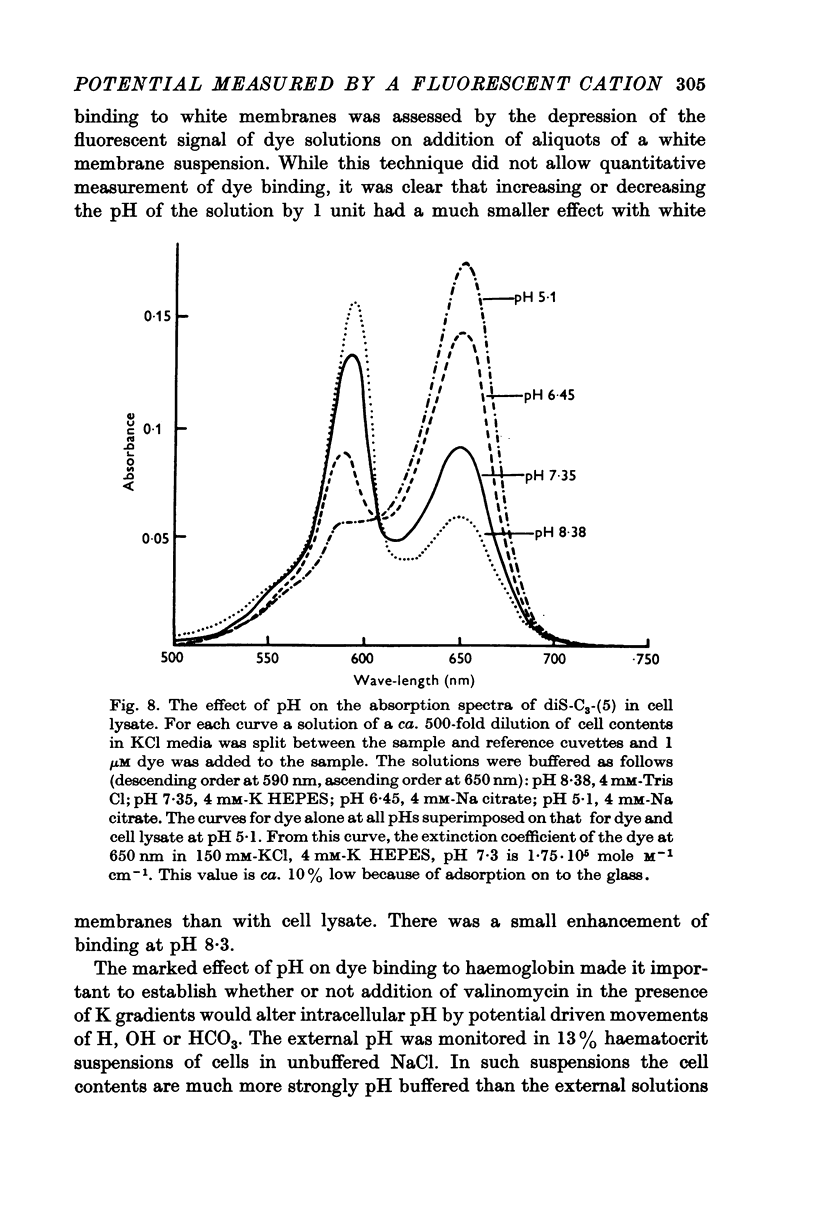
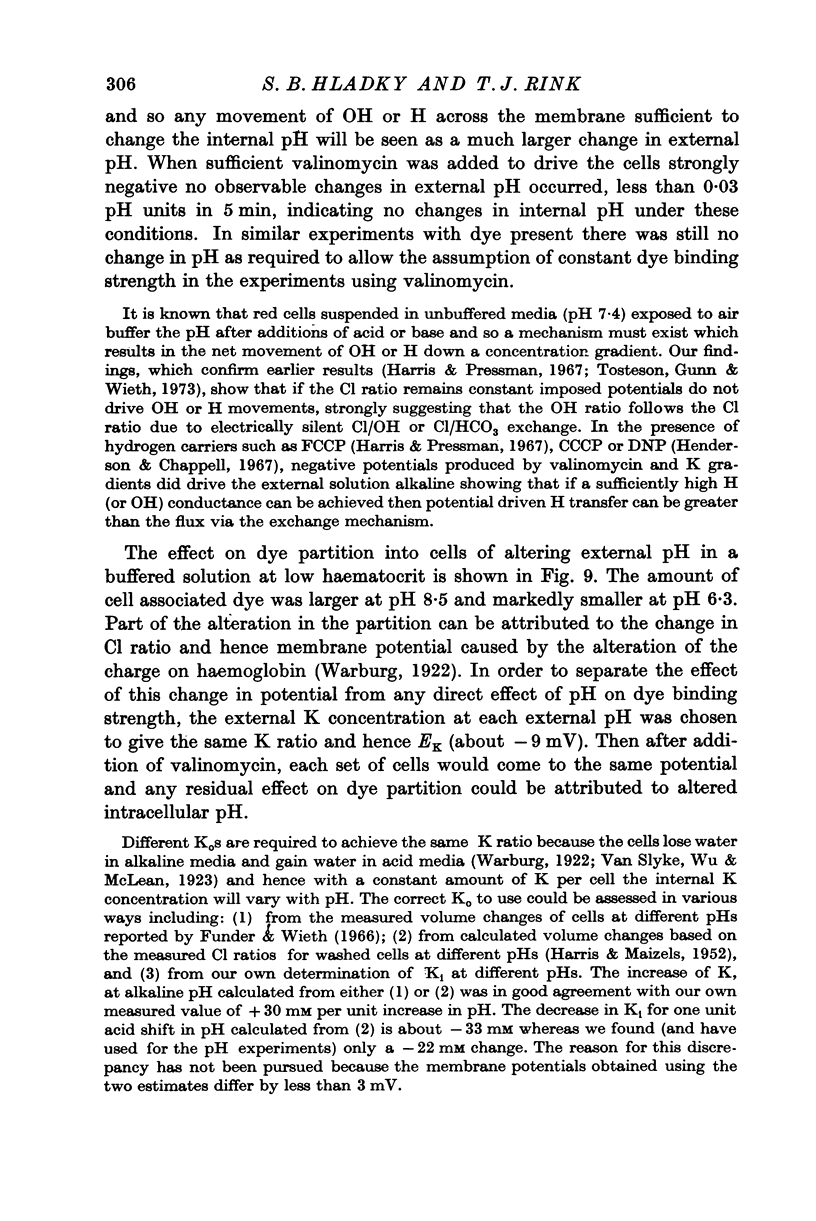
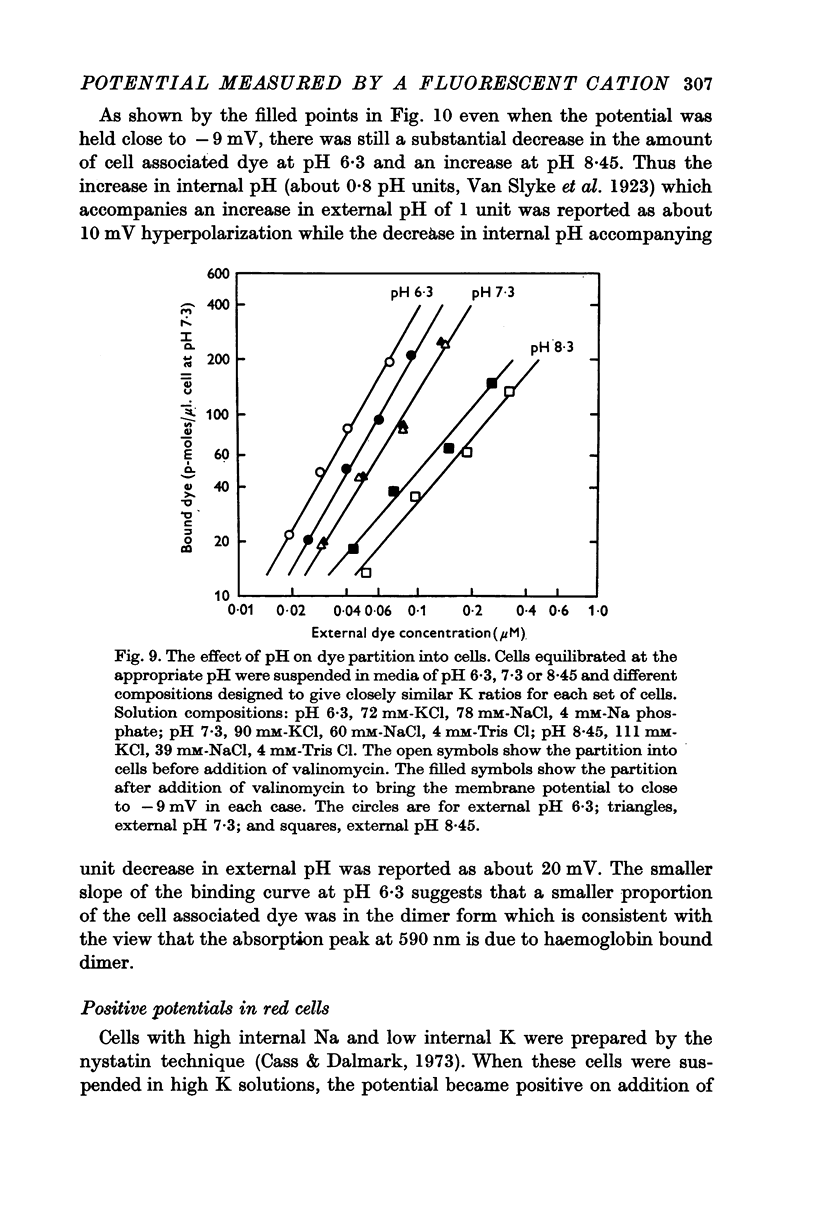
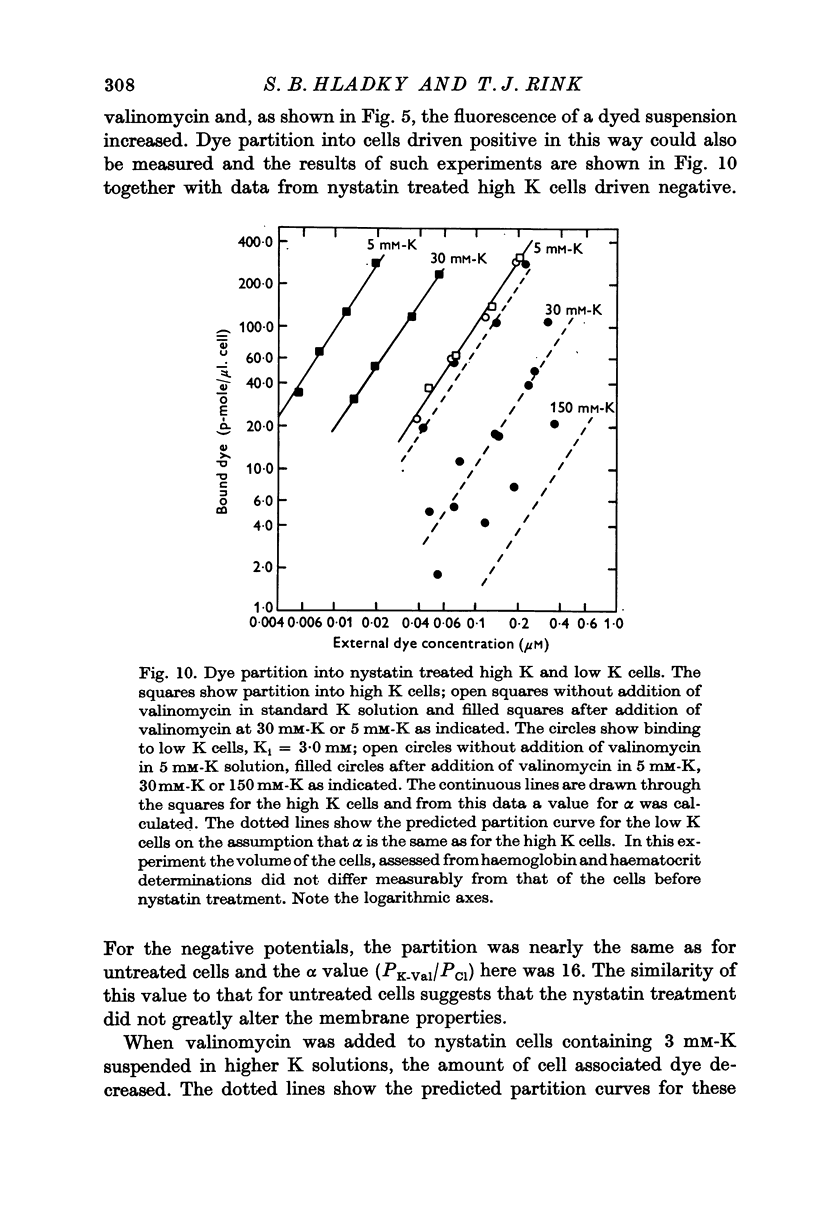
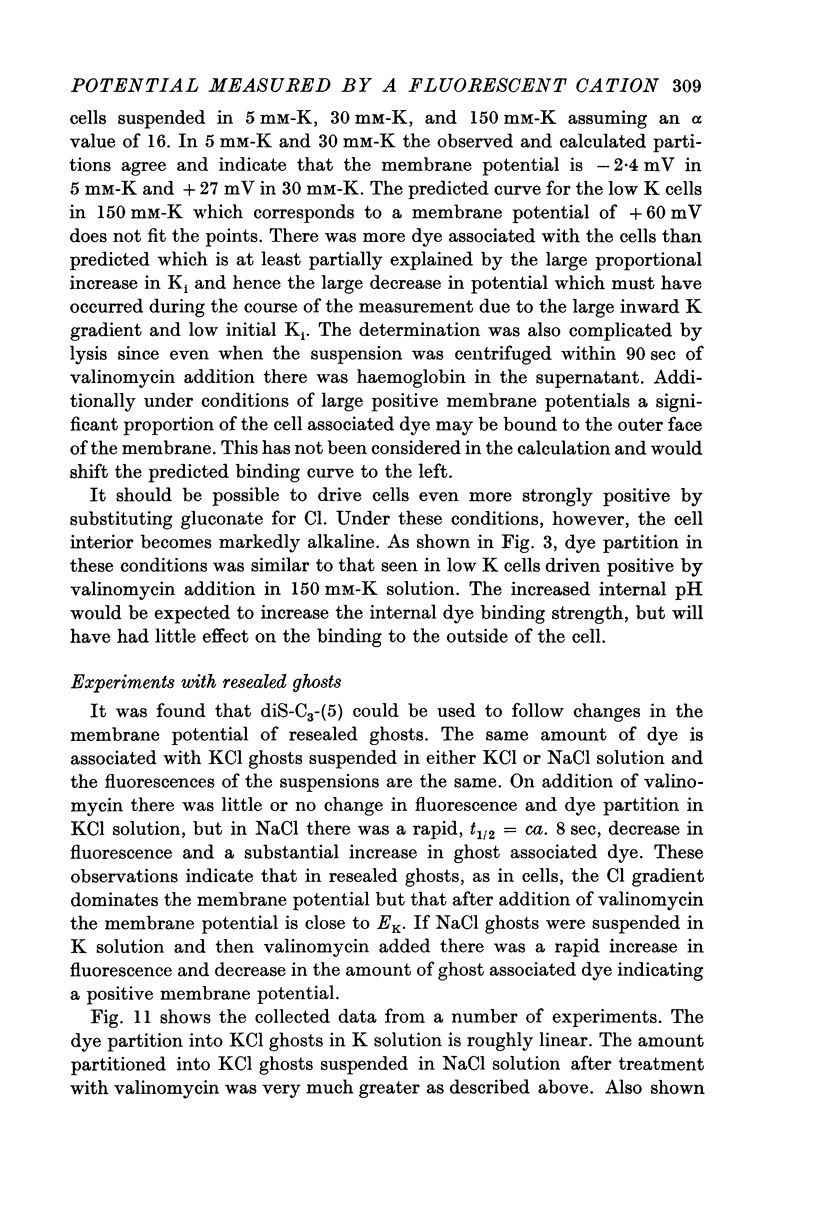
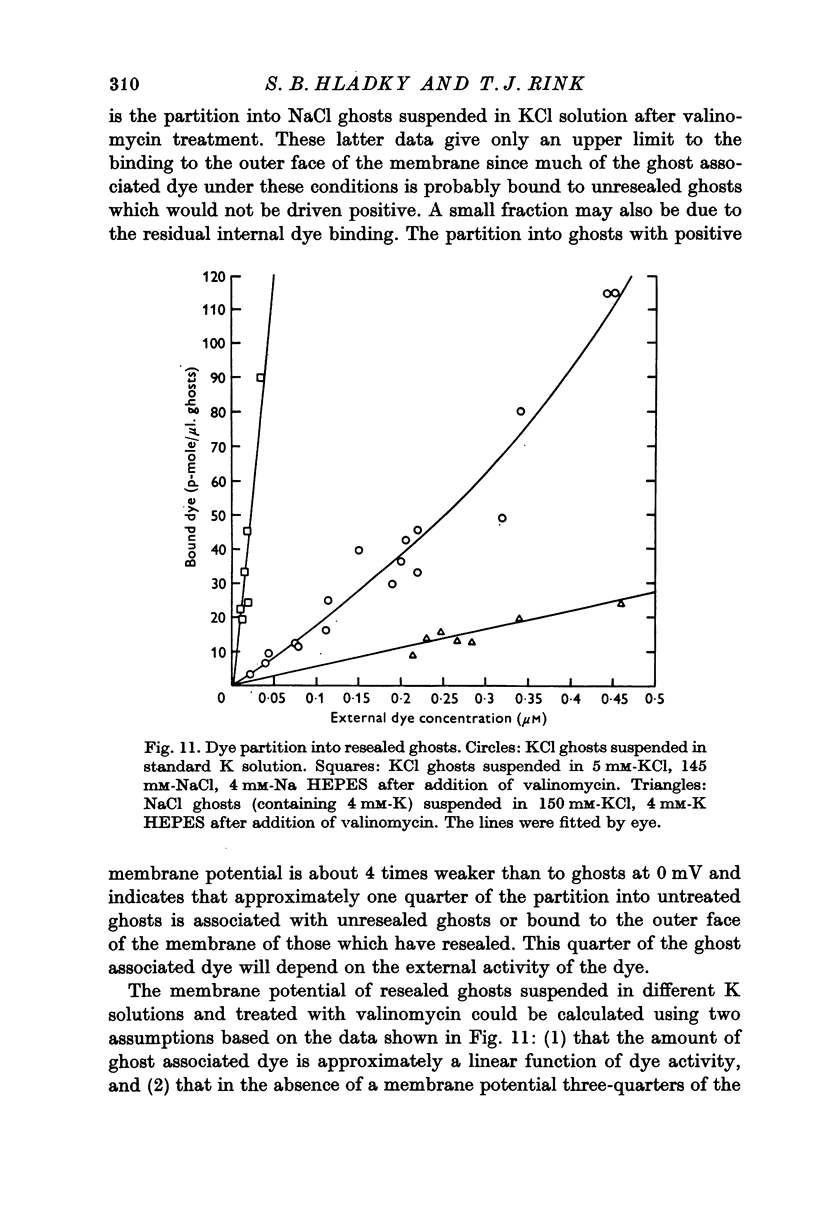
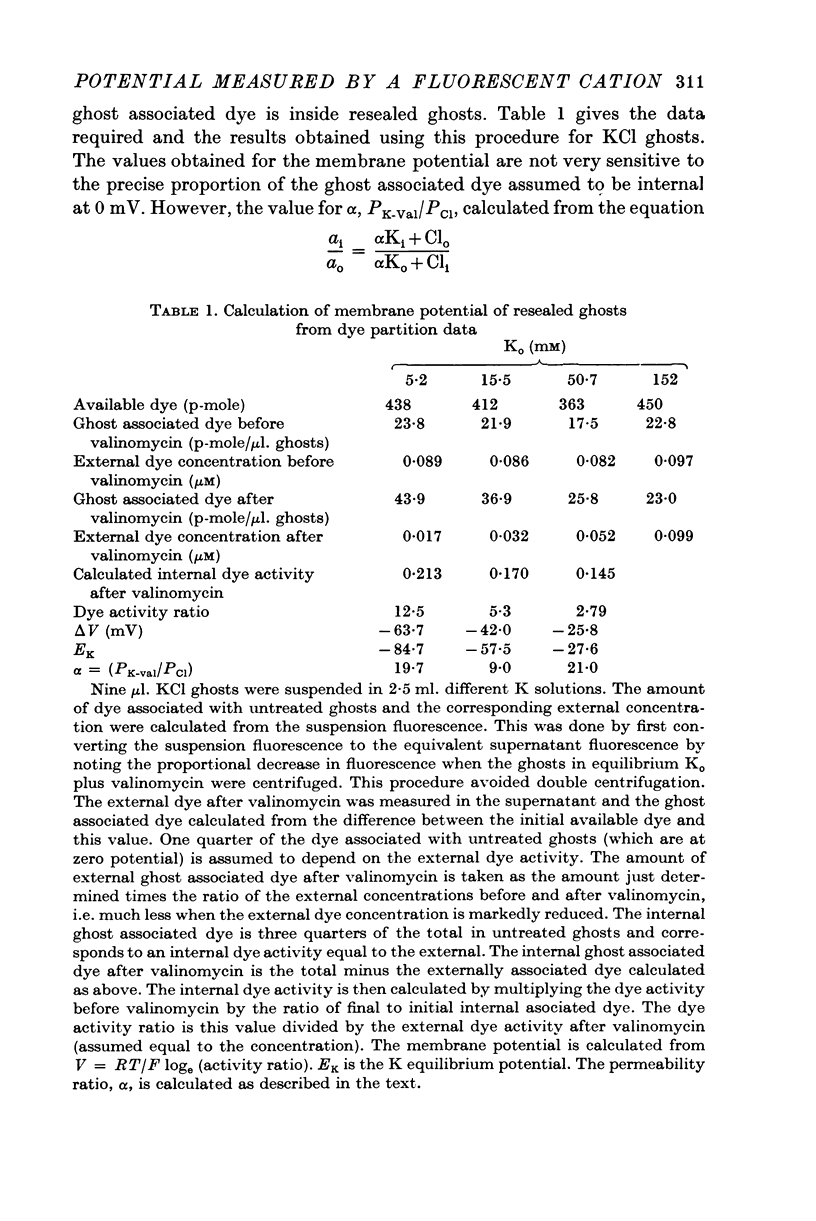
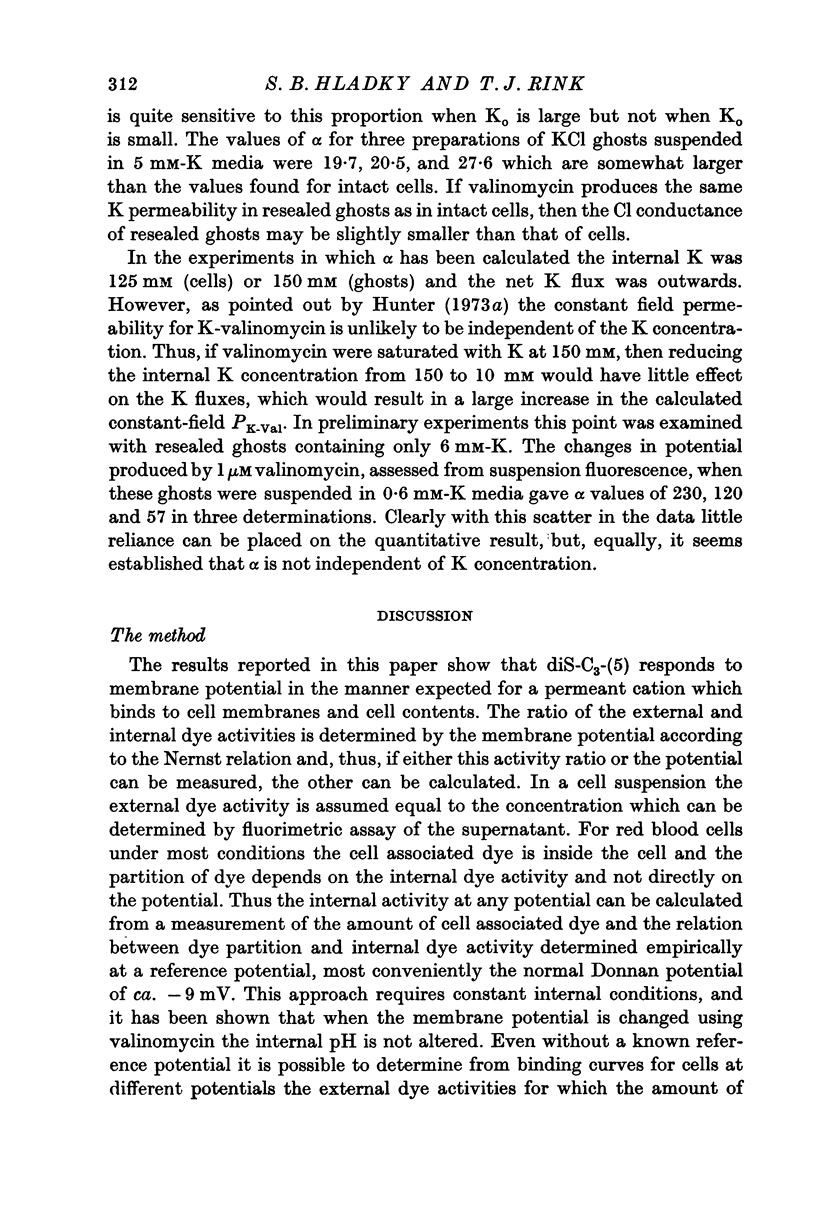
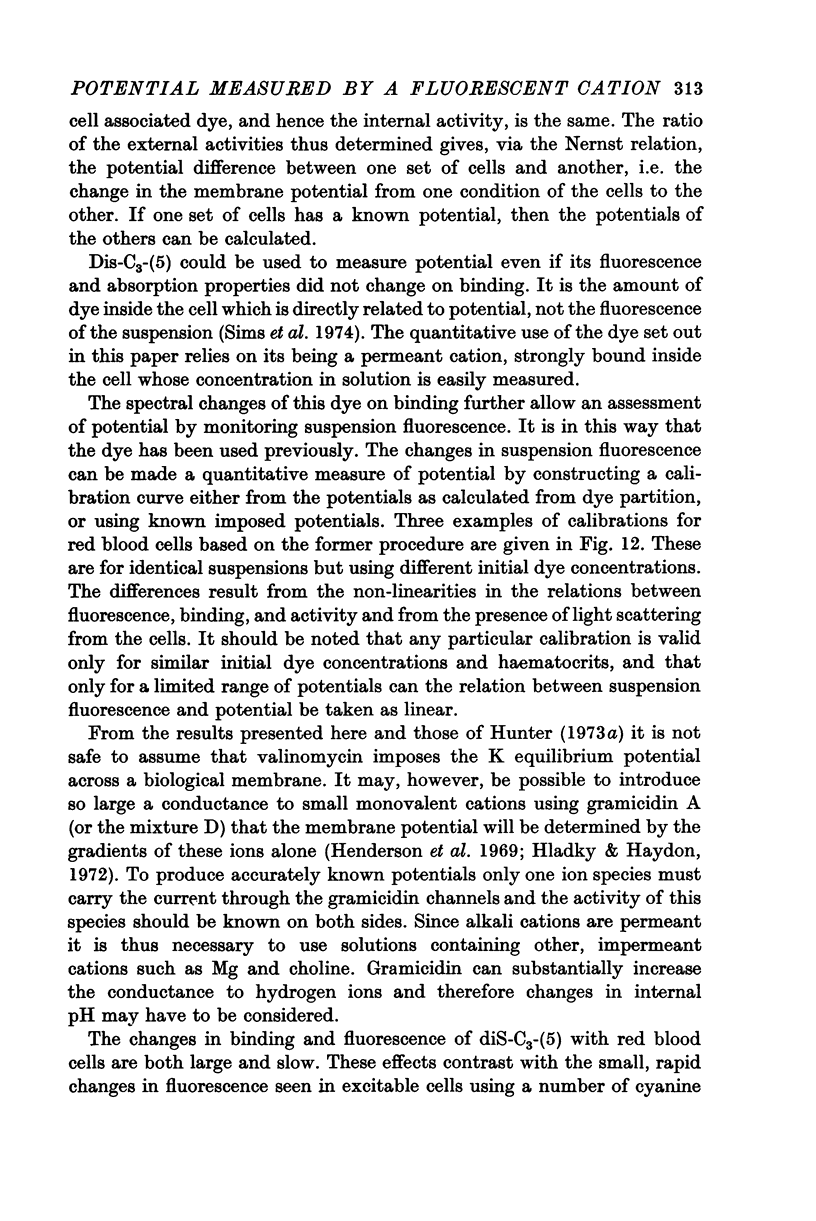
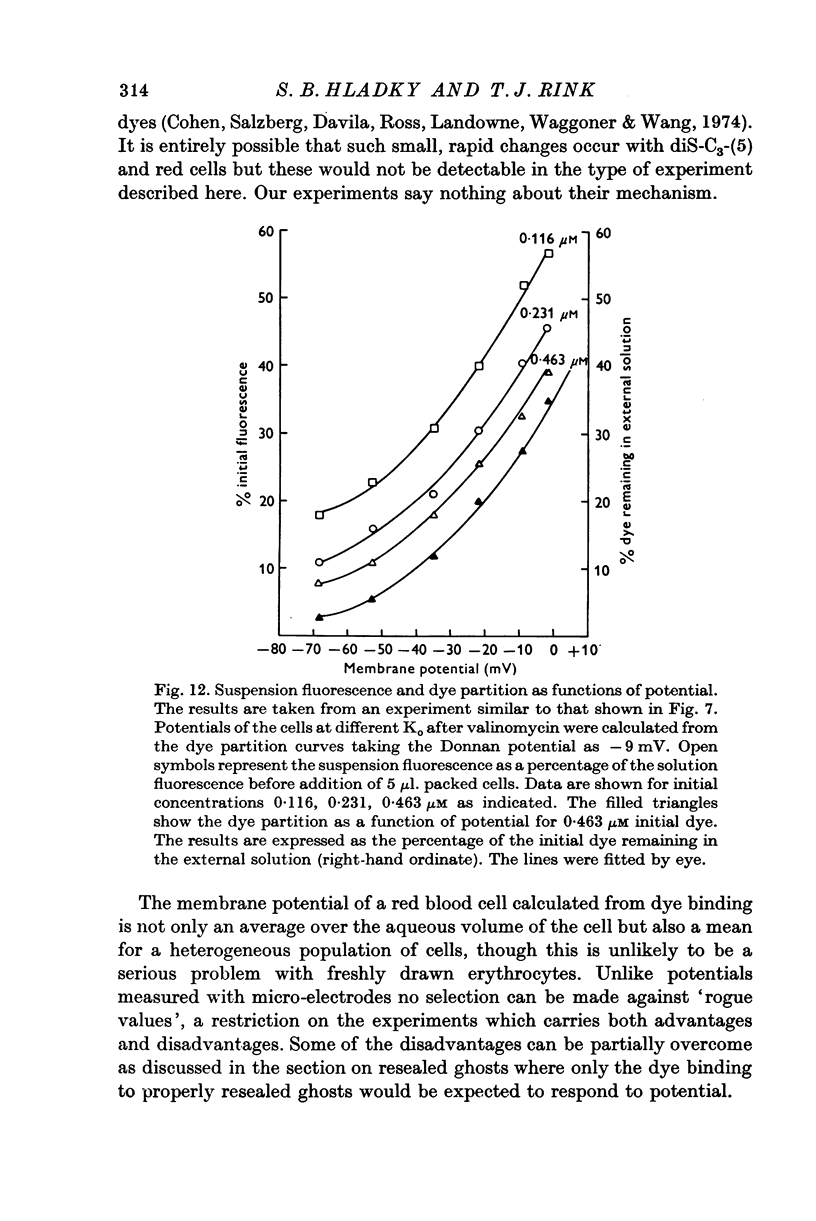
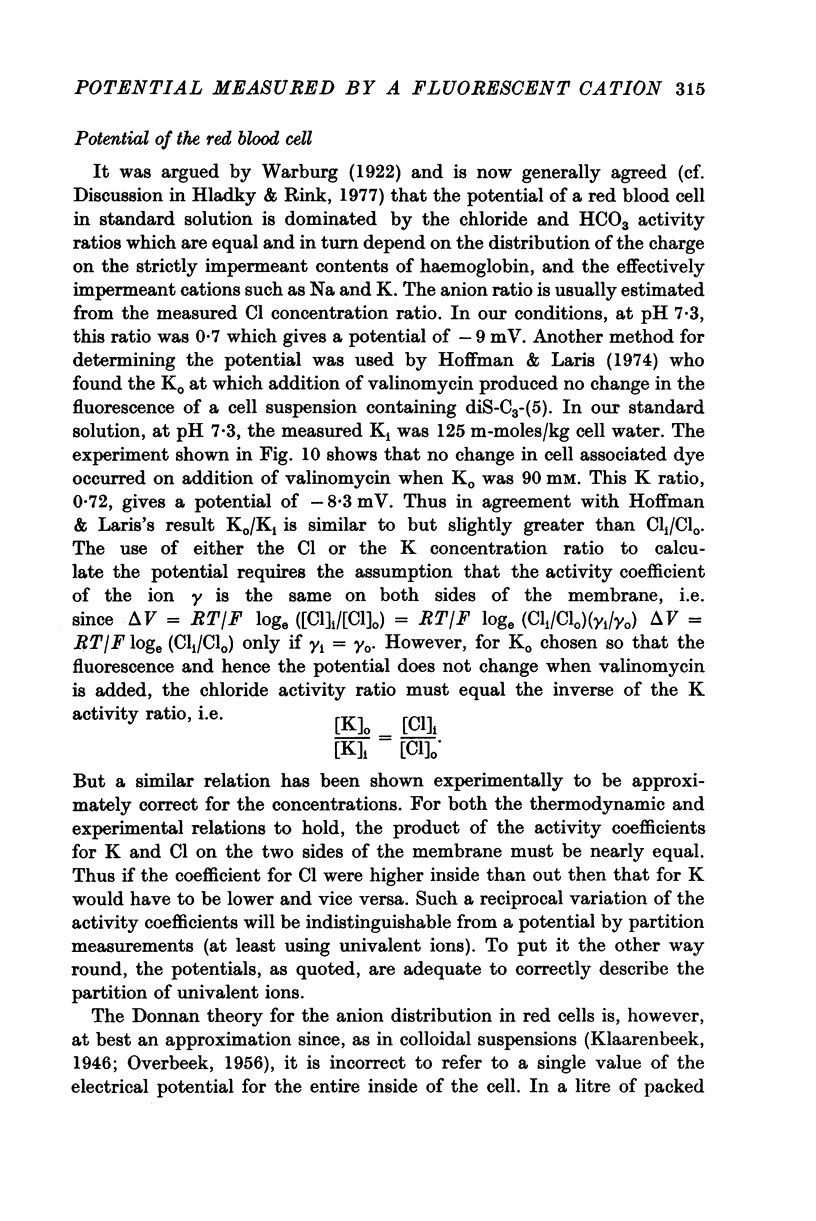
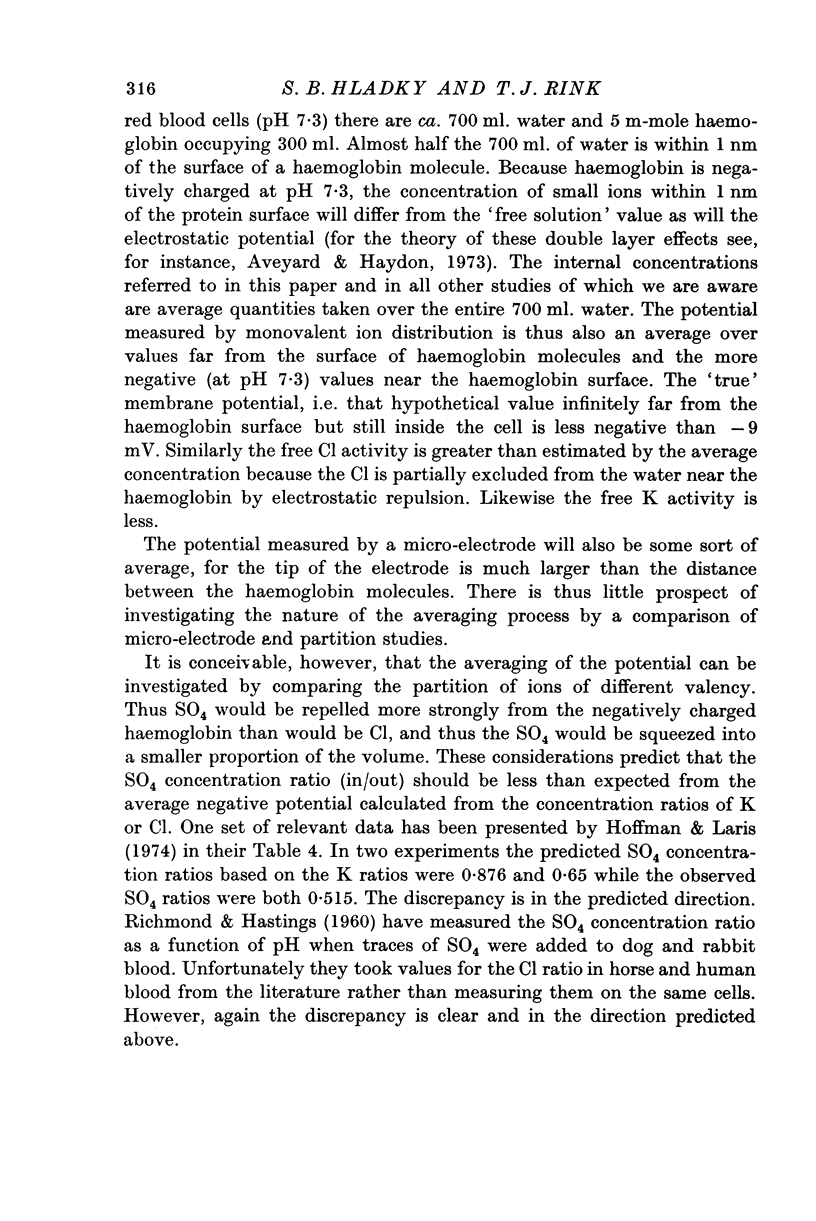
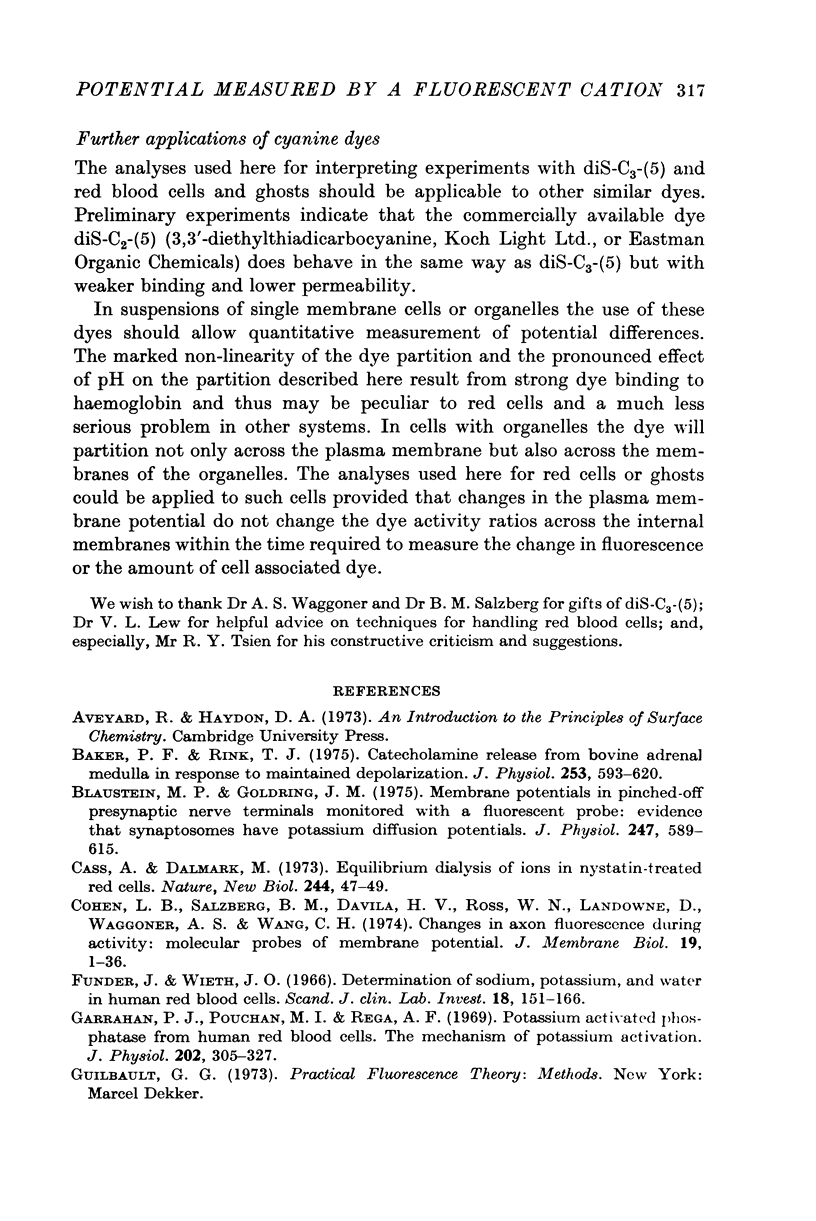
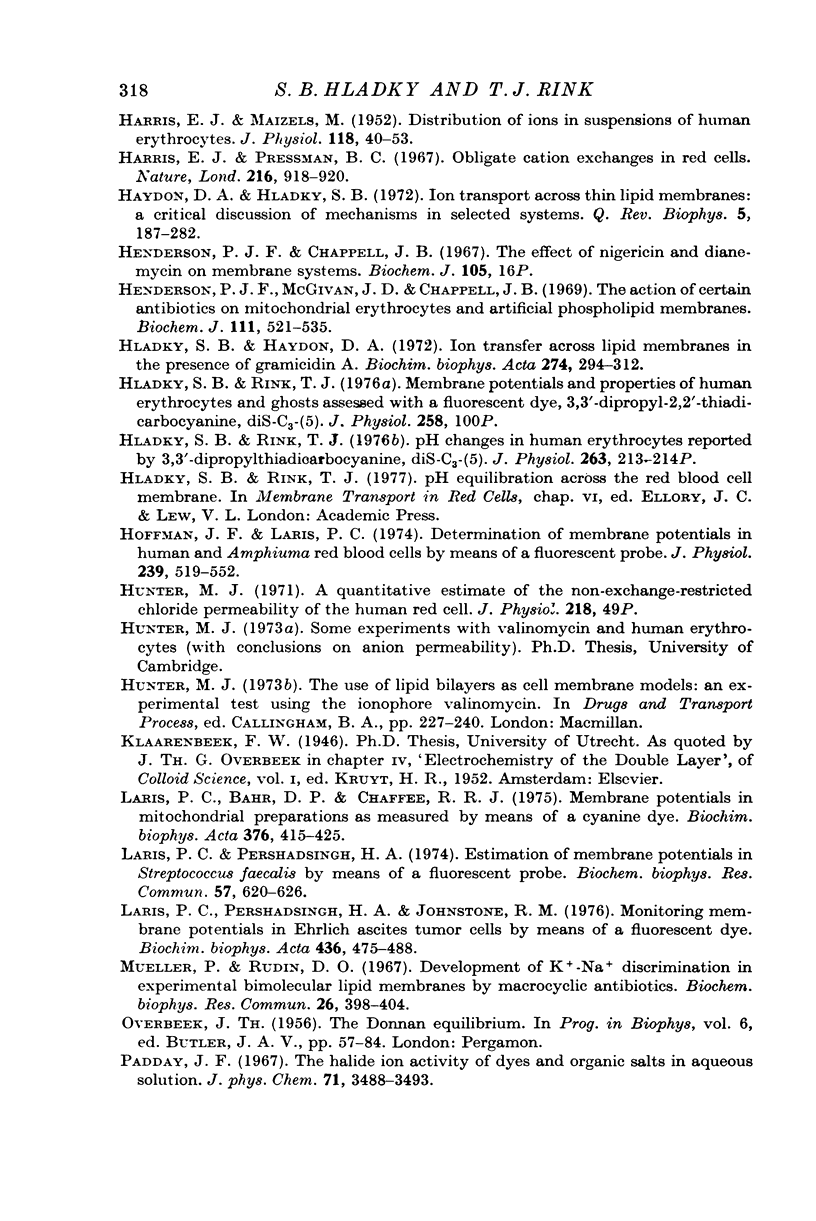
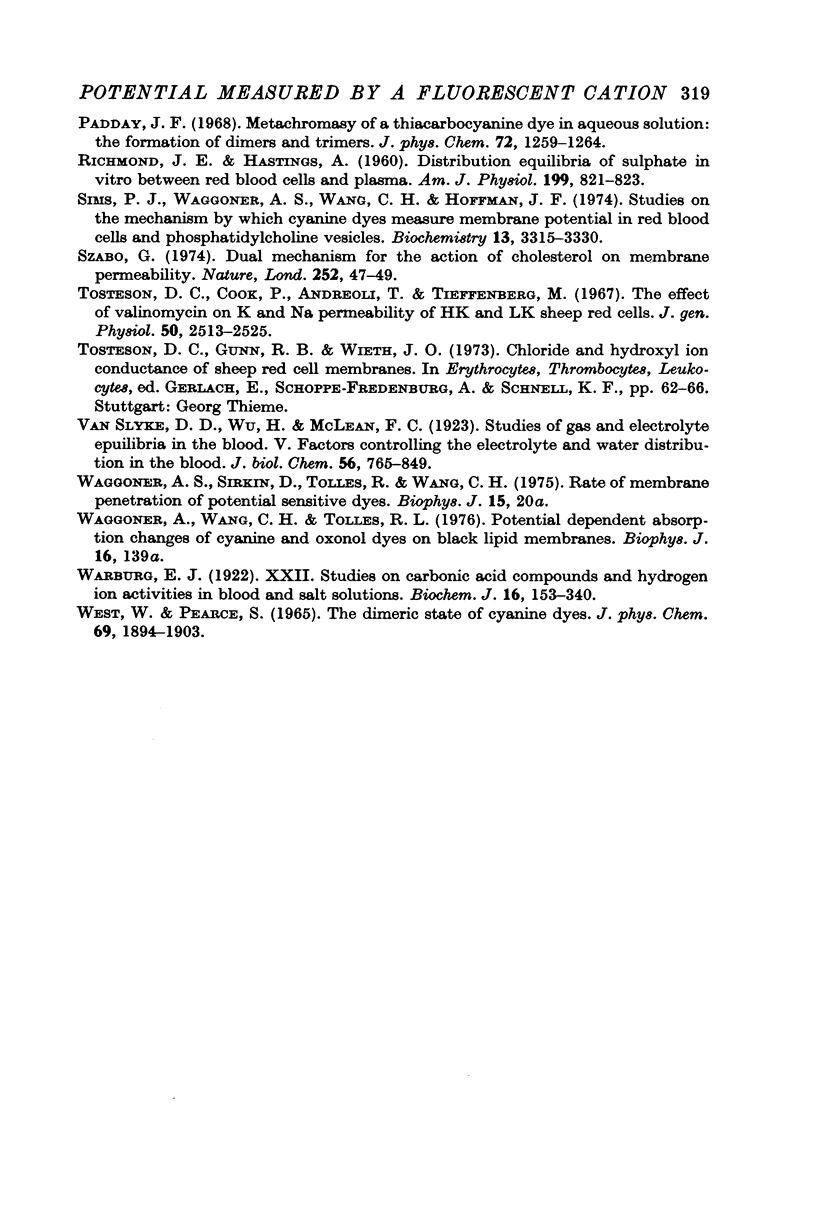
Selected References
These references are in PubMed. This may not be the complete list of references from this article.
- Baker P. F., Rink T. J. Catecholamine release from bovine adrenal medulla in response to maintained depolarization. J Physiol. 1975 Dec;253(2):593–620. doi: 10.1113/jphysiol.1975.sp011209. [DOI] [PMC free article] [PubMed] [Google Scholar]
- Blaustein M. P., Goldring J. M. Membrane potentials in pinched-off presynaptic nerve ternimals monitored with a fluorescent probe: evidence that synaptosomes have potassium diffusion potentials. J Physiol. 1975 Jun;247(3):589–615. doi: 10.1113/jphysiol.1975.sp010949. [DOI] [PMC free article] [PubMed] [Google Scholar]
- Cass A., Dalmark M. Equilibrium dialysis of ions in nystatin-treated red cells. Nat New Biol. 1973 Jul 11;244(132):47–49. doi: 10.1038/newbio244047a0. [DOI] [PubMed] [Google Scholar]
- Cohen L. B., Salzberg B. M., Davila H. V., Ross W. N., Landowne D., Waggoner A. S., Wang C. H. Changes in axon fluorescence during activity: molecular probes of membrane potential. J Membr Biol. 1974;19(1):1–36. doi: 10.1007/BF01869968. [DOI] [PubMed] [Google Scholar]
- Funder J., Wieth J. O. Determination of sodium, potassium, and water in human red blood cells. Elimination of sources of error in the development of a flame photometric method. Scand J Clin Lab Invest. 1966;18(2):151–166. doi: 10.3109/00365516609051811. [DOI] [PubMed] [Google Scholar]
- Garrahan P. J., Pouchan M. I., Rega A. F. Potassium activated phosphatase from human red blood cells. The mechanism of potassium activation. J Physiol. 1969 Jun;202(2):305–327. doi: 10.1113/jphysiol.1969.sp008813. [DOI] [PMC free article] [PubMed] [Google Scholar]
- HARRIS E. J., MAIZELS M. Distribution of ions in suspensions of human erythrocytes. J Physiol. 1952 Sep;118(1):40–53. doi: 10.1113/jphysiol.1952.sp004771. [DOI] [PMC free article] [PubMed] [Google Scholar]
- Harris E. J., Pressman B. C. Obligate cation exchanges in red cells. Nature. 1967 Dec 2;216(5118):918–920. doi: 10.1038/216918a0. [DOI] [PubMed] [Google Scholar]
- Haydon D. A., Hladky S. B. Ion transport across thin lipid membranes: a critical discussion of mechanisms in selected systems. Q Rev Biophys. 1972 May;5(2):187–282. doi: 10.1017/s0033583500000883. [DOI] [PubMed] [Google Scholar]
- Henderson P. J., McGivan J. D., Chappell J. B. The action of certain antibiotics on mitochondrial, erythrocyte and artificial phospholipid membranes. The role of induced proton permeability. Biochem J. 1969 Feb;111(4):521–535. doi: 10.1042/bj1110521. [DOI] [PMC free article] [PubMed] [Google Scholar]
- Hladky S. B., Haydon D. A. Ion transfer across lipid membranes in the presence of gramicidin A. I. Studies of the unit conductance channel. Biochim Biophys Acta. 1972 Aug 9;274(2):294–312. doi: 10.1016/0005-2736(72)90178-2. [DOI] [PubMed] [Google Scholar]
- Hladky S. B., Rink T. J. pH Changes in human erythrocytes reported by 3,3' dipropylthia-dicarbocyanine, diS-C3-(5) [proceedings]. J Physiol. 1976 Dec;263(1):213P–214P. [PubMed] [Google Scholar]
- Hoffman J. F., Laris P. C. Determination of membrane potentials in human and Amphiuma red blood cells by means of fluorescent probe. J Physiol. 1974 Jun;239(3):519–552. doi: 10.1113/jphysiol.1974.sp010581. [DOI] [PMC free article] [PubMed] [Google Scholar]
- Laris P. C., Bahr D. P., Chaffee R. R. Membrane potentials in mitochondrial preparations as measured by means of a cyanine dye. Biochim Biophys Acta. 1975 Mar 20;376(3):415–425. doi: 10.1016/0005-2728(75)90163-2. [DOI] [PubMed] [Google Scholar]
- Laris P. C., Pershadsingh H. A. Estimations of membrane potentials in Streptococcus faecalis by means of a fluorescent probe. Biochem Biophys Res Commun. 1974 Apr 8;57(3):620–626. doi: 10.1016/0006-291x(74)90591-9. [DOI] [PubMed] [Google Scholar]
- Laris P. C., Pershadsingh H. A., Johnstone R. M. Monitoring membrane potentials in Ehrlich ascites tumor cells by means of a fluorescent dye. Biochim Biophys Acta. 1976 Jun 17;436(2):475–488. doi: 10.1016/0005-2736(76)90209-1. [DOI] [PubMed] [Google Scholar]
- Mueller P., Rudin D. O. Development of K+-Na+ discrimination in experimental bimolecular lipid membranes by macrocyclic antibiotics. Biochem Biophys Res Commun. 1967 Feb 21;26(4):398–404. doi: 10.1016/0006-291x(67)90559-1. [DOI] [PubMed] [Google Scholar]
- RICHMOND J. E., HASTINGS A. B. Distribution equilibria of sulfate in vitro between red blood cells and plasma. Am J Physiol. 1960 Nov;199:821–823. doi: 10.1152/ajplegacy.1960.199.5.821. [DOI] [PubMed] [Google Scholar]
- Sims P. J., Waggoner A. S., Wang C. H., Hoffman J. F. Studies on the mechanism by which cyanine dyes measure membrane potential in red blood cells and phosphatidylcholine vesicles. Biochemistry. 1974 Jul 30;13(16):3315–3330. doi: 10.1021/bi00713a022. [DOI] [PubMed] [Google Scholar]
- Tosteson D. C., Cook P., Andreoli T., Tieffenberg M. The effect of valinomycin on potassium and sodium permeability of HK and LK sheep red cells. J Gen Physiol. 1967 Dec;50(11):2513–2525. doi: 10.1085/jgp.50.11.2513. [DOI] [PMC free article] [PubMed] [Google Scholar]
- Warburg E. J. Studies on Carbonic Acid Compounds and Hydrogen Ion Activities in Blood and Salt Solutions. A Contribution to the Theory of the Equation of Lawrence J. Henderson and K. A. Hasselbach: CHAP. XII. Biochem J. 1922;16(2):307–340. doi: 10.1042/bj0160307. [DOI] [PMC free article] [PubMed] [Google Scholar]