Abstract
1. Those features of a transmembrane current correlated with spike initiation were examined in Aplysia neurones using a Gaussian white-noise stimulus. This stimulus has the advantages that it presents numerous wave forms in random order without prejudgement as to their efficacies, and that it allows straightforward statistical calculations. 2. Stimulation with a repeating segment of Gaussian white-noise current revealed remarkable invariance in the firing times of the tested neurones and indicated a high degree of reliability of their response. 3. Frequencies (less than 5 Hz) involved in spike triggering propagated faithfully for up to several millimetres, justifying intrasomatic current injection to examine spike initiation at the trigger locus. 4. Examination of current wave forms preceding spikes indicated that a wide variety could be effective. Hence, a statistical analysis was performed, including computation of probability densities, averages, standard deviations and correlation coefficients of pairs of current values. Each statistic was displayed as a function of time before the spike. 5. The average current trajectory preceding a spike was multiphasic and depended on the presence and polarity of a d.c. bias. An early relatively small inward- or outward-going phase was followed by a large outward phase before the spike. The early phase tended to oppose the polarity of the d.c. bias. 6. The late outward phase of the average current trajectory reached a maximum 40--75 msec before triggering the action potential (AP) and returned to near zero values at the moment of triggering. The fact that the current peak occurs in advance of the AP may be partially explained by a phase delay between the transmembrane current and potential. The failure of the average current trajectory to return to control values immediately following the peak argues for a positive role of the declining phase in spike triggering. 7. Probability densities preceding spikes were Gaussian, indicating that the average was also the most probable value. Although the densities were broad, confirming that spikes were preceded by a wide variety of current wave forms, their standard deviations were reduced significantly with respect to controls, suggesting preferred status of the average current trajectory in spike triggering. 8. The matrix of correlation coefficients between current pairs suggested that spikes tended to be preceded by wave forms that in part kept close to the average current trajectory and in part preserved its shape. 9. The average first and second derivatives of spike-evoking epochs revealed that current slope and acceleration, respectively, were most crucial in the last 200 msec before spike triggering, and that these dynamic stimulus components were more important for a cell maintained under a depolarizing, rather than a hyperpolarizing bias. 10...
Full text
PDF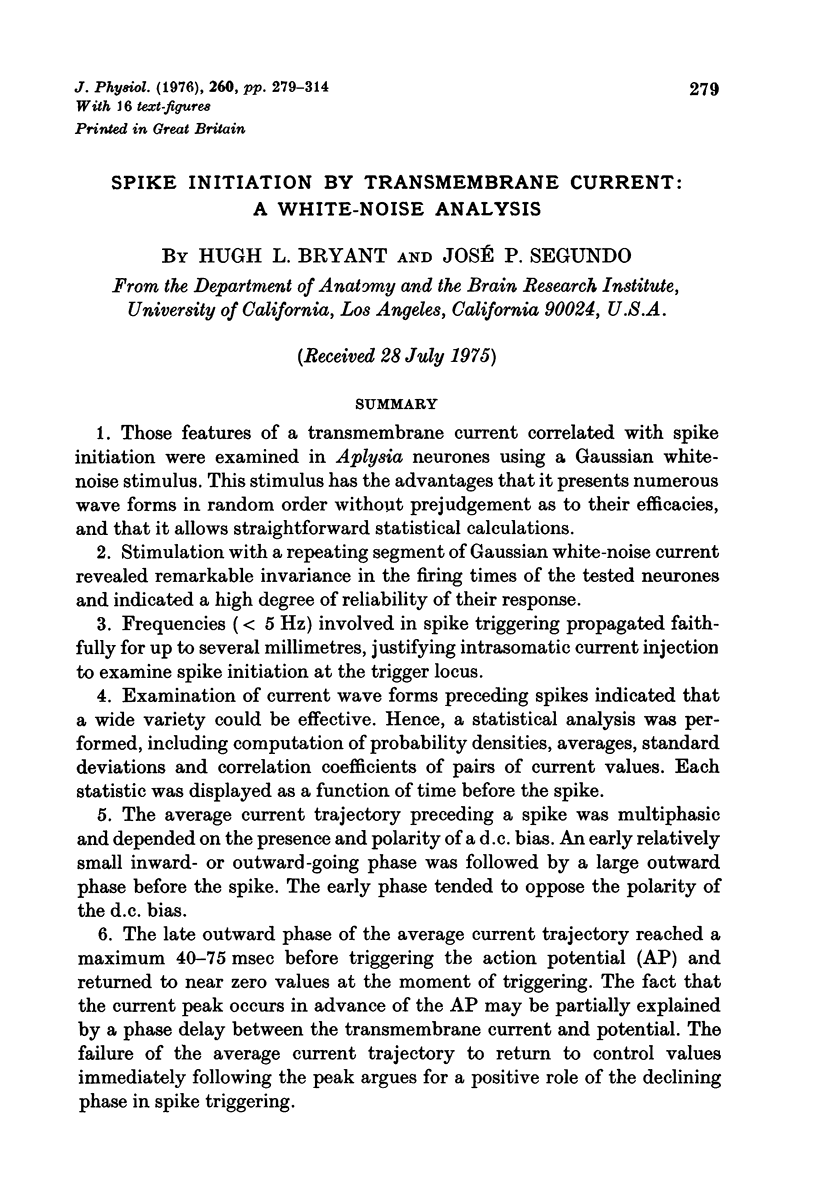
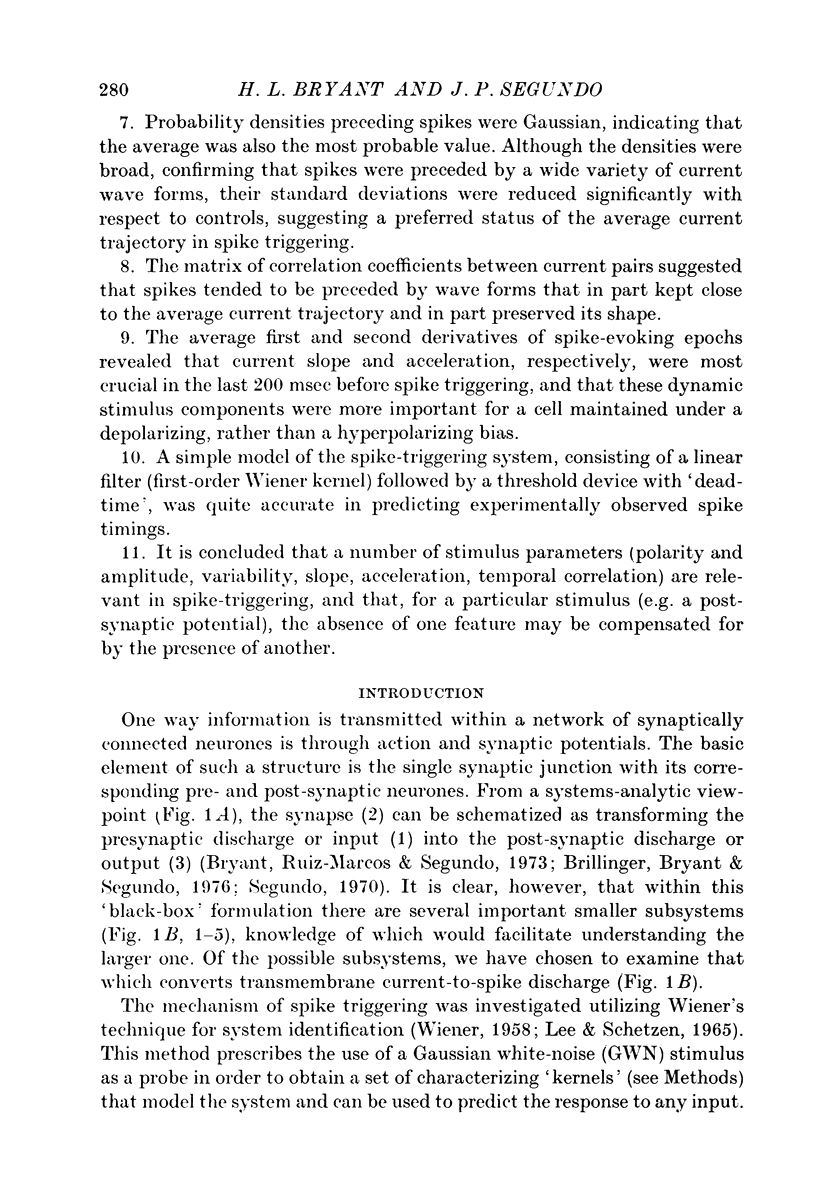
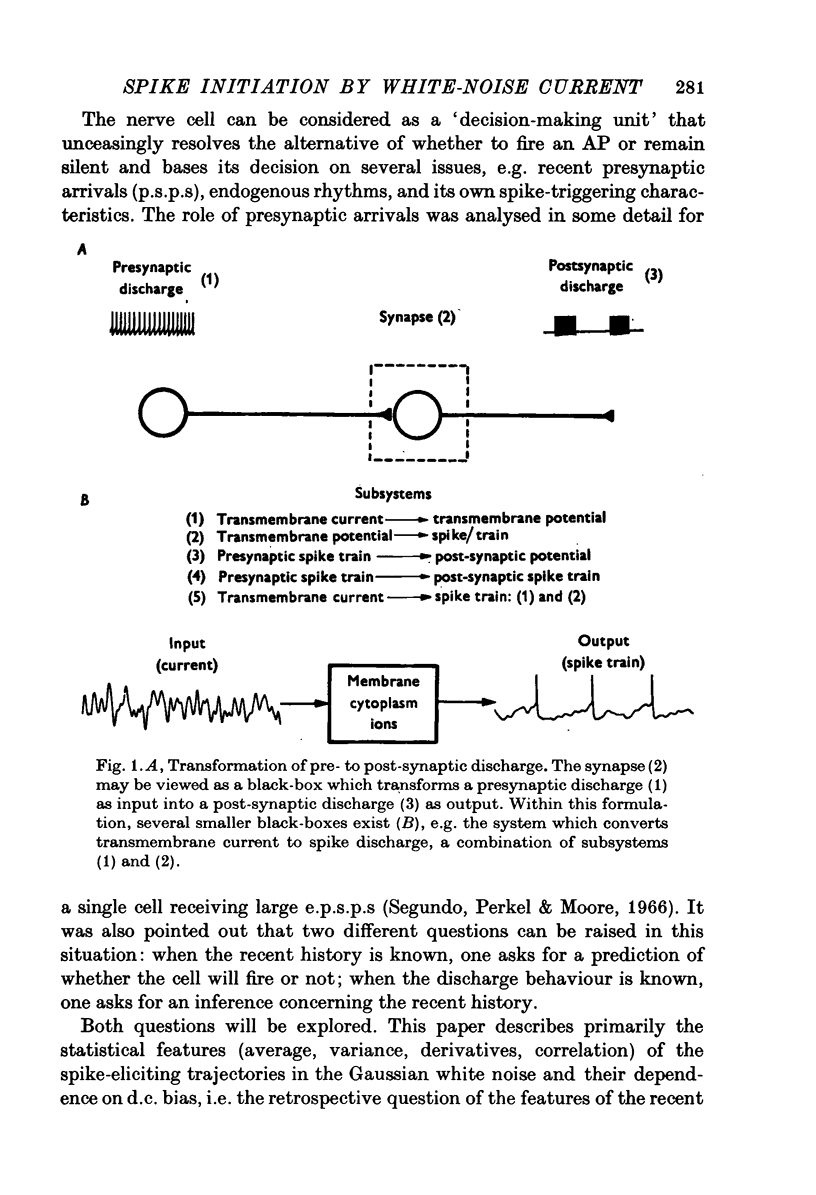
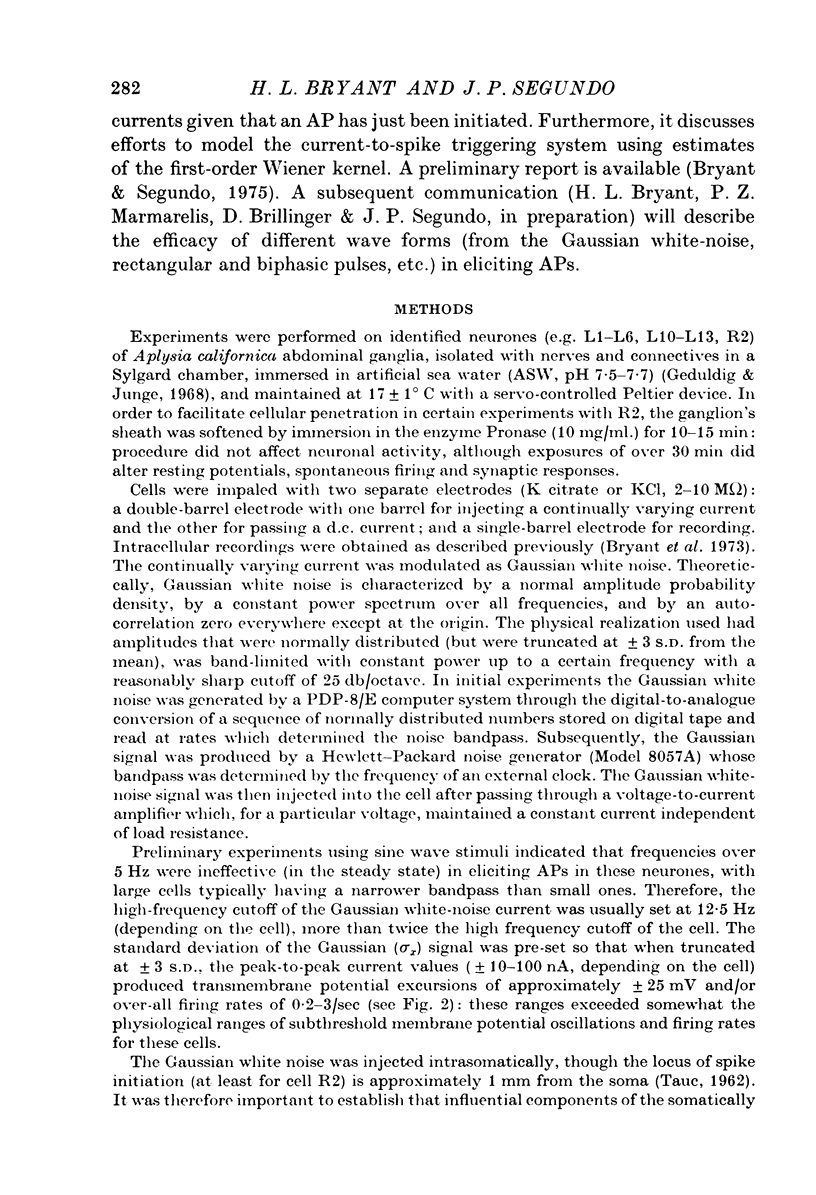
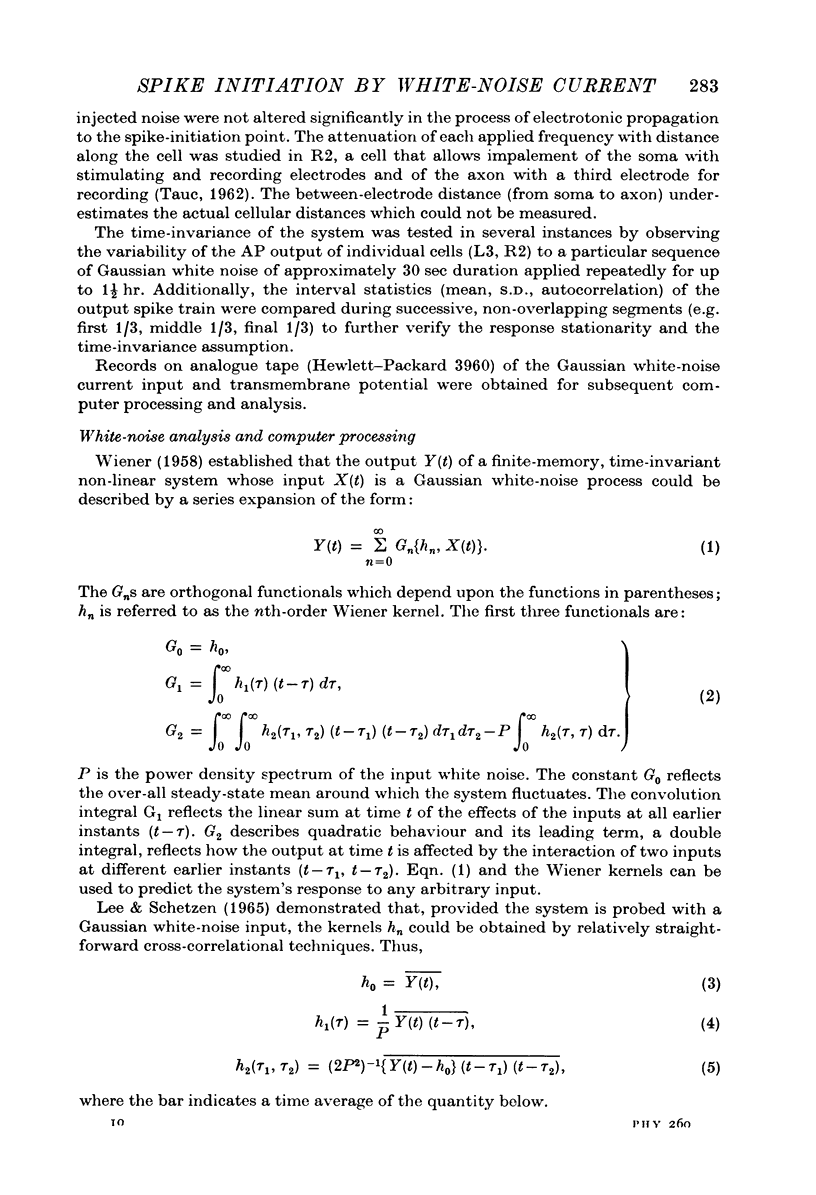
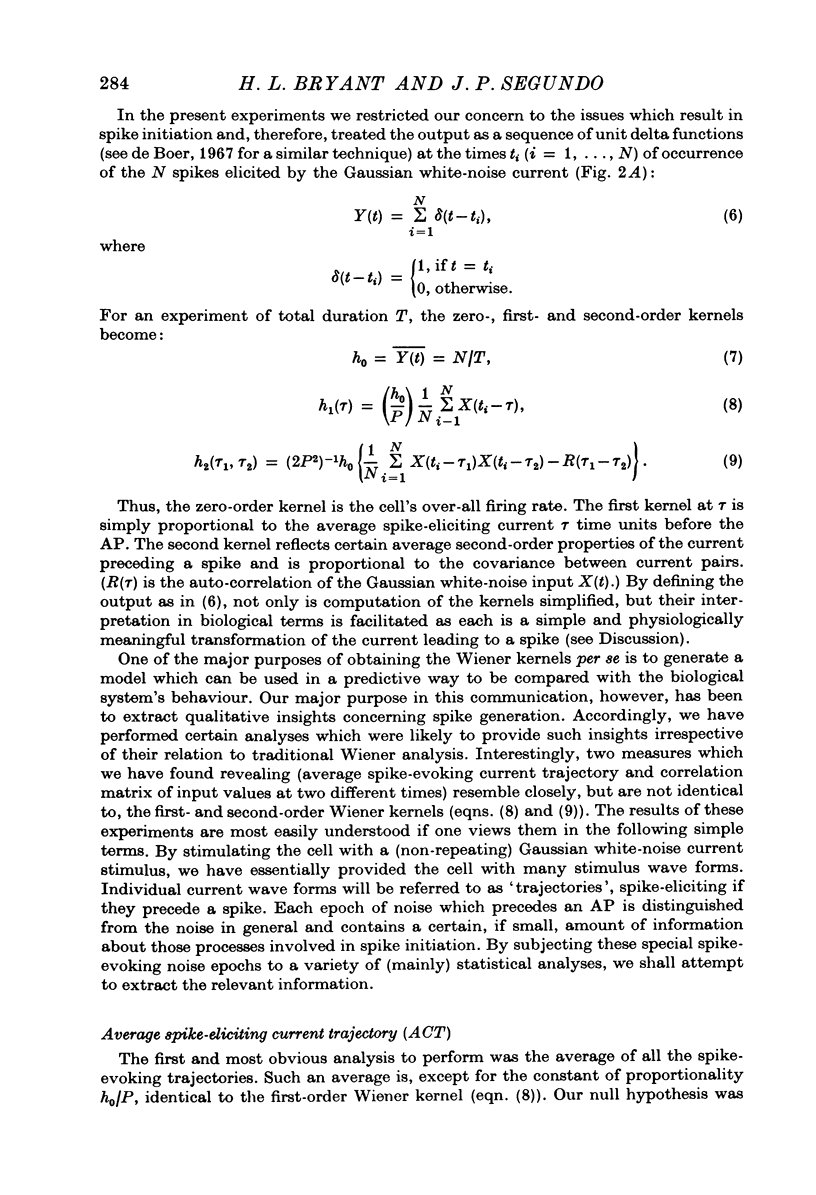
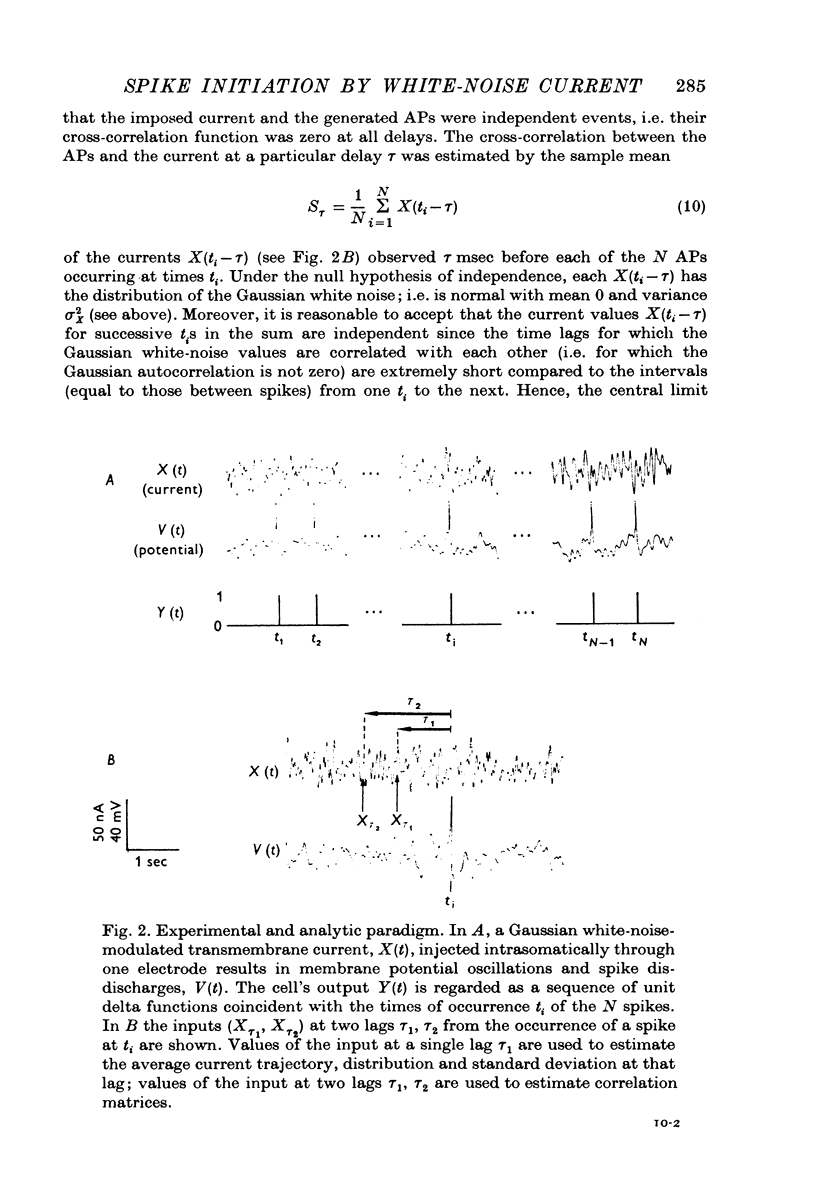
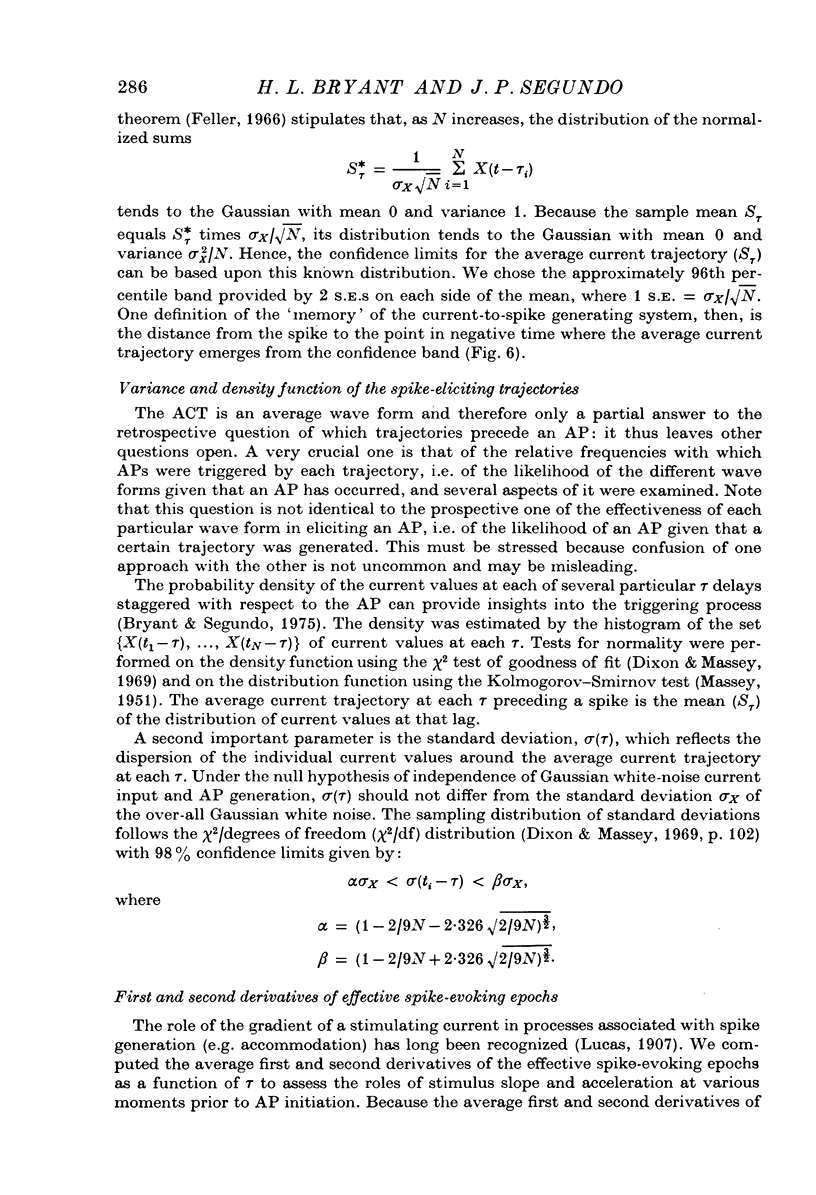
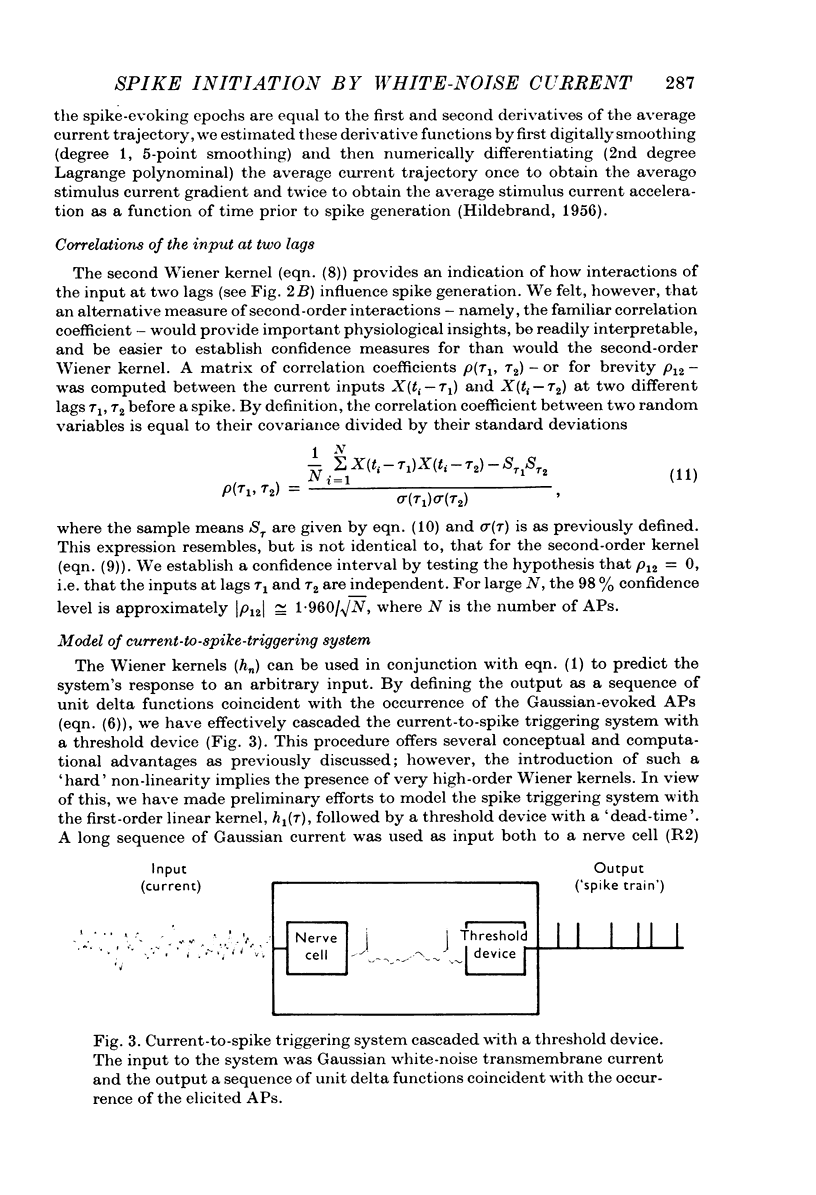
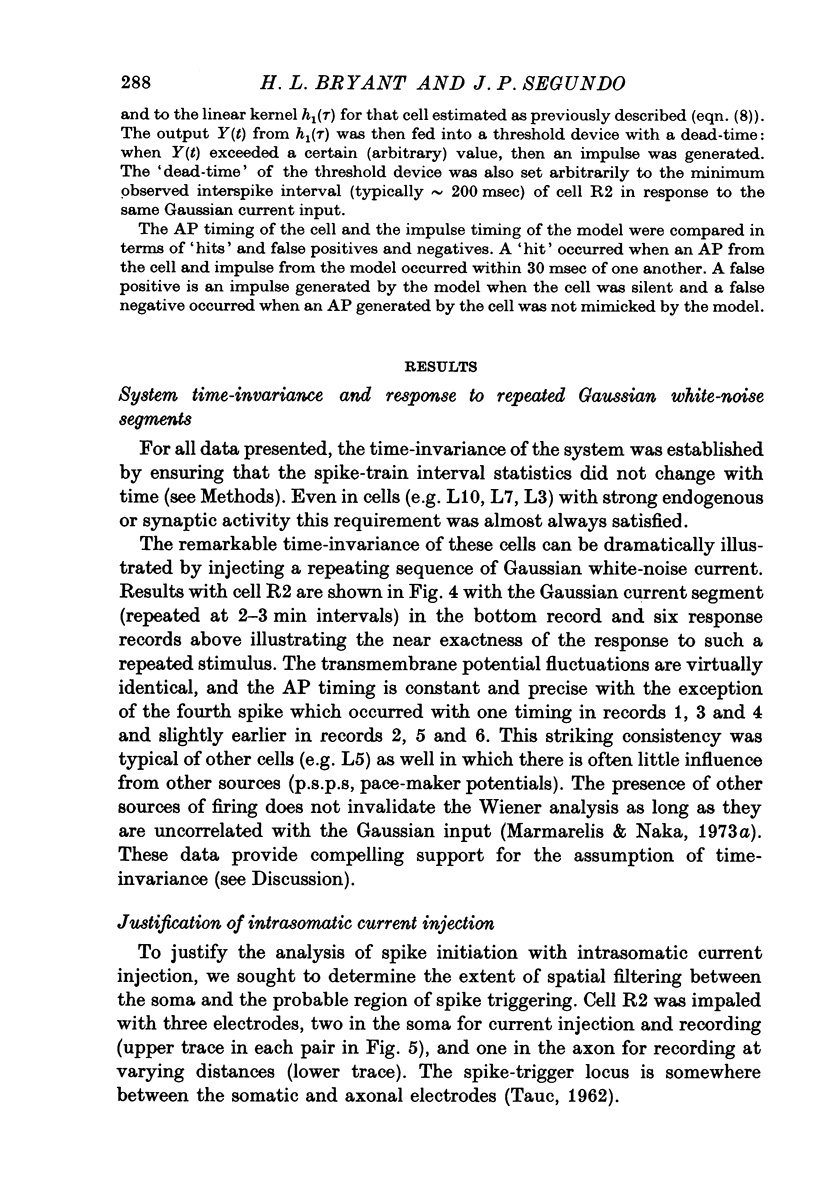
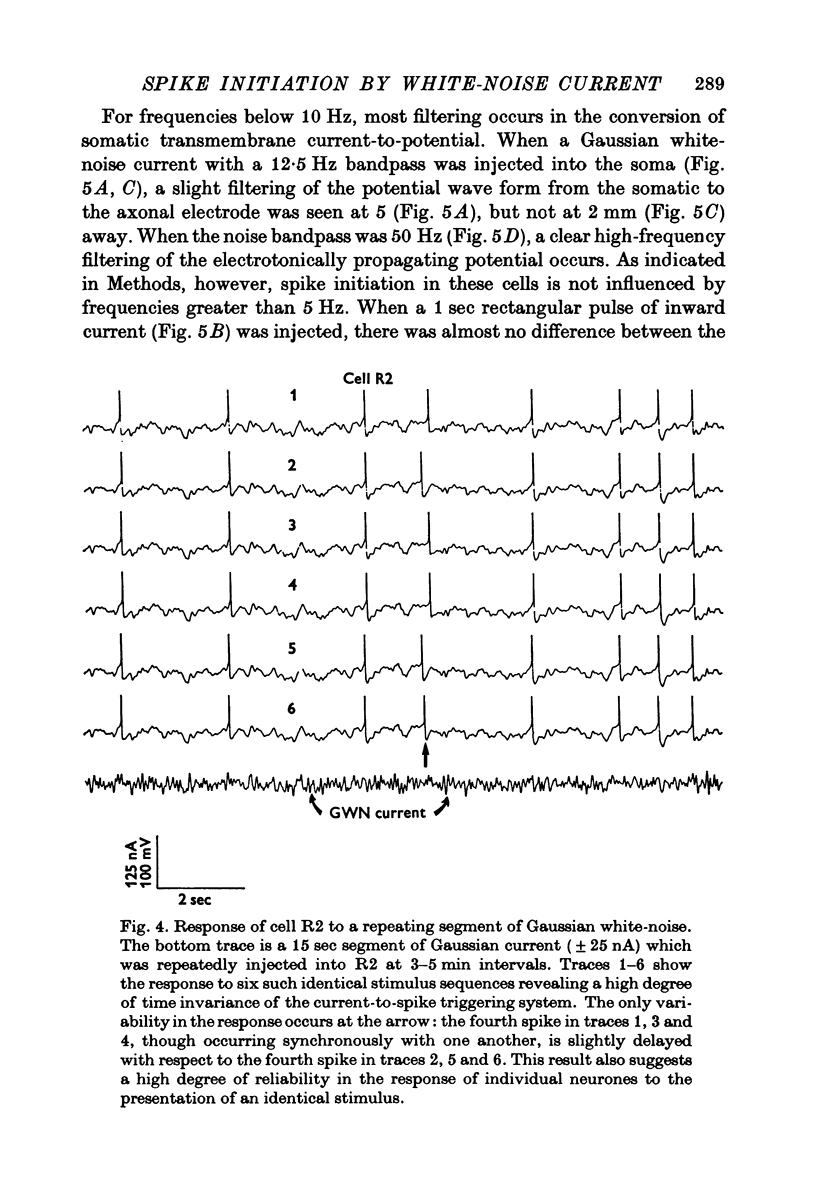
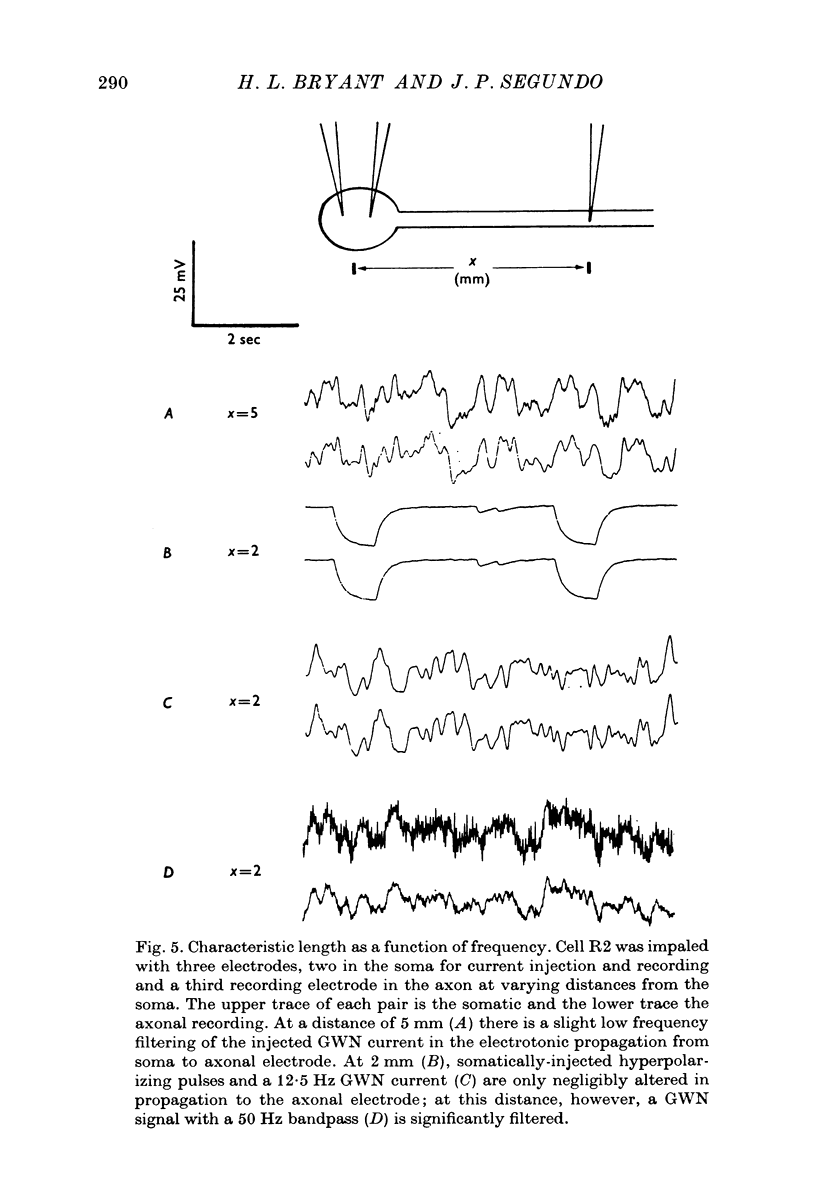
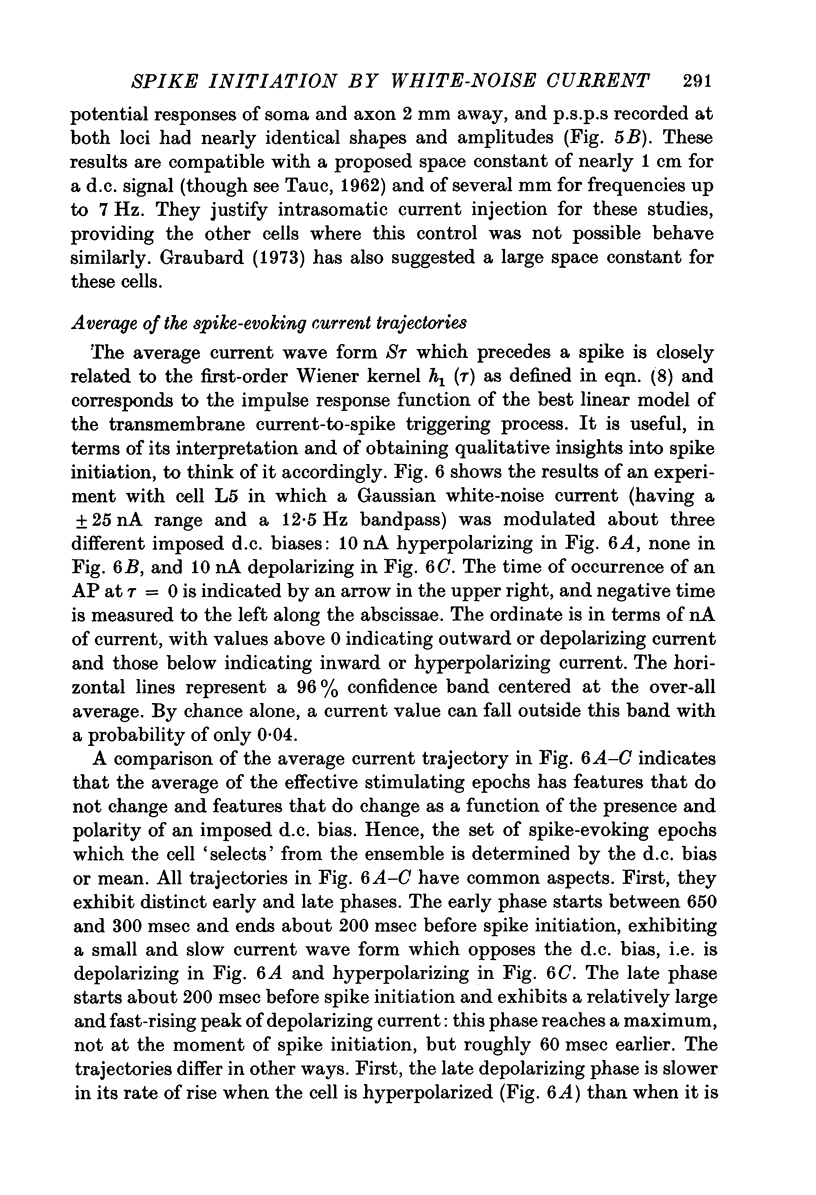
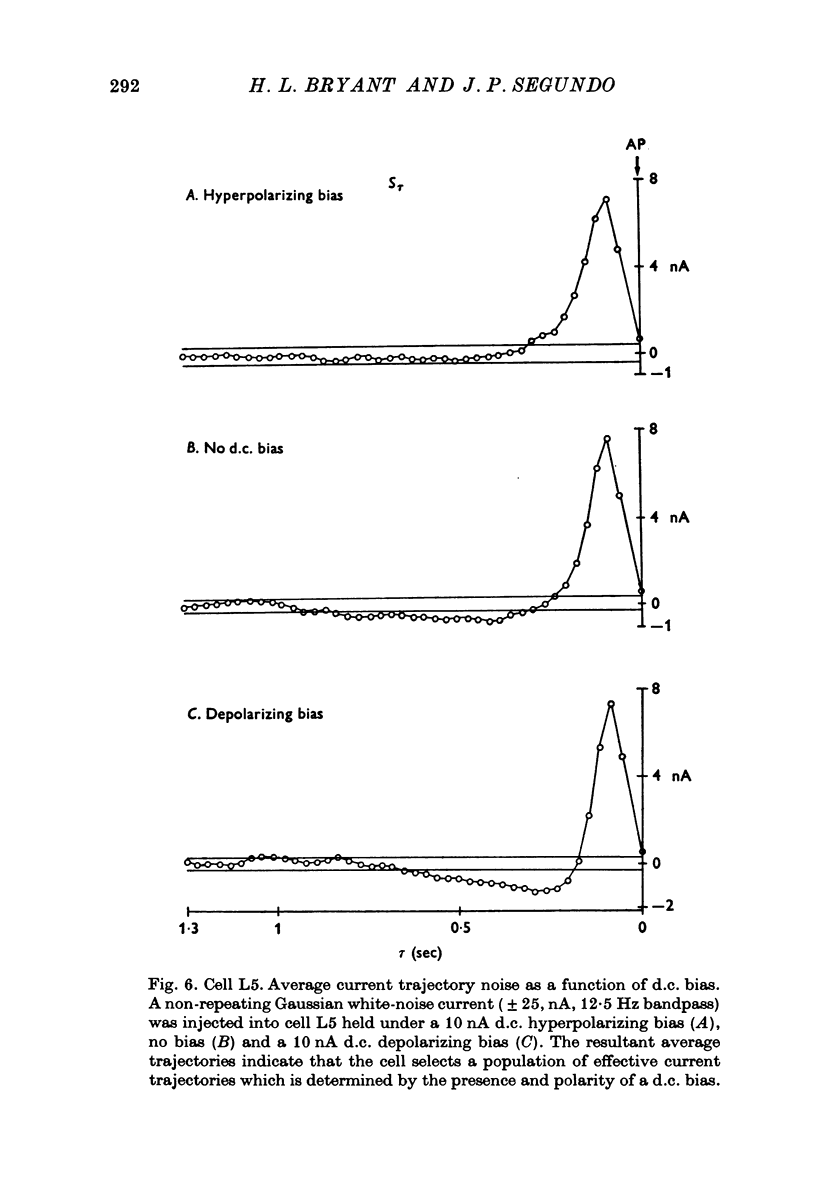
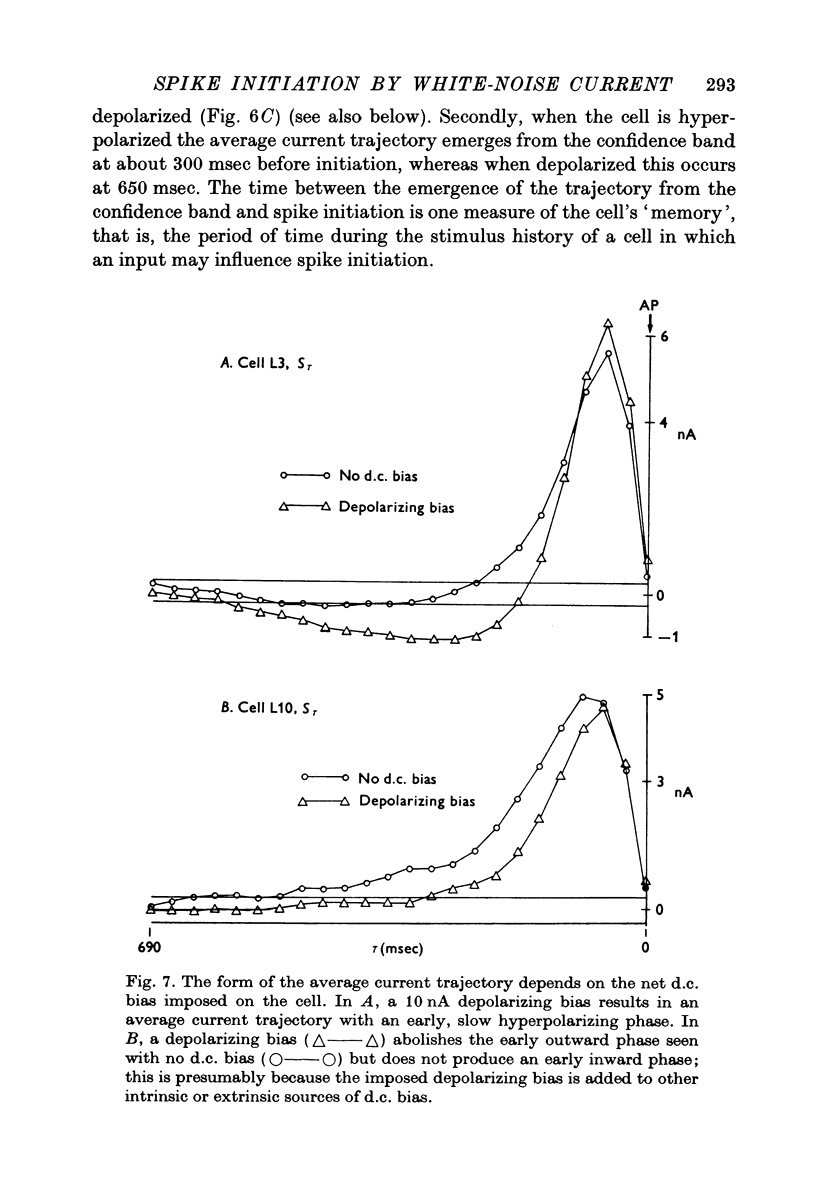
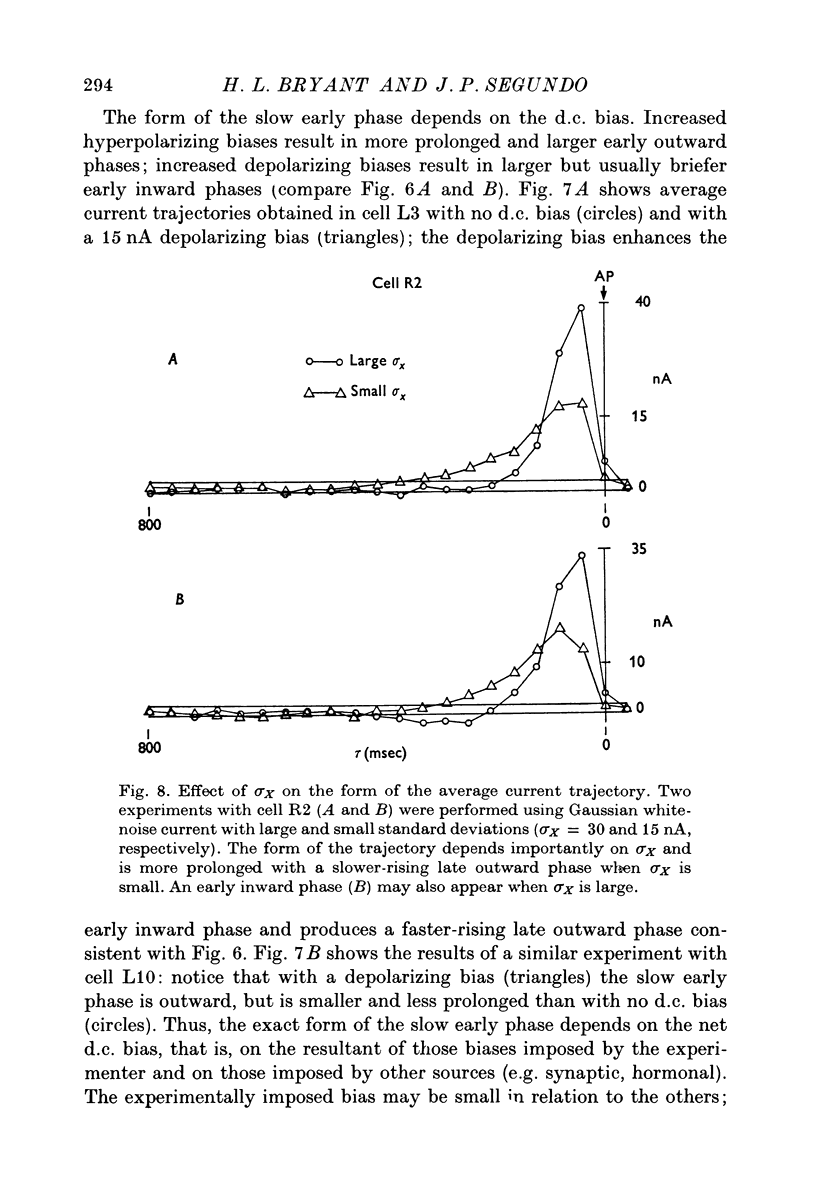
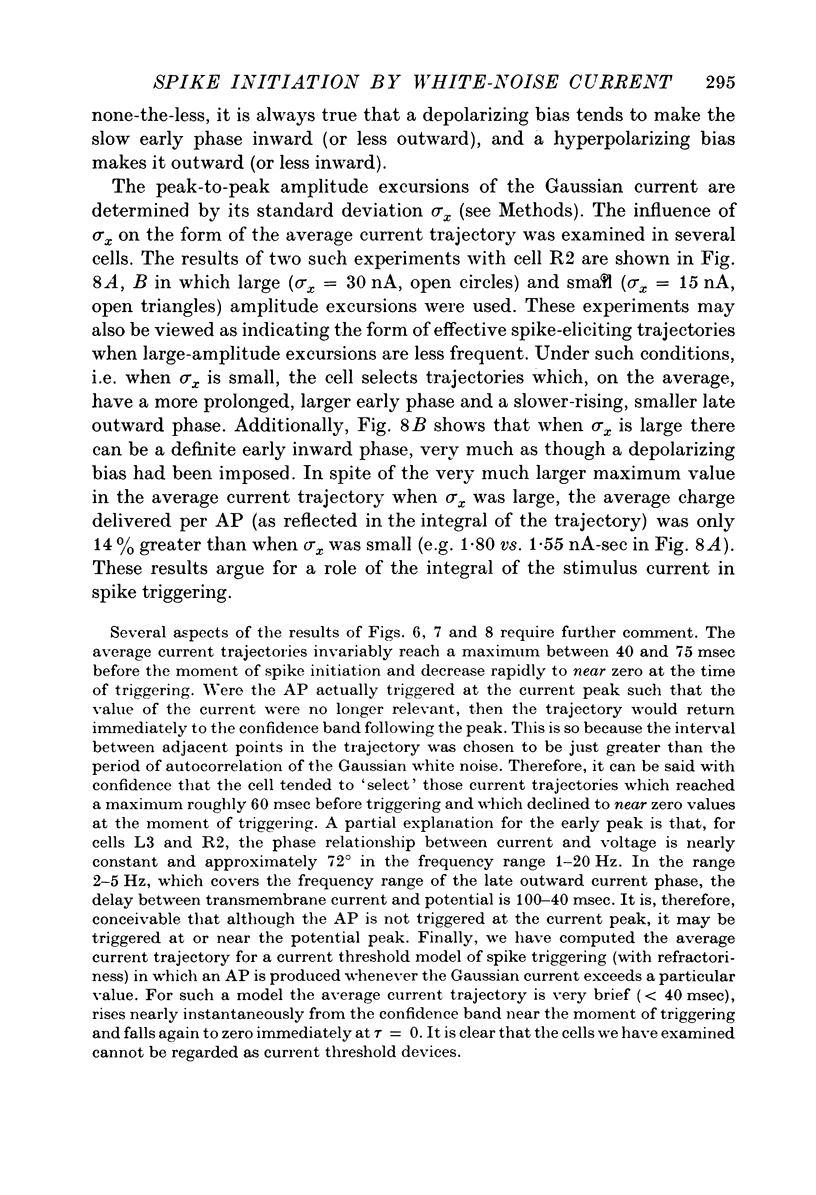
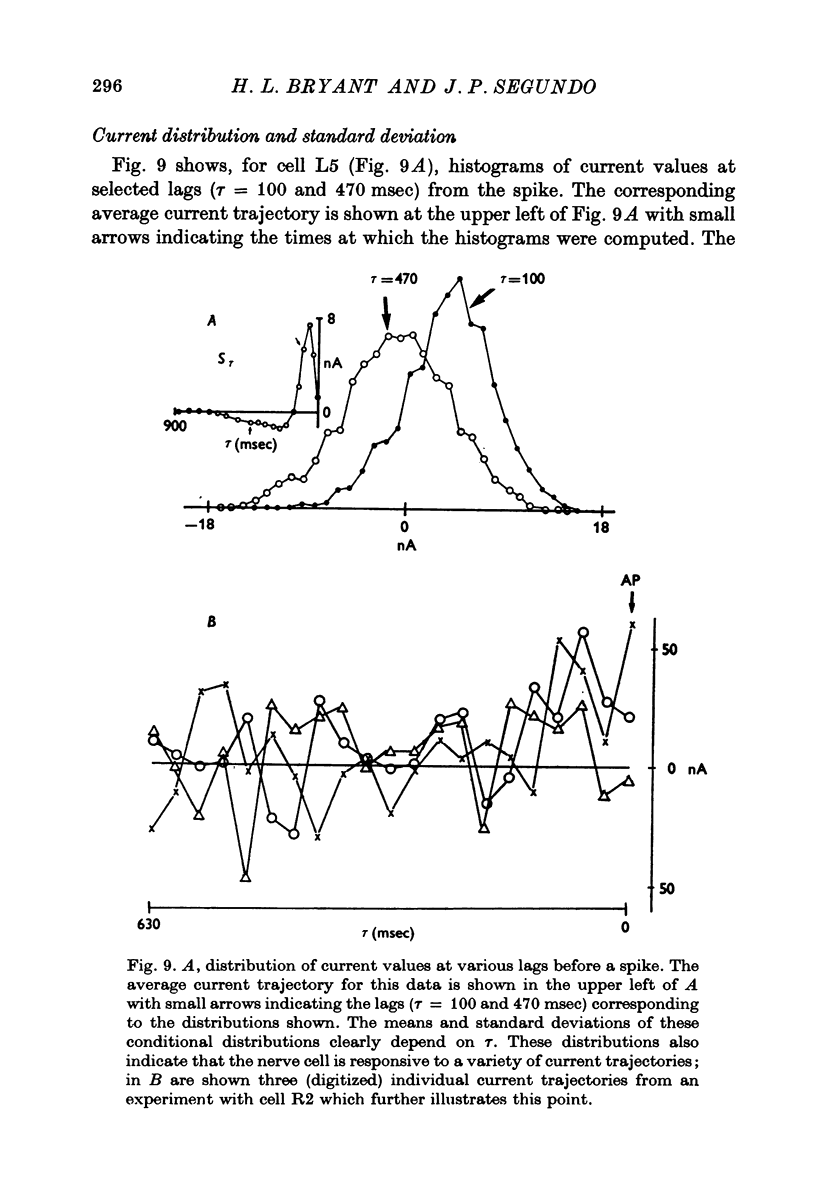
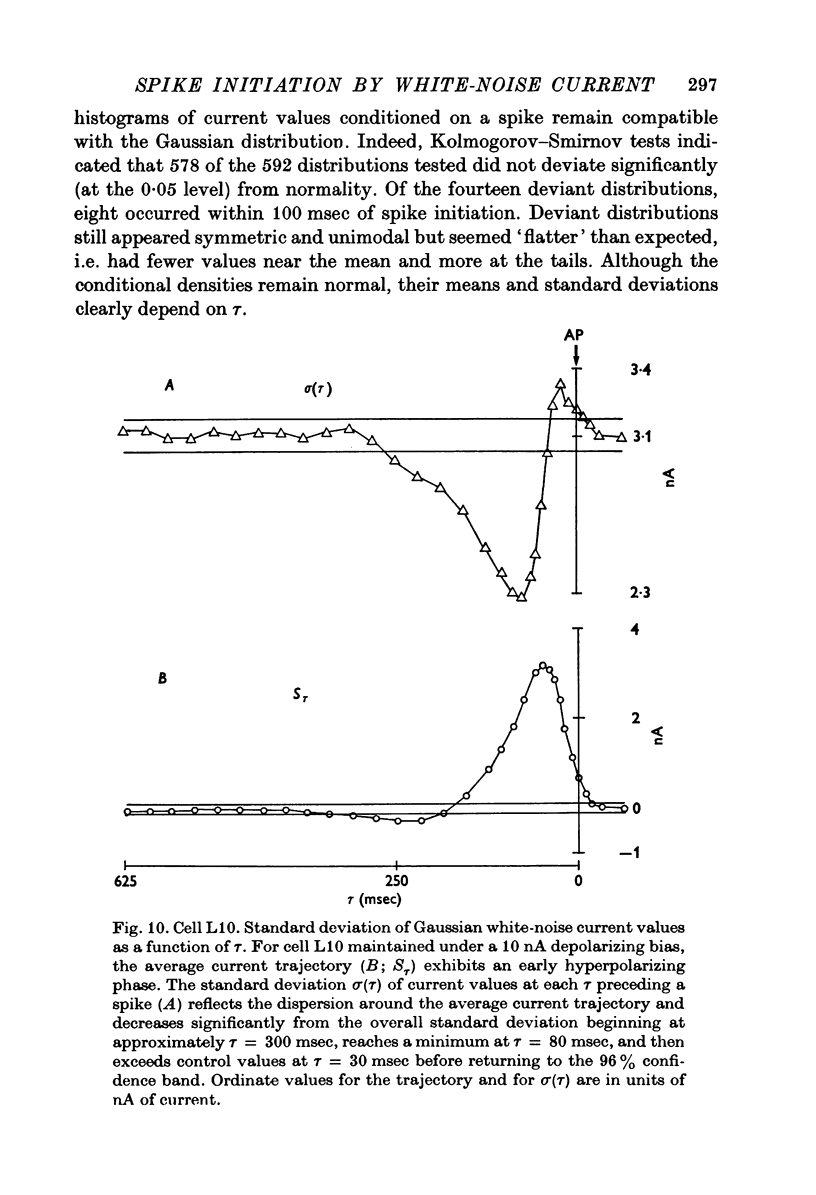
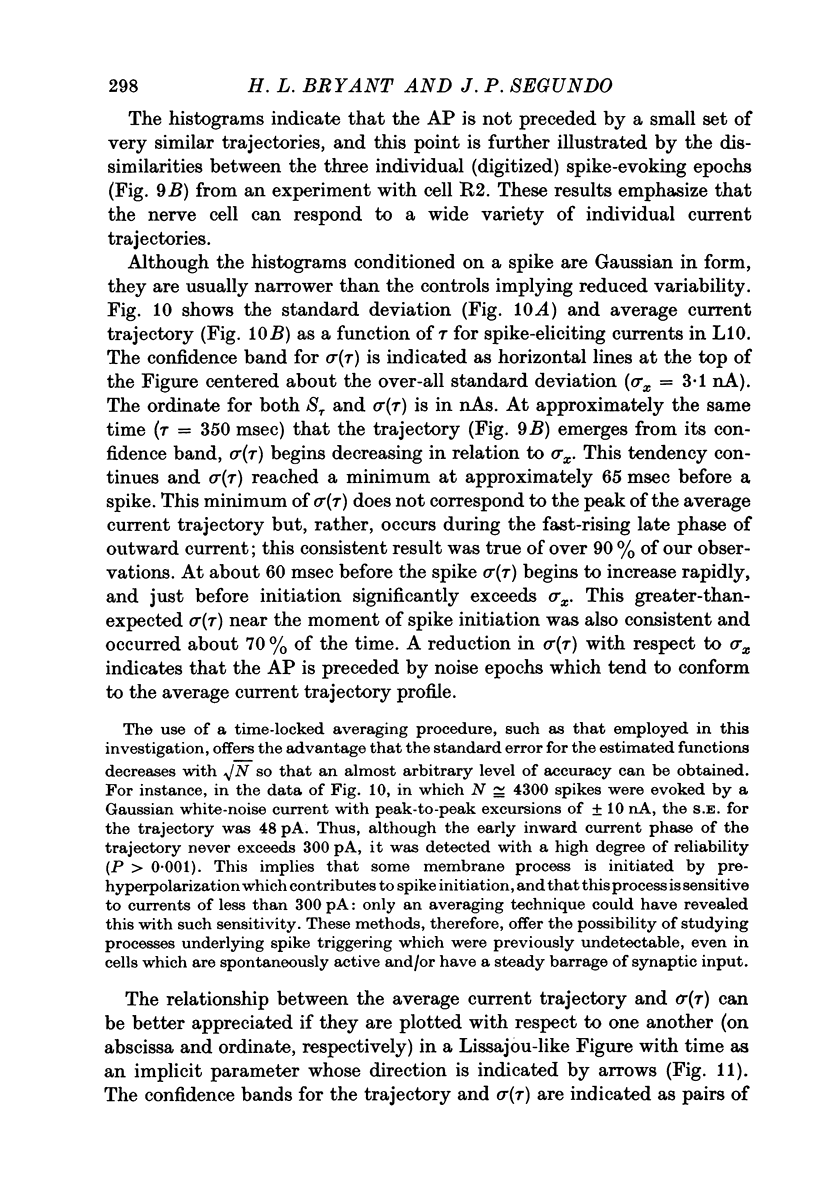
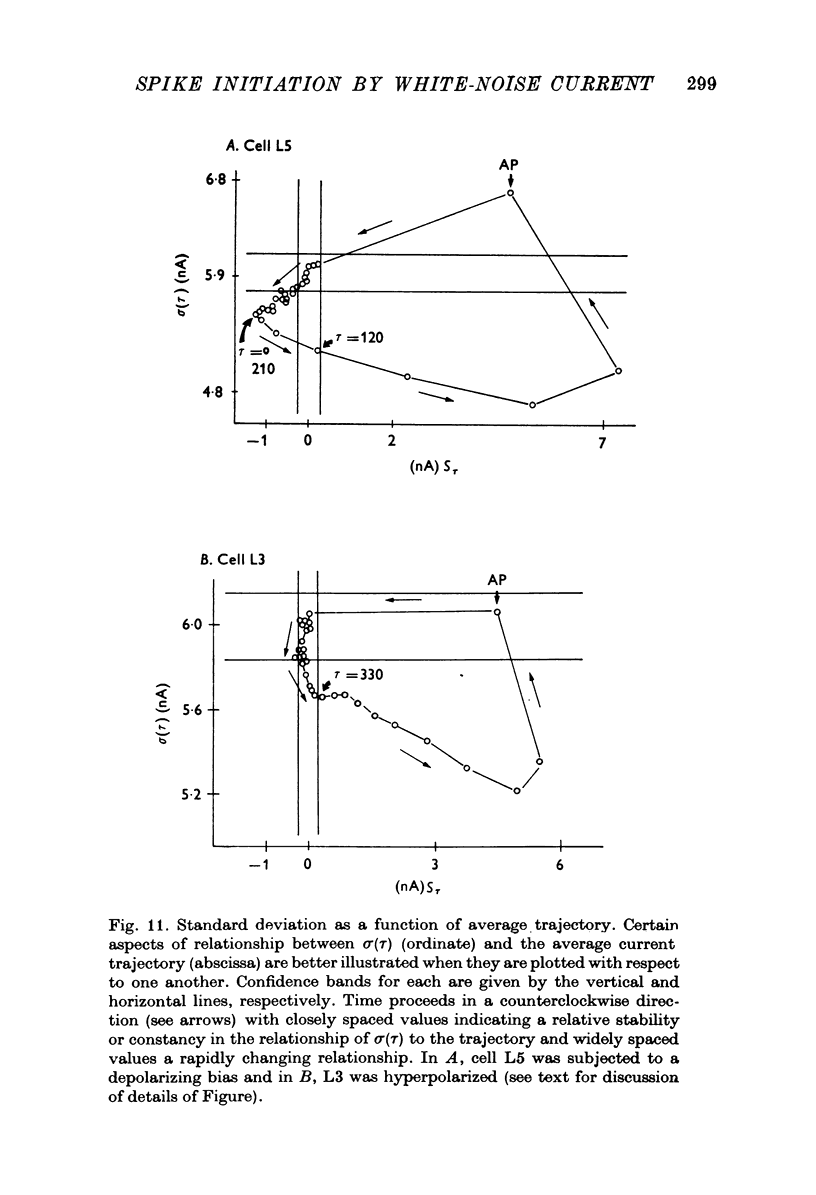
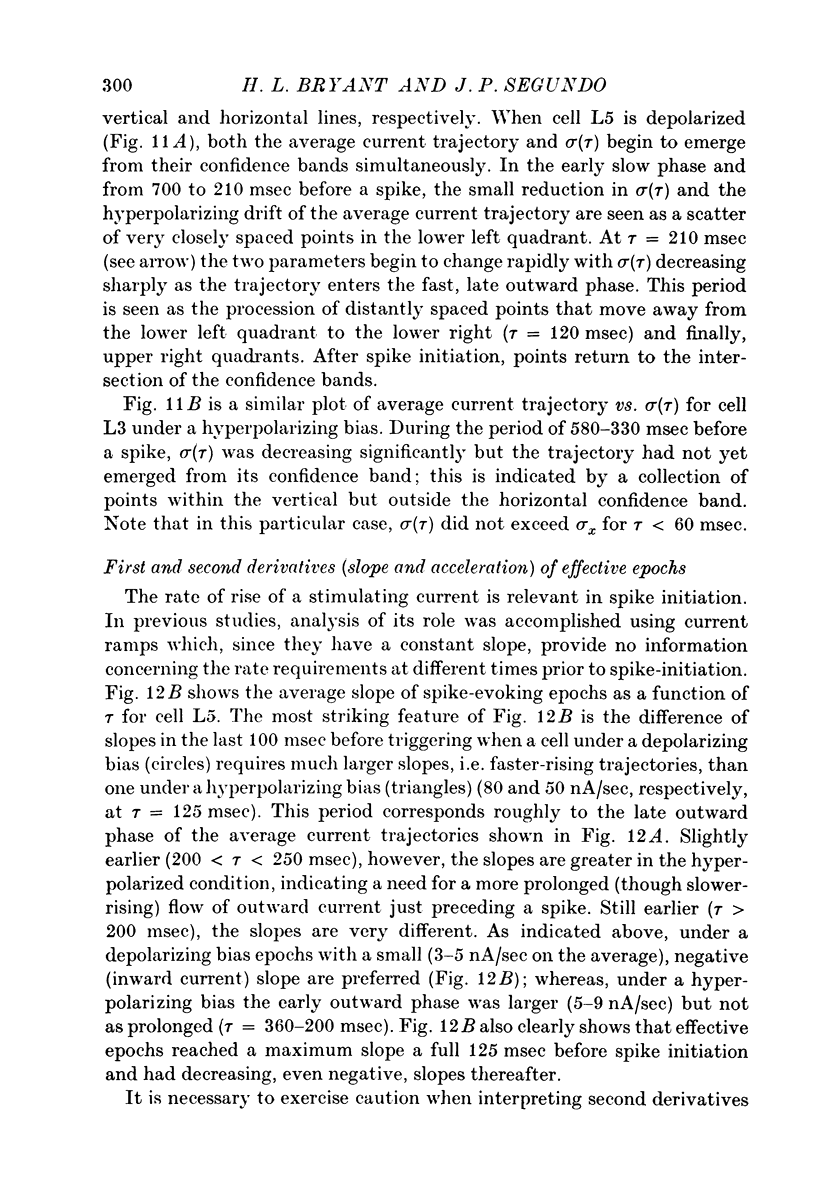
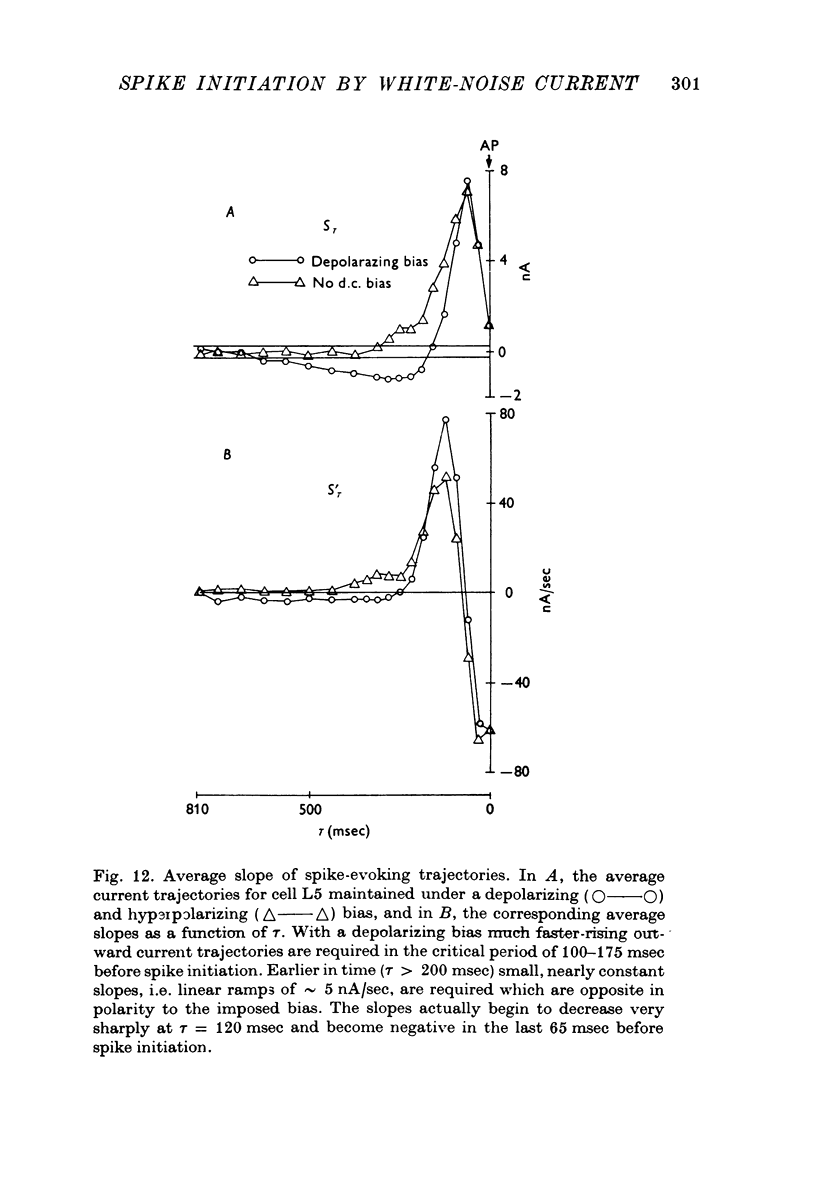
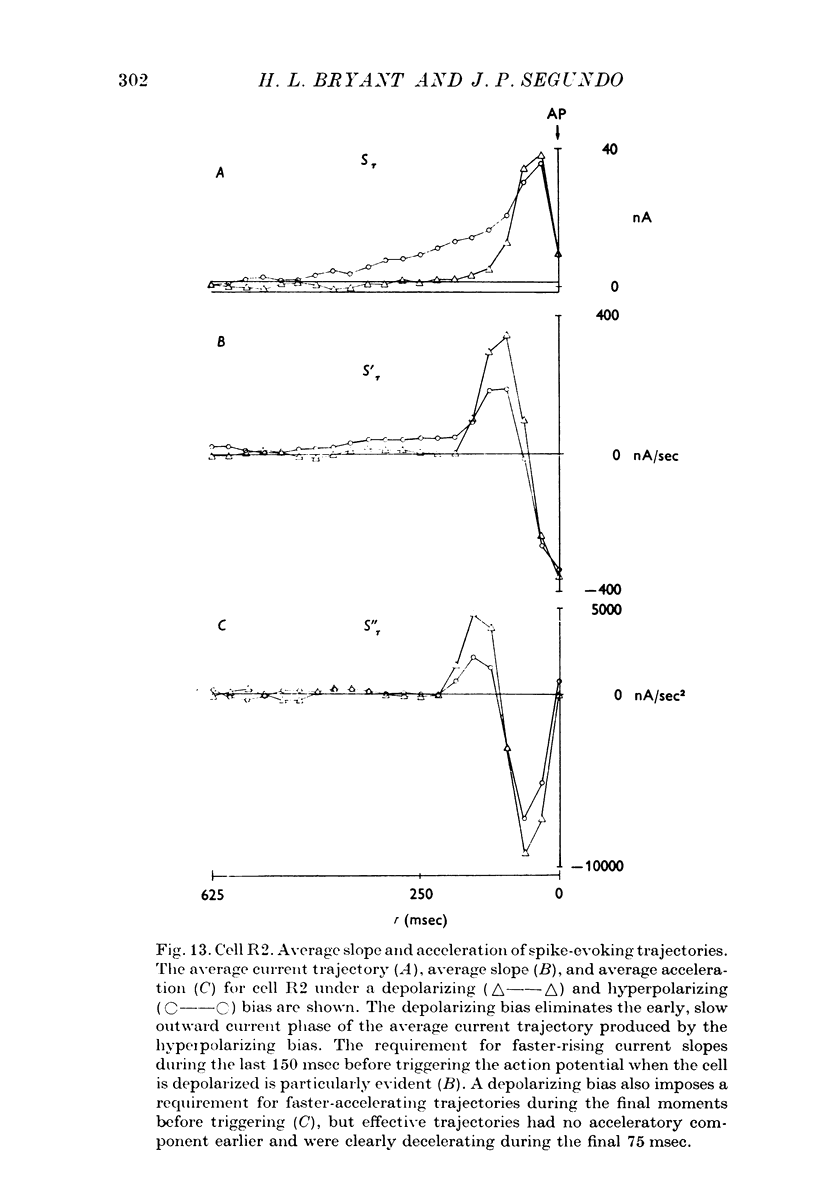
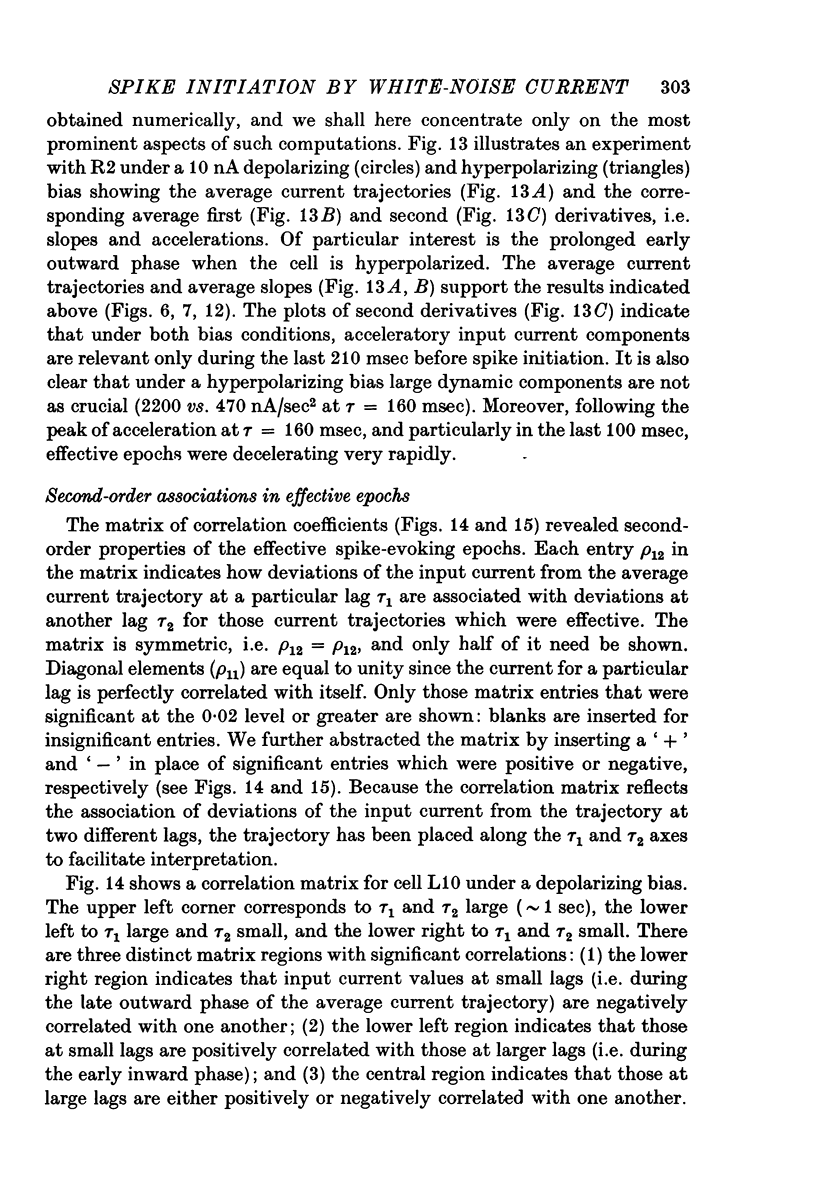
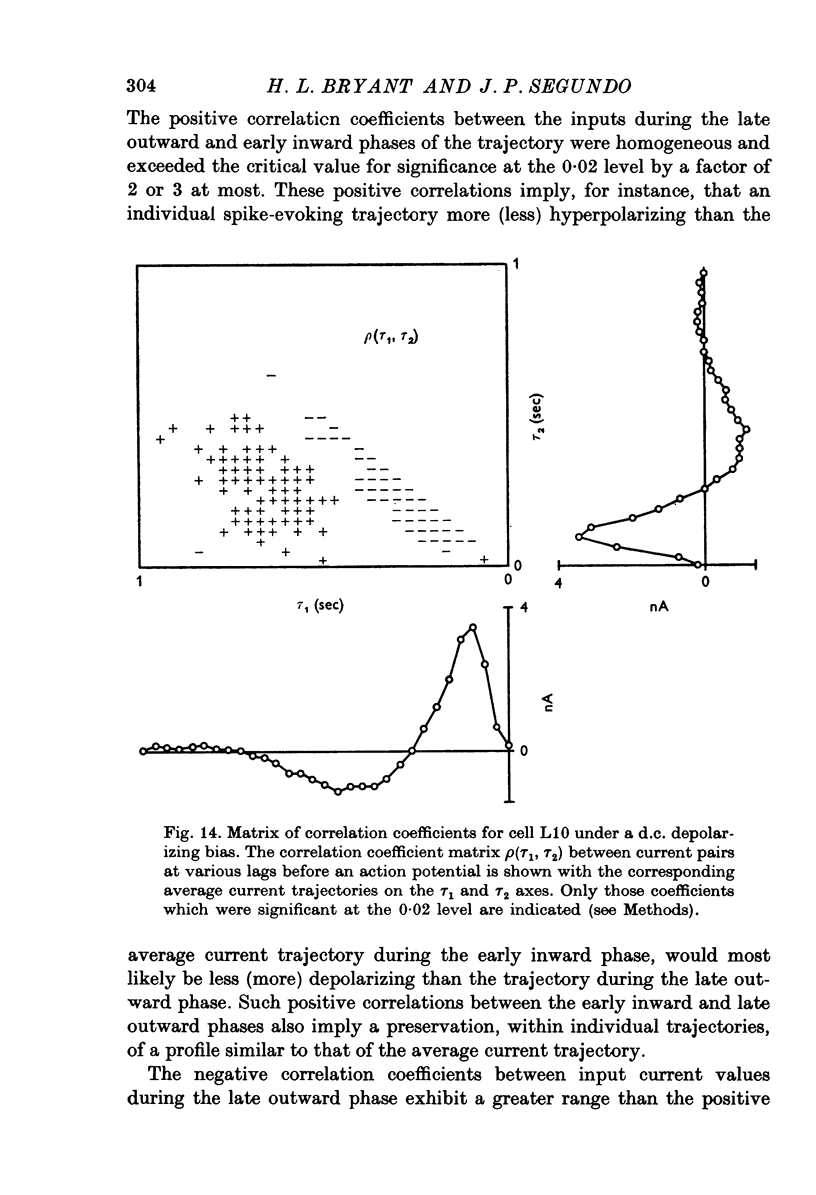
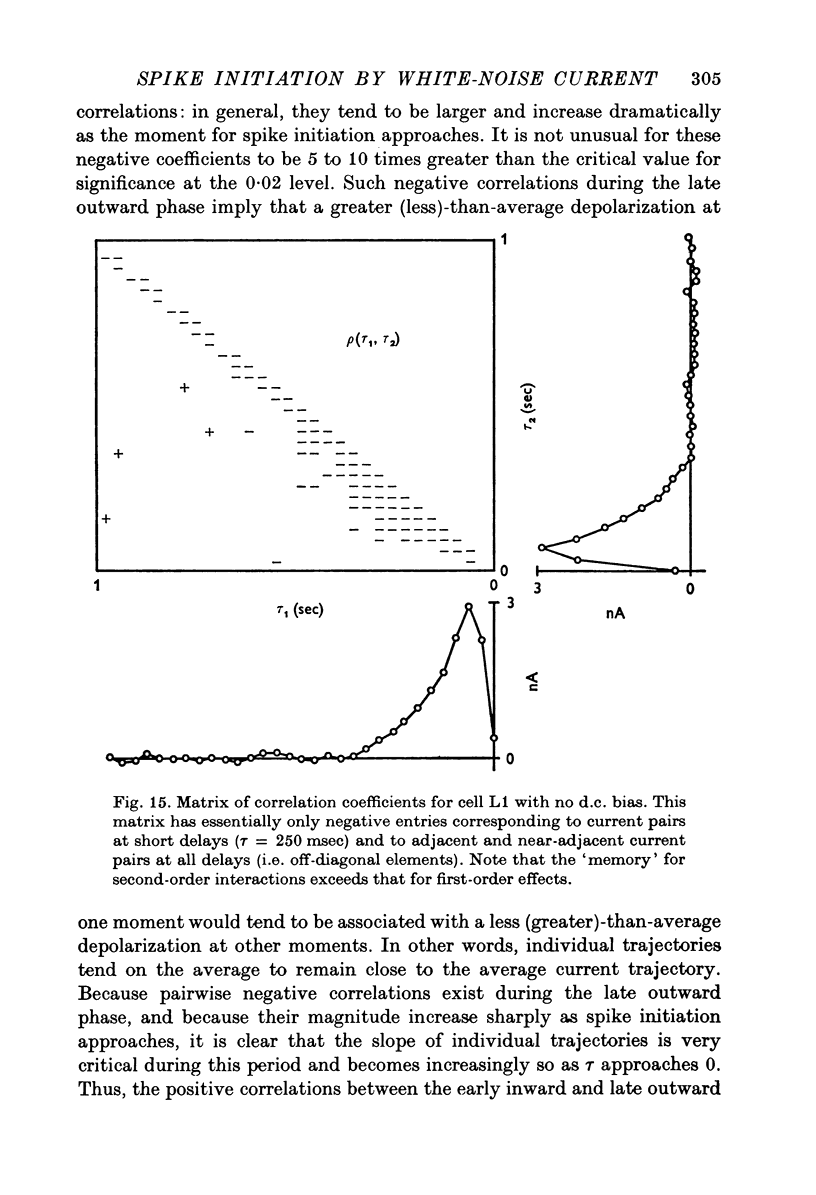
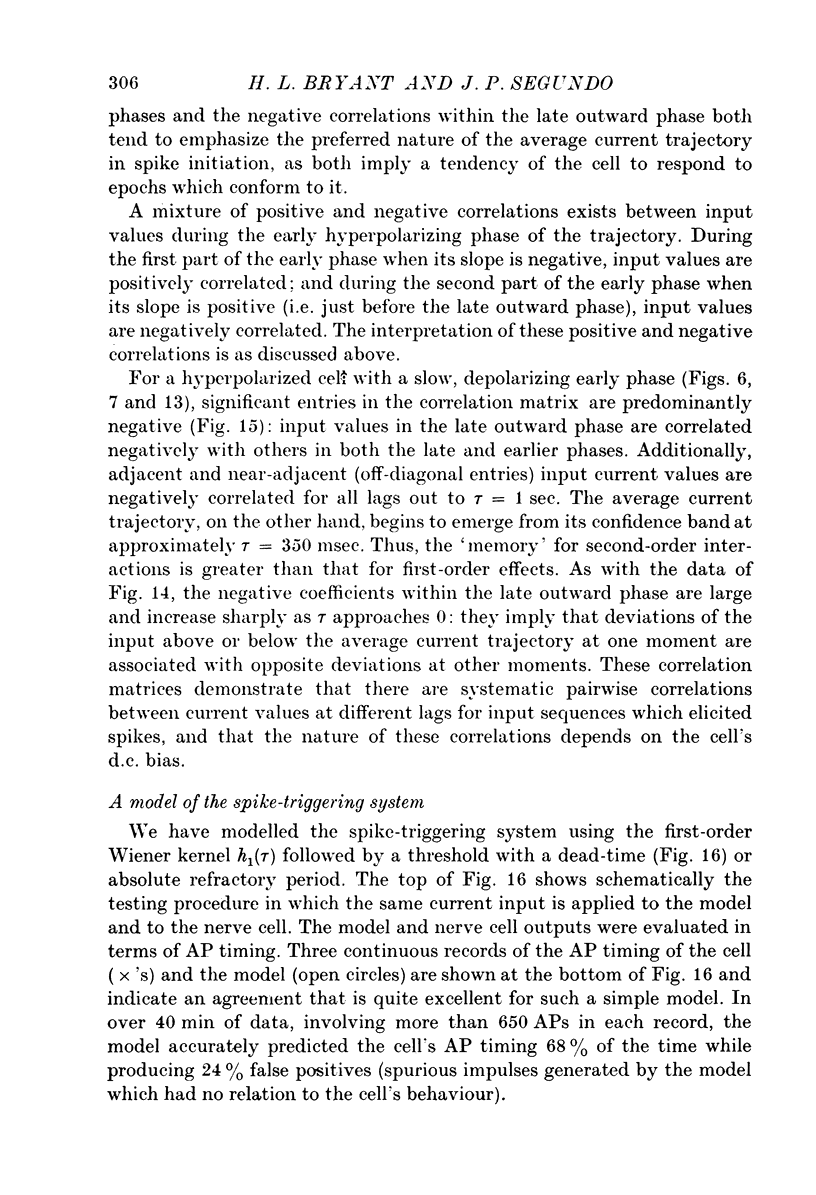
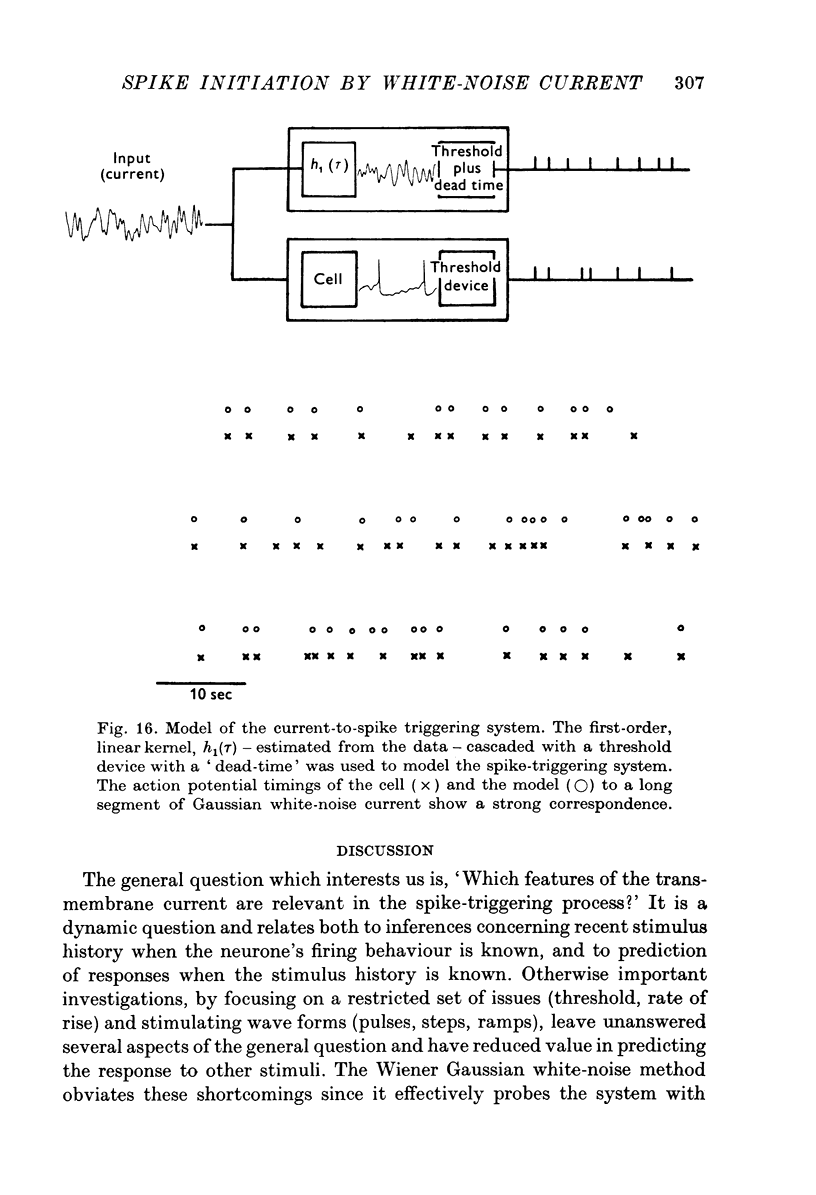
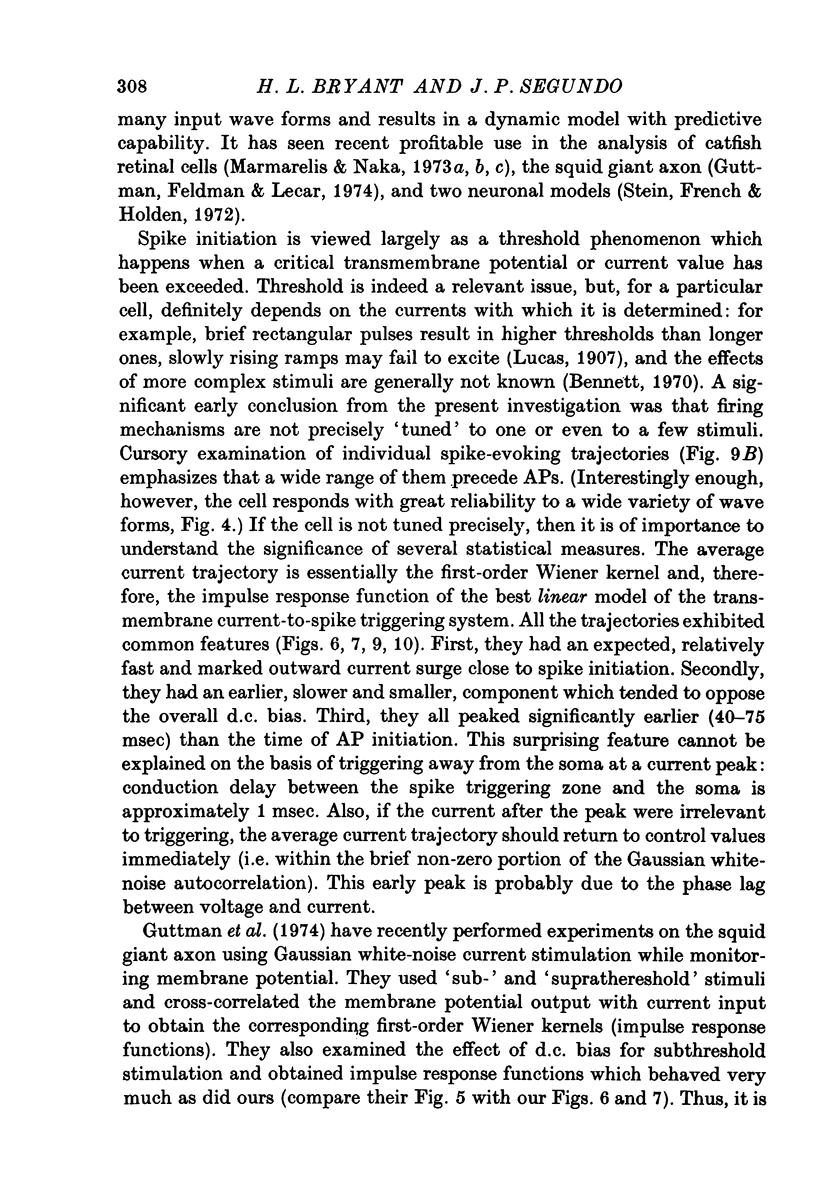
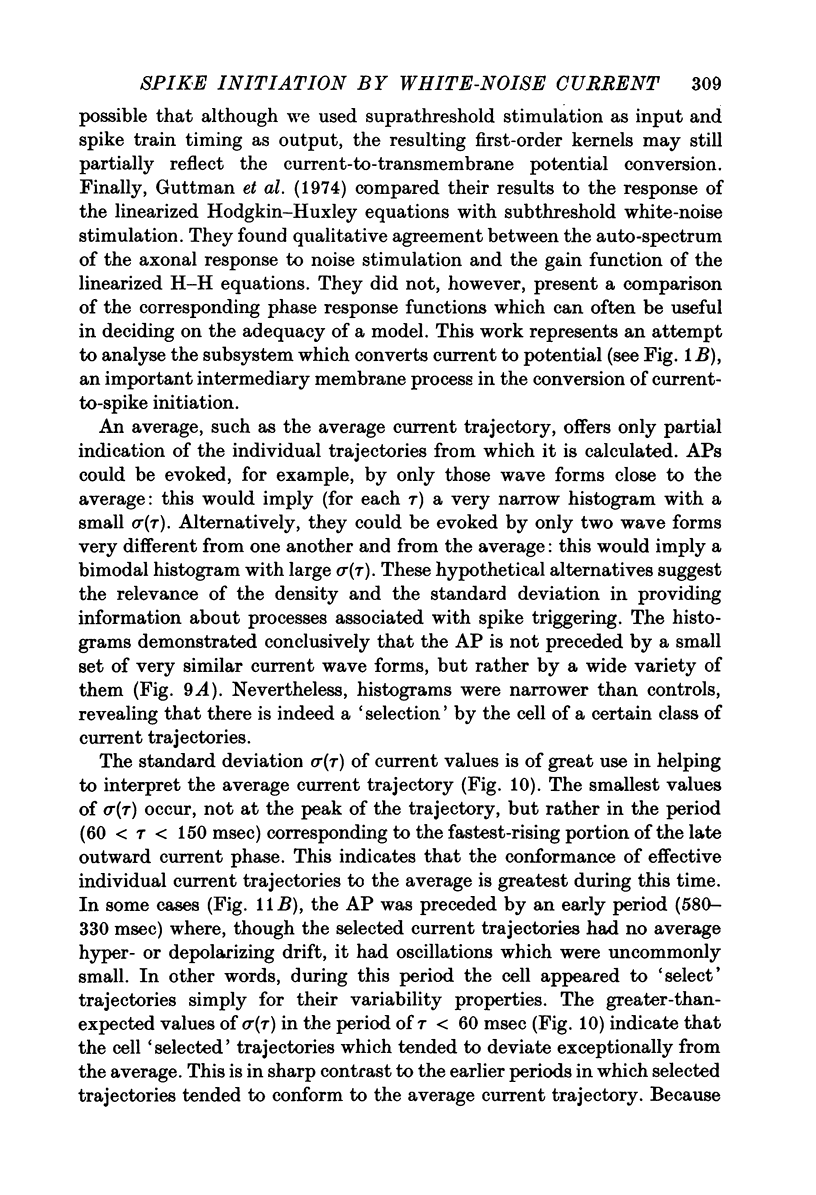
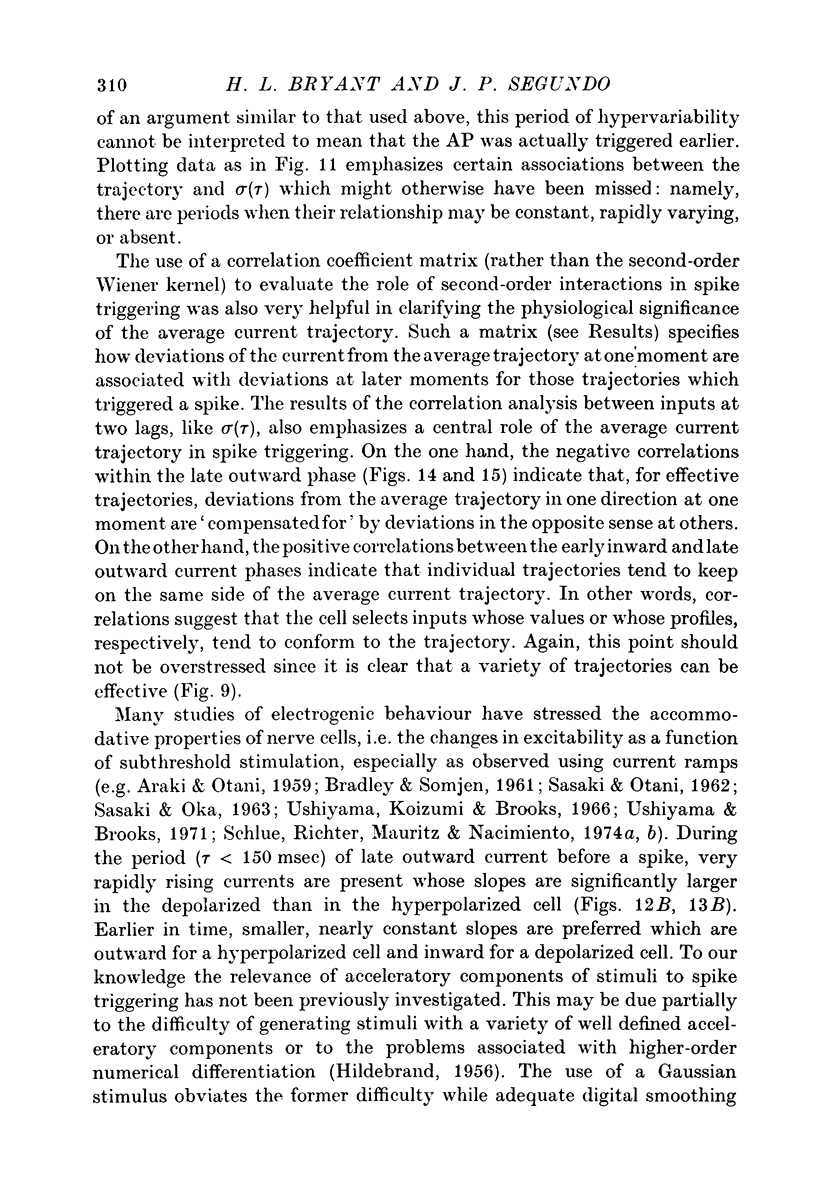
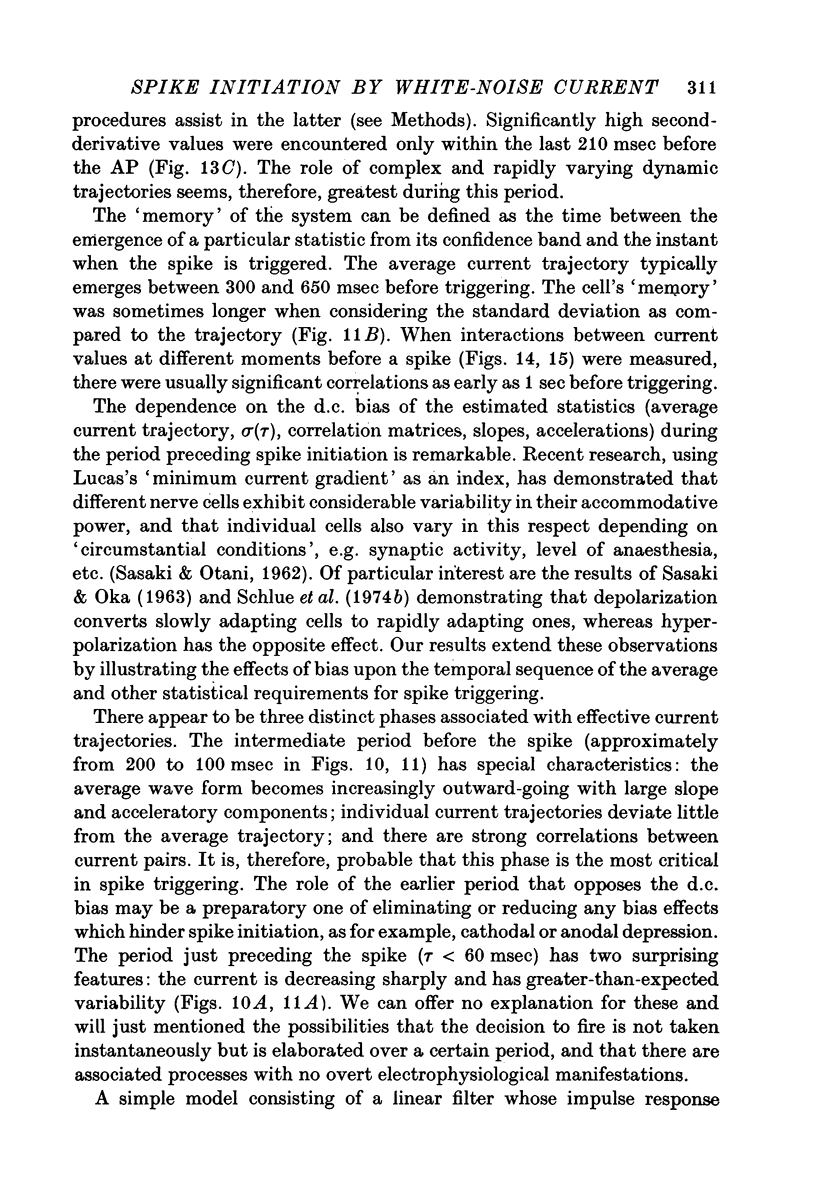
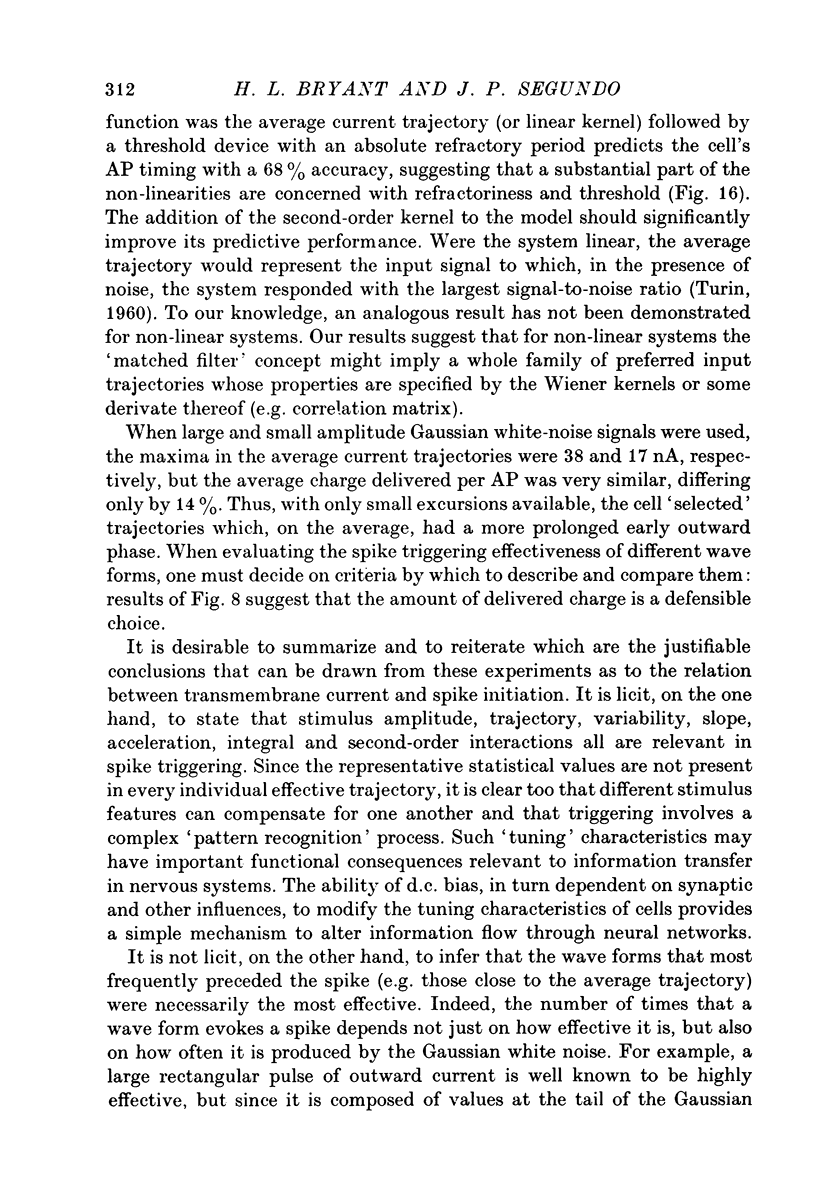
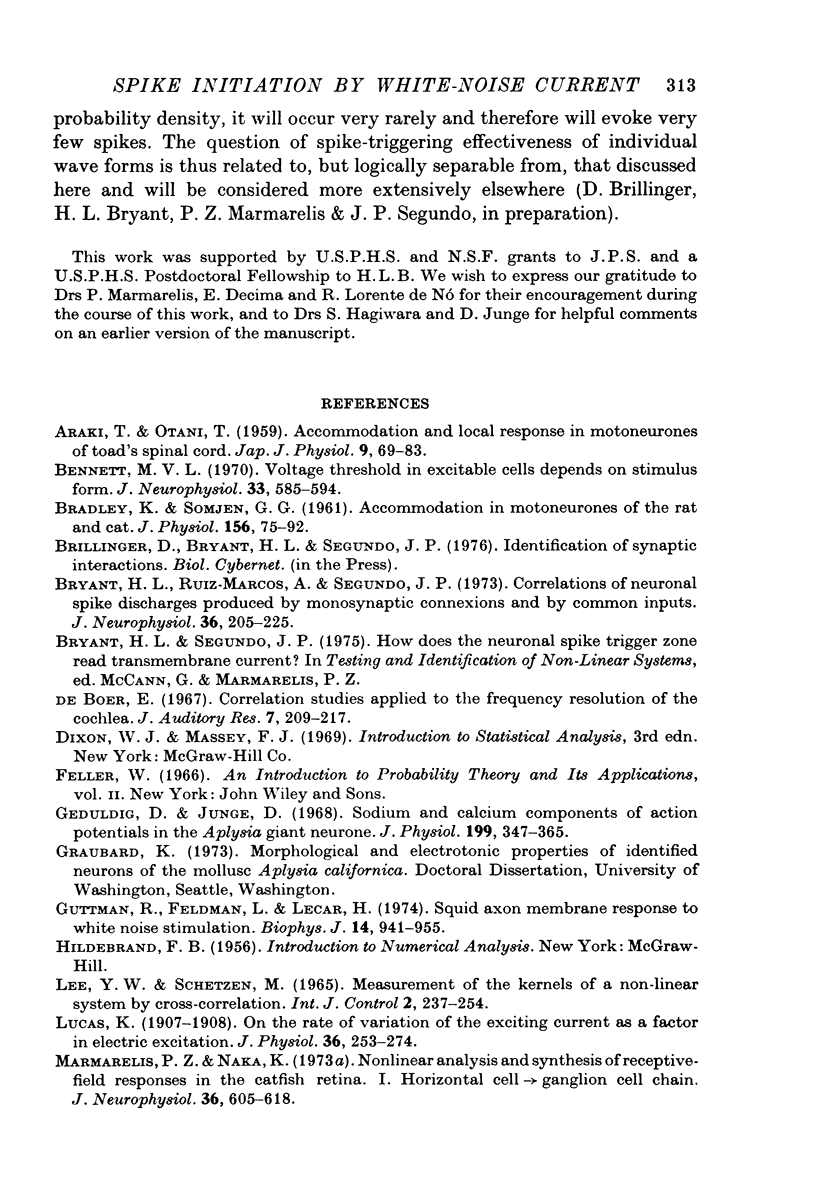
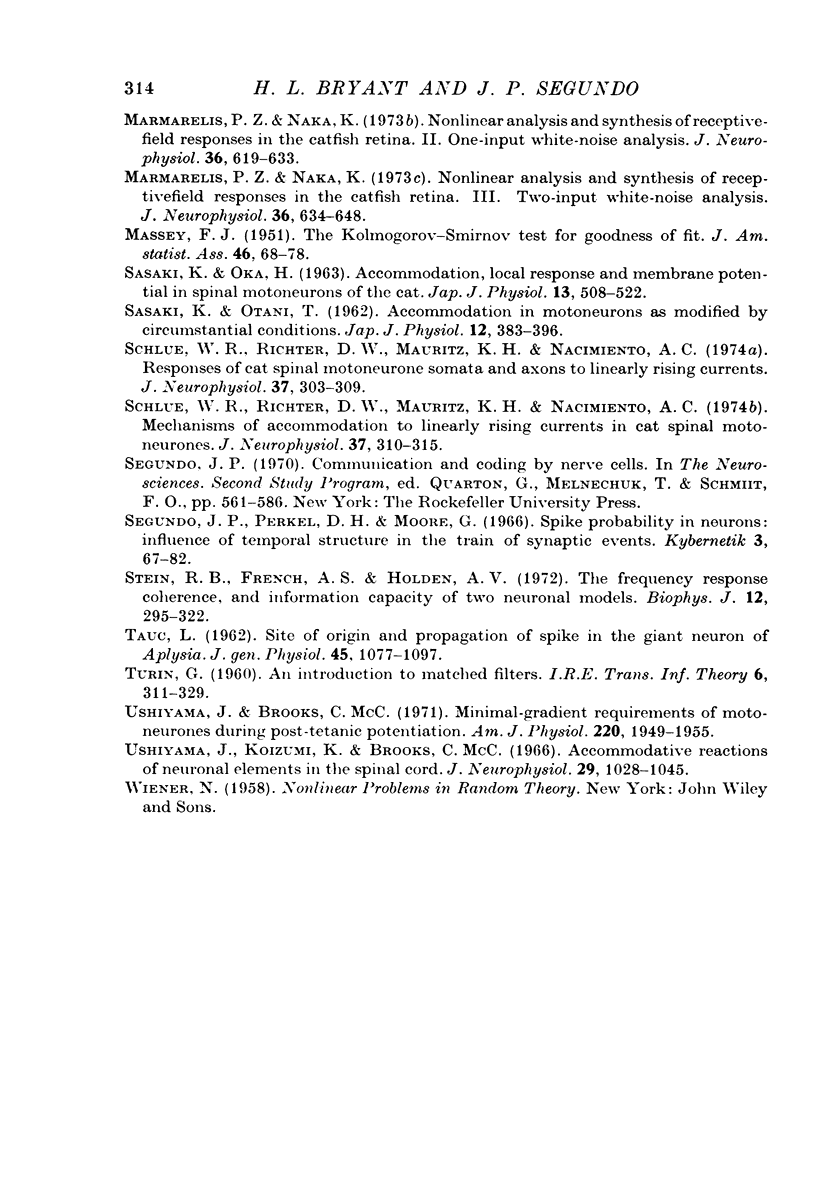
Selected References
These references are in PubMed. This may not be the complete list of references from this article.
- ARAKI T., OTANI T. Accommodation and local response in motoneurons of toad's spinal cord. Jpn J Physiol. 1959 Mar 25;9(1):69–83. doi: 10.2170/jjphysiol.9.69. [DOI] [PubMed] [Google Scholar]
- Bennett M. V., Hille B., Obara S. Voltage threshold in excitable cells depends on stimulus form. J Neurophysiol. 1970 Sep;33(5):585–594. doi: 10.1152/jn.1970.33.5.585. [DOI] [PubMed] [Google Scholar]
- Bradley K., Somjen G. G. Accommodation in motoneurones of the rat and the cat. J Physiol. 1961 Apr;156(1):75–92. doi: 10.1113/jphysiol.1961.sp006659. [DOI] [PMC free article] [PubMed] [Google Scholar]
- Bryant H. L., Jr, Marcos A. R., Segundo J. P. Correlations of neuronal spike discharges produced by monosynaptic connections and by common inputs. J Neurophysiol. 1973 Mar;36(2):205–225. doi: 10.1152/jn.1973.36.2.205. [DOI] [PubMed] [Google Scholar]
- Geduldig D., Junge D. Sodium and calcium components of action potentials in the Aplysia giant neurone. J Physiol. 1968 Dec;199(2):347–365. doi: 10.1113/jphysiol.1968.sp008657. [DOI] [PMC free article] [PubMed] [Google Scholar]
- Guttman R., Feldman L., Lecar H. Squid axon membrane response to white noise stimulation. Biophys J. 1974 Dec;14(12):941–955. doi: 10.1016/S0006-3495(74)85961-8. [DOI] [PMC free article] [PubMed] [Google Scholar]
- Lucas K. On the rate of variation of the exciting current as a factor in electric excitation. J Physiol. 1907 Dec 31;36(4-5):253–274. doi: 10.1113/jphysiol.1907.sp001231. [DOI] [PMC free article] [PubMed] [Google Scholar]
- Marmarelis P. Z., Naka K. I. Nonlinear analysis and synthesis of receptive-field responses in the catfish retina. 3. Two-input white-noise analysis. J Neurophysiol. 1973 Jul;36(4):634–648. doi: 10.1152/jn.1973.36.4.634. [DOI] [PubMed] [Google Scholar]
- Marmarelis P. Z., Naka K. I. Nonlinear analysis and synthesis of receptive-field responses in the catfish retina. I. Horizontal cell leads to ganglion cell chain. J Neurophysiol. 1973 Jul;36(4):605–618. doi: 10.1152/jn.1973.36.4.605. [DOI] [PubMed] [Google Scholar]
- Marmarelis P. Z., Naka K. I. Nonlinear analysis and synthesis of receptive-field responses in the catfish retina. II. One-input white-noise analysis. J Neurophysiol. 1973 Jul;36(4):619–633. doi: 10.1152/jn.1973.36.4.619. [DOI] [PubMed] [Google Scholar]
- SASAKI K., OKA H. ACCOMMODATION, LOCAL RESPONSE AND MEMBRANE POTENTIAL IN SPINAL MOTONEURONS OF THE CAT. Jpn J Physiol. 1963 Oct 15;13:508–522. doi: 10.2170/jjphysiol.13.508. [DOI] [PubMed] [Google Scholar]
- SASAKI K., OTANI T. Accommodation in motoneurons as modified by circumstantial conditions. Jpn J Physiol. 1962 Aug 15;12:383–396. doi: 10.2170/jjphysiol.12.383. [DOI] [PubMed] [Google Scholar]
- Schlue W. R., Richter D. W., Mauritz K. H., Nacimiento A. C. Mechanisms of accommodation to linearly rising currents in cat spinal motoneurons. J Neurophysiol. 1974 Mar;37(2):310–315. doi: 10.1152/jn.1974.37.2.310. [DOI] [PubMed] [Google Scholar]
- Schlue W. R., Richter D. W., Mauritz K. H., Nacimiento A. C. Responses of cat spinal motoneuron somata and axons to linearly rising currents. J Neurophysiol. 1974 Mar;37(2):303–309. doi: 10.1152/jn.1974.37.2.303. [DOI] [PubMed] [Google Scholar]
- Segundo J. P., Perkel D. H., Moore G. P. Spike probability in neurones: influence of temporal structure in the train of synaptic events. Kybernetik. 1966 May;3(2):67–82. doi: 10.1007/BF00299899. [DOI] [PubMed] [Google Scholar]
- Stein R. B., French A. S., Holden A. V. The frequency response, coherence, and information capacity of two neuronal models. Biophys J. 1972 Mar;12(3):295–322. doi: 10.1016/S0006-3495(72)86087-9. [DOI] [PMC free article] [PubMed] [Google Scholar]
- TAUC L. Site of origin and propagation in spike in the giant neuron of Aplysia. J Gen Physiol. 1962 Jul;45:1077–1097. doi: 10.1085/jgp.45.6.1077. [DOI] [PMC free article] [PubMed] [Google Scholar]
- Ushiyama J., Brooks C. M. Minimal-gradient requirements of motoneurons during posttetanic potentiation. Am J Physiol. 1971 Jun;220(6):1949–1955. doi: 10.1152/ajplegacy.1971.220.6.1949. [DOI] [PubMed] [Google Scholar]
- Ushiyama J., Koizumi K., Brooks C. M. Accommodative reactions of neuronal elements in the spinal cord. J Neurophysiol. 1966 Nov;29(6):1028–1045. doi: 10.1152/jn.1966.29.6.1028. [DOI] [PubMed] [Google Scholar]