Abstract
1. An intracellular voltage clamp in conjunction with a patch pipette utilizing feed-back to monitor local current from the soma membrane were used to analyse transient and stationary currents in bursting pacemaker neurones in Helix pomatia and H. levantina. 2. A weak, net inward current flows during small (less than or equal 20 mV) depolarizations. This current exhibits slow activation kinetics, persistence during prolonged depolarization, and slow turning off at end of depolarization. Consequently, the steady-state current-voltage curve exhibits a region of negative resistance from about -55 to -35 mV. 3. The slow inward current and the negative resistance characteristic are rapidly and completely abolished by substitution of Co2+ or La3+ for Ca2+ and are partially blocked by the Ca-blocking drug D-600. Substitution of Tris or glucose for Na+ significantly reduces the inward current only after 15-20 min exposure, recovery being equally slow. 4. The inward current and the negative resistance characteristic of the I-V curve are greatly enhanced by Ba2+ substitution for Ca2+. This is ascribed in part to Ba2+ carrying current through the slow inward current channels and in part to a suppression of the late K+ current by Ba2+. 5. The inward current is also present in many non-bursting neurones but fails to appear as a net inward current due to short circuiting by a leakage current or by the delayed potassium current. In these cells the slow inward current contributes to inward going rectification. Replacement of Ca2+ with Ba2+ enhances the current so as to produce a net inward current during small depolarizations in these neurones. 6. It is concluded that the slow inward current is carried primarily by Ca2+ in the soma membrane of bursting pace-maker neurones and a number of non-bursting cells examined in the parietal ganglion of Helix. 7. The sensitivity to small depolarizations and persistence during prolonged depolarization suggests two roles for the Ca system in the generation of slow pace-maker oscillations. In this model the Ca system contributes to the slow depolarization which constitutes the onset of the pace-maker wave, and also contributes to the increment in [Ca] in which activates the Ca-sensitive K+ conductance responsible for repolarization. The inhibition of spontaneous bursting by Ca-blocking agents supports this model.
Full text
PDF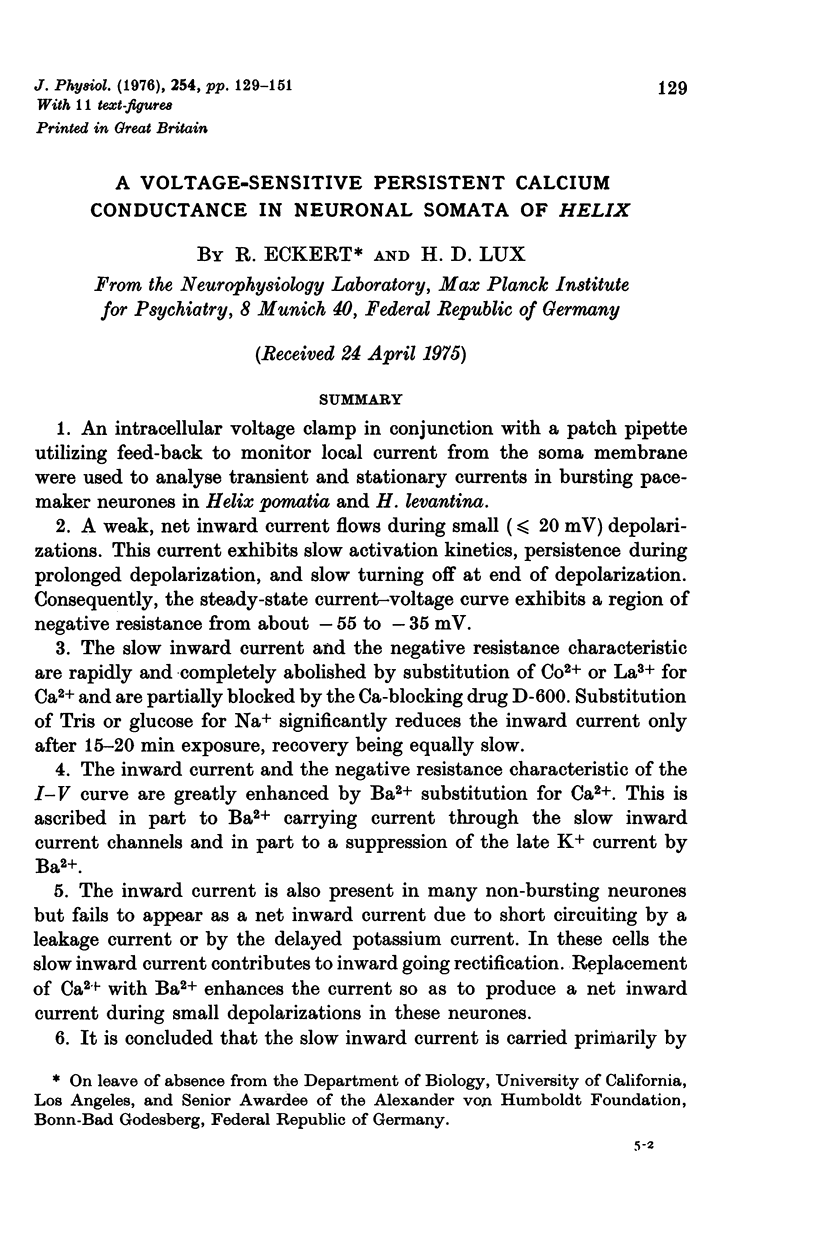
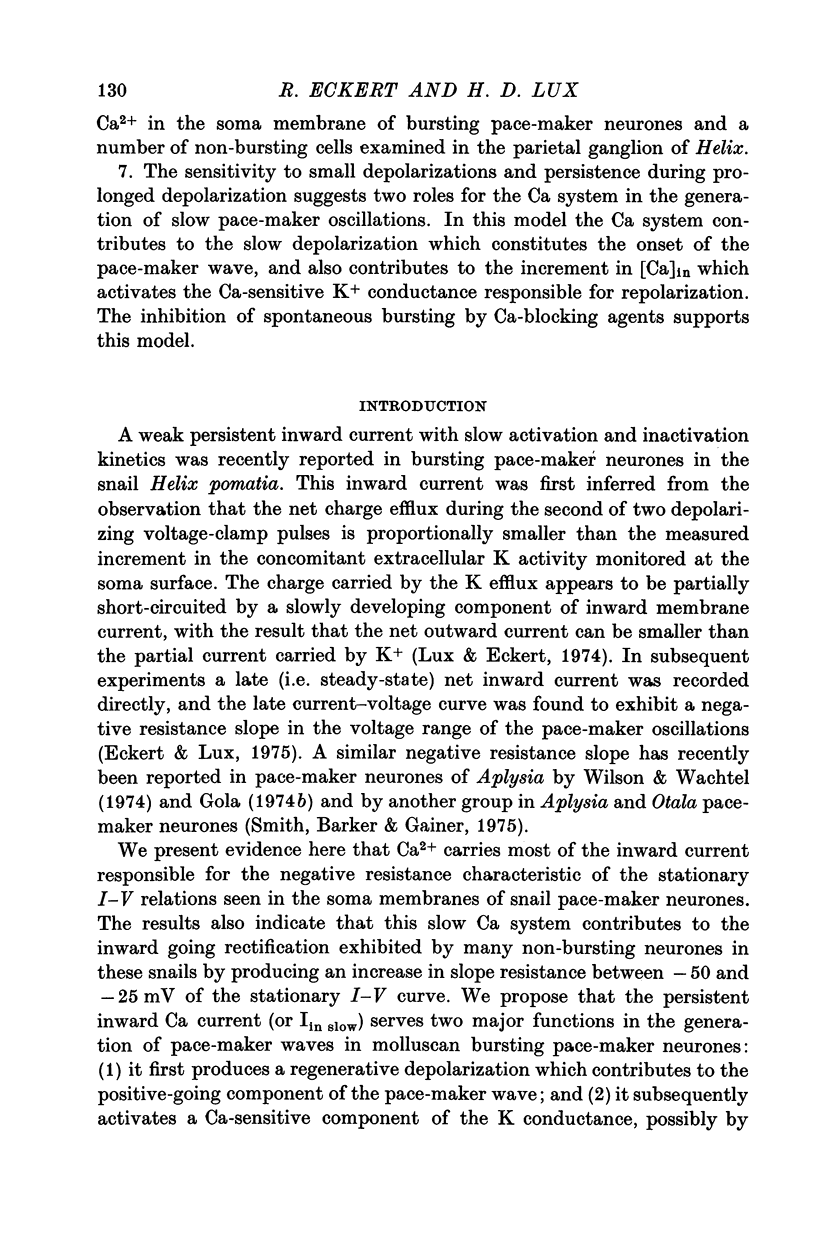
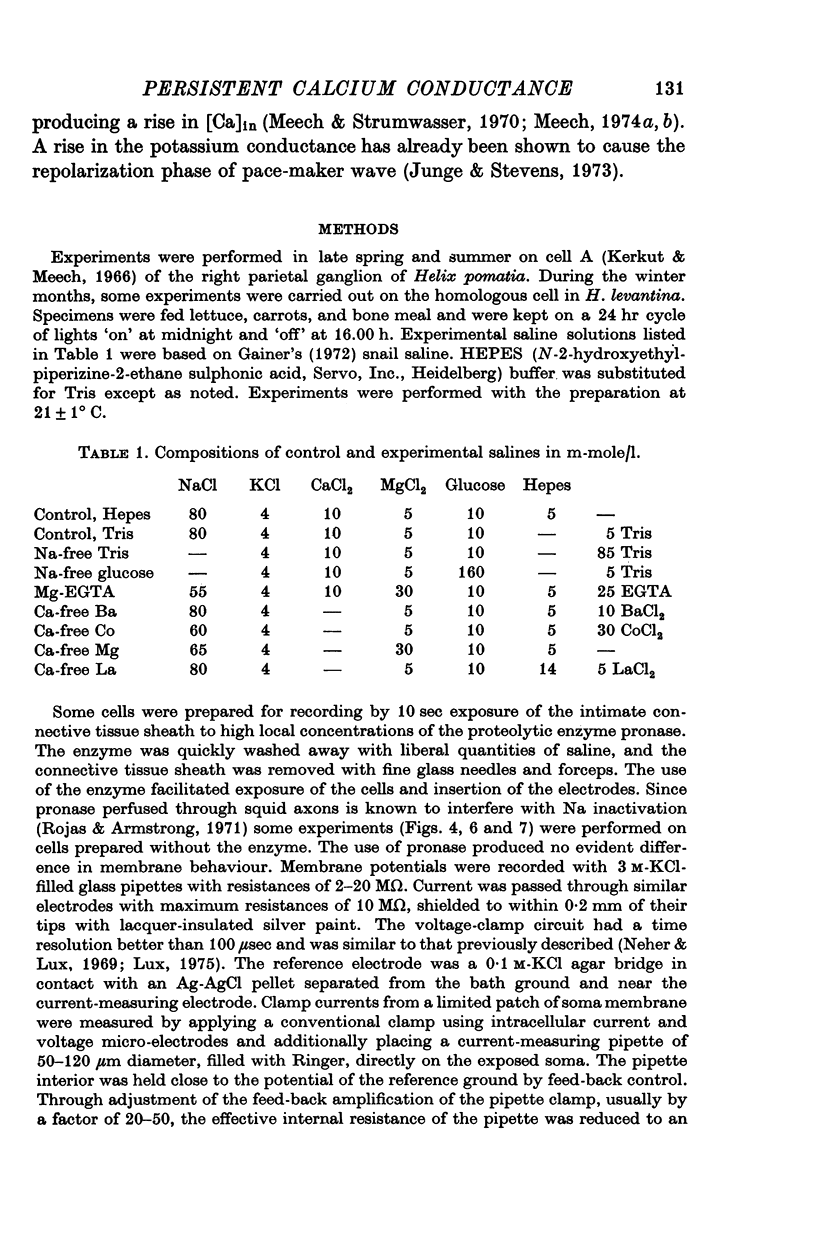
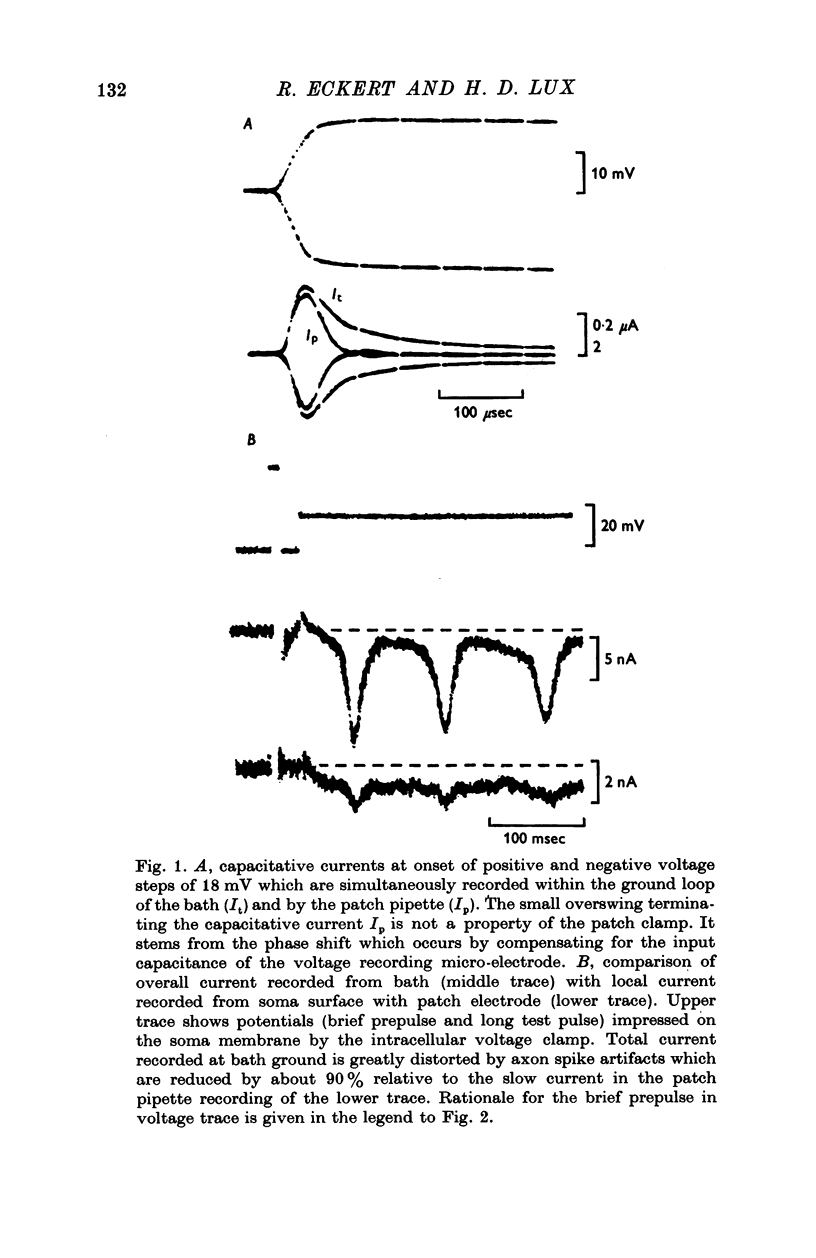
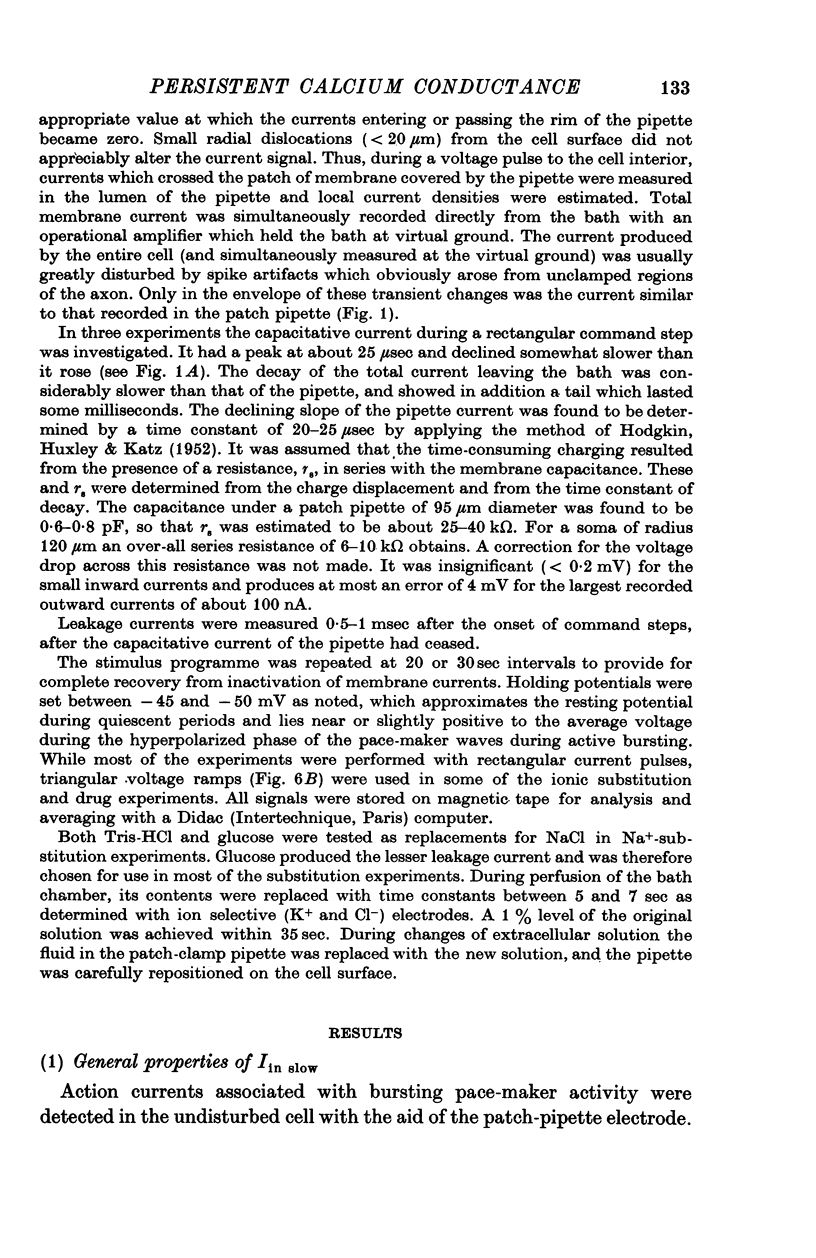
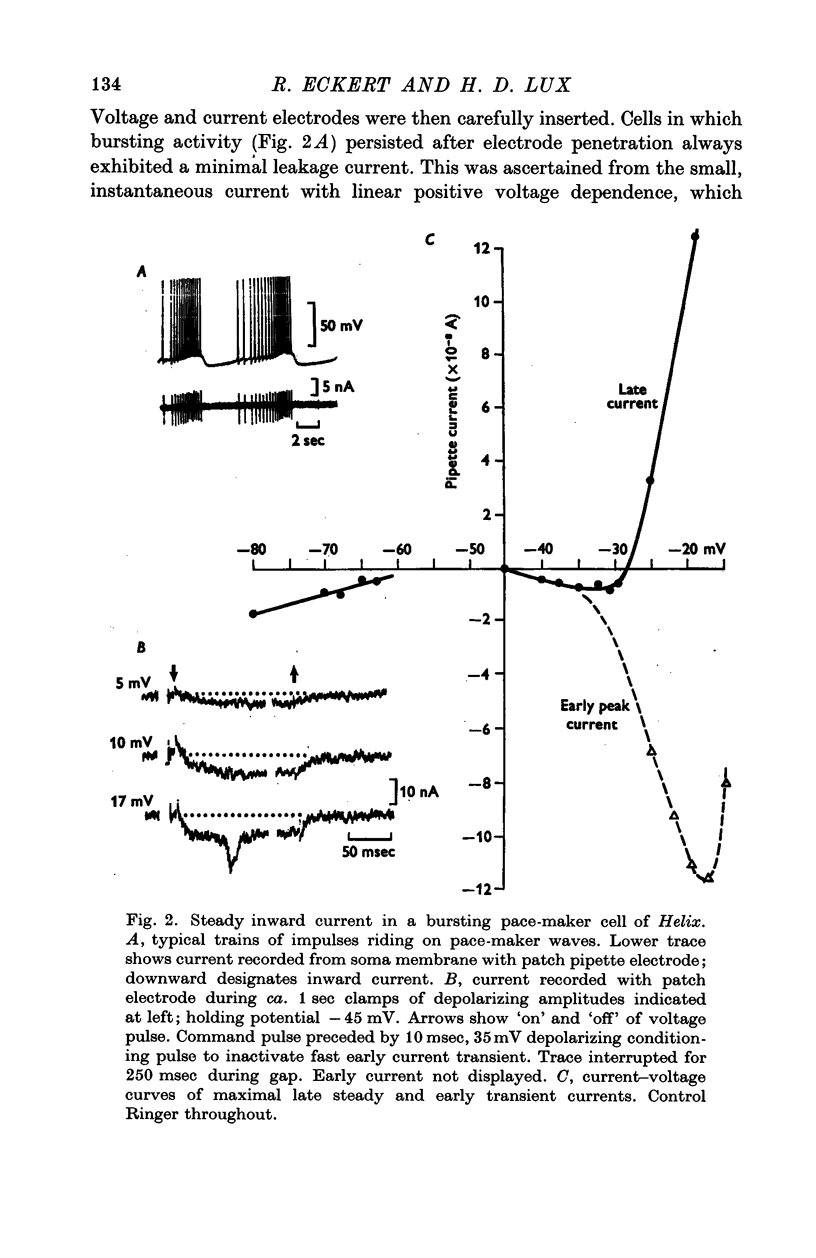
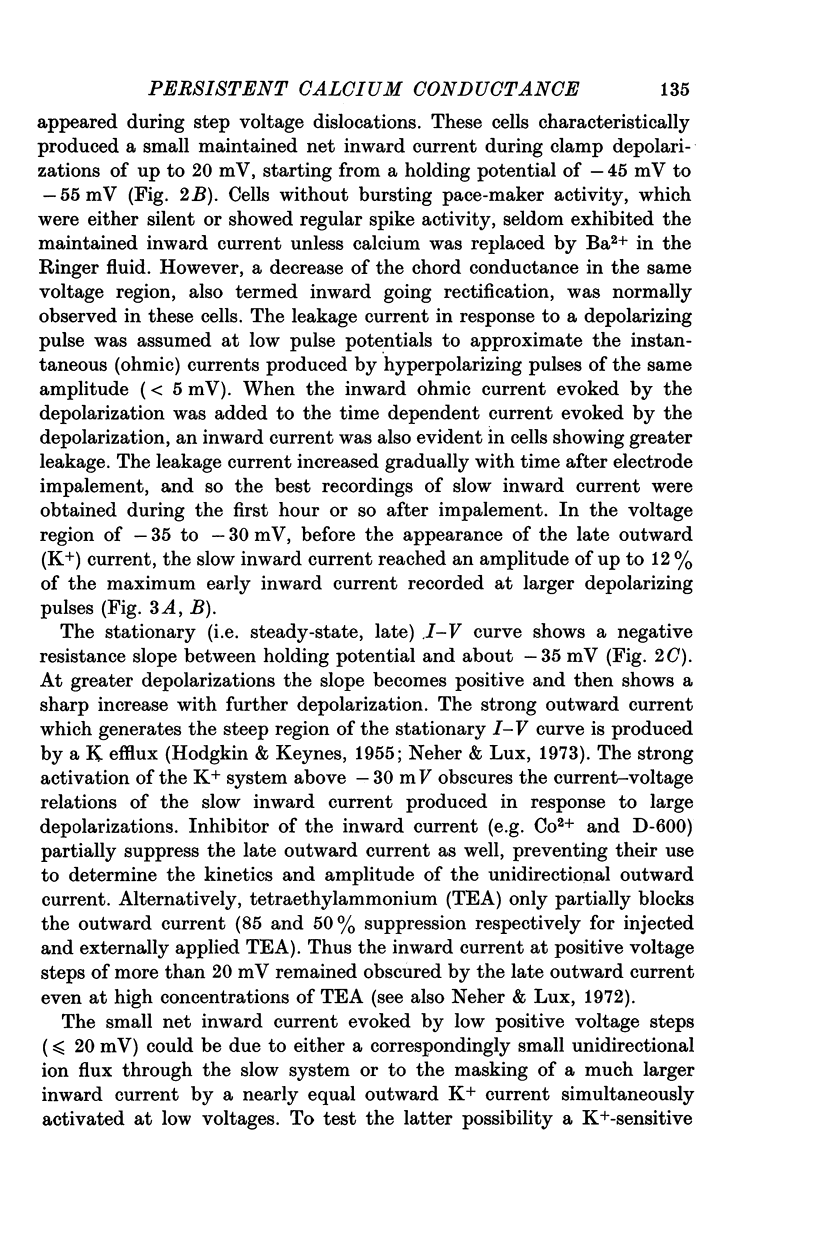
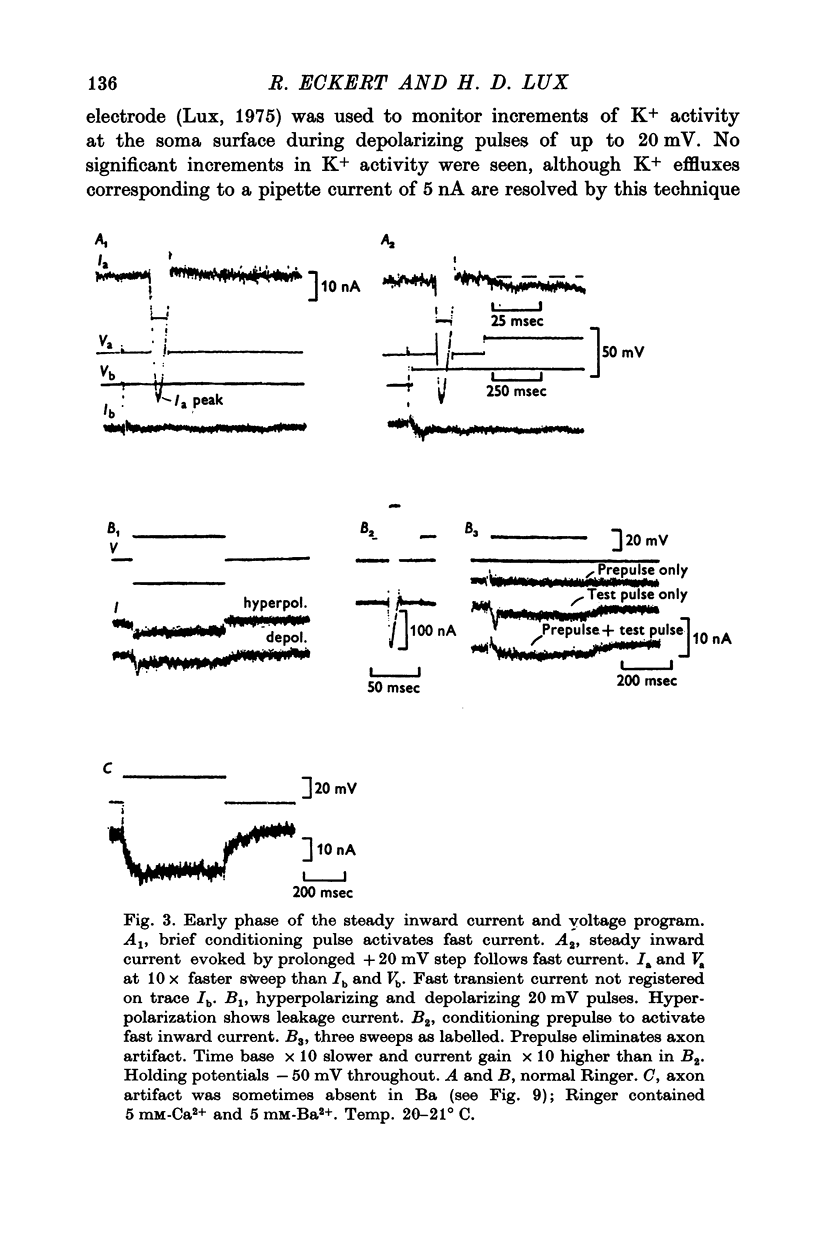
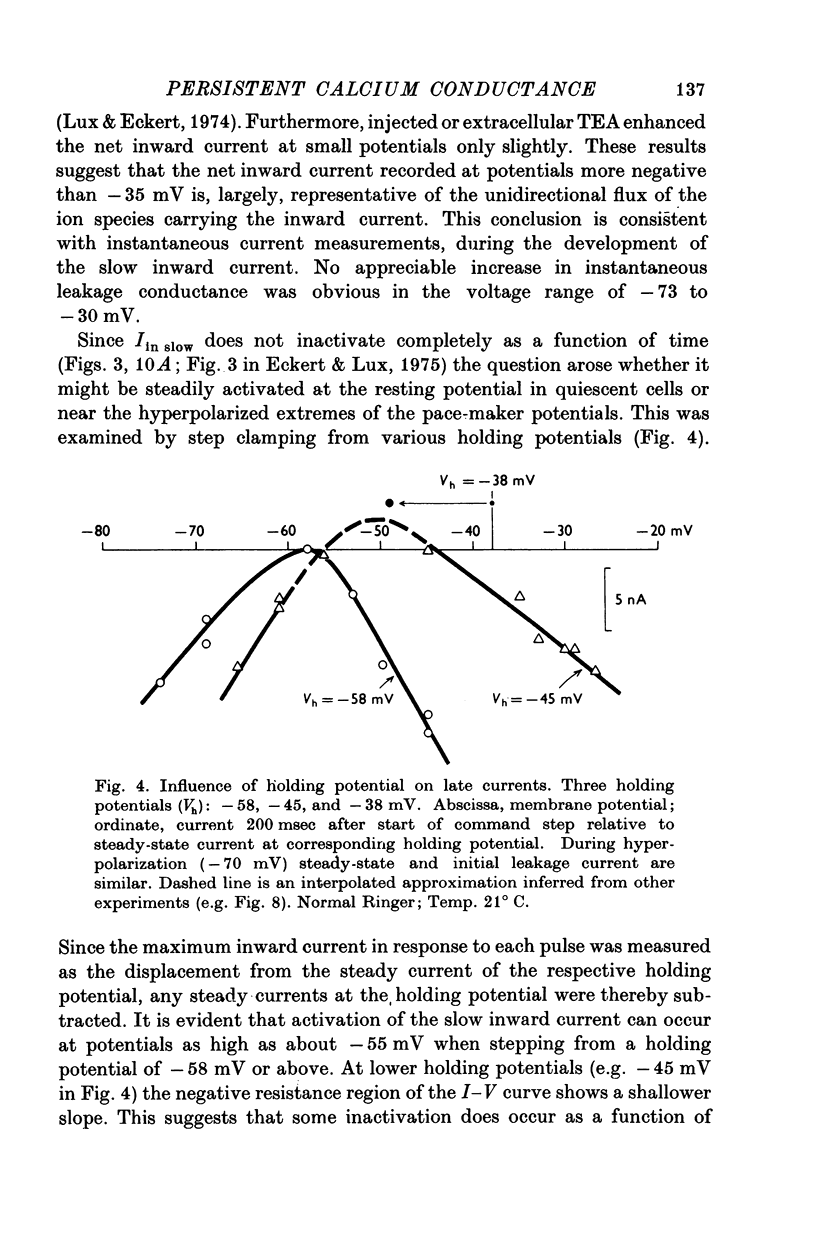
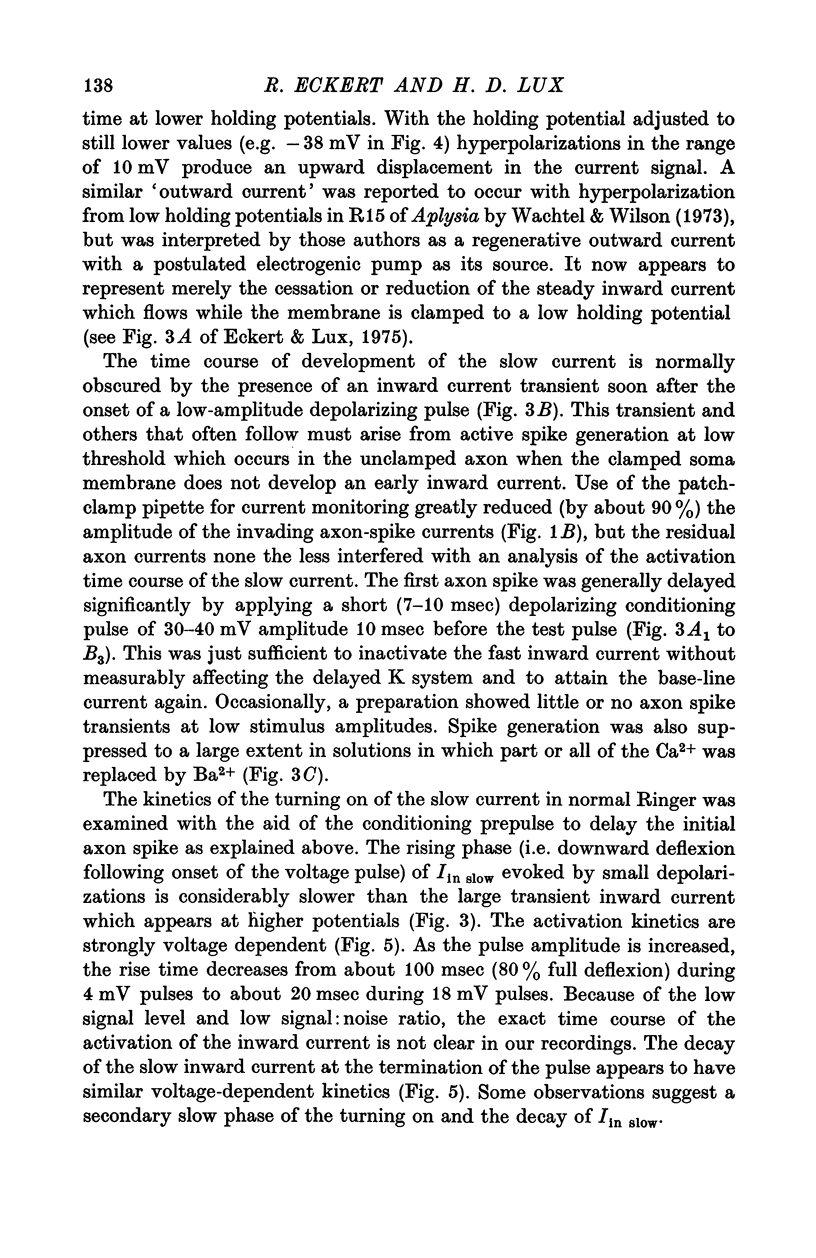
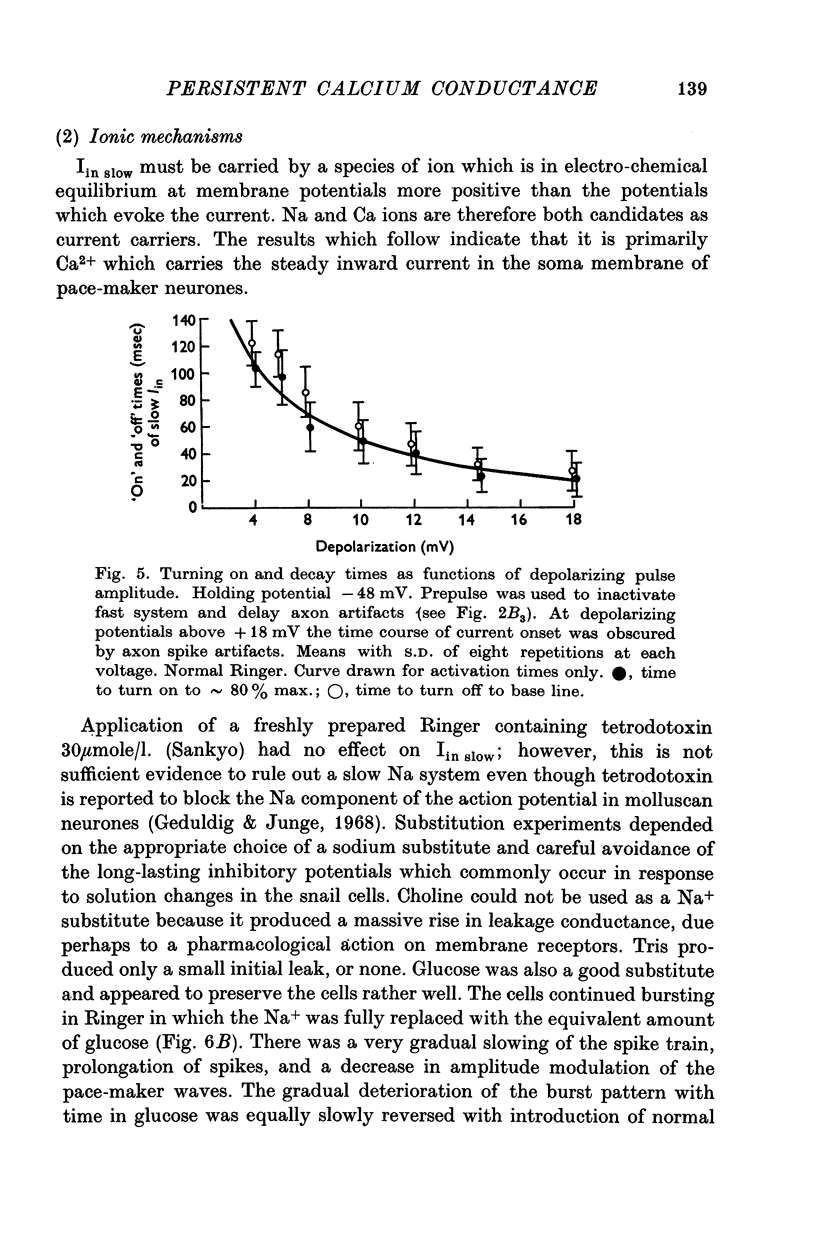
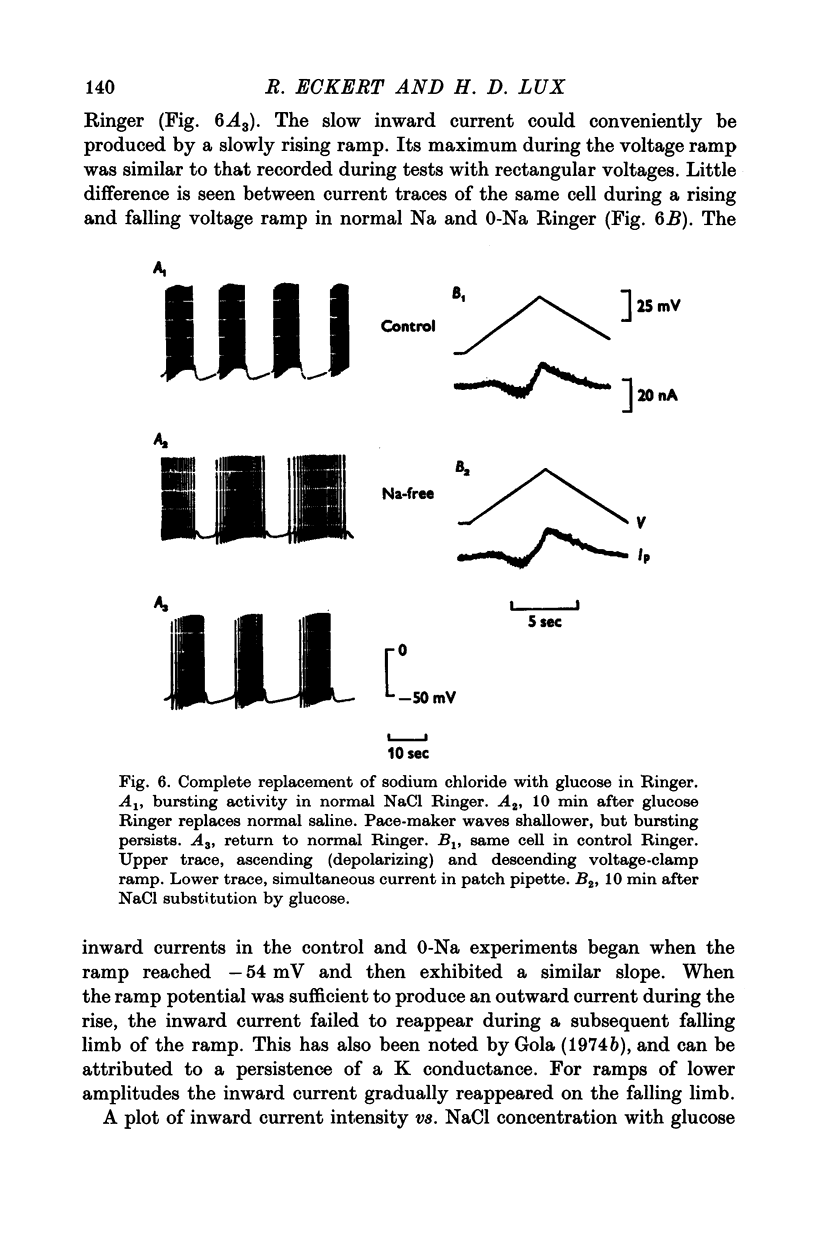
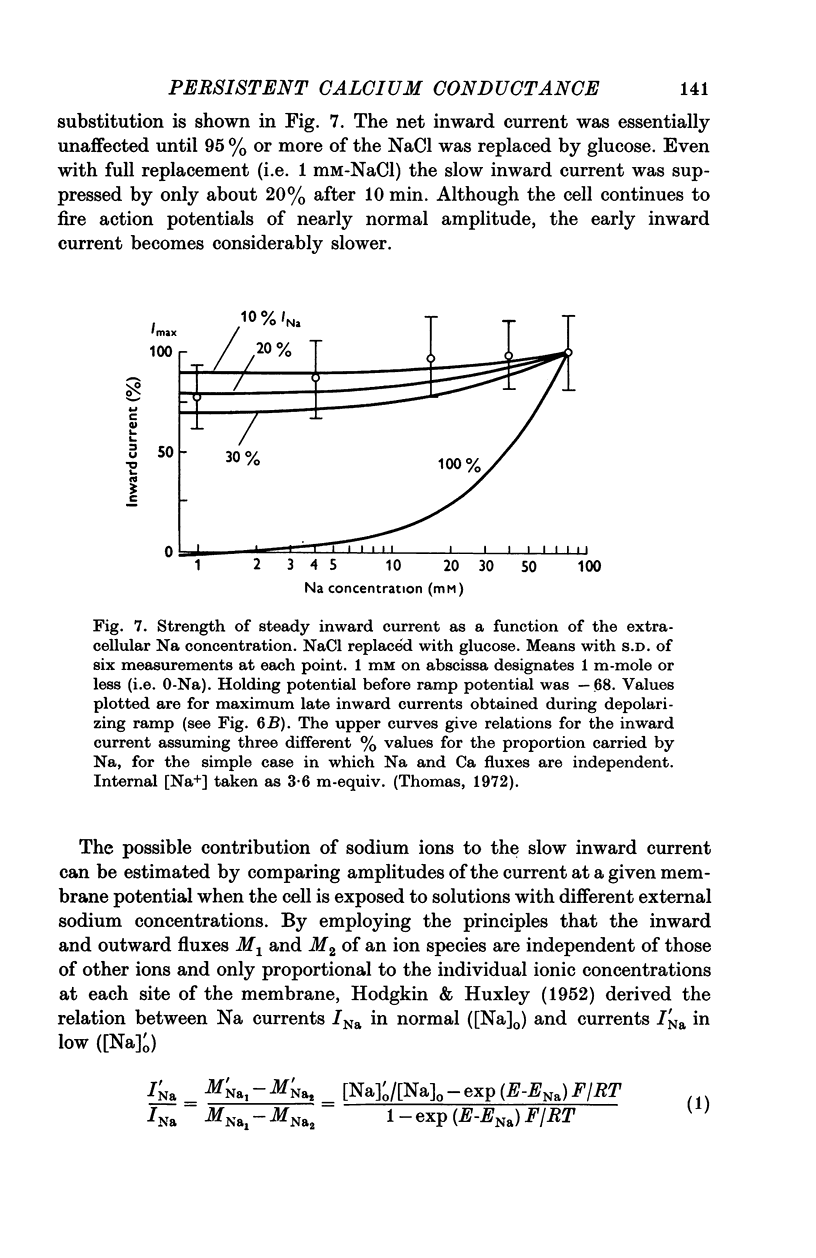
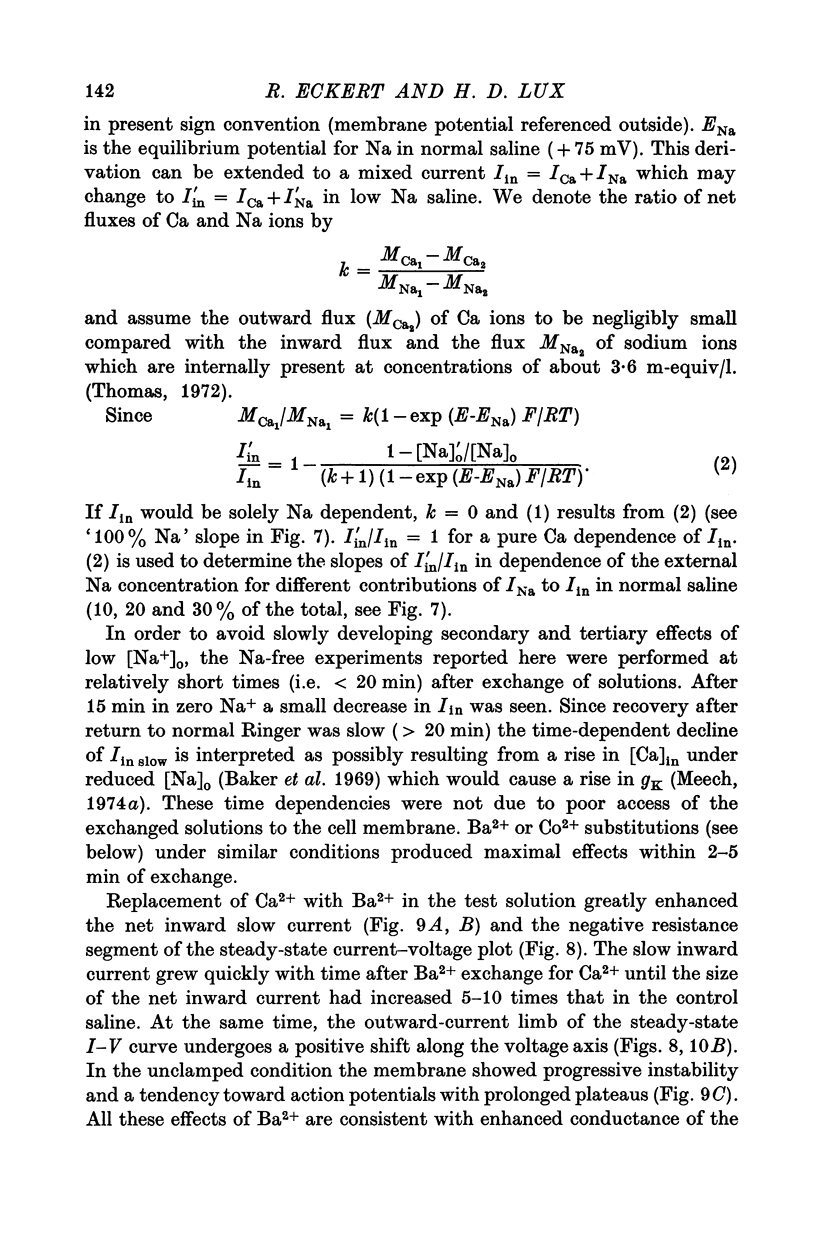
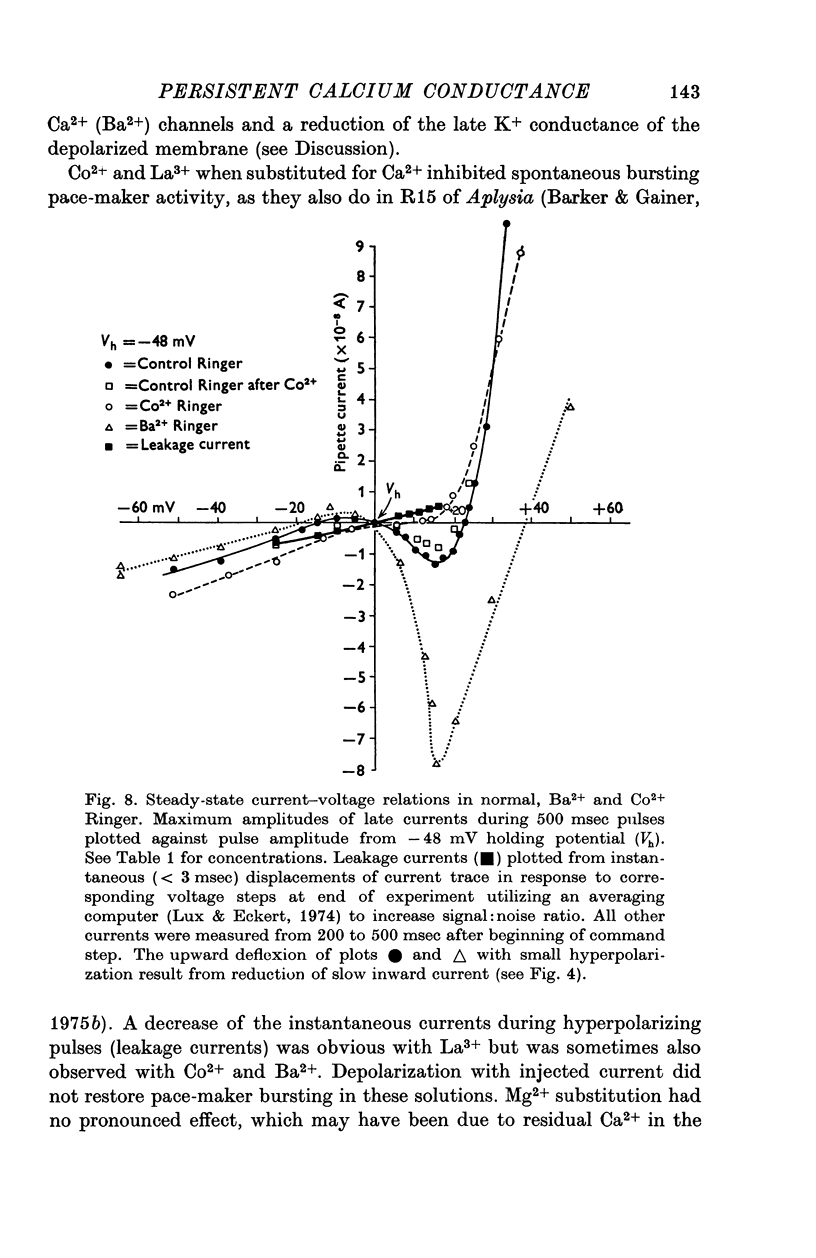
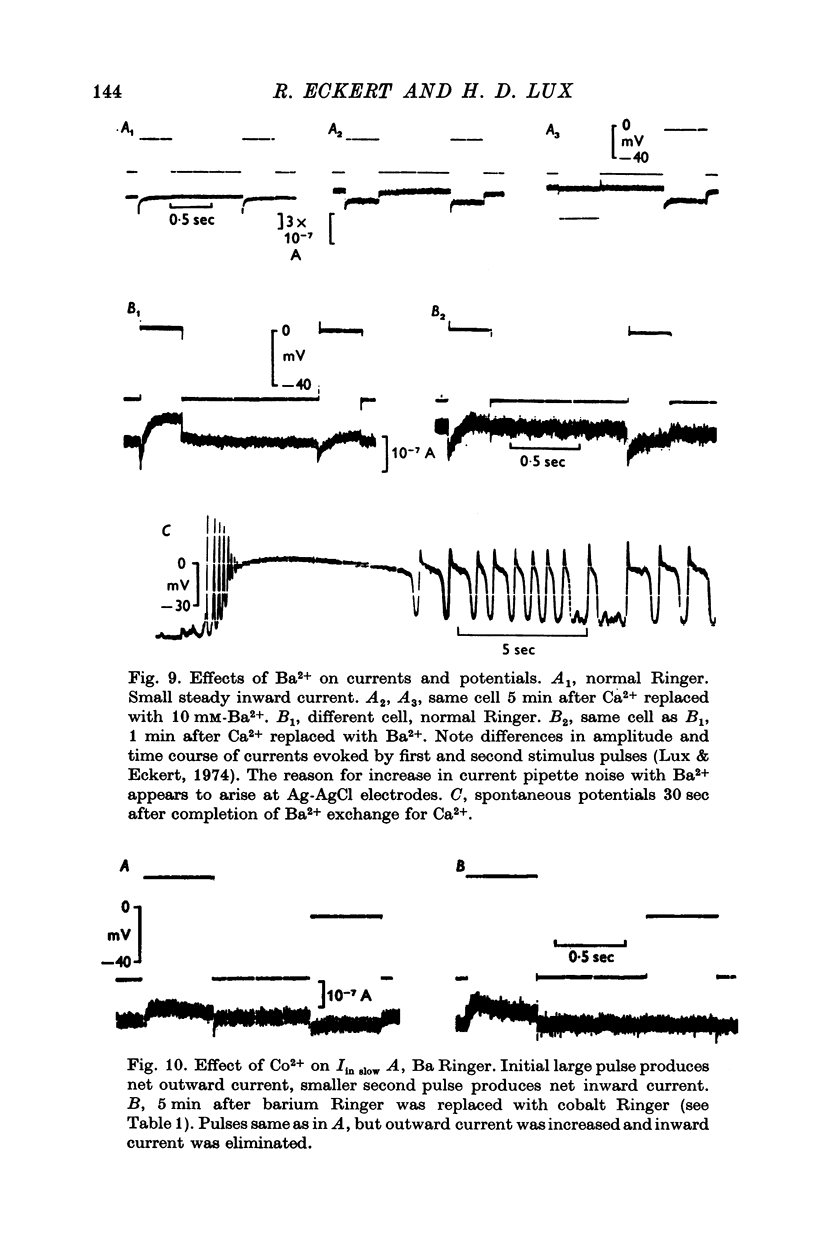
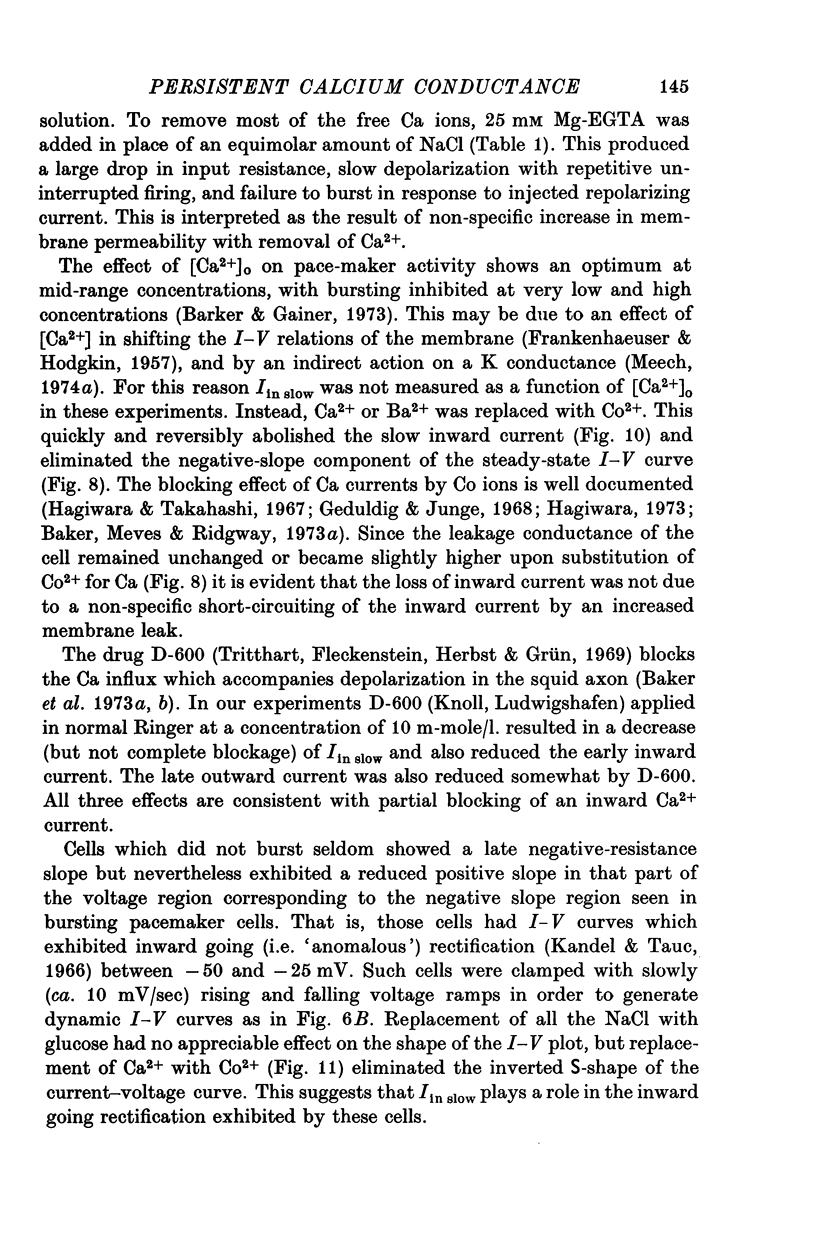
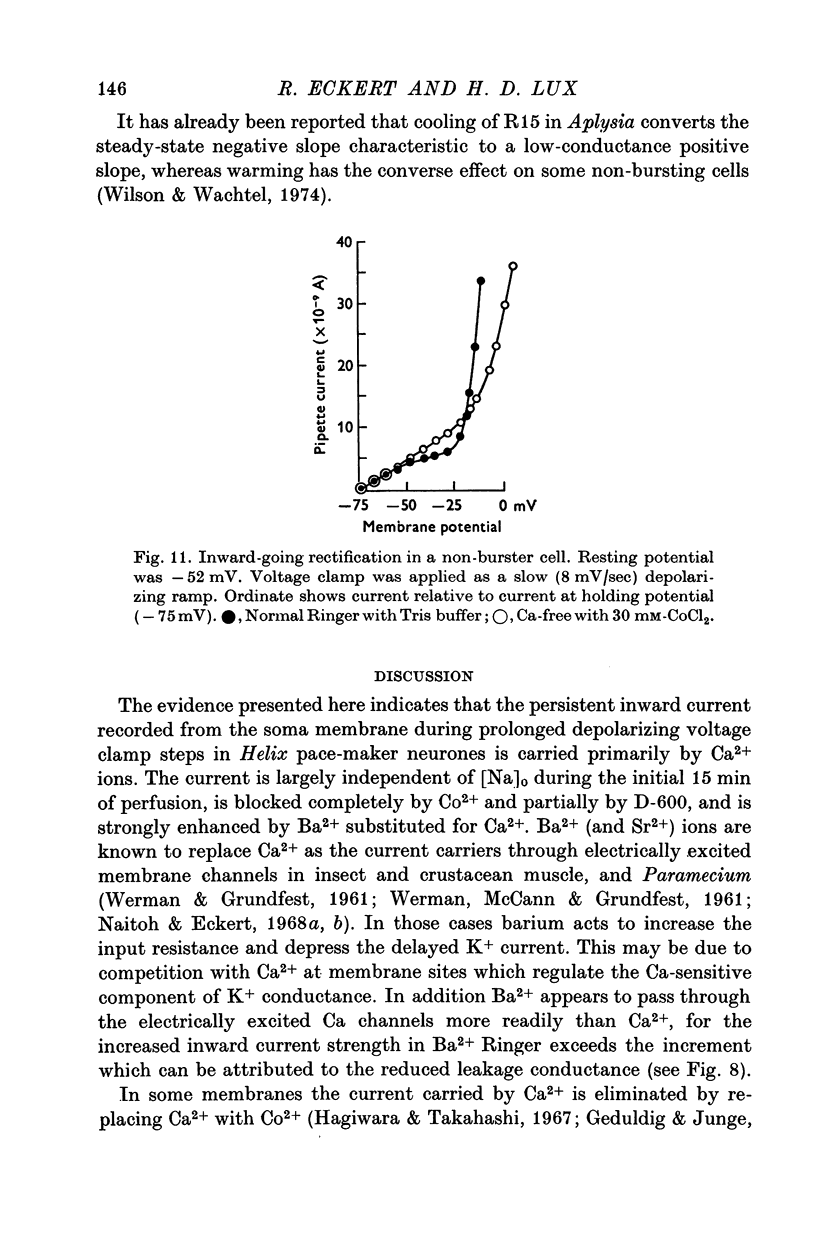
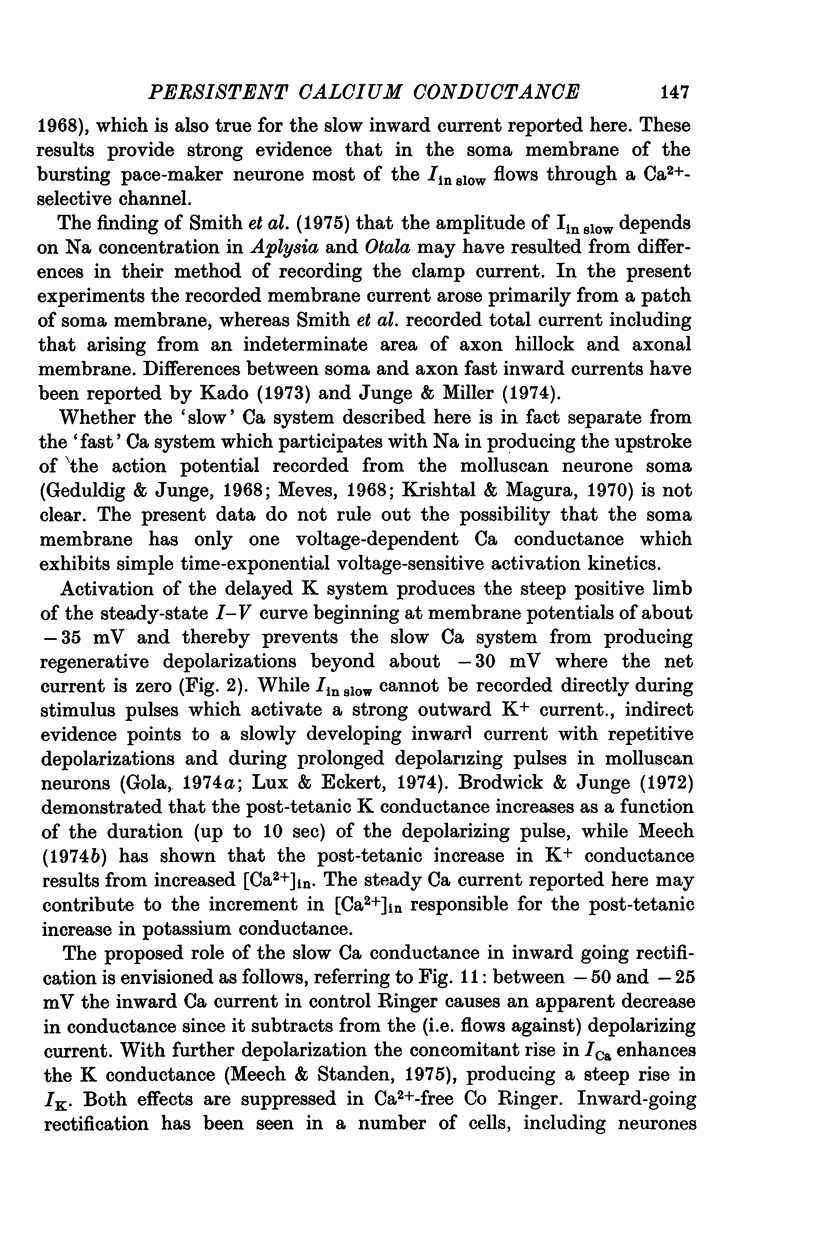
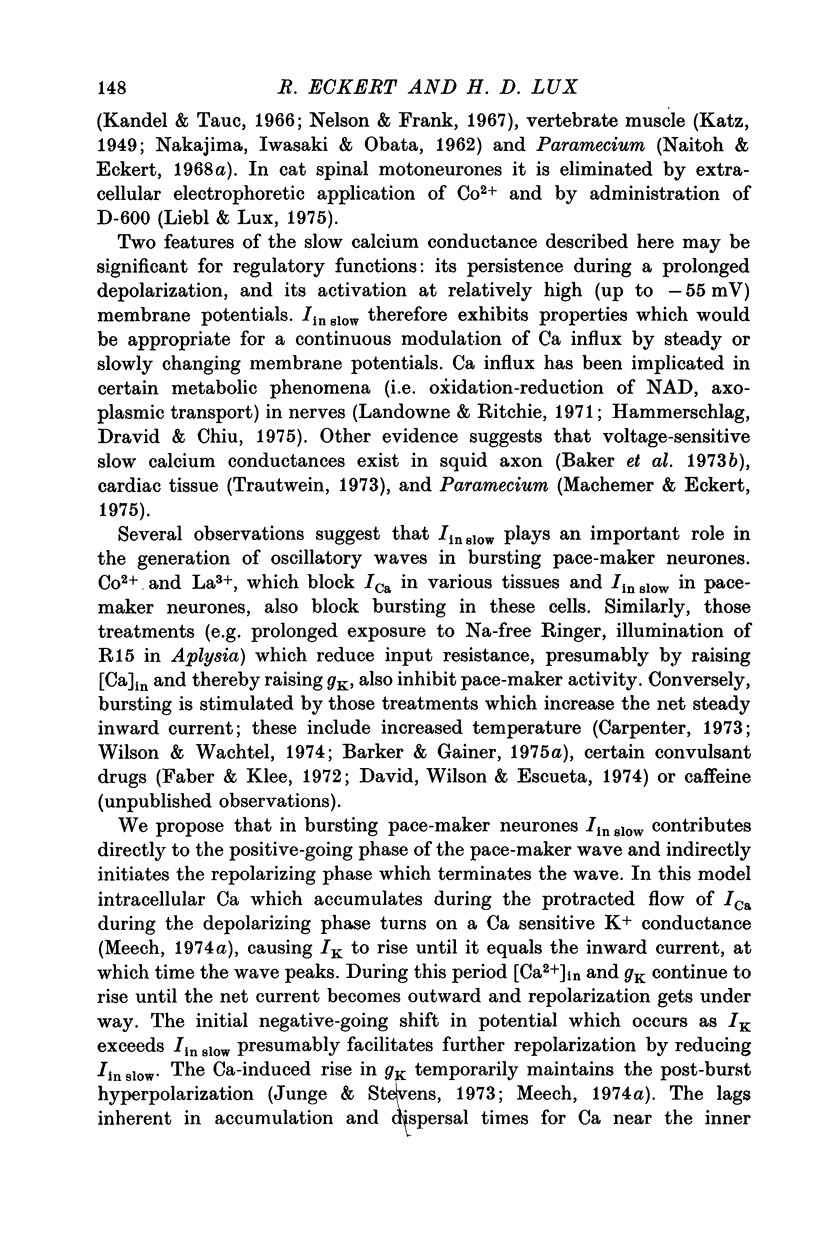
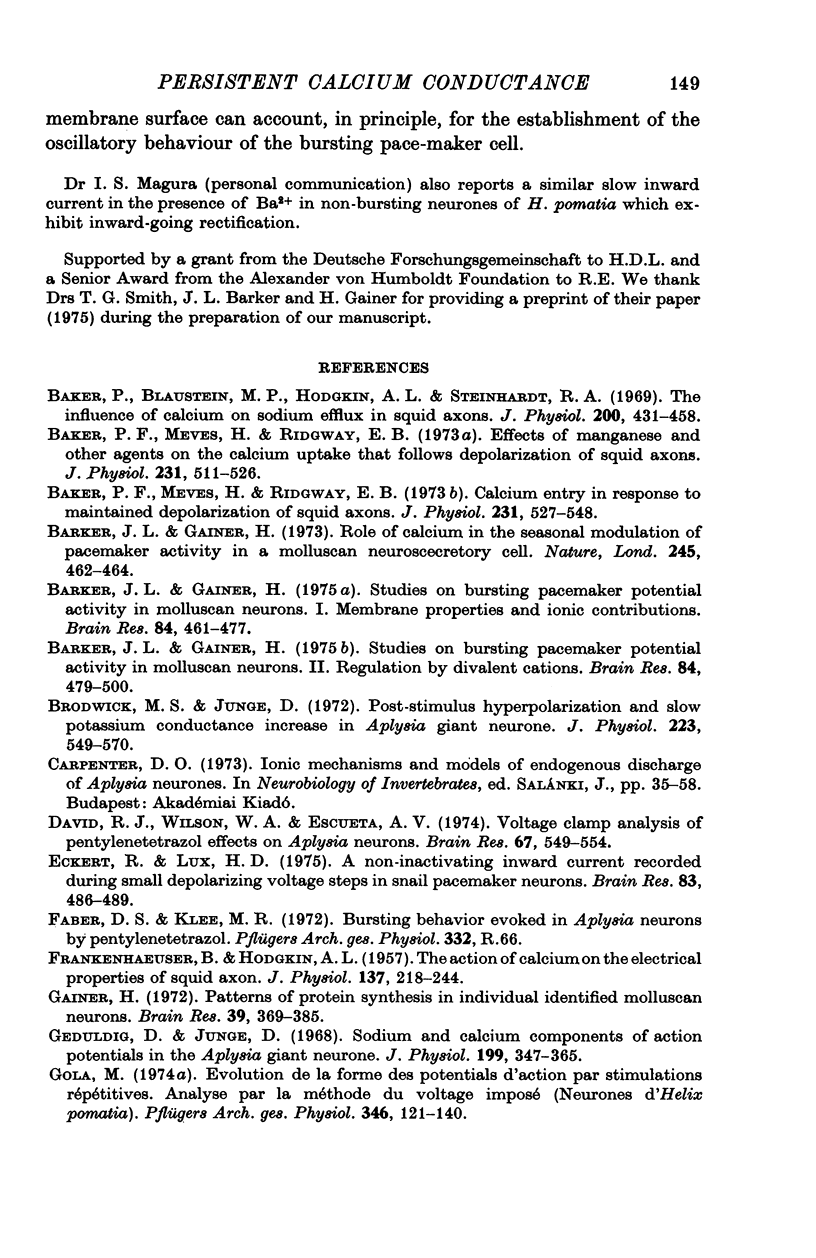
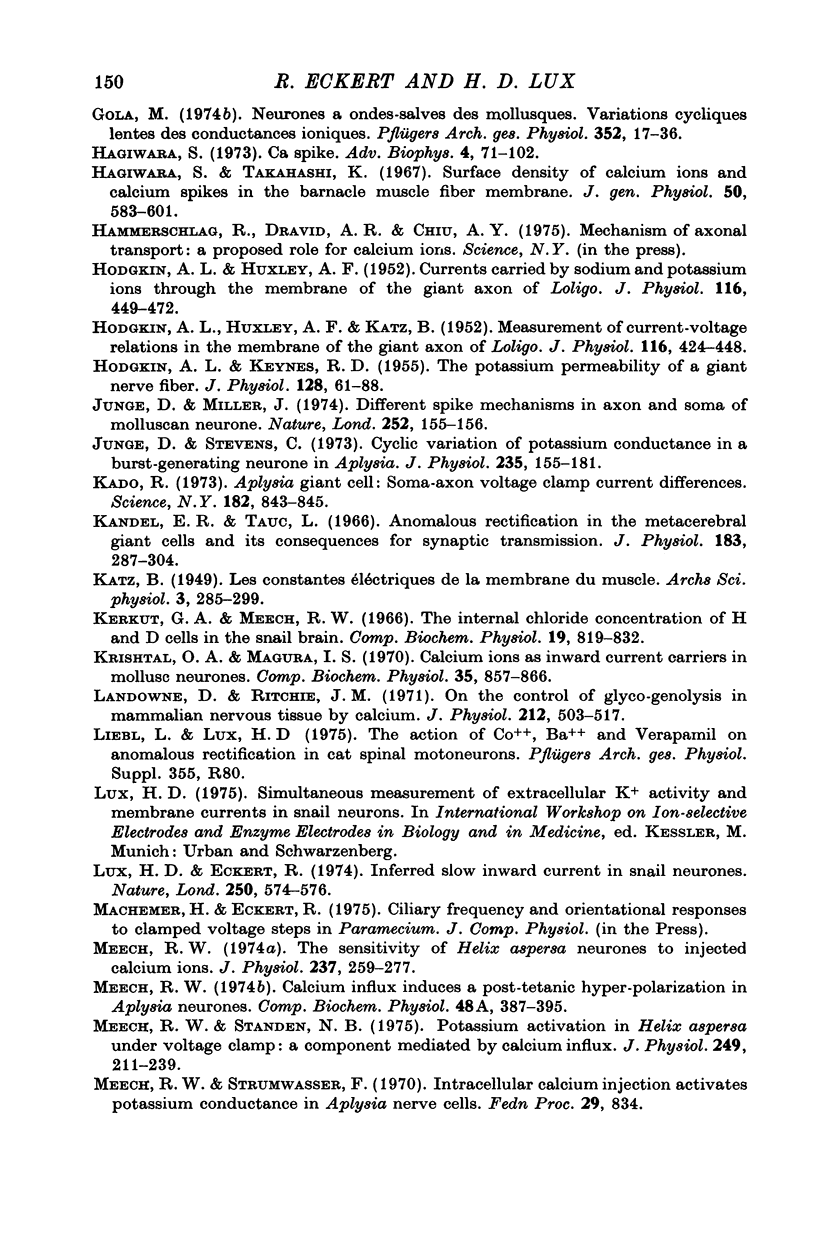
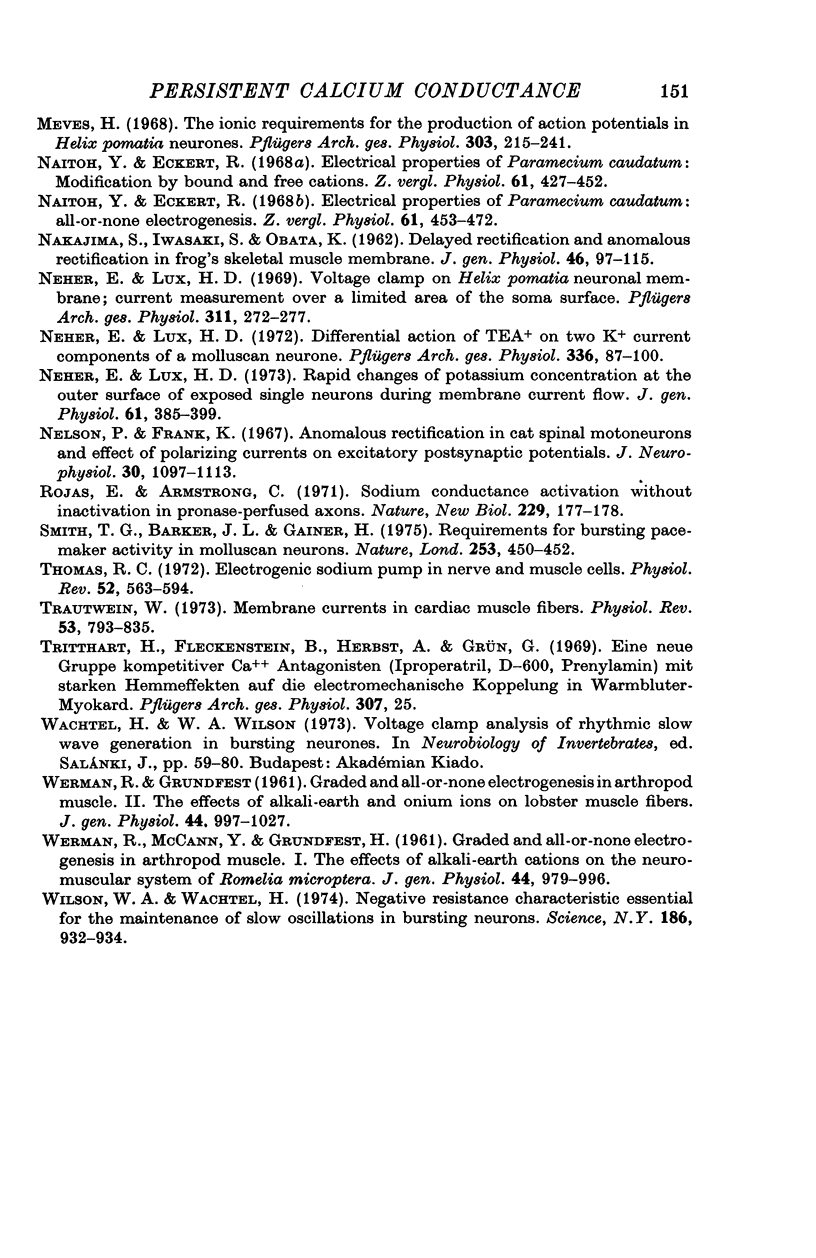
Selected References
These references are in PubMed. This may not be the complete list of references from this article.
- Baker P. F., Blaustein M. P., Hodgkin A. L., Steinhardt R. A. The influence of calcium on sodium efflux in squid axons. J Physiol. 1969 Feb;200(2):431–458. doi: 10.1113/jphysiol.1969.sp008702. [DOI] [PMC free article] [PubMed] [Google Scholar]
- Baker P. F., Meves H., Ridgway E. B. Calcium entry in response to maintained depolarization of squid axons. J Physiol. 1973 Jun;231(3):527–548. doi: 10.1113/jphysiol.1973.sp010247. [DOI] [PMC free article] [PubMed] [Google Scholar]
- Baker P. F., Meves H., Ridgway E. B. Effects of manganese and other agents on the calcium uptake that follows depolarization of squid axons. J Physiol. 1973 Jun;231(3):511–526. doi: 10.1113/jphysiol.1973.sp010246. [DOI] [PMC free article] [PubMed] [Google Scholar]
- Barker J. L., Gainer H. Role of calcium in the seasonal modulation of pacemaker activity in a molluscan neurosecretory cell. Nature. 1973 Oct 26;245(5426):462–464. doi: 10.1038/245462b0. [DOI] [PubMed] [Google Scholar]
- Barker J. L., Gainer H. Studies on bursting pacemaker potential activity in molluscan neurons. I. Membrane properties and ionic contributions. Brain Res. 1975 Feb 14;84(3):461–477. doi: 10.1016/0006-8993(75)90766-0. [DOI] [PubMed] [Google Scholar]
- Barker J. L., Gainer H. Studies on bursting pacemaker potential activity in molluscan neurons. II. Regulations by divalanet cations. Brain Res. 1975 Feb 14;84(3):479–500. doi: 10.1016/0006-8993(75)90767-2. [DOI] [PubMed] [Google Scholar]
- Brodwick M. S., Junge D. Post-stimulus hyperpolarization and slow potassium conductance increase in Aplysia giant neurone. J Physiol. 1972 Jun;223(2):549–570. doi: 10.1113/jphysiol.1972.sp009862. [DOI] [PMC free article] [PubMed] [Google Scholar]
- David R. J., Wilson W. A., Escueta A. V. Voltage clamp analysis of pentylenetetrazol effects on Aplysia neurons. Brain Res. 1974 Mar 8;67(3):549–554. doi: 10.1016/0006-8993(74)90505-8. [DOI] [PubMed] [Google Scholar]
- Eckert R., Lux H. D. A non-inactivating inward current recorded during small depolarizing voltage steps in snail pacemaker neurons. Brain Res. 1975 Jan 17;83(3):486–489. doi: 10.1016/0006-8993(75)90840-9. [DOI] [PubMed] [Google Scholar]
- Gainer H. Patterns of protein synthesis in individual, identified molluscan neurons. Brain Res. 1972 Apr 28;39(2):369–385. doi: 10.1016/0006-8993(72)90442-8. [DOI] [PubMed] [Google Scholar]
- Geduldig D., Junge D. Sodium and calcium components of action potentials in the Aplysia giant neurone. J Physiol. 1968 Dec;199(2):347–365. doi: 10.1113/jphysiol.1968.sp008657. [DOI] [PMC free article] [PubMed] [Google Scholar]
- Gola M. Evolution de la frome des potentiels d'action par stimulations répétitives. Analyse par la méthode du voltage imposé (neurones d'Helix pomatia. Pflugers Arch. 1974 Feb 4;346(2):121–140. doi: 10.1007/BF00587012. [DOI] [PubMed] [Google Scholar]
- Gola M. Neurones à ondes-salves des mollusques. Variations cycliques lentes des conductances ioniques. Pflugers Arch. 1974;352(1):17–36. doi: 10.1007/BF01061947. [DOI] [PubMed] [Google Scholar]
- HODGKIN A. L., HUXLEY A. F. Currents carried by sodium and potassium ions through the membrane of the giant axon of Loligo. J Physiol. 1952 Apr;116(4):449–472. doi: 10.1113/jphysiol.1952.sp004717. [DOI] [PMC free article] [PubMed] [Google Scholar]
- HODGKIN A. L., HUXLEY A. F., KATZ B. Measurement of current-voltage relations in the membrane of the giant axon of Loligo. J Physiol. 1952 Apr;116(4):424–448. doi: 10.1113/jphysiol.1952.sp004716. [DOI] [PMC free article] [PubMed] [Google Scholar]
- HODGKIN A. L., KEYNES R. D. The potassium permeability of a giant nerve fibre. J Physiol. 1955 Apr 28;128(1):61–88. doi: 10.1113/jphysiol.1955.sp005291. [DOI] [PMC free article] [PubMed] [Google Scholar]
- Hagiwara S. Ca spike. Adv Biophys. 1973;4:71–102. [PubMed] [Google Scholar]
- Hagiwara S., Takahashi K. Surface density of calcium ions and calcium spikes in the barnacle muscle fiber membrane. J Gen Physiol. 1967 Jan;50(3):583–601. doi: 10.1085/jgp.50.3.583. [DOI] [PMC free article] [PubMed] [Google Scholar]
- Junge D., Miller J. Different spike mechanisms in axon and soma of molluscan neurone. Nature. 1974 Nov 8;252(5479):155–156. doi: 10.1038/252155a0. [DOI] [PubMed] [Google Scholar]
- Junge D., Stephens C. L. Cyclic variation of potassium conductance in a burst-generating neurone in Aplysia. J Physiol. 1973 Nov;235(1):155–181. doi: 10.1113/jphysiol.1973.sp010382. [DOI] [PMC free article] [PubMed] [Google Scholar]
- Kado R. T. Aplysia giant cell: soma-axon voltage clamp current differences. Science. 1973 Nov 23;182(4114):843–845. doi: 10.1126/science.182.4114.843. [DOI] [PubMed] [Google Scholar]
- Kandel E. R., Tauc L. Anomalous rectification in the metacerebral giant cells and its consequences for synaptic transmission. J Physiol. 1966 Mar;183(2):287–304. doi: 10.1113/jphysiol.1966.sp007867. [DOI] [PMC free article] [PubMed] [Google Scholar]
- Krishtal O. A., Magura I. S. Calcium ions as inward current carriers in mollusc neurones. Comp Biochem Physiol. 1970 Aug 15;35(4):857–866. doi: 10.1016/0010-406x(70)90080-0. [DOI] [PubMed] [Google Scholar]
- Landowne D., Ritchie J. M. On the control of glycogenolysis in mammalian nervous tissue by calcium. J Physiol. 1971 Jan;212(2):503–517. doi: 10.1113/jphysiol.1971.sp009338. [DOI] [PMC free article] [PubMed] [Google Scholar]
- Lux H. D., Eckert R. Inferred slow inward current in snail neurones. Nature. 1974 Aug 16;250(467):574–576. doi: 10.1038/250574a0. [DOI] [PubMed] [Google Scholar]
- Meech R. W., Standen N. B. Potassium activation in Helix aspersa neurones under voltage clamp: a component mediated by calcium influx. J Physiol. 1975 Jul;249(2):211–239. doi: 10.1113/jphysiol.1975.sp011012. [DOI] [PMC free article] [PubMed] [Google Scholar]
- Meech R. W. The sensitivity of Helix aspersa neurones to injected calcium ions. J Physiol. 1974 Mar;237(2):259–277. doi: 10.1113/jphysiol.1974.sp010481. [DOI] [PMC free article] [PubMed] [Google Scholar]
- Meves H. The ionic requirements for the production of action potentials in helix pomatia neurones. Pflugers Arch. 1968;304(3):215–241. doi: 10.1007/BF00592126. [DOI] [PubMed] [Google Scholar]
- NAKAJIMA S., IWASAKI S., OBATA K. Delayed rectification and anomalous rectification in frog's skeletal muscle membrane. J Gen Physiol. 1962 Sep;46:97–115. doi: 10.1085/jgp.46.1.97. [DOI] [PMC free article] [PubMed] [Google Scholar]
- Neher E., Lux H. D. Differential action of TEA + on two K + -current componentss of a molluscan neurone. Pflugers Arch. 1972;336(2):87–100. doi: 10.1007/BF00592924. [DOI] [PubMed] [Google Scholar]
- Neher E., Lux H. D. Rapid changes of potassium concentration at the outer surface of exposed single neurons during membrane current flow. J Gen Physiol. 1973 Mar;61(3):385–399. doi: 10.1085/jgp.61.3.385. [DOI] [PMC free article] [PubMed] [Google Scholar]
- Neher E., Lux H. D. Voltage clamp on Helix pomatia neuronal membrane; current measurement over a limited area of the soma surface. Pflugers Arch. 1969;311(3):272–277. doi: 10.1007/BF00590532. [DOI] [PubMed] [Google Scholar]
- Nelson P. G., Frank K. Anomalous rectification in cat spinal motoneurons and effect of polarizing currents on excitatory postsynaptic potential. J Neurophysiol. 1967 Sep;30(5):1097–1113. doi: 10.1152/jn.1967.30.5.1097. [DOI] [PubMed] [Google Scholar]
- Rojas E., Armstrong C. Sodium conductance activation without inactivation in pronase-perfused axons. Nat New Biol. 1971 Feb 10;229(6):177–178. doi: 10.1038/newbio229177a0. [DOI] [PubMed] [Google Scholar]
- Smith T. G., Jr, Barker J. L., Gainer H. Requirements for bursting pacemaker potential activity in molluscan neurones. Nature. 1975 Feb 6;253(5491):450–452. doi: 10.1038/253450a0. [DOI] [PubMed] [Google Scholar]
- Thomas R. C. Electrogenic sodium pump in nerve and muscle cells. Physiol Rev. 1972 Jul;52(3):563–594. doi: 10.1152/physrev.1972.52.3.563. [DOI] [PubMed] [Google Scholar]
- WERMAN R., GRUNDFEST H. Graded and all-or-none electrogenesis in arthropod muscle. II. The effects of alkali-earth and onium ions on lobster muscle fibers. J Gen Physiol. 1961 May;44:997–1027. doi: 10.1085/jgp.44.5.997. [DOI] [PMC free article] [PubMed] [Google Scholar]
- WERMAN R., McCANN F. V., GRUNDFEST H. Graded and all-or-none electrogenesis in arthropod muscle. I. The effects of alkali-earth cations on the neuromuscular system of Romalea microptera. J Gen Physiol. 1961 May;44:979–995. doi: 10.1085/jgp.44.5.979. [DOI] [PMC free article] [PubMed] [Google Scholar]
- Wilson W. A., Wachtel H. Negative resistance characteristic essential for the maintenance of slow oscillations in bursting neurons. Science. 1974 Dec 6;186(4167):932–934. doi: 10.1126/science.186.4167.932. [DOI] [PubMed] [Google Scholar]