Abstract
1. Voltage-clamp experiments were carried out using the techniques described in the preceding paper. 2. In one series of experiments an attempt was made to disrupt the T-system with glycerol treatment. Muscles were soaked in Ringer + 400 mM glycerol for 1 hr at room temperature, transferred to Ringer + 5 mM calcium + 5 mM magnesium for 20-30 min, and then cooled to around 2 degrees C and placed in an isosmotic test solution containing tetrodotoxin for electrical measurements. 3. The density of charge seen in isosmotic tetraethylammonium (TEA) solution with strong depolarization, normalized according to fibre capacitance, was decreased by glycerol treatment to about one third the amount seen in untreated hypertonic fibres. 4. An analysis of fibre capacitance revealed that only 0-4 of the tubular capacitance was removed by this particular glycerol procedure. If the density of charge with respect to capacitance is corrected for this decrease in capacitance, the results indicate that glycerol treatment removed or immobilized 0-77 of the charge initially present. Thus the effect of glycerol treatment to reduce charge does not depend entirely on disrupting the electrical continuity of the T-system. 5. The effects of maintained depolarization were studied using a TEA Ringer made hypertonic with sucrose. When the voltage was changed from -80 to -21 mV the measurable charge movement declined exponentially to zero with a time constant of 13-24 sec. On repolarization the process recovered exponentially to the initial level with a time constant of 21-53 sec. 6. Experiments were also carried out using a sodium Ringer made hypertonic with sucrose. For small depolarizations only charge movement currents were seen, whereas for large depolarizations large delayed ionic currents, presumably carried by potassium, were observed. With moderate depolarizations in the range V = -40 to -30 mV, both components were of comparable magnitude. 7. A plot of the fractional charge movement (Q/Qmax) vs. V fitted at moderate depolarizations is similar to that of n infinity vs. V fitted at larger depolarizations. Values of n infinity were obtained by fitting the delayed ionic current to n4(V - VK). For voltages between -40 and -30 mV the time constant for charge tauQ was always less than taun; values of taun/tauQ varied from 1-6 to 4-3. 8. Glycerol treatment had little if any effect on the kinetics of delayed rectifier currents. Values of gK measured in isosmotic solution following glycerol were about one third the values obtained in untreated fibres in a hypertonic solution (osmolality three times normal). The threefold difference in gK is probably due to a similar difference in internal potassium concentration. 9. These results and others are difficult to reconcile with the idea that the charge movement process acts as a gate for potassium channels. It seems more likely that charge movement is a step in the activation of contraction.
Full text
PDF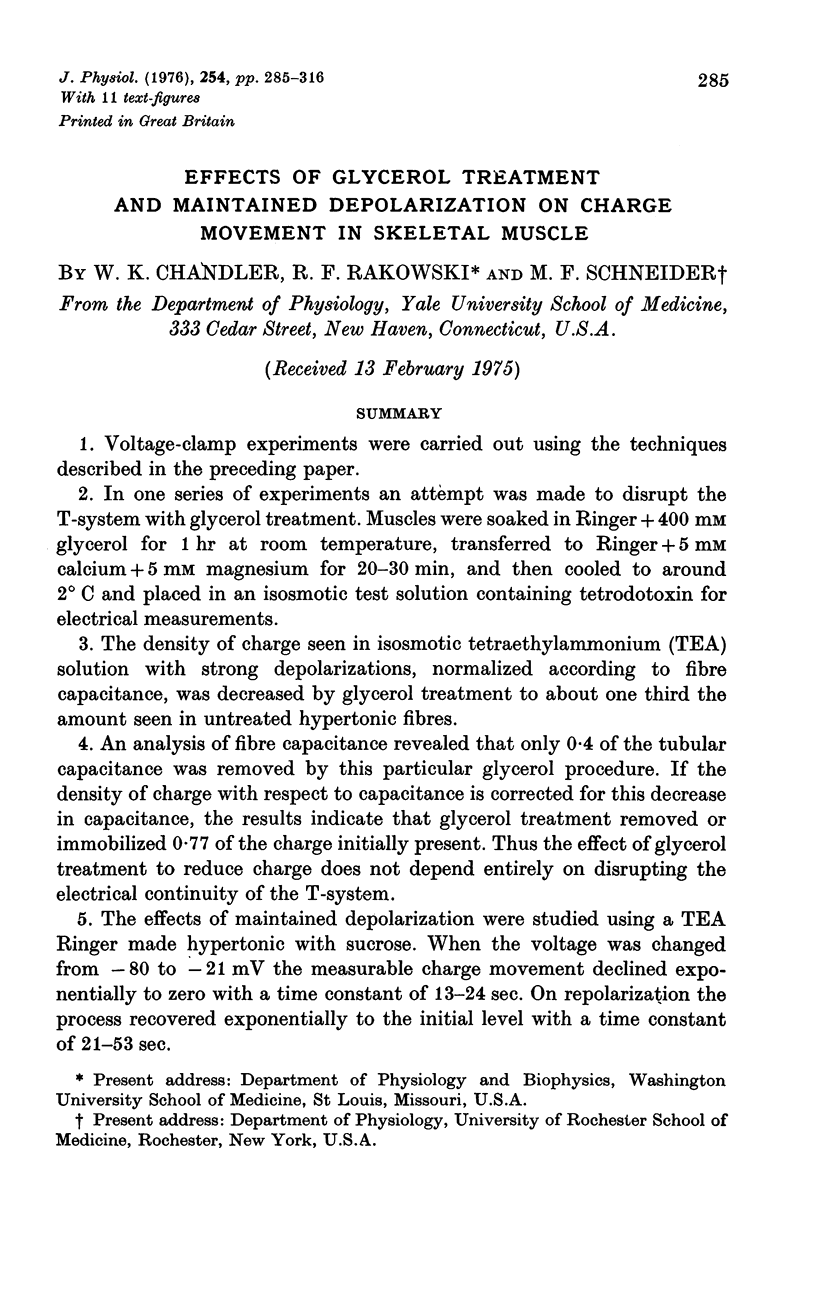
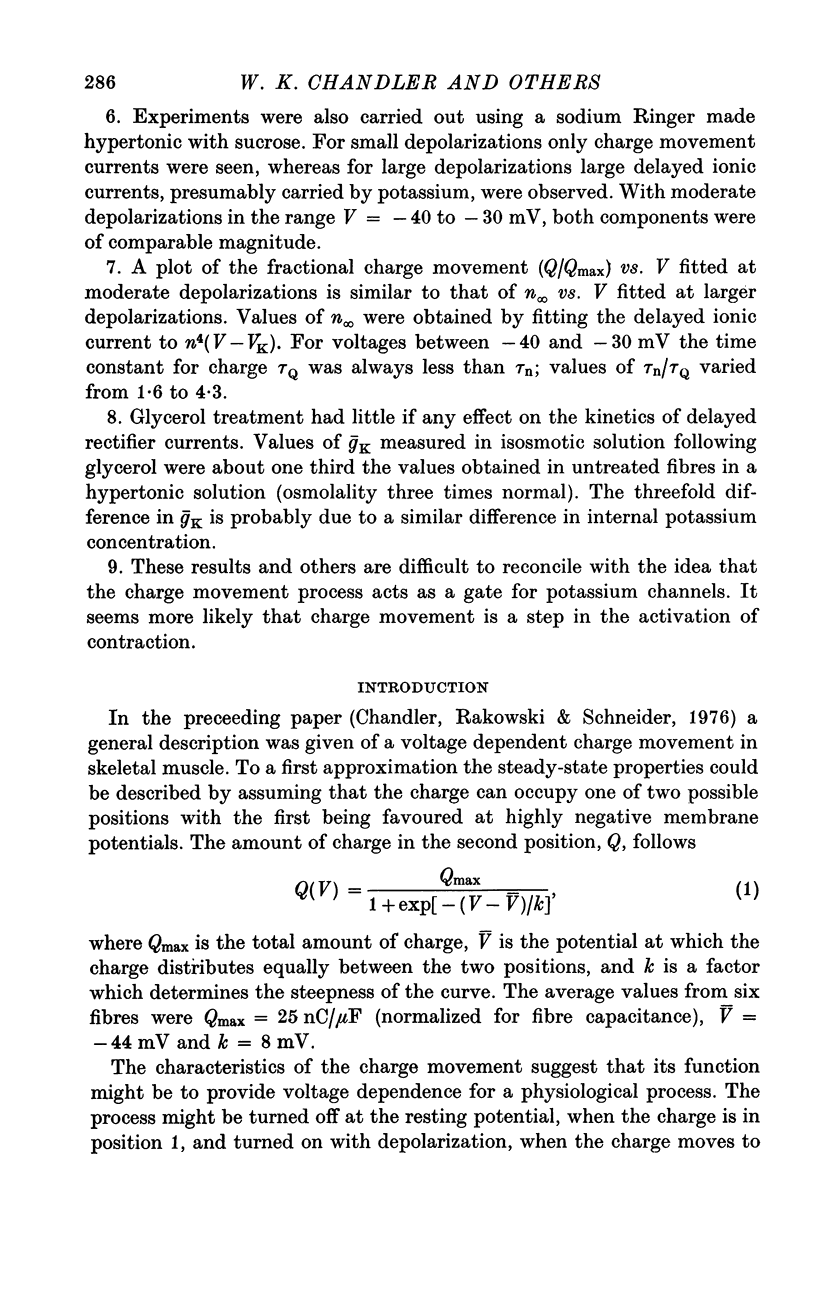
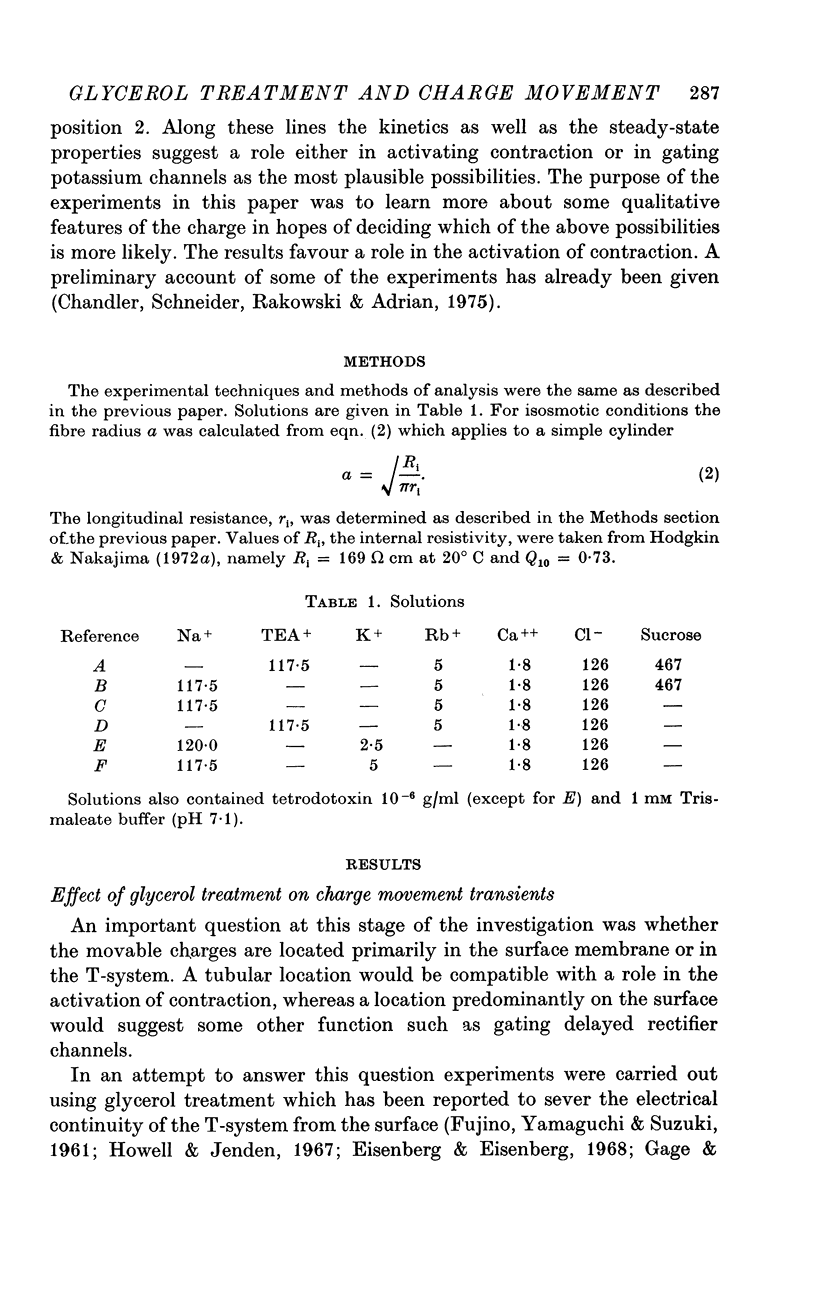
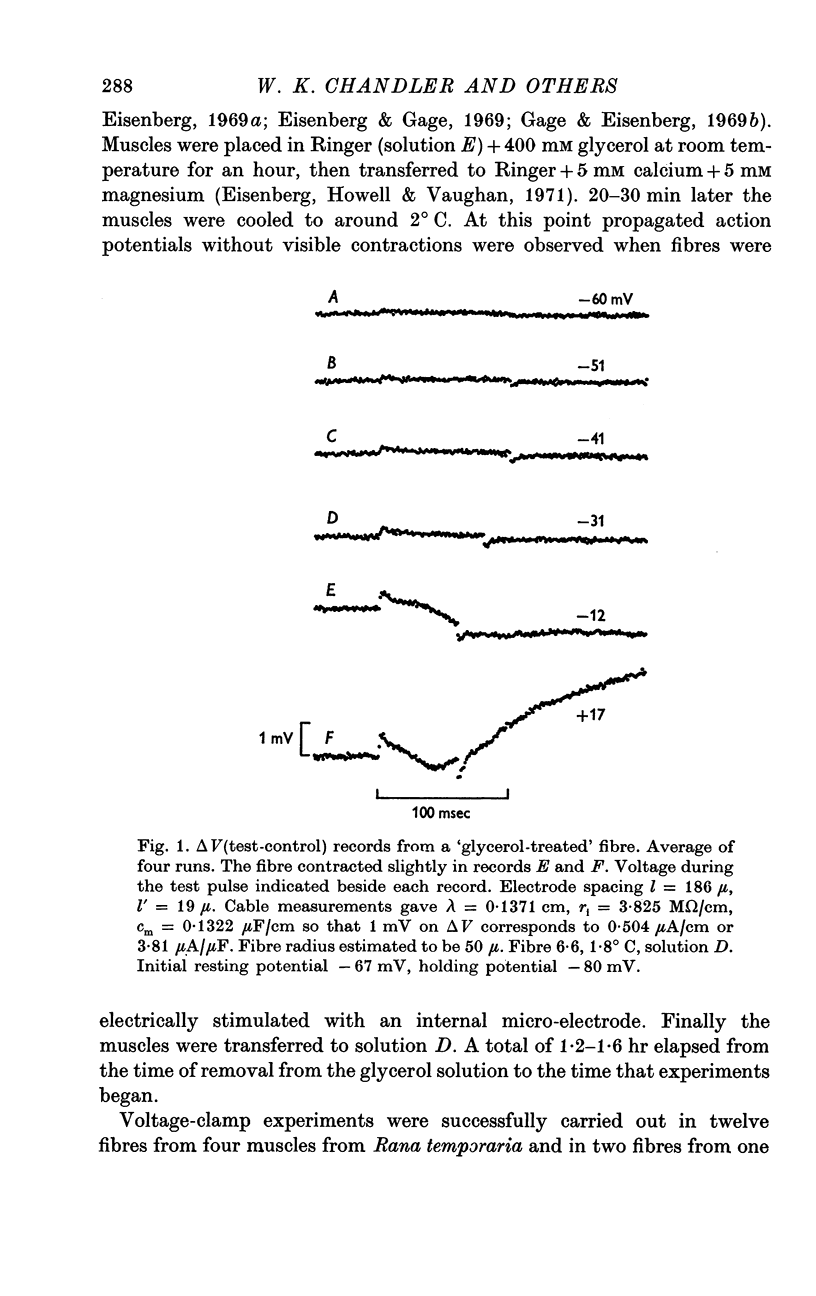
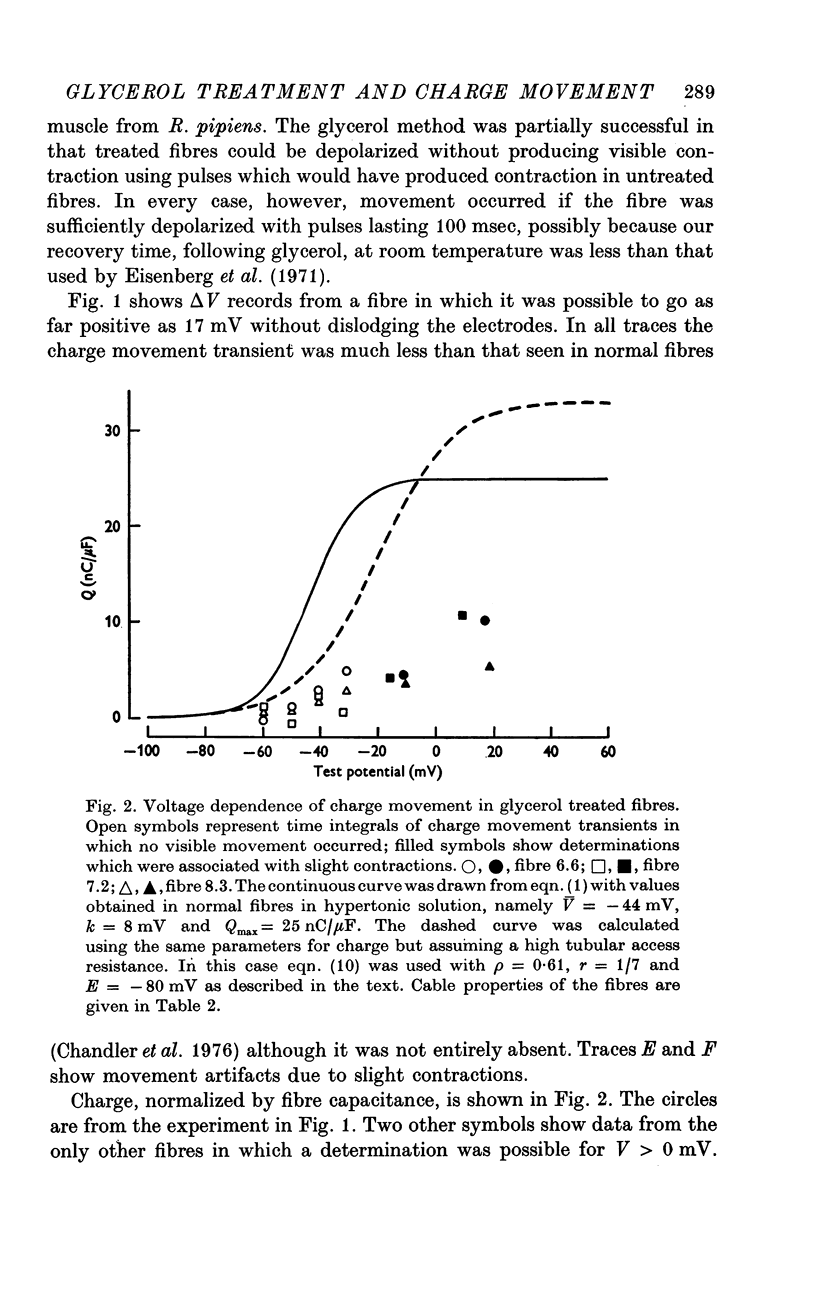
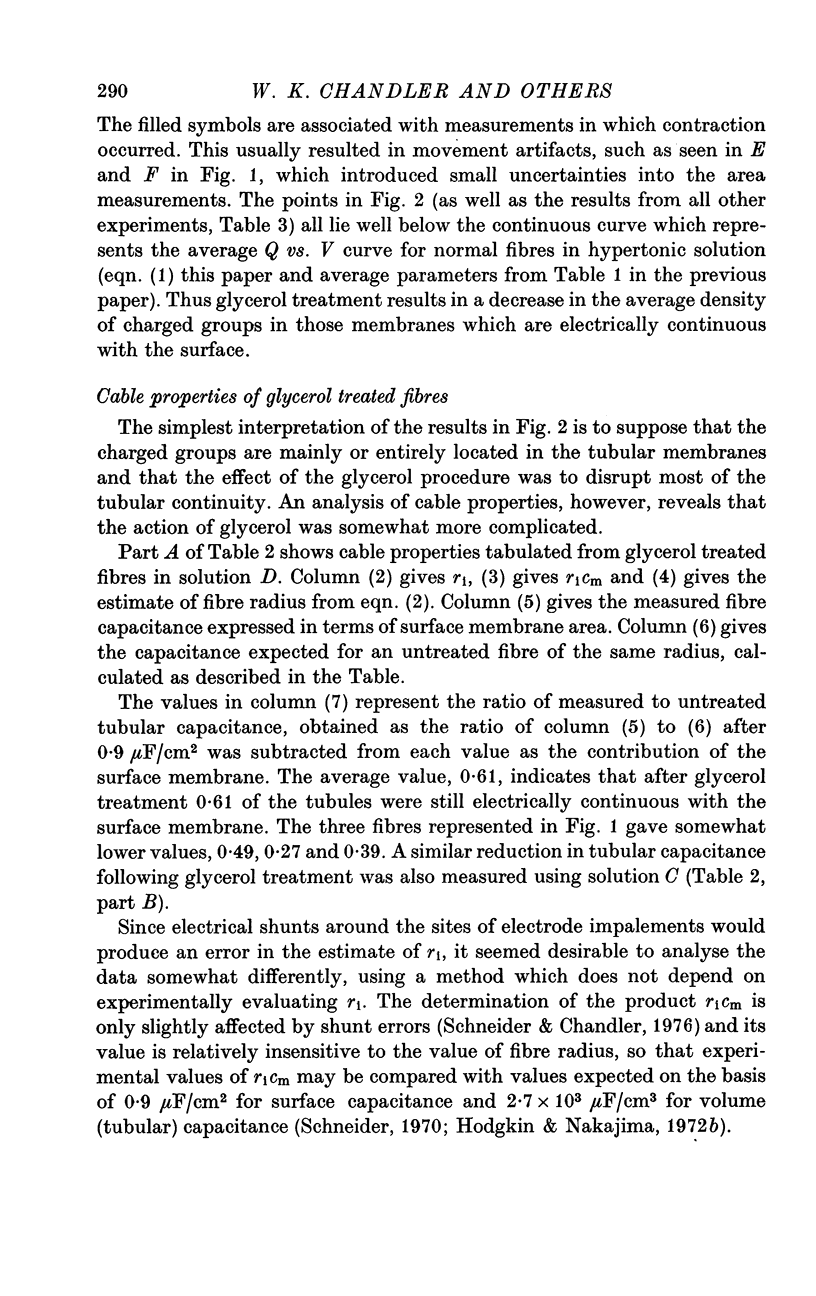
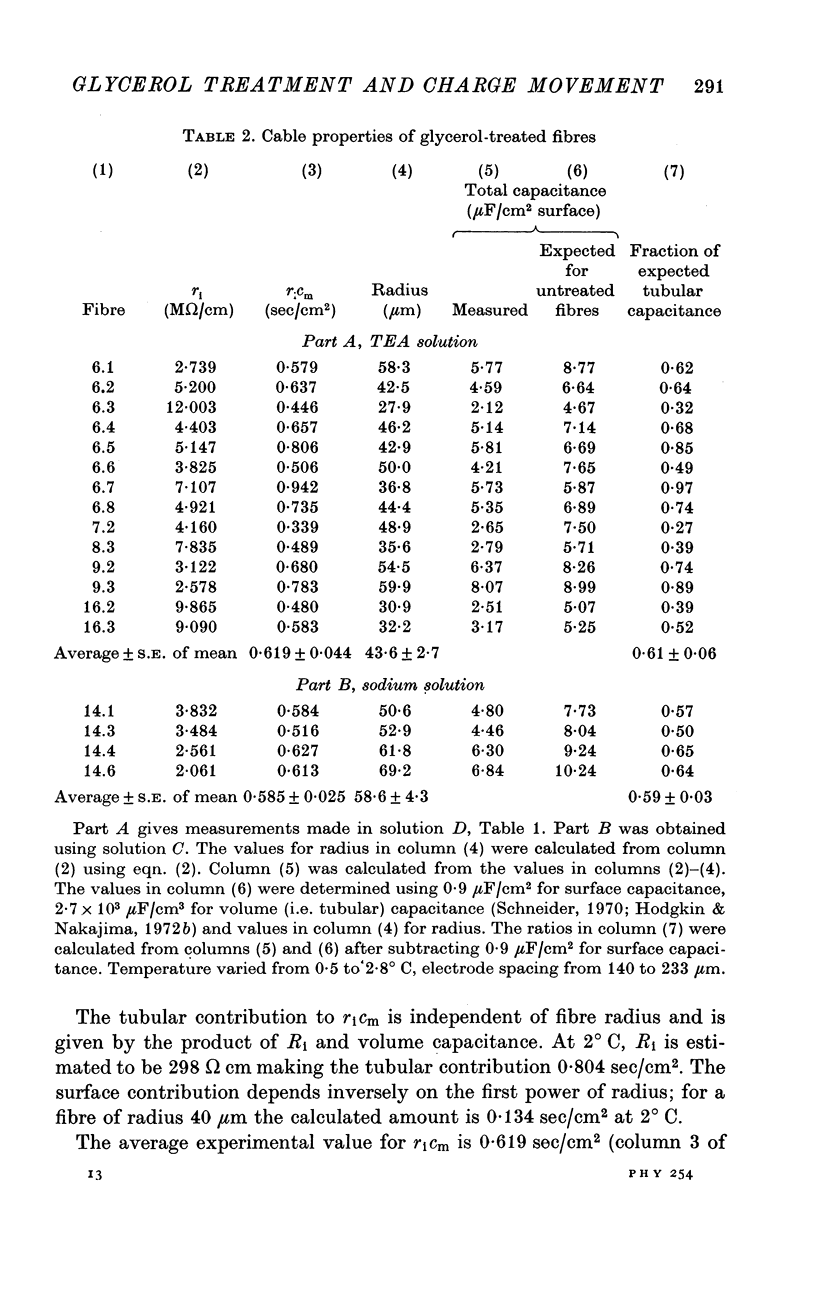
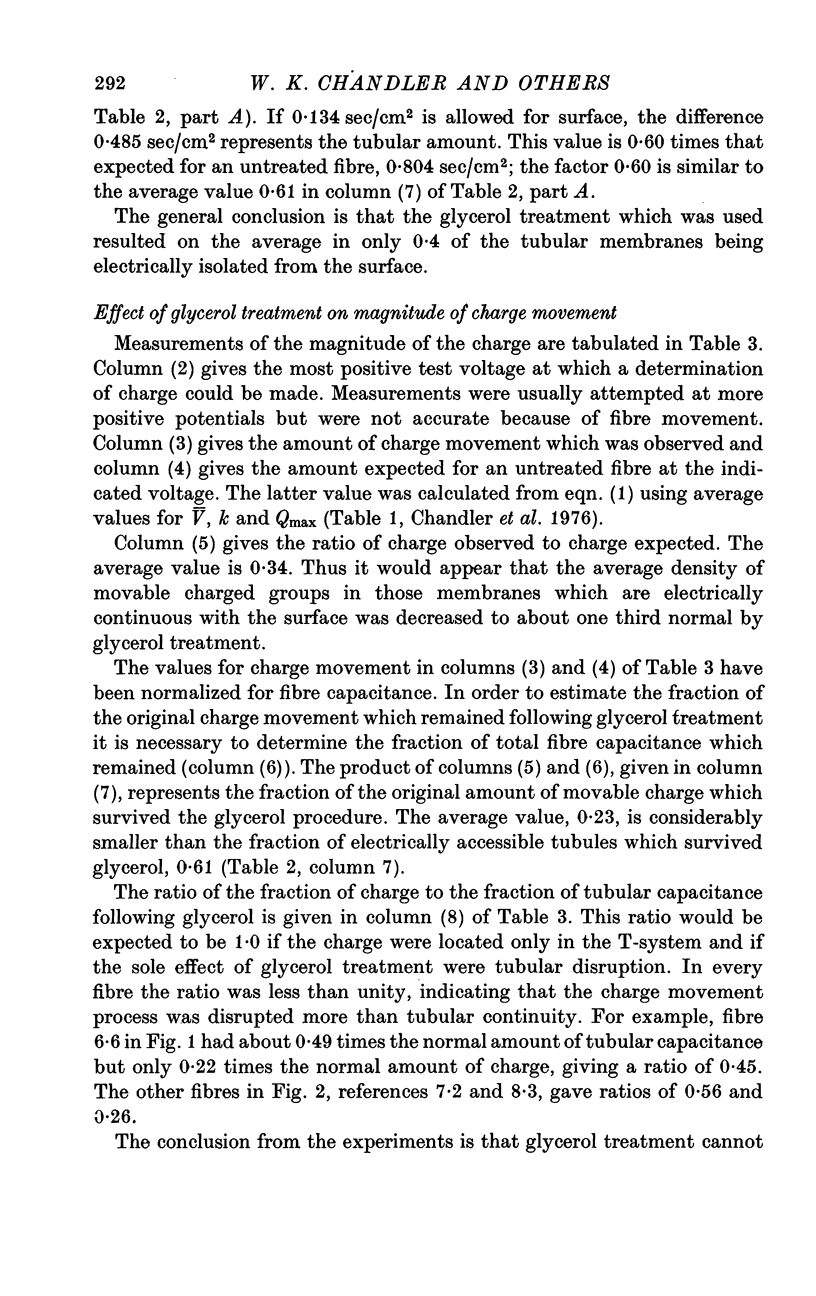
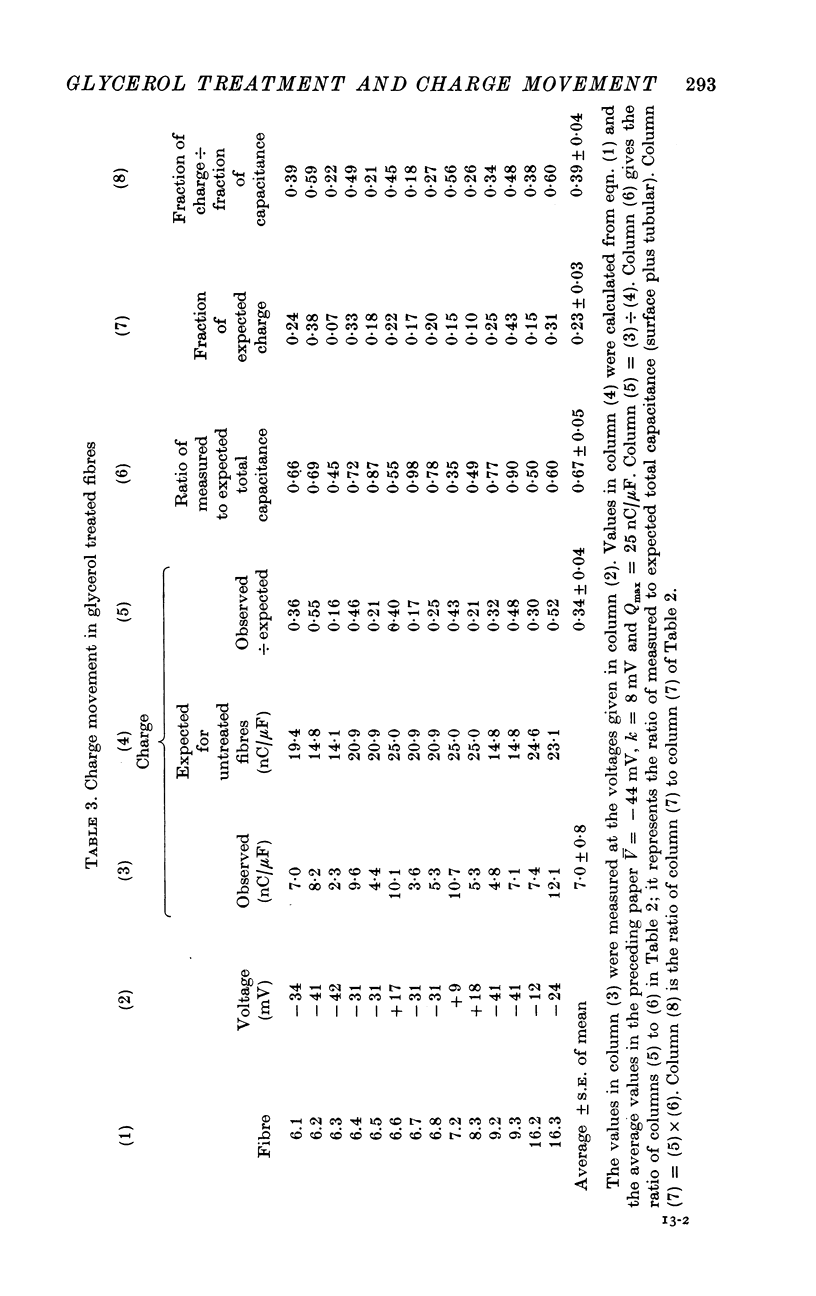
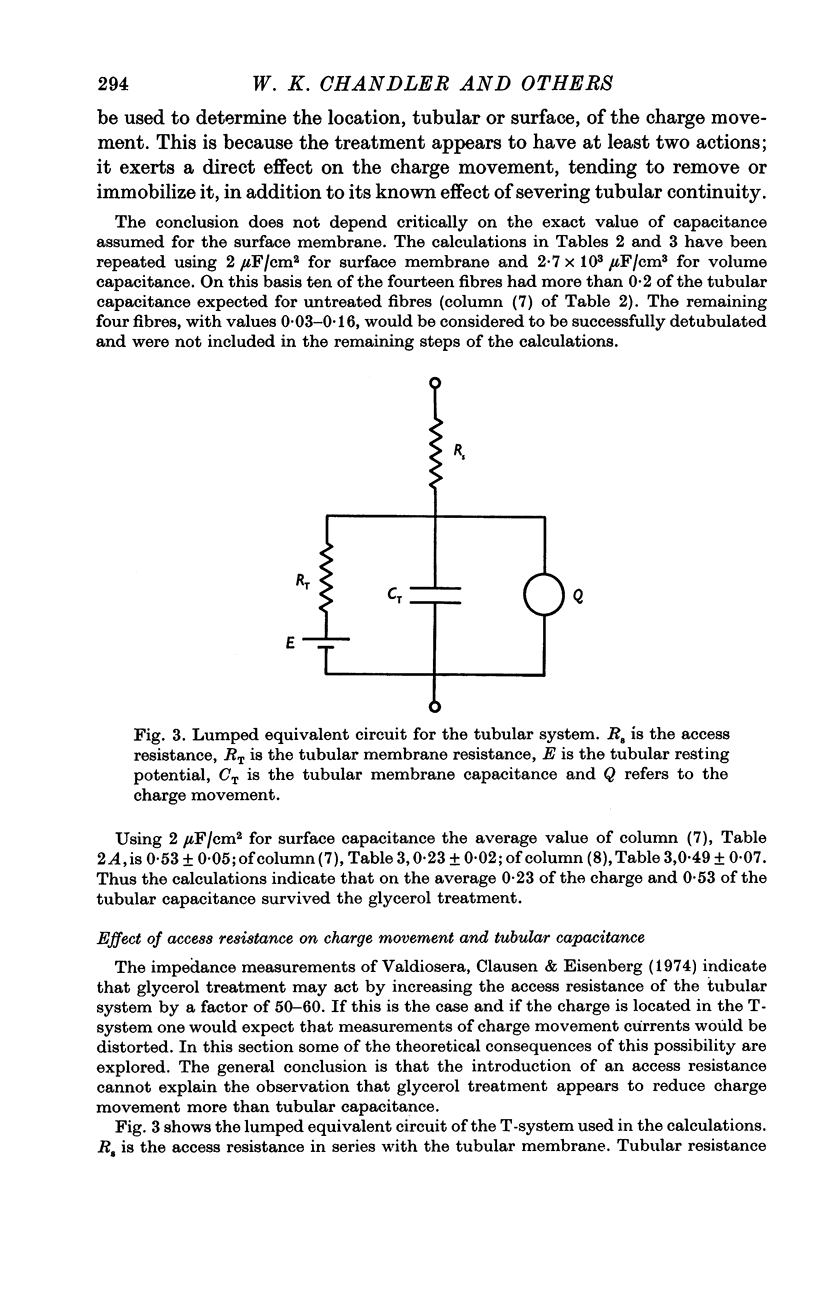
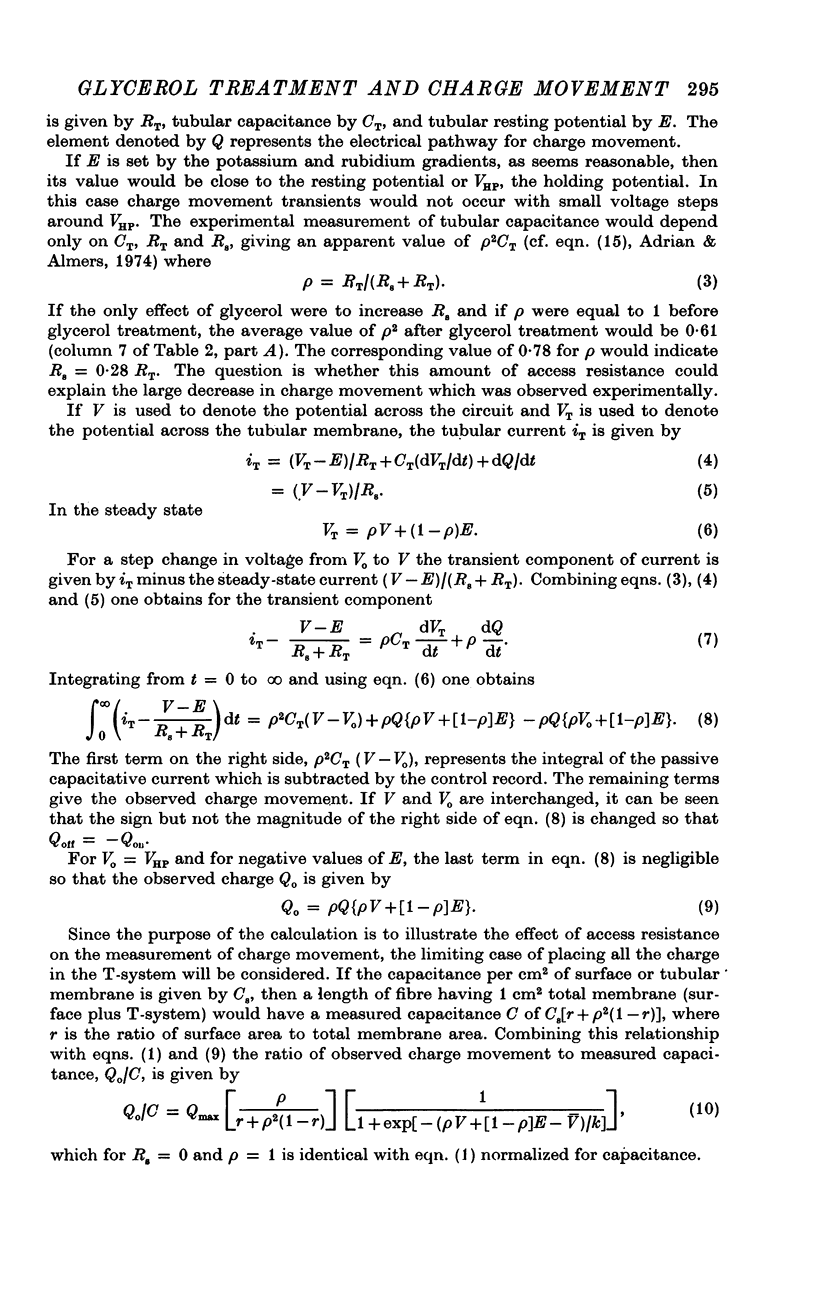
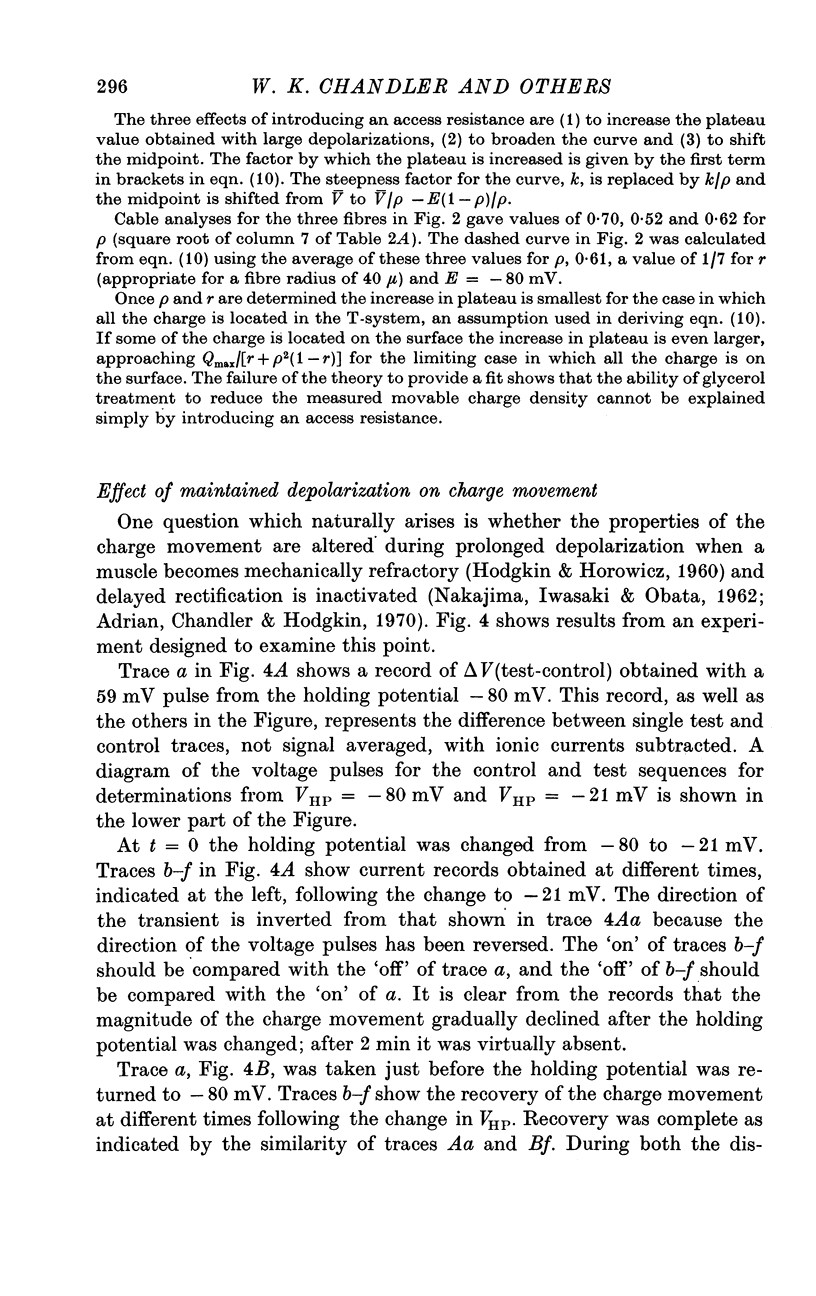
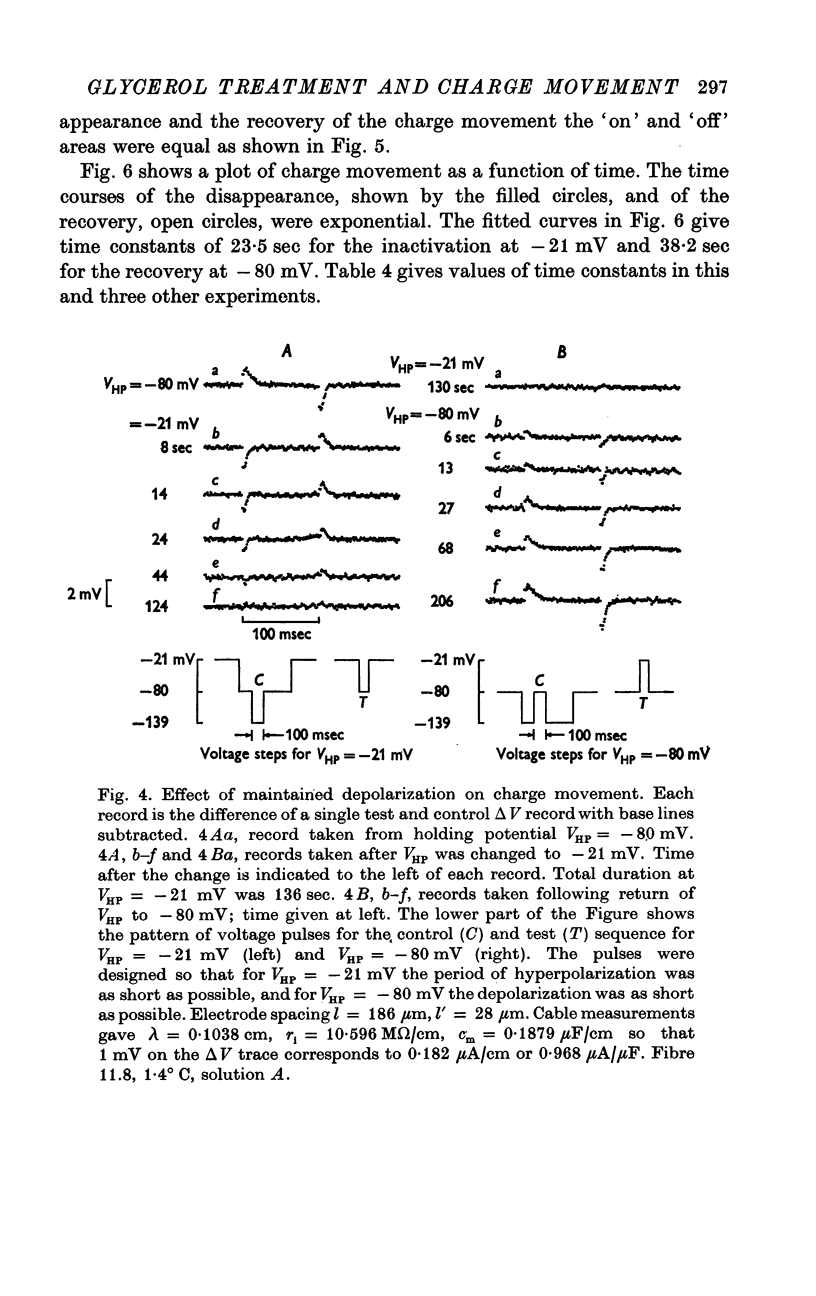
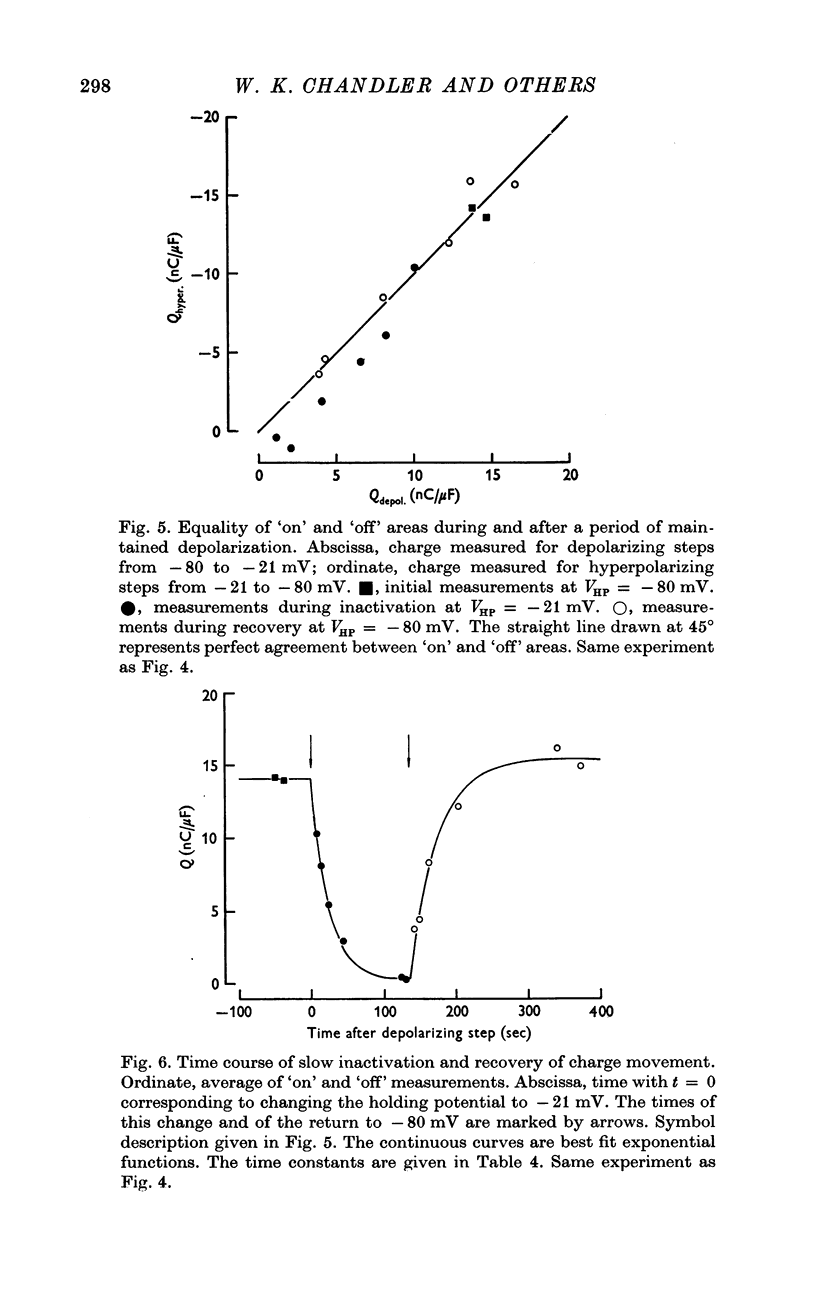
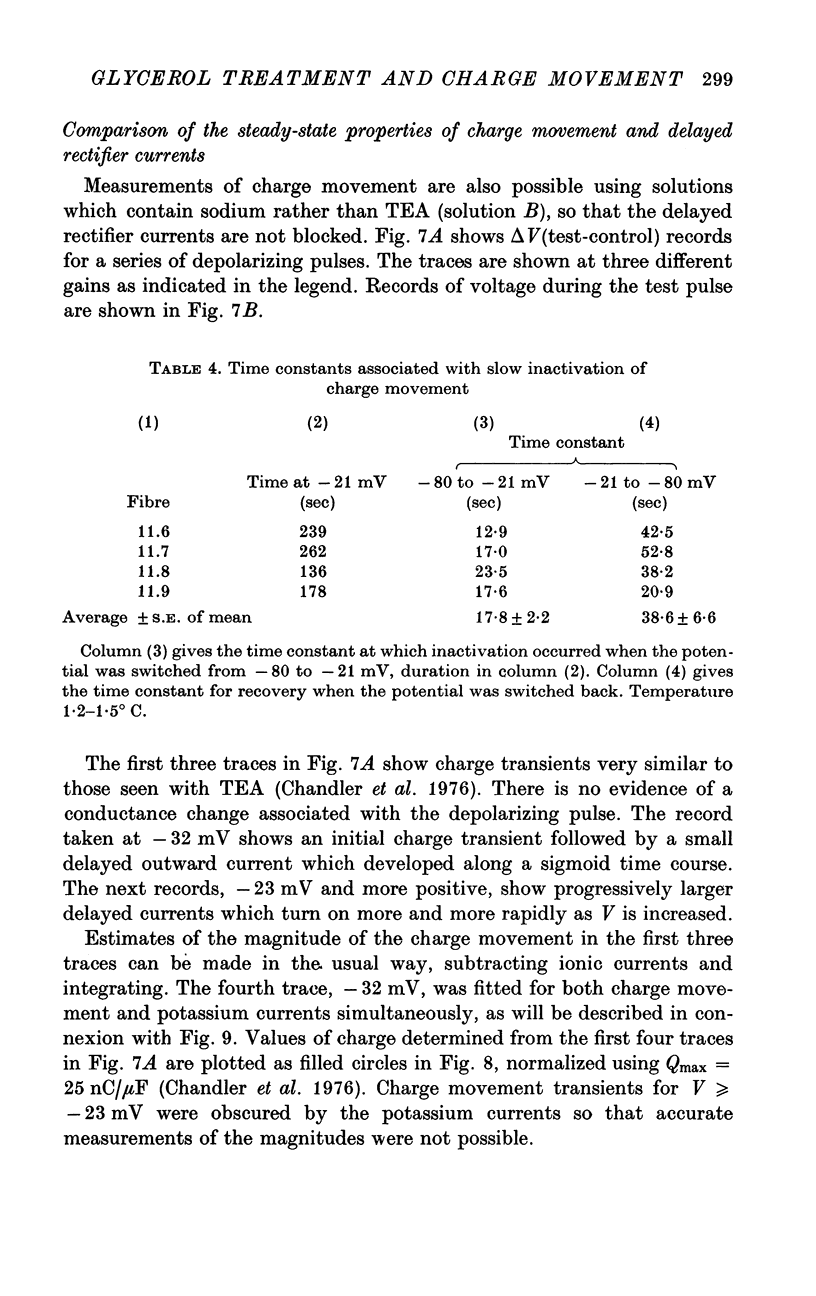
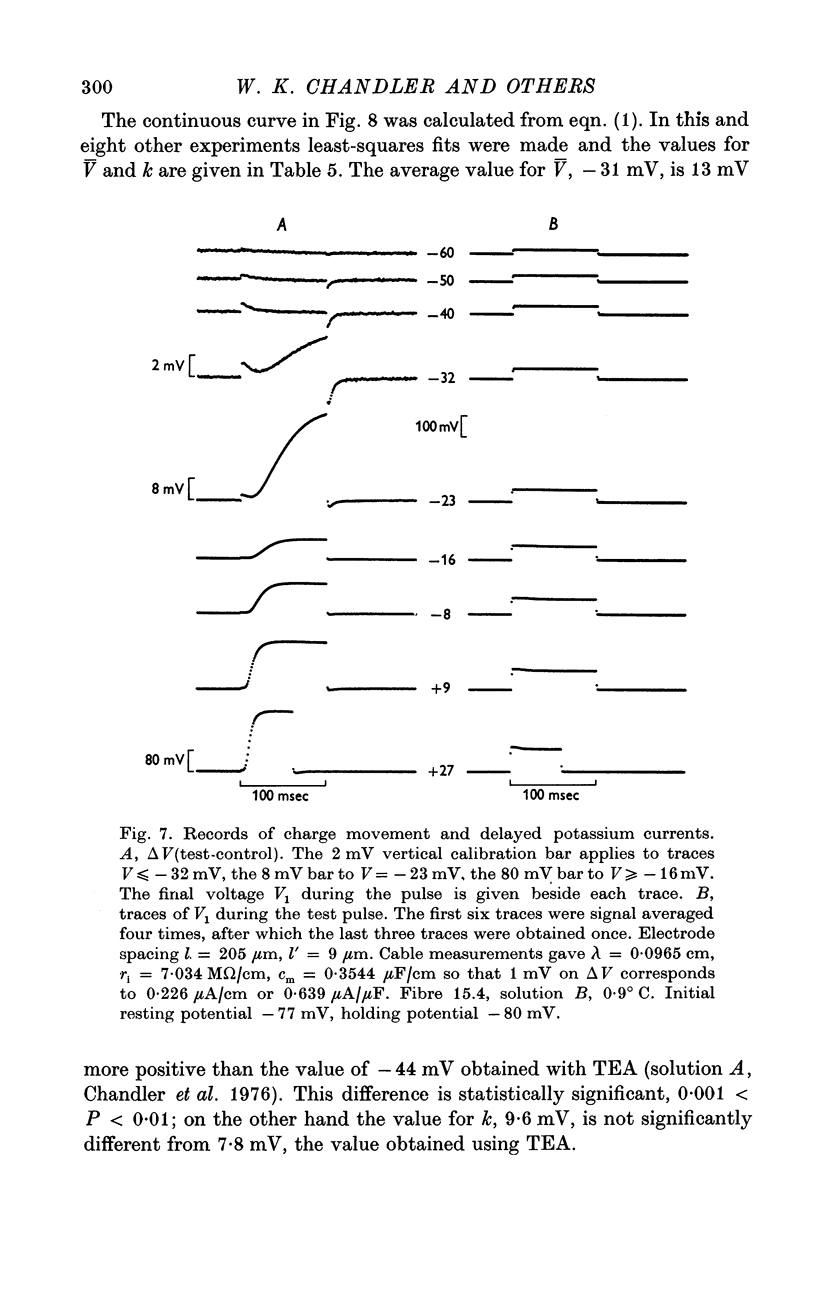
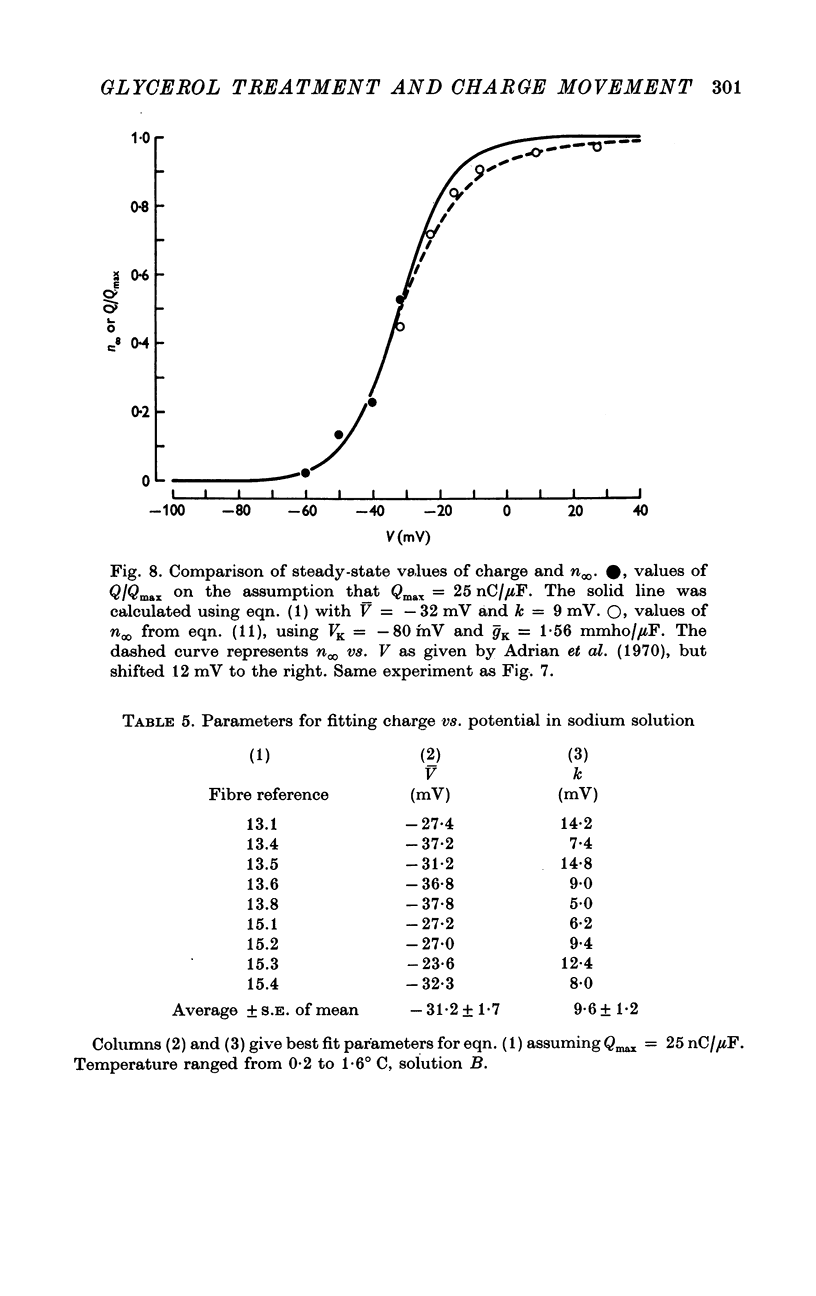
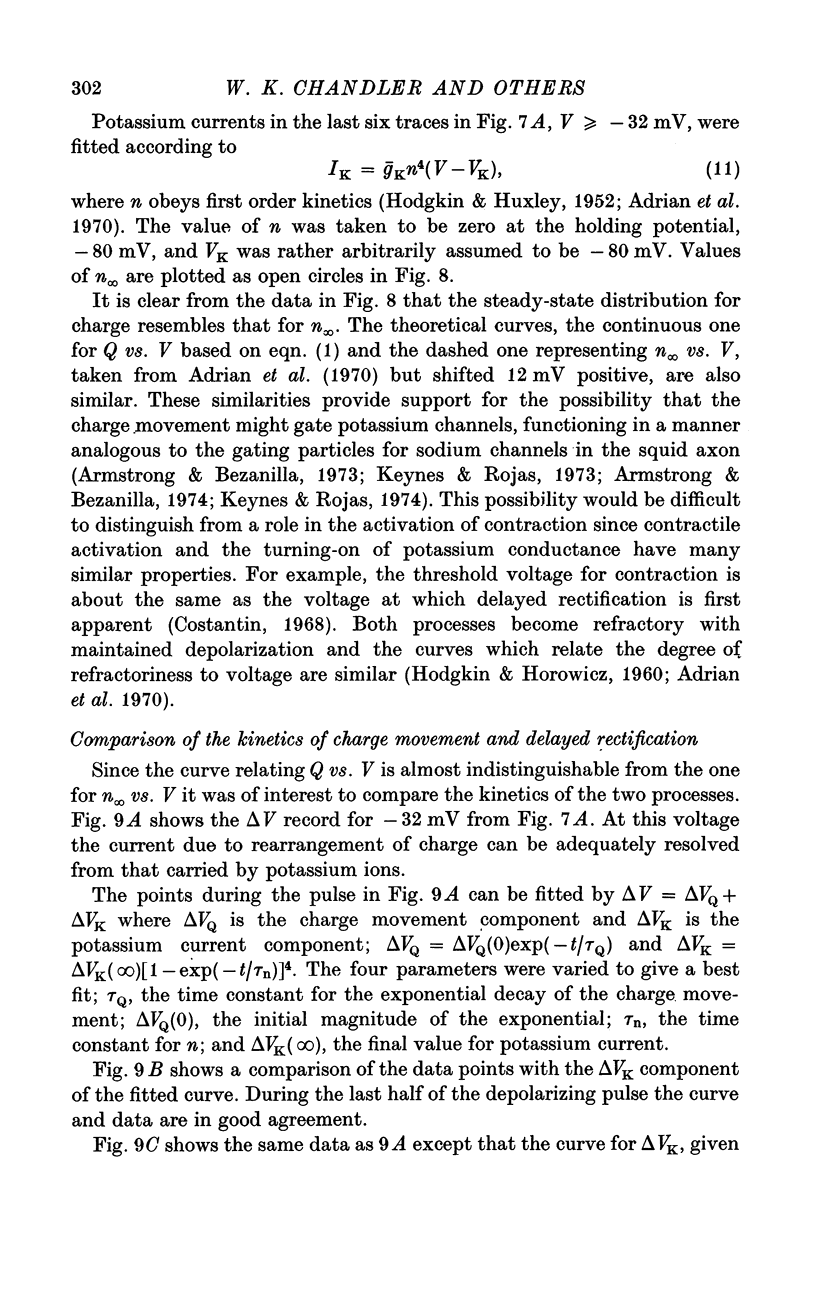
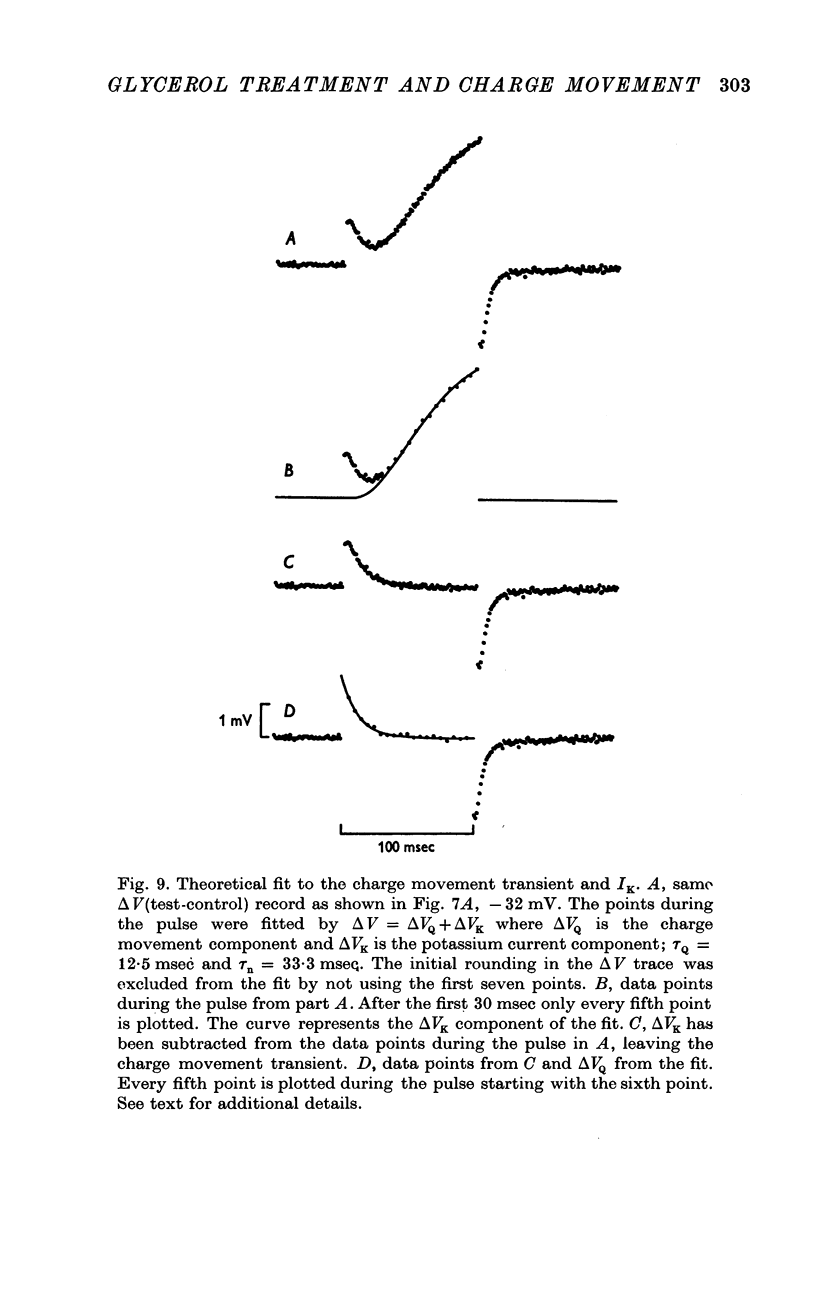
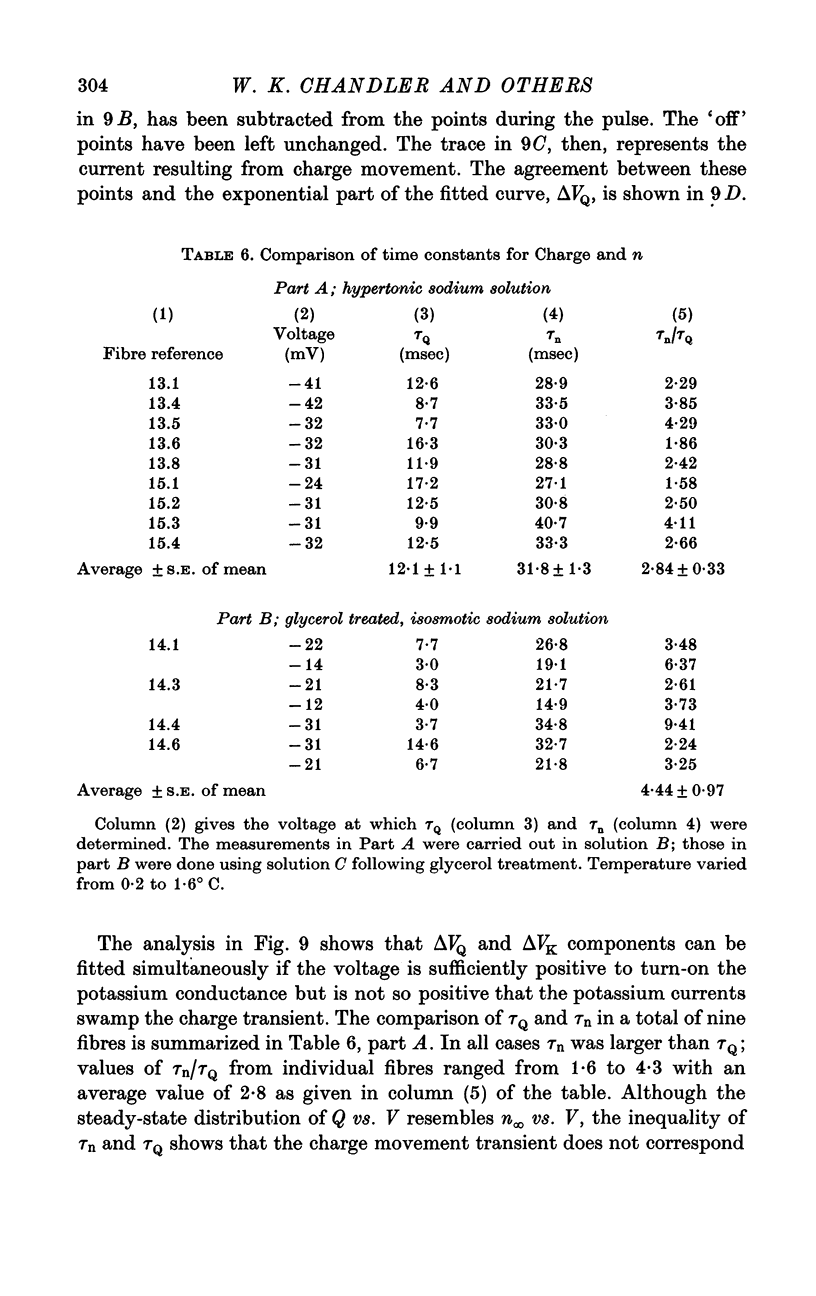
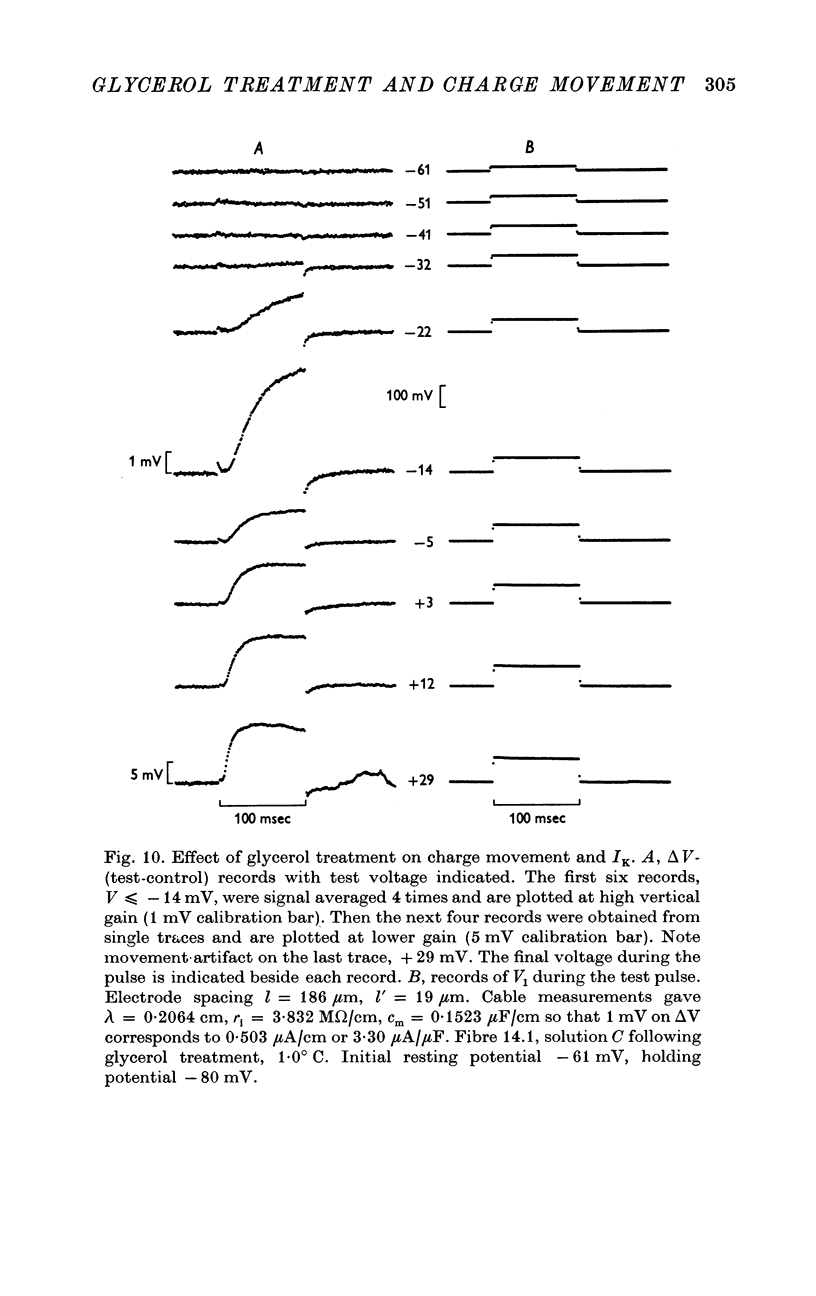
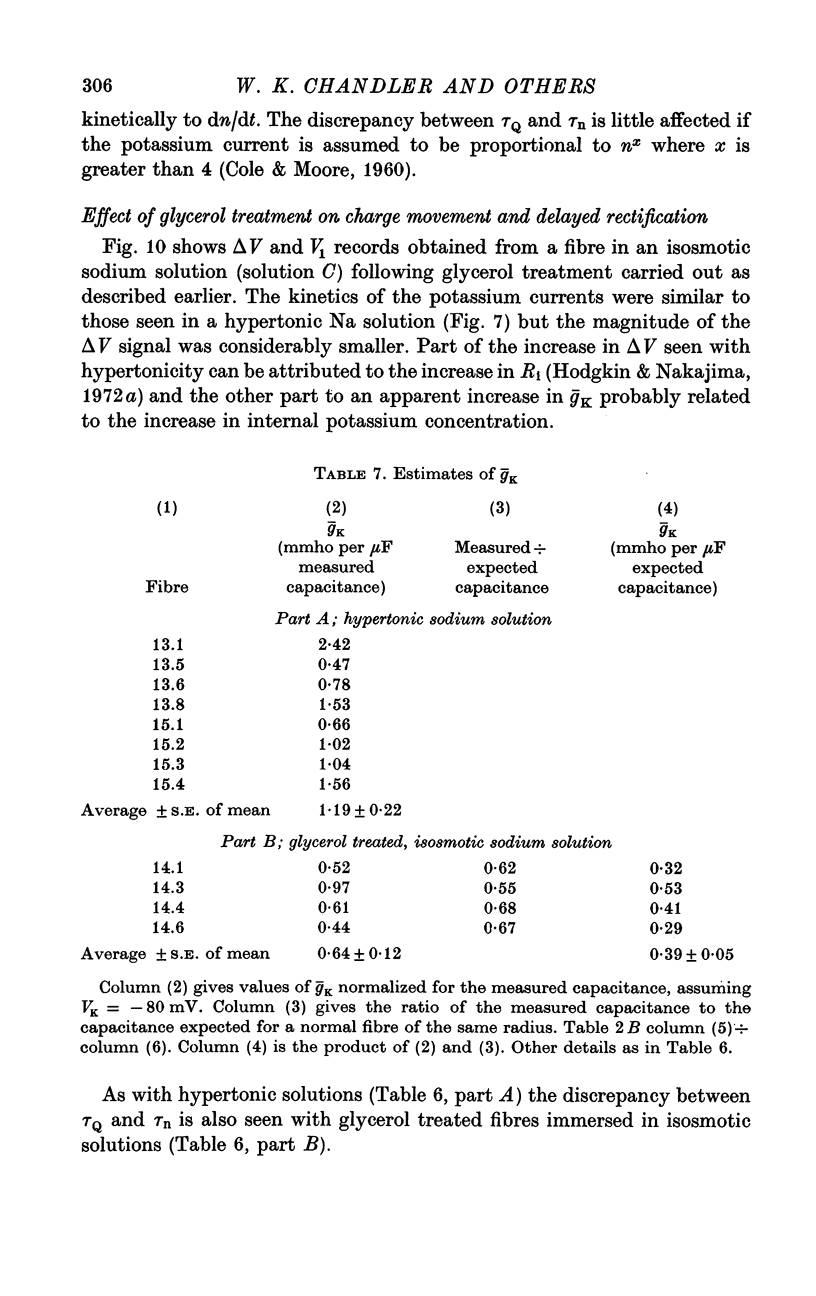
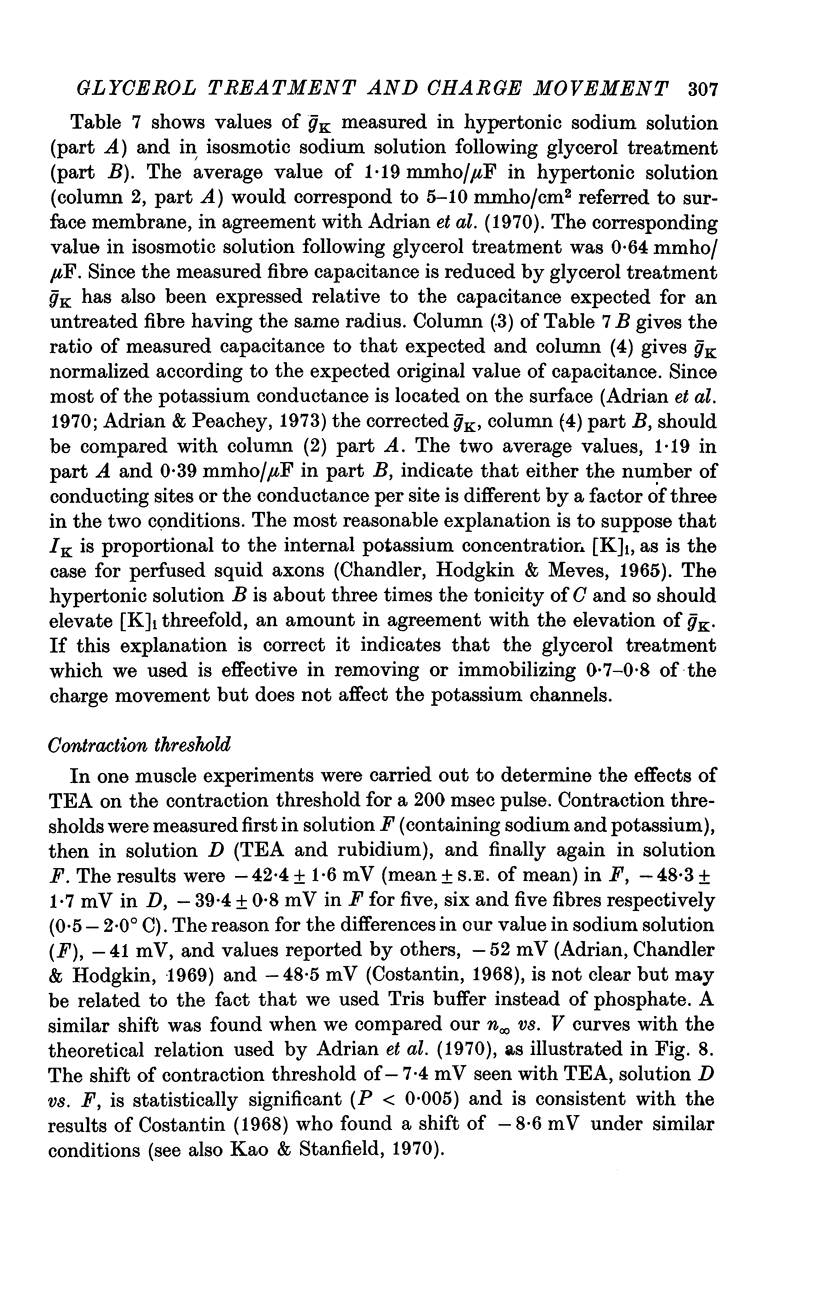
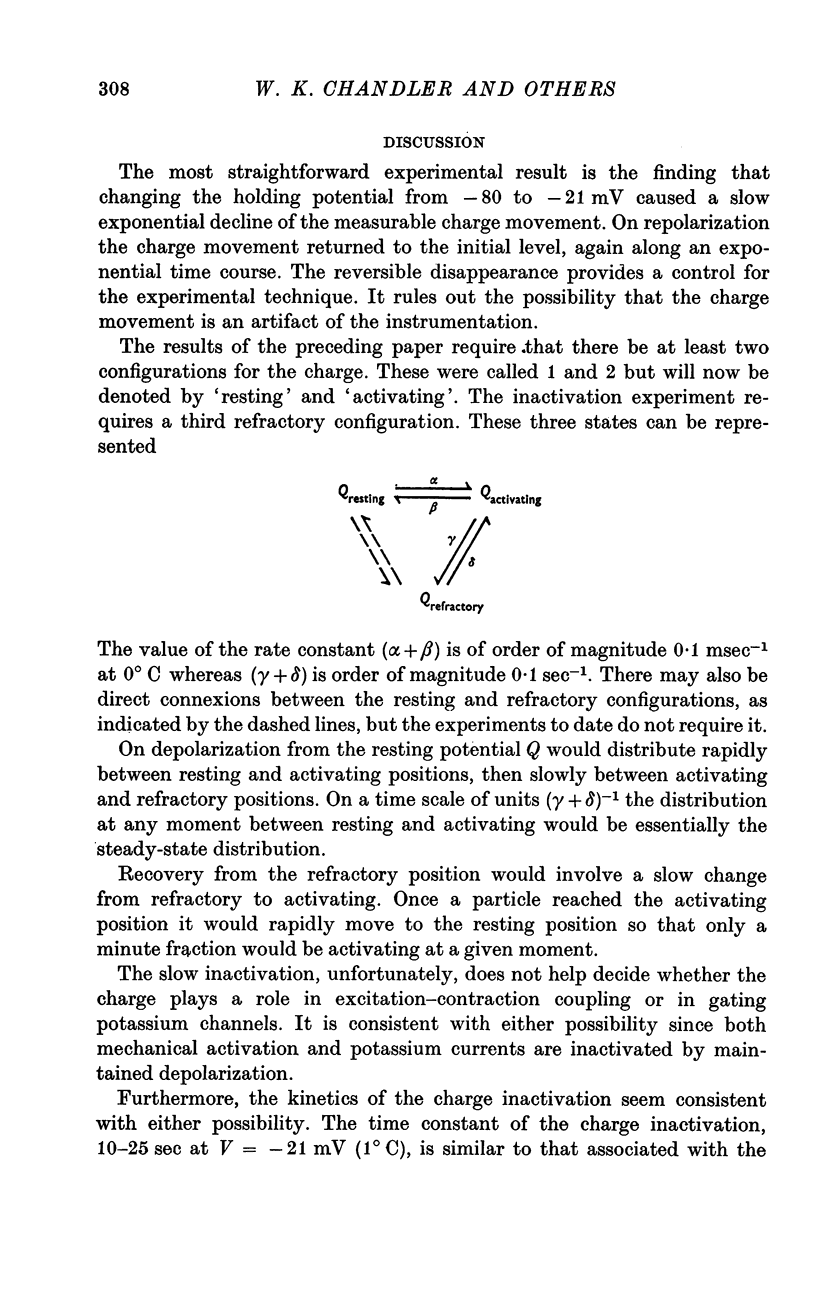
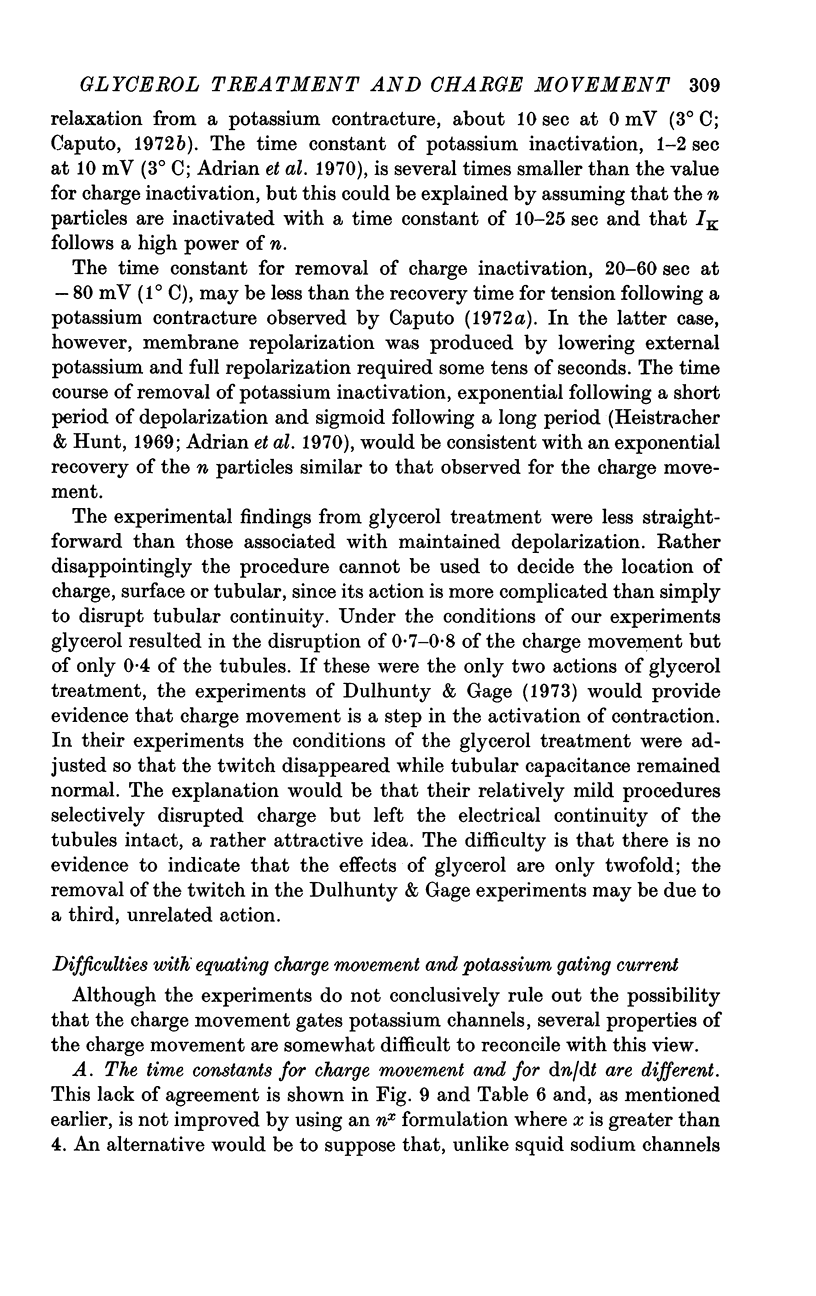
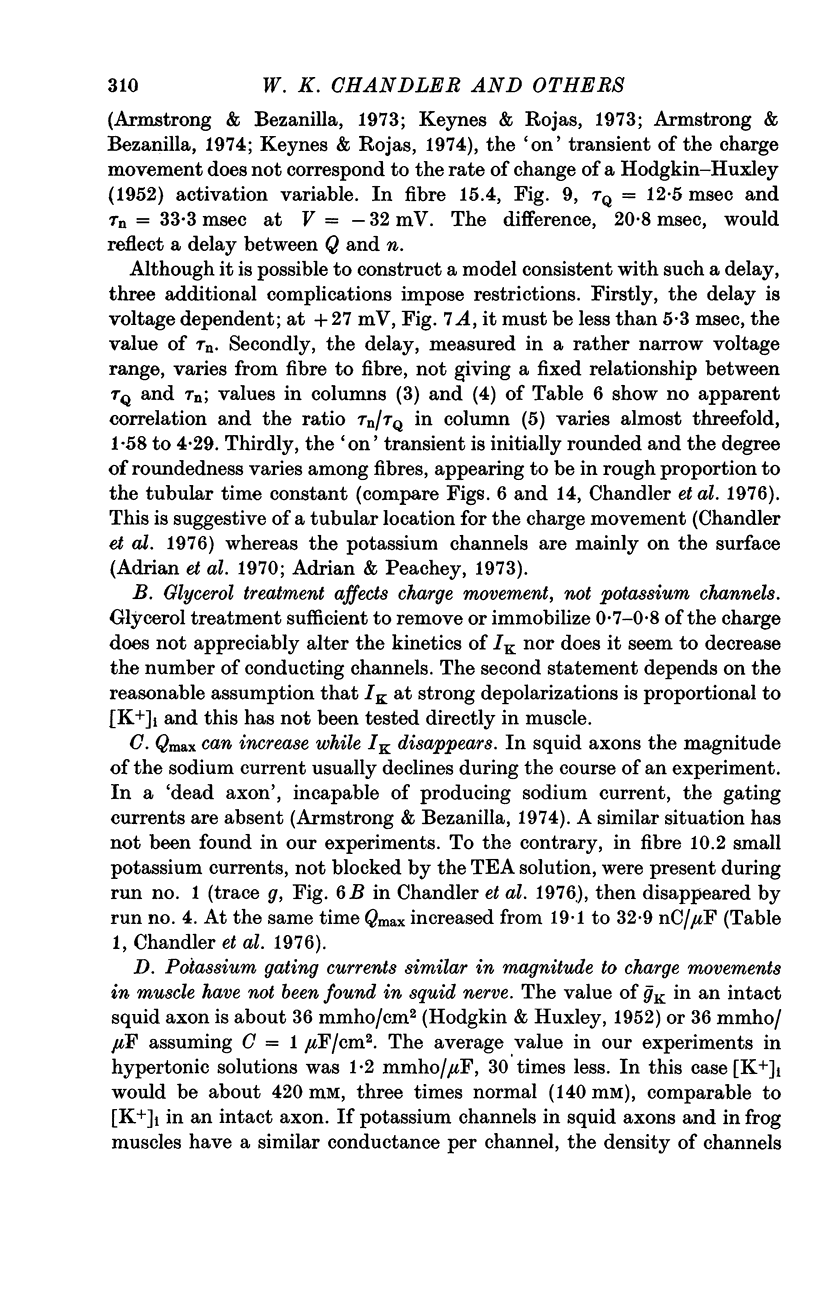
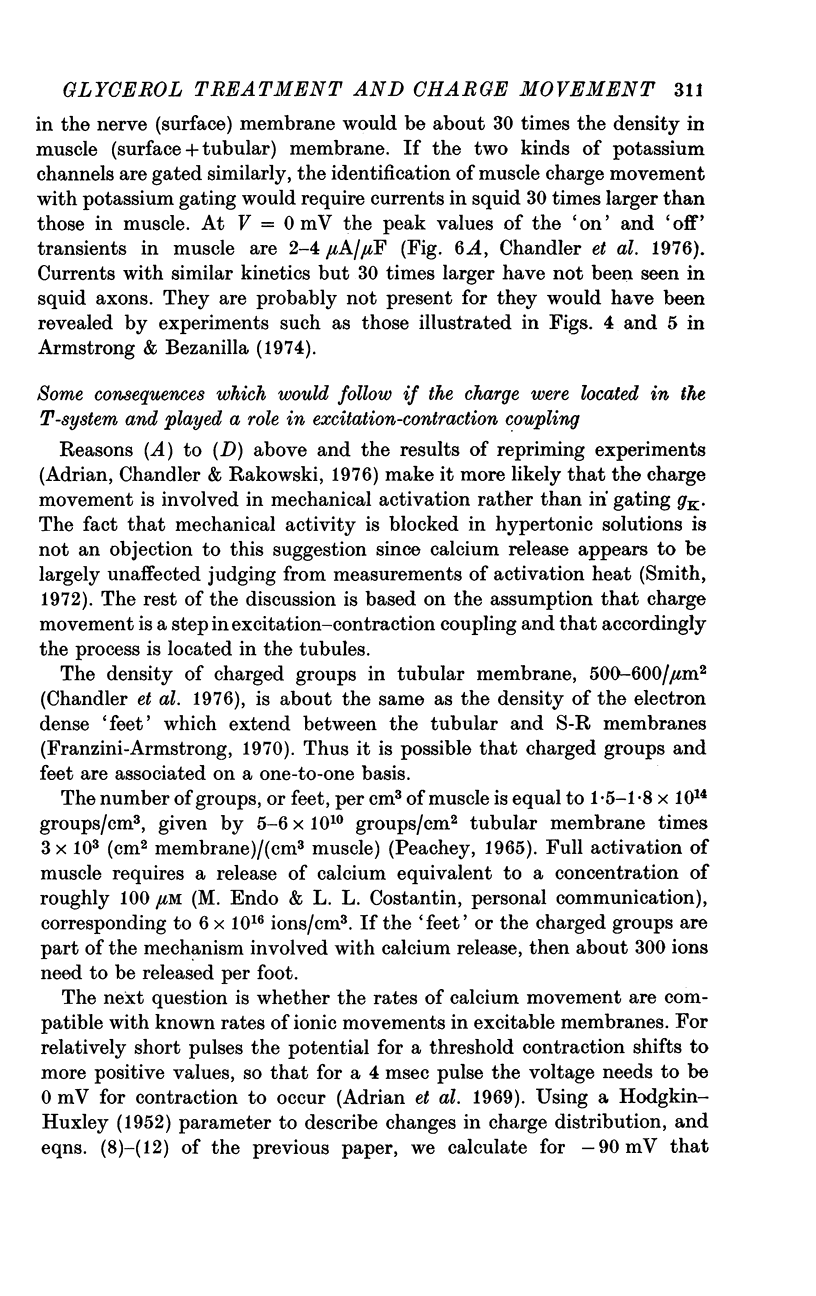
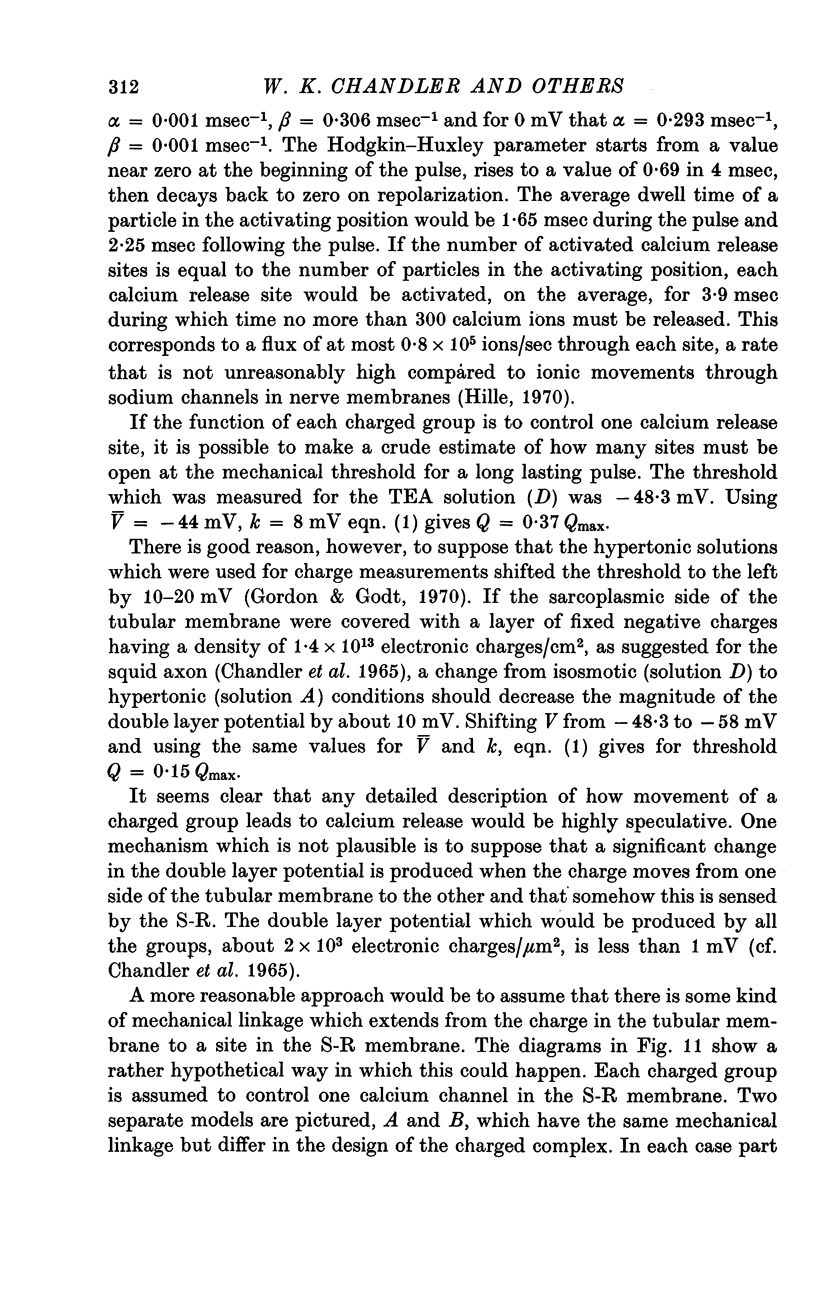
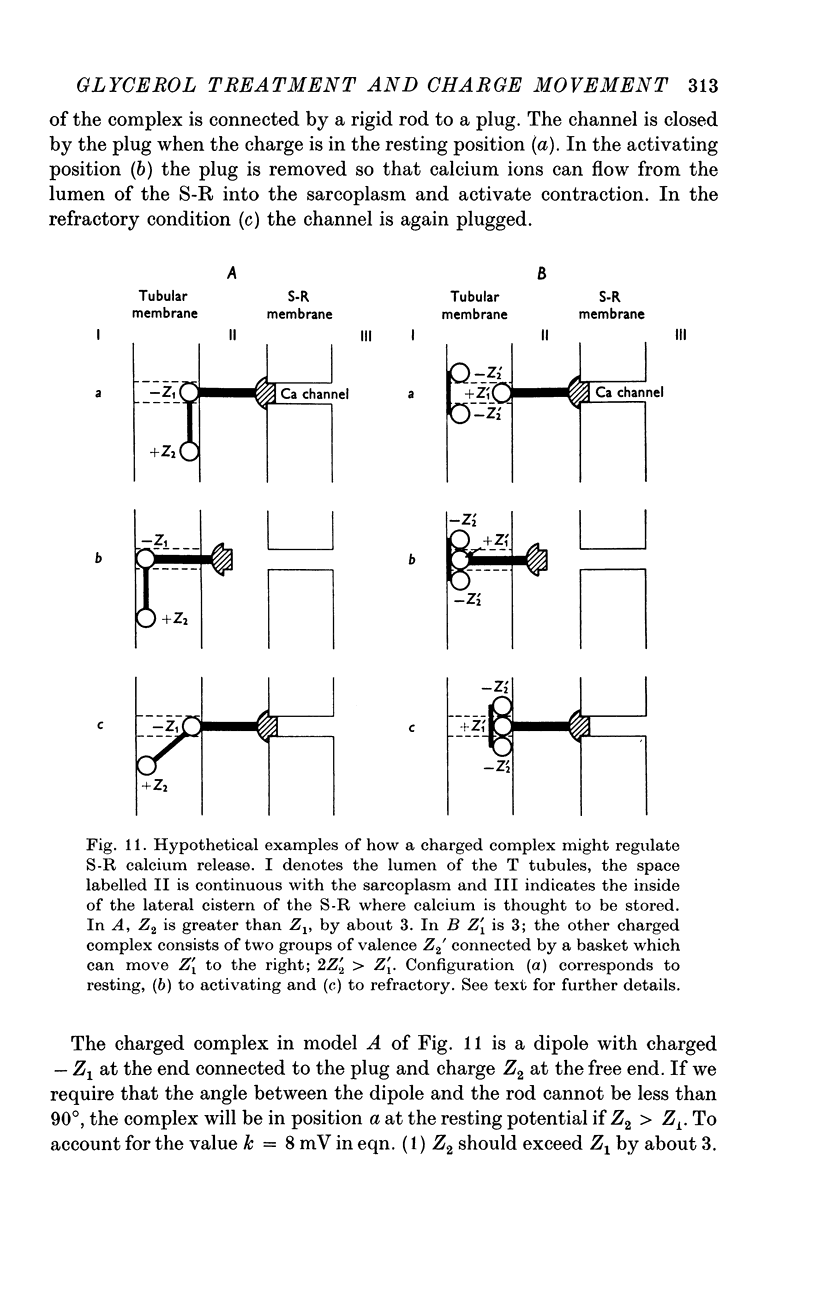
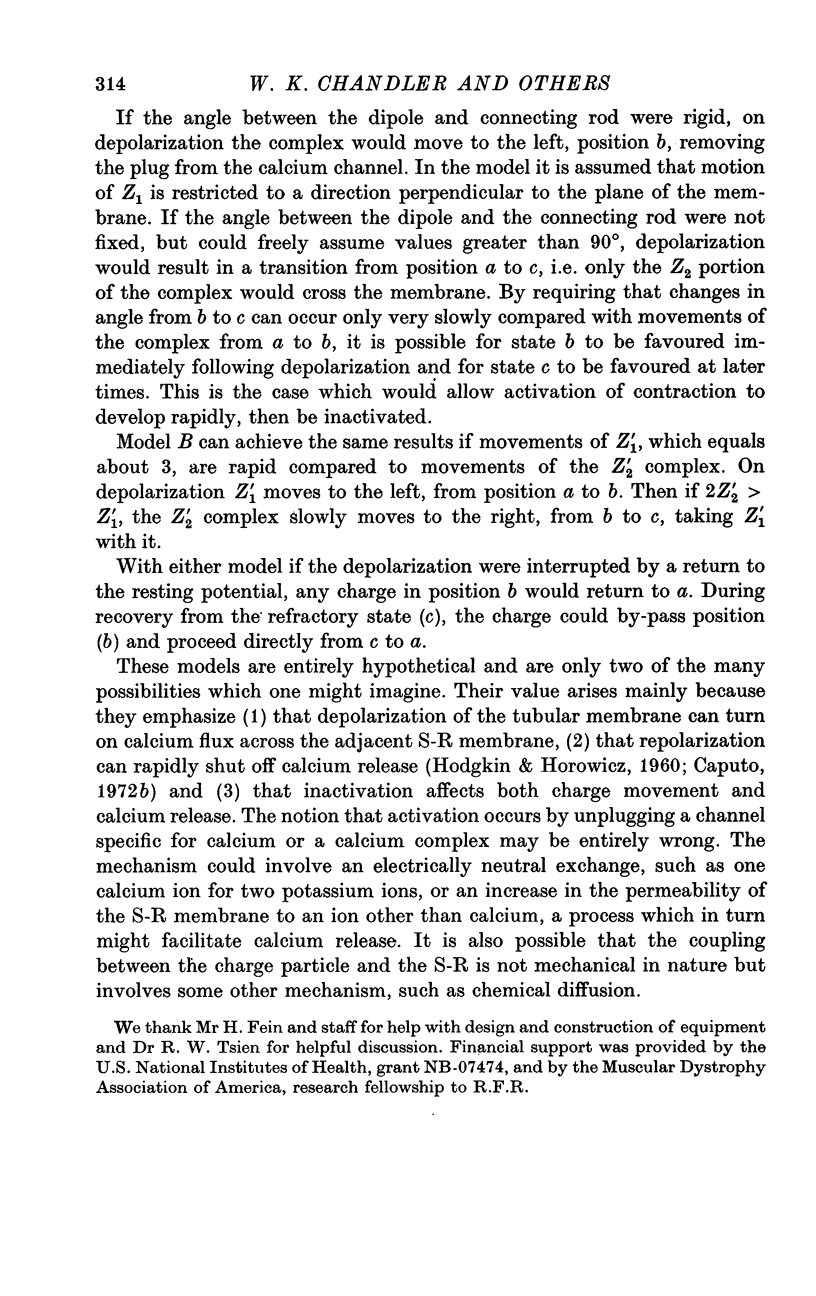
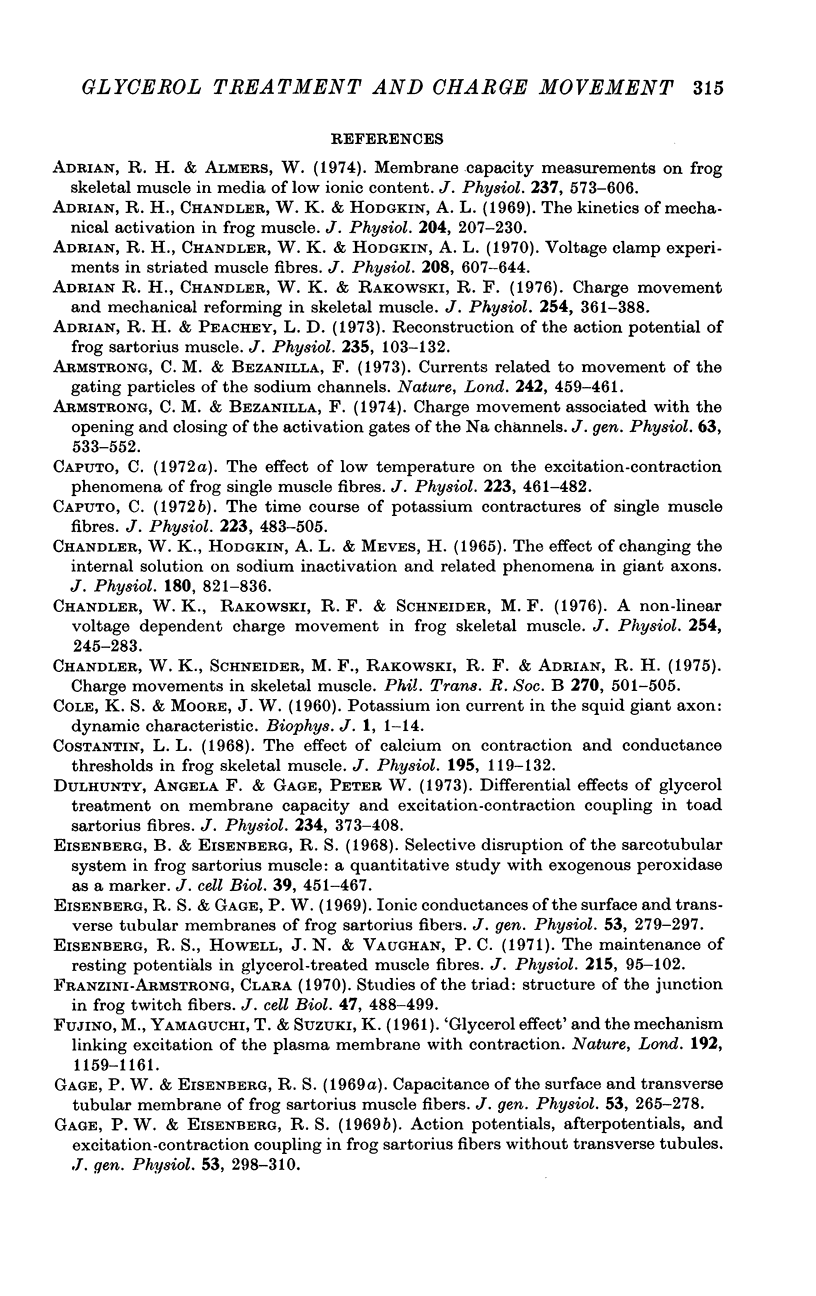
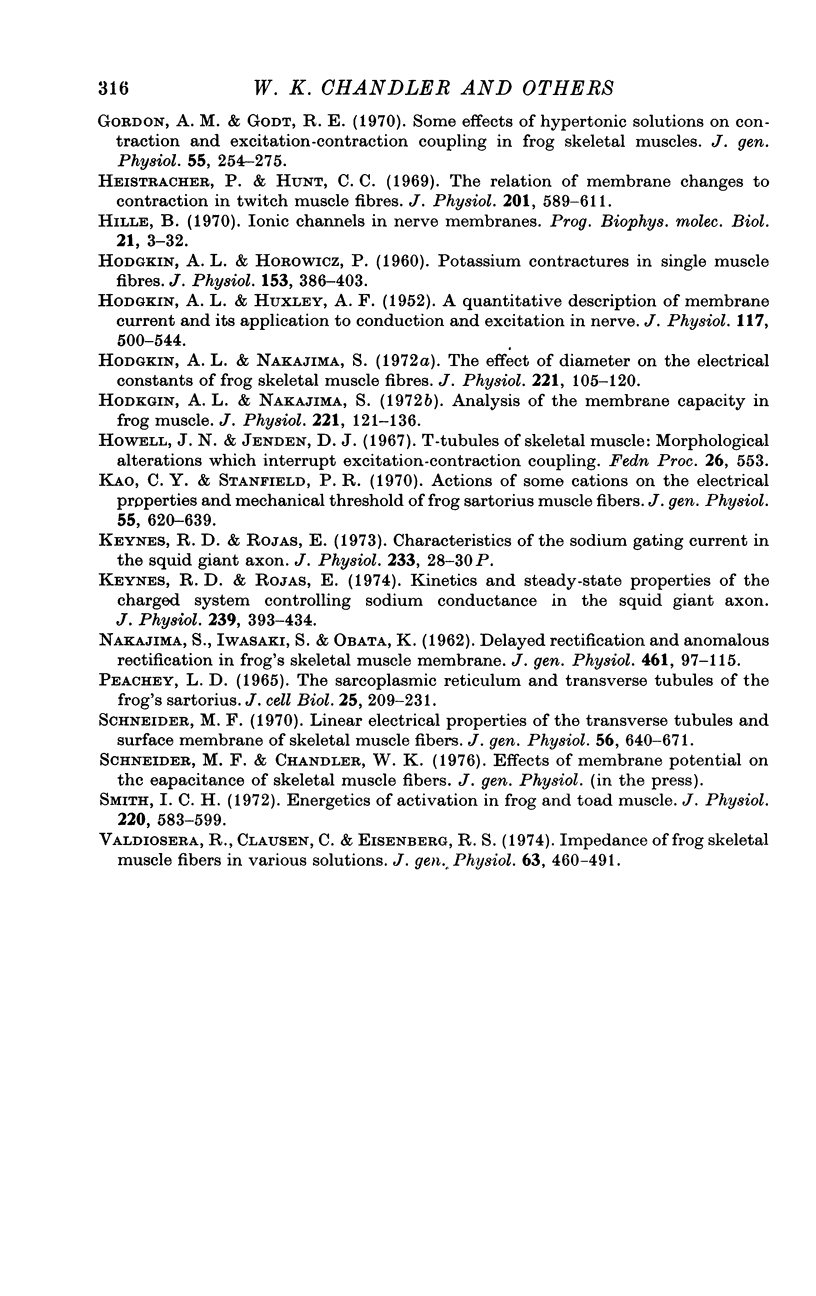
Selected References
These references are in PubMed. This may not be the complete list of references from this article.
- Adrian R. H., Almers W. Membrane capacity measurements on frog skeletal muscle in media of low ion content. J Physiol. 1974 Mar;237(3):573–605. doi: 10.1113/jphysiol.1974.sp010499. [DOI] [PMC free article] [PubMed] [Google Scholar]
- Adrian R. H., Chandler W. K., Hodgkin A. L. The kinetics of mechanical activation in frog muscle. J Physiol. 1969 Sep;204(1):207–230. doi: 10.1113/jphysiol.1969.sp008909. [DOI] [PMC free article] [PubMed] [Google Scholar]
- Adrian R. H., Chandler W. K., Hodgkin A. L. Voltage clamp experiments in striated muscle fibres. J Physiol. 1970 Jul;208(3):607–644. doi: 10.1113/jphysiol.1970.sp009139. [DOI] [PMC free article] [PubMed] [Google Scholar]
- Adrian R. H., Chandler W. K., Rakowski R. F. Charge movement and mechanical repriming in skeletal muscle. J Physiol. 1976 Jan;254(2):361–388. doi: 10.1113/jphysiol.1976.sp011236. [DOI] [PMC free article] [PubMed] [Google Scholar]
- Adrian R. H., Peachey L. D. Reconstruction of the action potential of frog sartorius muscle. J Physiol. 1973 Nov;235(1):103–131. doi: 10.1113/jphysiol.1973.sp010380. [DOI] [PMC free article] [PubMed] [Google Scholar]
- Armstrong C. M., Bezanilla F. Charge movement associated with the opening and closing of the activation gates of the Na channels. J Gen Physiol. 1974 May;63(5):533–552. doi: 10.1085/jgp.63.5.533. [DOI] [PMC free article] [PubMed] [Google Scholar]
- Armstrong C. M., Bezanilla F. Currents related to movement of the gating particles of the sodium channels. Nature. 1973 Apr 13;242(5398):459–461. doi: 10.1038/242459a0. [DOI] [PubMed] [Google Scholar]
- COLE K. S., MOORE J. W. Potassium ion current in the squid giant axon: dynamic characteristic. Biophys J. 1960 Sep;1:1–14. doi: 10.1016/s0006-3495(60)86871-3. [DOI] [PMC free article] [PubMed] [Google Scholar]
- Caputo C. The effect of low temperature on the excitation-contraction coupling phenomena of frog single muscle fibres. J Physiol. 1972 Jun;223(2):461–482. doi: 10.1113/jphysiol.1972.sp009858. [DOI] [PMC free article] [PubMed] [Google Scholar]
- Caputo C. The time course of potassium contractures of single muscle fibres. J Physiol. 1972 Jun;223(2):483–505. doi: 10.1113/jphysiol.1972.sp009859. [DOI] [PMC free article] [PubMed] [Google Scholar]
- Chandler W. K., Hodgkin A. L., Meves H. The effect of changing the internal solution on sodium inactivation and related phenomena in giant axons. J Physiol. 1965 Oct;180(4):821–836. doi: 10.1113/jphysiol.1965.sp007733. [DOI] [PMC free article] [PubMed] [Google Scholar]
- Chandler W. K., Rakowski R. F., Schneider M. F. A non-linear voltage dependent charge movement in frog skeletal muscle. J Physiol. 1976 Jan;254(2):245–283. doi: 10.1113/jphysiol.1976.sp011232. [DOI] [PMC free article] [PubMed] [Google Scholar]
- Chandler W. K., Schneider M. F., Rakowski R. F., Adrian R. H. Charge movements in skeletal muscle. Philos Trans R Soc Lond B Biol Sci. 1975 Jun 10;270(908):501–505. doi: 10.1098/rstb.1975.0026. [DOI] [PubMed] [Google Scholar]
- Costantin L. L. The effect o f calcium on contraction and conductance thresholds in frog skeletal muscle. J Physiol. 1968 Mar;195(1):119–132. doi: 10.1113/jphysiol.1968.sp008450. [DOI] [PMC free article] [PubMed] [Google Scholar]
- Dulhunty A. F., Gage P. W. Differential effects of glycerol treatment on membrane capacity and excitation-contraction coupling in toad sartorius fibres. J Physiol. 1973 Oct;234(2):373–408. doi: 10.1113/jphysiol.1973.sp010350. [DOI] [PMC free article] [PubMed] [Google Scholar]
- Eisenberg B., Eisenberg R. S. Selective disruption of the sarcotubular system in frog sartorius muscle. A quantitative study with exogenous peroxidase as a marker. J Cell Biol. 1968 Nov;39(2):451–467. doi: 10.1083/jcb.39.2.451. [DOI] [PMC free article] [PubMed] [Google Scholar]
- Eisenberg R. S., Gage P. W. Ionic conductances of the surface and transverse tubular membranes of frog sartorius fibers. J Gen Physiol. 1969 Mar;53(3):279–297. doi: 10.1085/jgp.53.3.279. [DOI] [PMC free article] [PubMed] [Google Scholar]
- Eisenberg R. S., Howell J. N., Vaughan P. C. The maintenance of resting potentials in glycerol-treated muscle fibres. J Physiol. 1971 May;215(1):95–102. doi: 10.1113/jphysiol.1971.sp009459. [DOI] [PMC free article] [PubMed] [Google Scholar]
- FUJINO M., YAMAGUCHI T., SUZUKI K. 'Glycerol effect' and the mechanism linking excitation of the plasma membrane with contraction. Nature. 1961 Dec 23;192:1159–1161. doi: 10.1038/1921159a0. [DOI] [PubMed] [Google Scholar]
- Franzini-Armstrong C. STUDIES OF THE TRIAD : I. Structure of the Junction in Frog Twitch Fibers. J Cell Biol. 1970 Nov 1;47(2):488–499. doi: 10.1083/jcb.47.2.488. [DOI] [PMC free article] [PubMed] [Google Scholar]
- Gage P. W., Eisenberg R. S. Action potentials, afterpotentials, and excitation-contraction coupling in frog sartorius fibers without transverse tubules. J Gen Physiol. 1969 Mar;53(3):298–310. doi: 10.1085/jgp.53.3.298. [DOI] [PMC free article] [PubMed] [Google Scholar]
- Gage P. W., Eisenberg R. S. Capacitance of the surface and transverse tubular membrane of frog sartorius muscle fibers. J Gen Physiol. 1969 Mar;53(3):265–278. doi: 10.1085/jgp.53.3.265. [DOI] [PMC free article] [PubMed] [Google Scholar]
- Gordon A. M., Godt R. E. Some effects of hypertonic solutions on contraction and excitation-contraction coupling in frog skeletal muscles. J Gen Physiol. 1970 Feb;55(2):254–275. doi: 10.1085/jgp.55.2.254. [DOI] [PMC free article] [PubMed] [Google Scholar]
- HODGKIN A. L., HOROWICZ P. Potassium contractures in single muscle fibres. J Physiol. 1960 Sep;153:386–403. doi: 10.1113/jphysiol.1960.sp006541. [DOI] [PMC free article] [PubMed] [Google Scholar]
- HODGKIN A. L., HUXLEY A. F. A quantitative description of membrane current and its application to conduction and excitation in nerve. J Physiol. 1952 Aug;117(4):500–544. doi: 10.1113/jphysiol.1952.sp004764. [DOI] [PMC free article] [PubMed] [Google Scholar]
- Heistracher P., Hunt C. C. The relation of membrane changes ot contraction in twitch muscle fibres. J Physiol. 1969 May;201(3):589–611. doi: 10.1113/jphysiol.1969.sp008774. [DOI] [PMC free article] [PubMed] [Google Scholar]
- Hodgkin A. L., Nakajima S. Analysis of the membrane capacity in frog muscle. J Physiol. 1972 Feb;221(1):121–136. doi: 10.1113/jphysiol.1972.sp009743. [DOI] [PMC free article] [PubMed] [Google Scholar]
- Hodgkin A. L., Nakajima S. The effect of diameter on the electrical constants of frog skeletal muscle fibres. J Physiol. 1972 Feb;221(1):105–120. doi: 10.1113/jphysiol.1972.sp009742. [DOI] [PMC free article] [PubMed] [Google Scholar]
- Kao C. Y., Stanfield P. R. Actions of some cations on the electrical properties and mechanical threshold of frog sartorius muscle fibers. J Gen Physiol. 1970 May;55(5):620–639. doi: 10.1085/jgp.55.5.620. [DOI] [PMC free article] [PubMed] [Google Scholar]
- Keynes R. D., Rojas E. Kinetics and steady-state properties of the charged system controlling sodium conductance in the squid giant axon. J Physiol. 1974 Jun;239(2):393–434. doi: 10.1113/jphysiol.1974.sp010575. [DOI] [PMC free article] [PubMed] [Google Scholar]
- NAKAJIMA S., IWASAKI S., OBATA K. Delayed rectification and anomalous rectification in frog's skeletal muscle membrane. J Gen Physiol. 1962 Sep;46:97–115. doi: 10.1085/jgp.46.1.97. [DOI] [PMC free article] [PubMed] [Google Scholar]
- Peachey L. D. The sarcoplasmic reticulum and transverse tubules of the frog's sartorius. J Cell Biol. 1965 Jun;25(3 Suppl):209–231. doi: 10.1083/jcb.25.3.209. [DOI] [PubMed] [Google Scholar]
- Schneider M. F. Linear electrical properties of the transverse tubules and surface membrane of skeletal muscle fibers. J Gen Physiol. 1970 Nov;56(5):640–671. doi: 10.1085/jgp.56.5.640. [DOI] [PMC free article] [PubMed] [Google Scholar]
- Smith I. C. Energetics of activation in frog and toad muscle. J Physiol. 1972 Feb;220(3):583–599. doi: 10.1113/jphysiol.1972.sp009724. [DOI] [PMC free article] [PubMed] [Google Scholar]
- Valdiosera R., Clausen C., Eisenberg R. S. Impedance of frog skeletal muscle fibers in various solutions. J Gen Physiol. 1974 Apr;63(4):460–491. doi: 10.1085/jgp.63.4.460. [DOI] [PMC free article] [PubMed] [Google Scholar]