Abstract
1. Rohon-Beard cells in the spinal cord of Xenopus laevis tadpoles have been studied in animals 4-days to 2-weeks-old (Nieuwkoop & Faber, 1956, stages 45-49). These neurones have an unusually large resting membrane potential of -88 mV, in Ringer solution containing 3-0 mM K+. 2. Their resting potential (R..) depends on the concentration gradient of K+ across the cell membrane. These cells follow the prediction of the Nernst equation for a K+-selective electrode, down to external K+ concentrations as low as 1-0 mM (R.P. -118 mV). 3. The resting potentials of muscle cells in these animals exhibit the same dependence on external [K+], as has been shown previously. 4. Rohon-Beard cells can be driven antidromically, bu stimulation of the anterior end of the spinal cord with brief current pulses through a suction electrode. Antidromic action potentials fail to invade the cell body with repeated stimulation at 1Hz. 5. Even when impulses fail to invade Rohon-Beard somata, slow depolarizations can be produced by single shocks or trains of shocks which cause impulse activity in other neurones. The response can be observed to a single stimulus or to a train of stimuli. The magnitude of the depolarization is graded, depending on the number of stimuli and the frequency of stimulation. 6. Support is presented for the hypothesis that the slow depolarization in Rohon-Beard cells is mediated by the release of K+ into their environment by the impulse activity of neighbouring neurones. The slow depolarization increases in solutions containing 1-5 mM-K+, and decreases in solutions containing 6-0 mM-K+. The changes are in quantitative agreement with those anticipated by theory. 7. The slow depolarization is unlikely to be due to a conductance change produced by a synaptic transmitter, since hyperpolarization and depolarization of the Rohon-Beard cell with injected current do not change the amplitude of the response. Further, low Ca-high Mg solutions which block neuromuscular transmission do not block the response. 8. The possible role of the slow depolarizing response in the physiological activity of these neurones is discussed.
Full text
PDF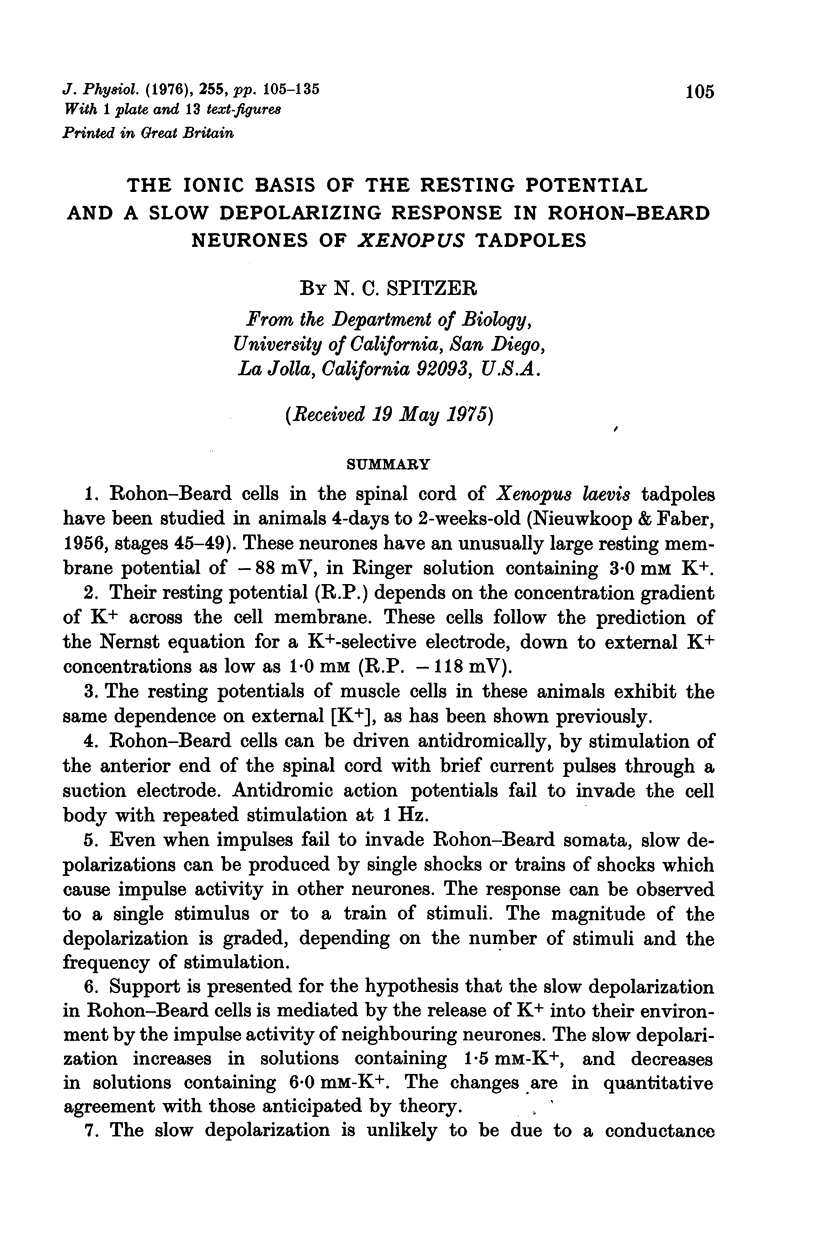
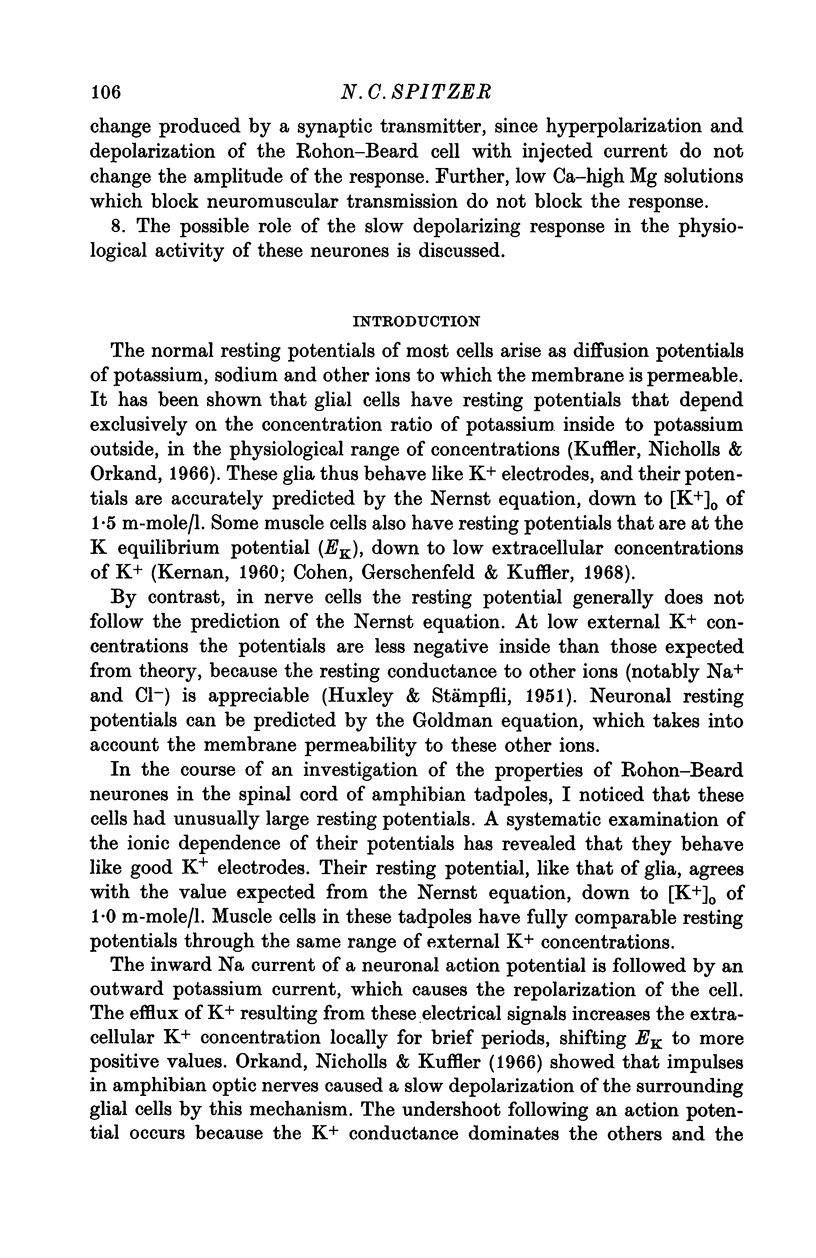
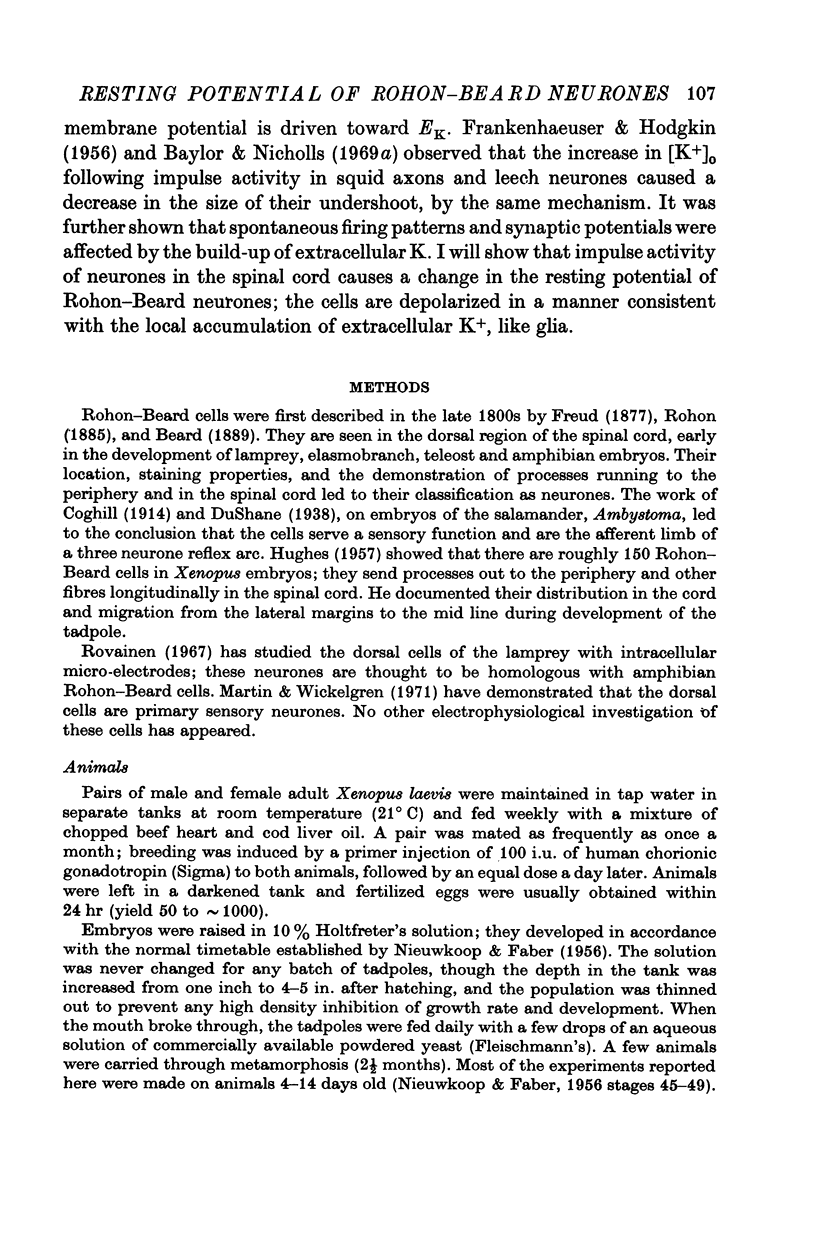
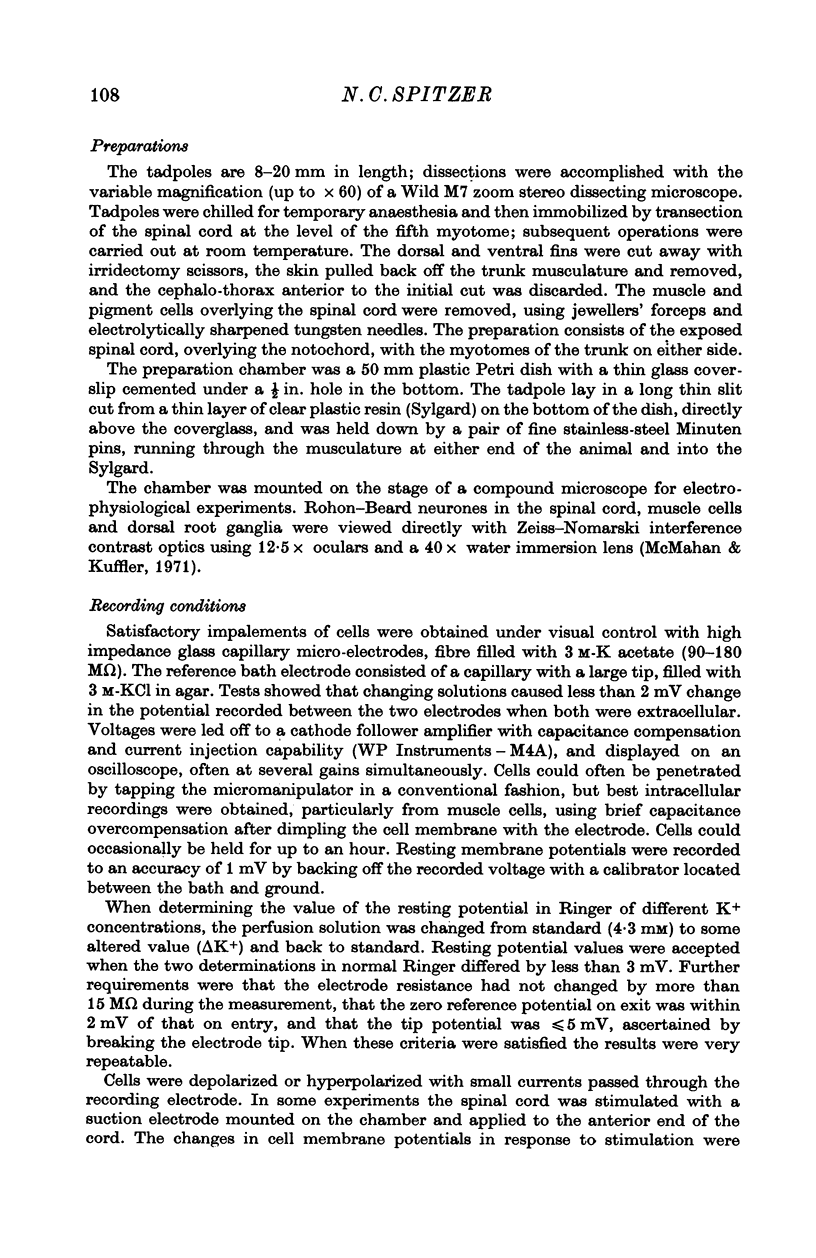
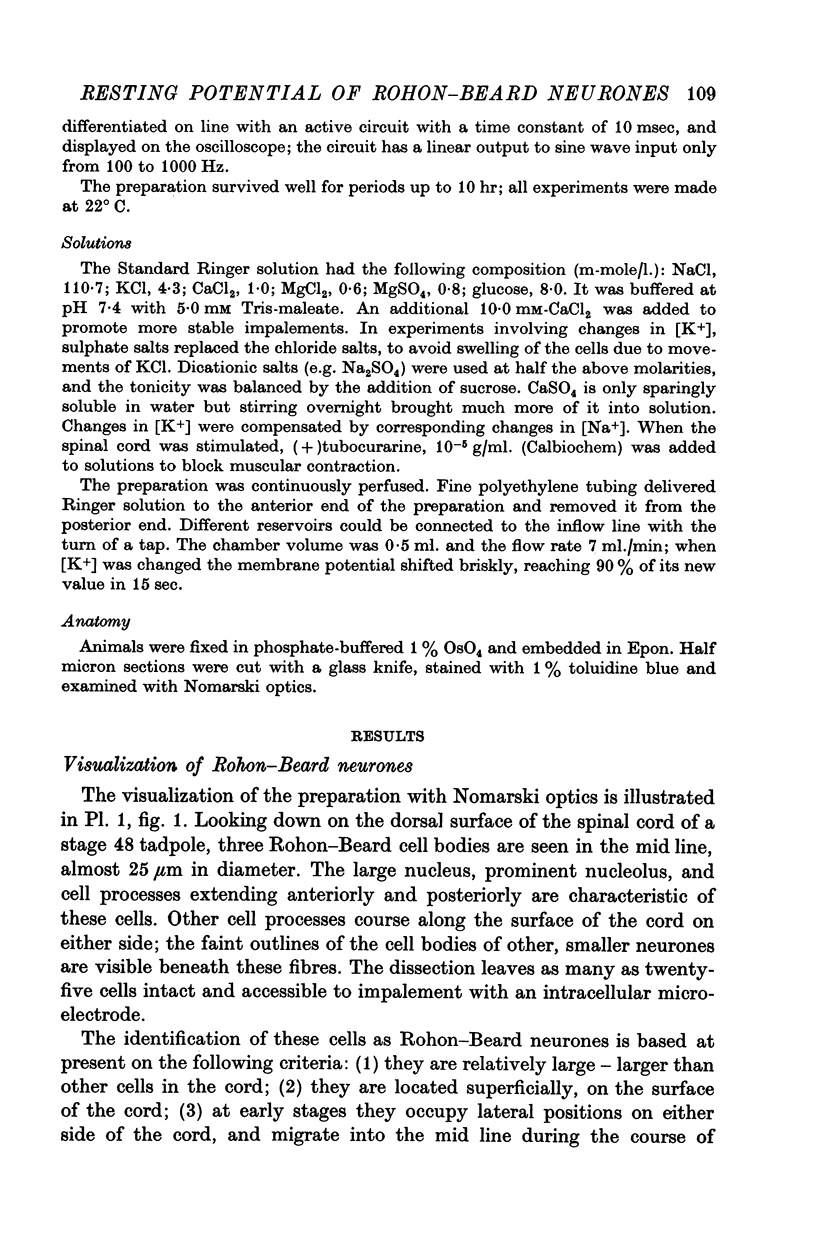
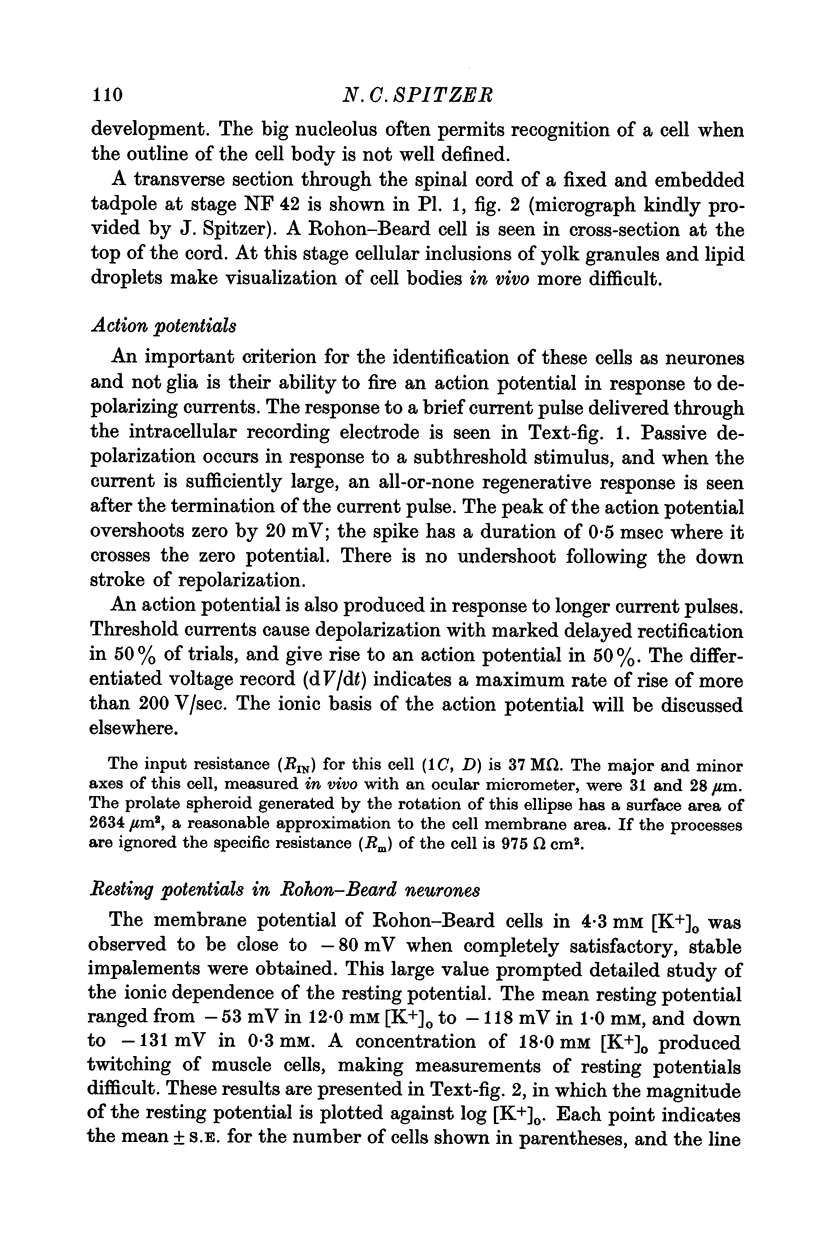
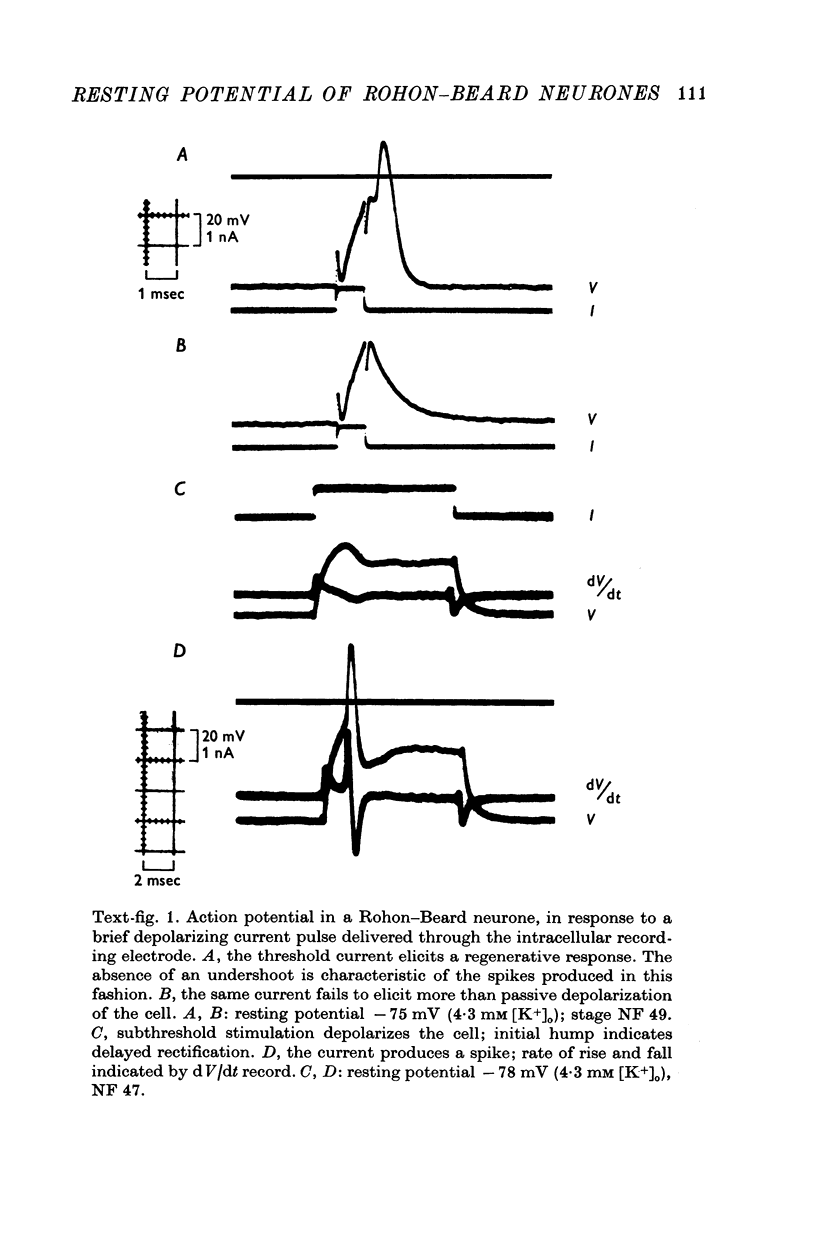
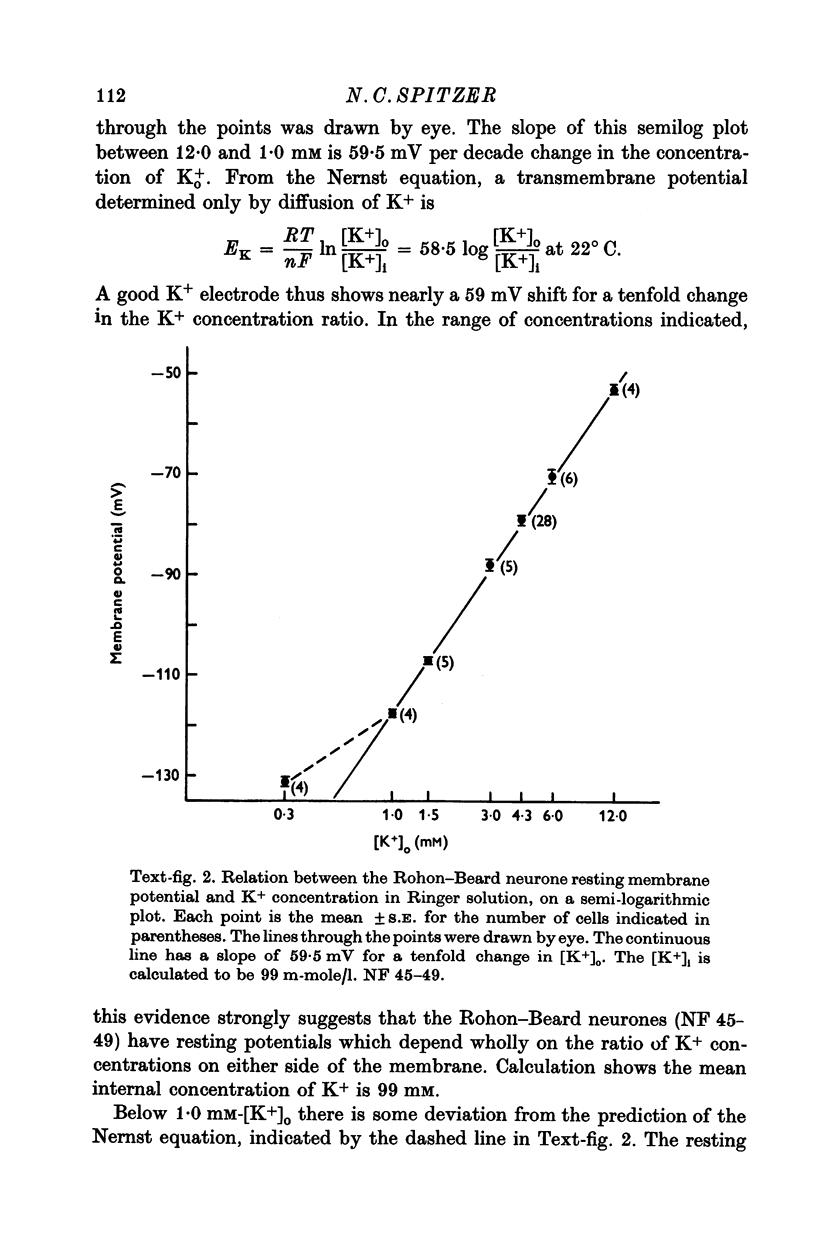
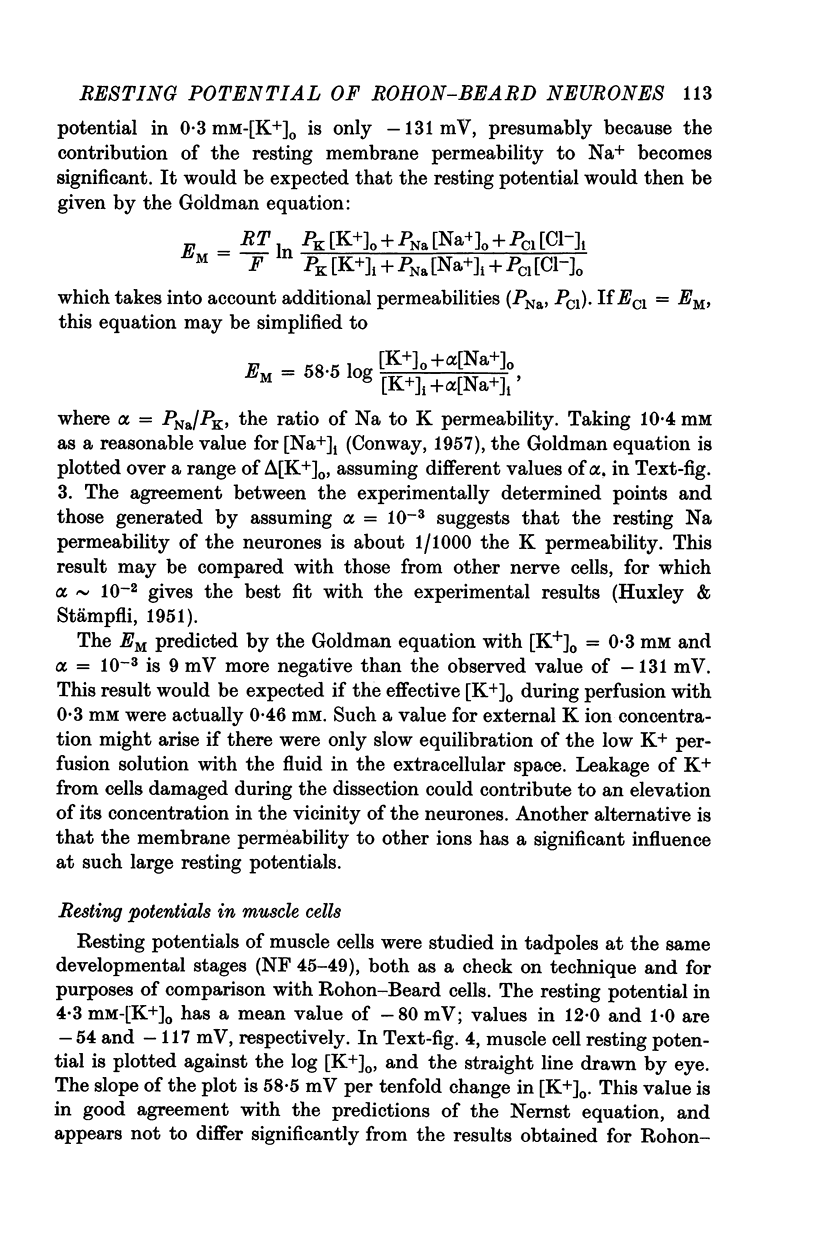
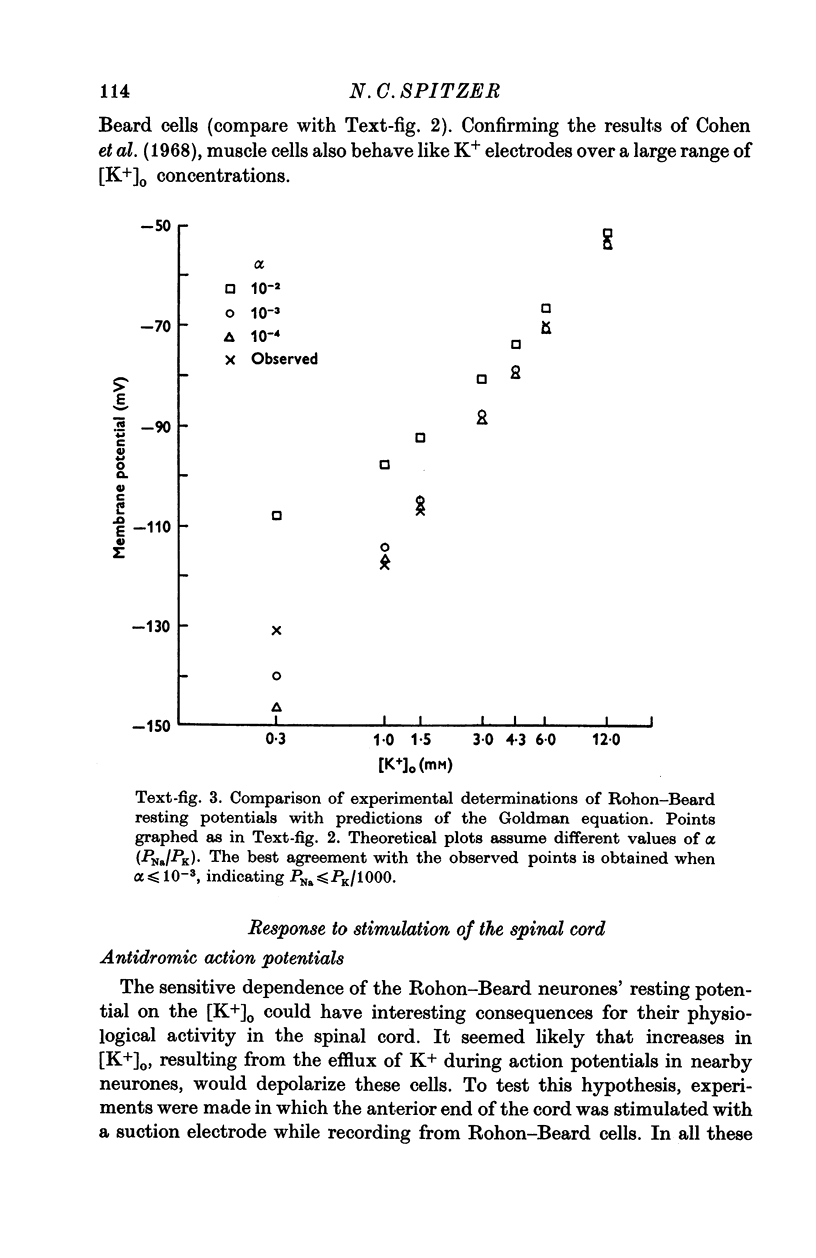
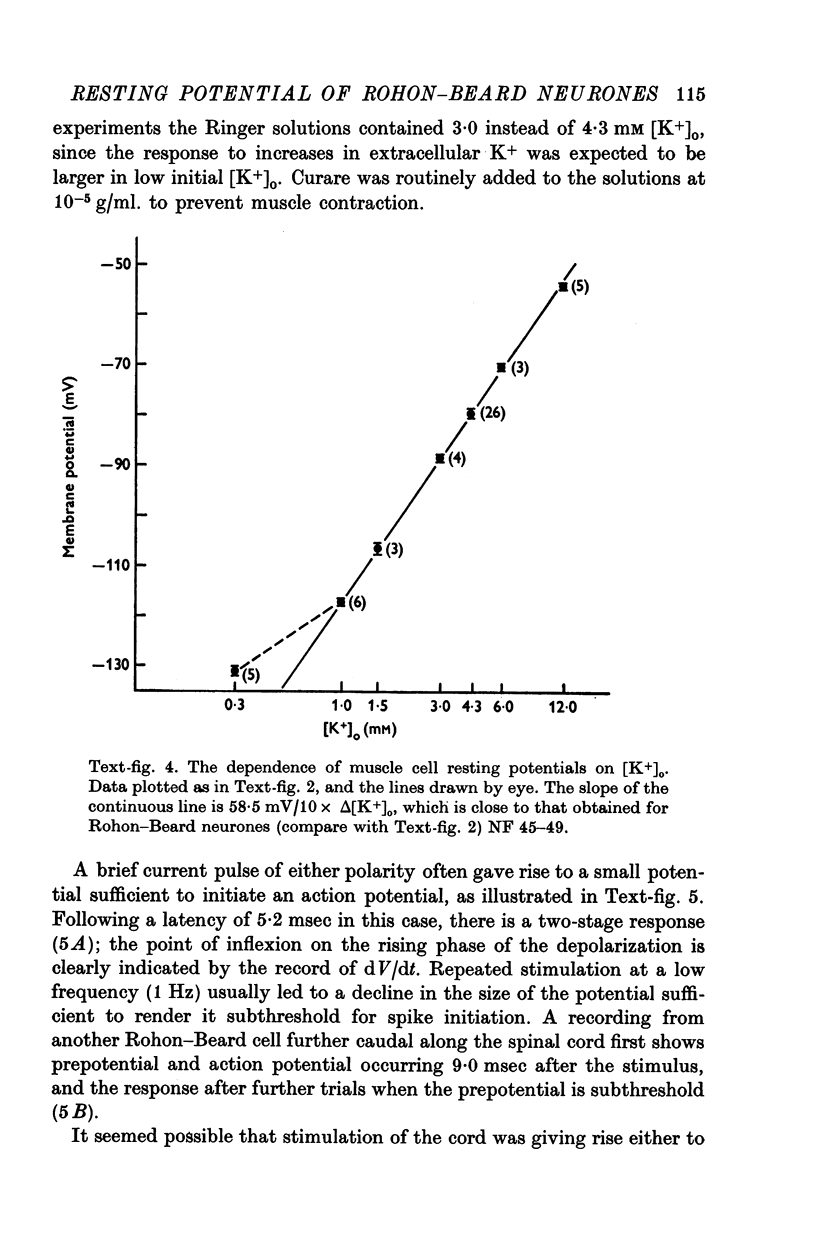
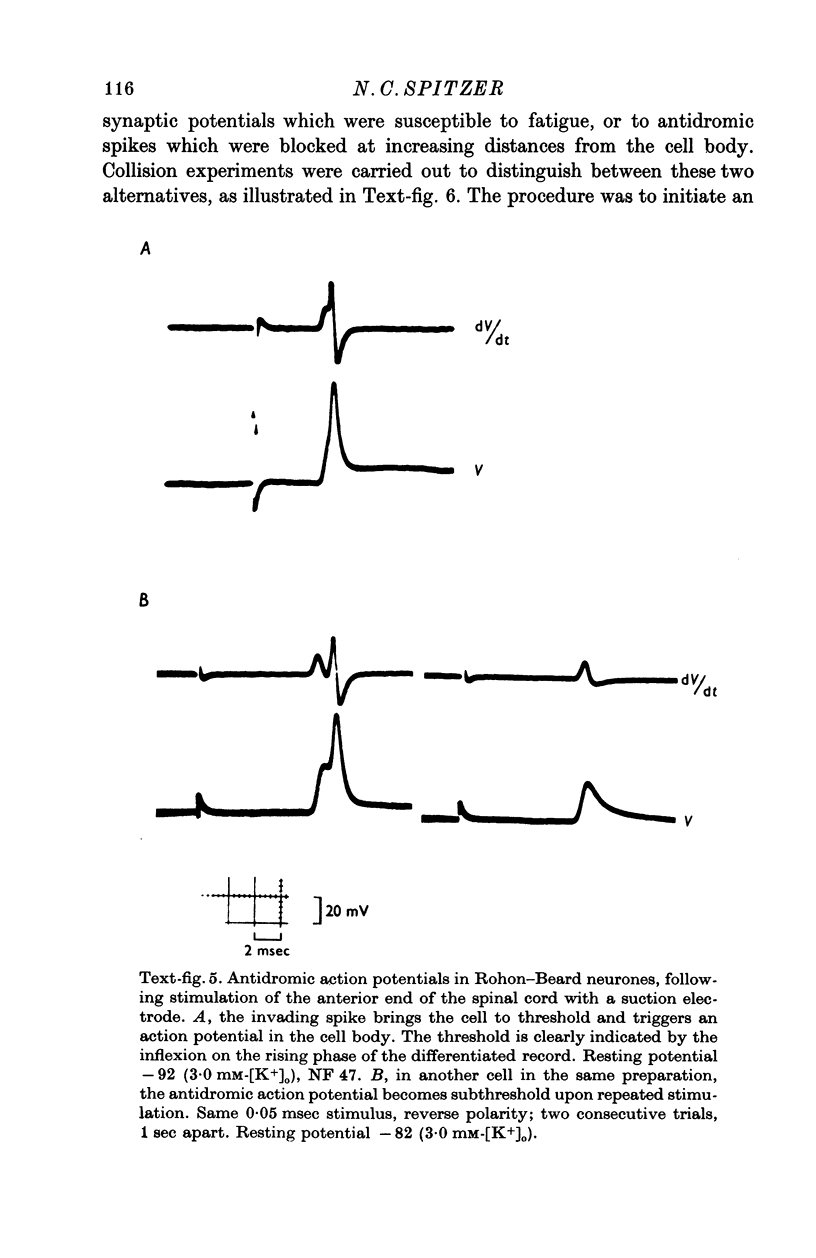
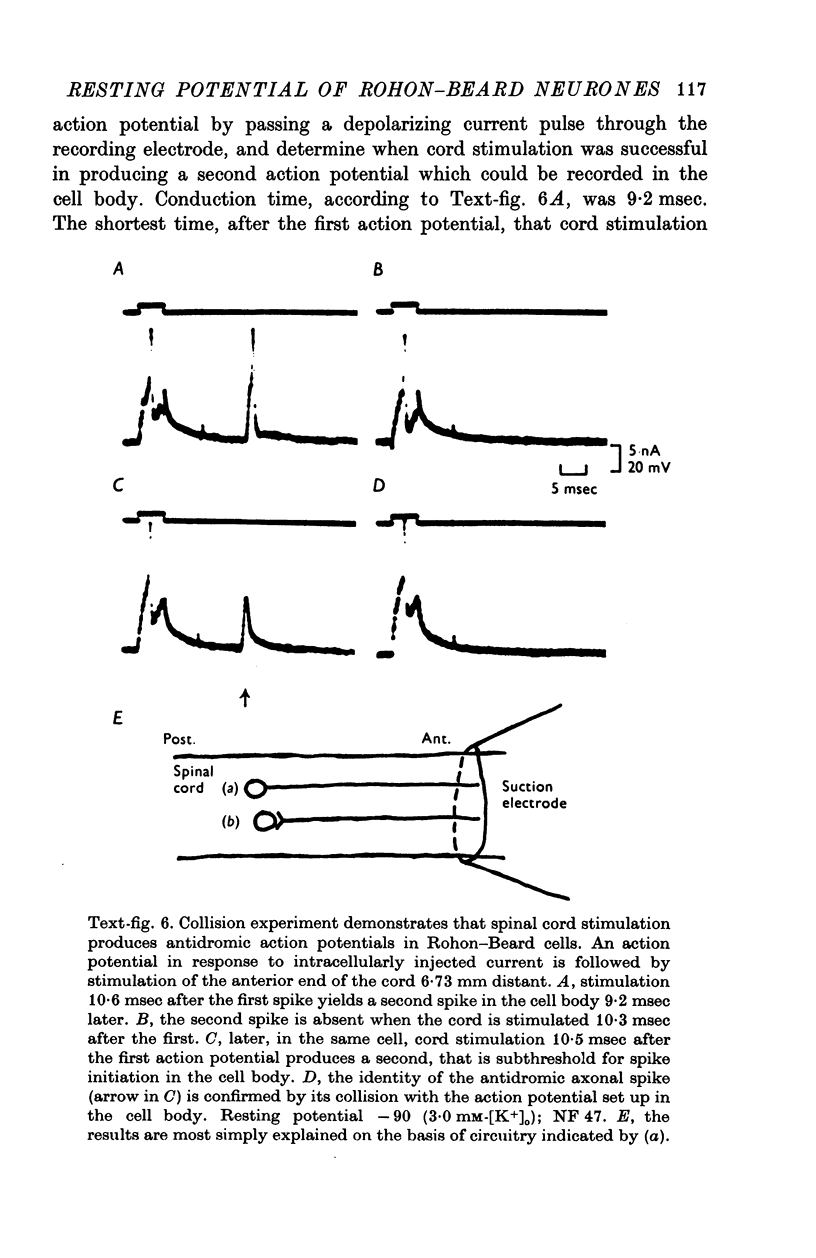
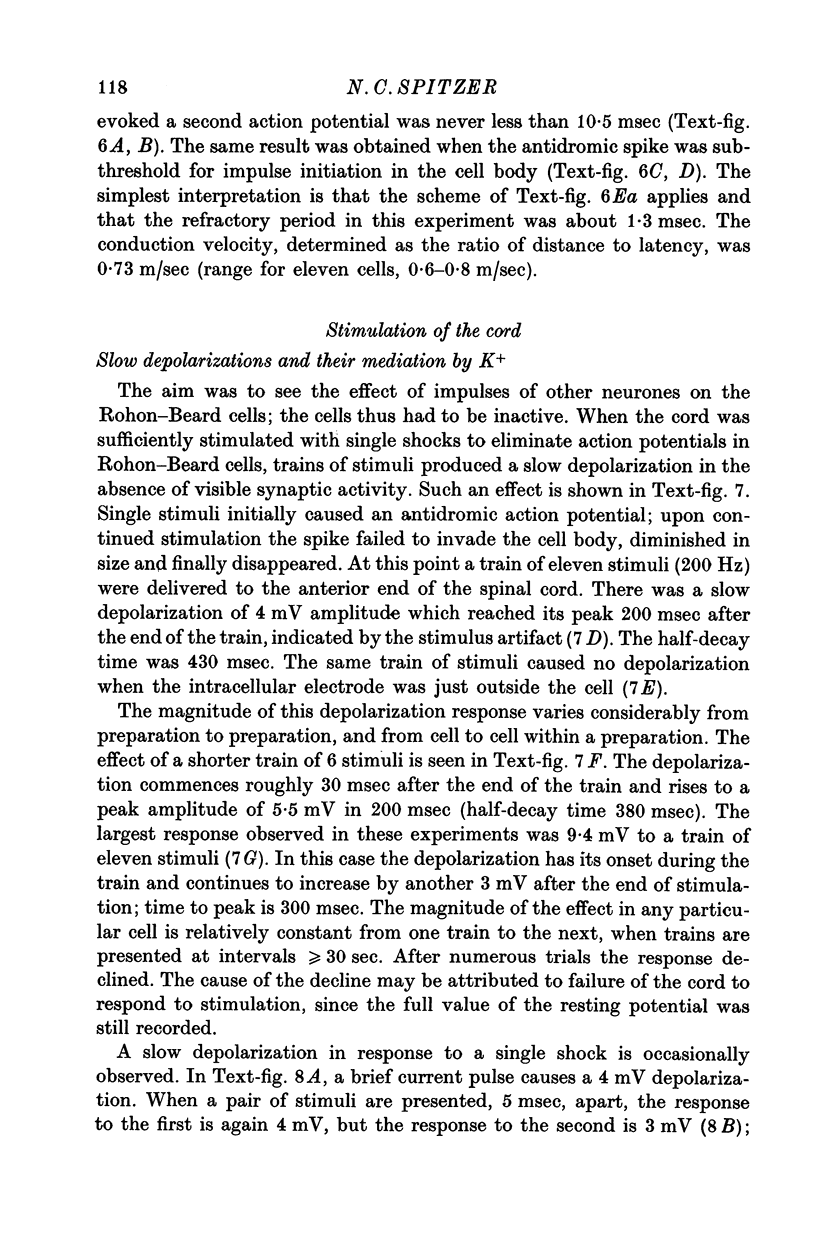
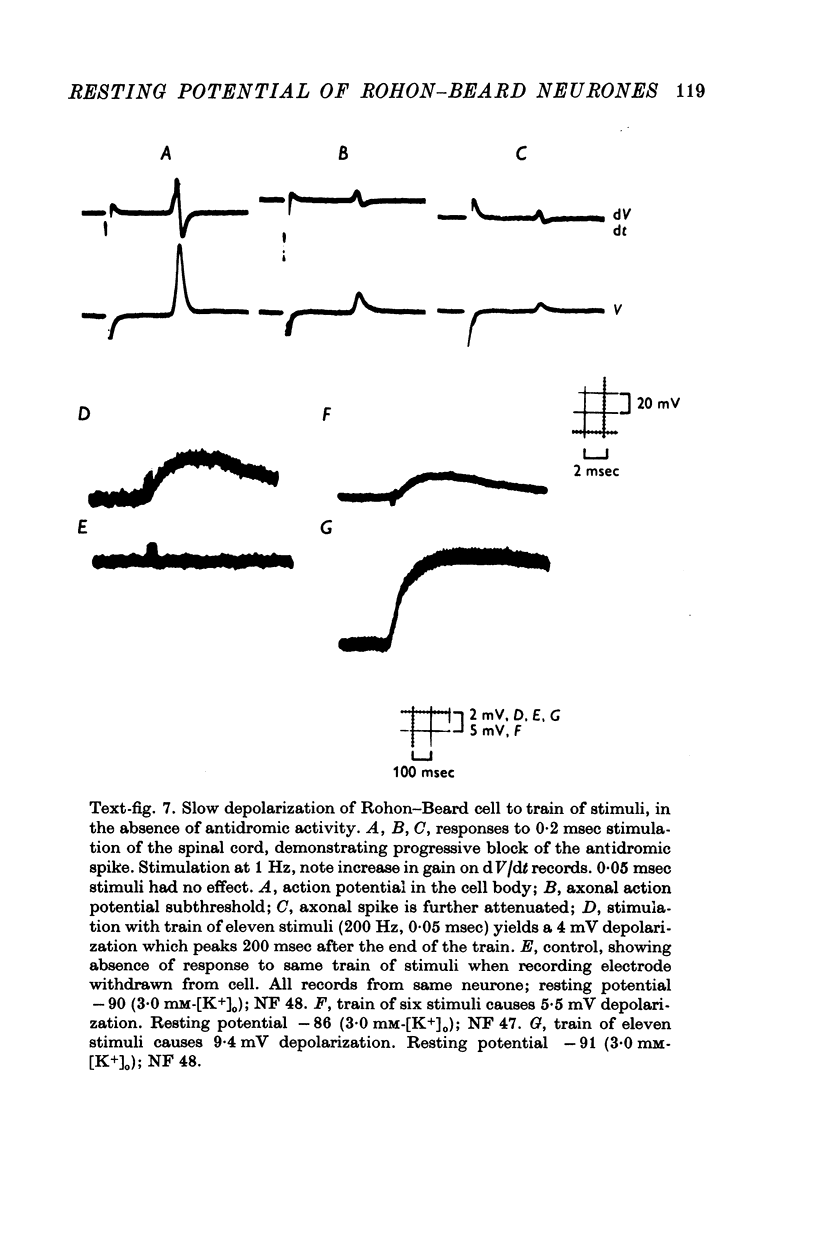
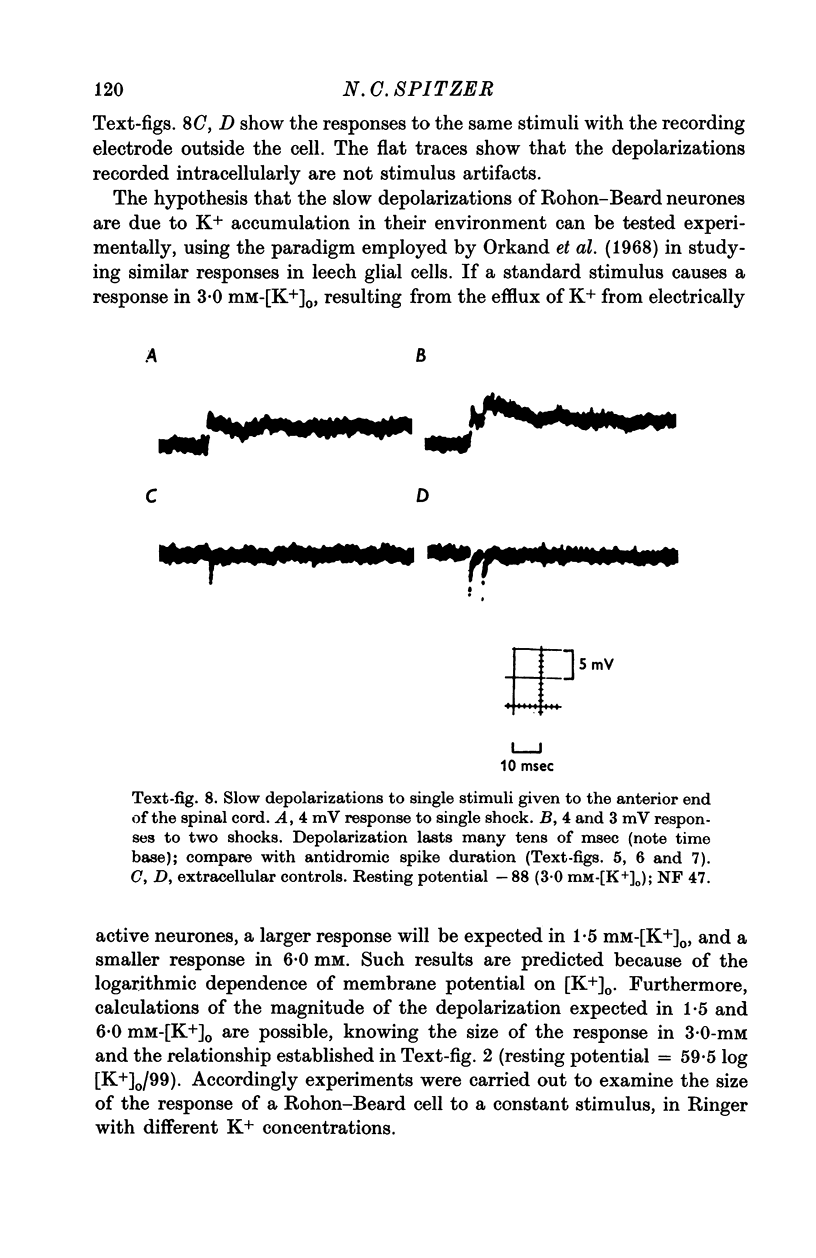
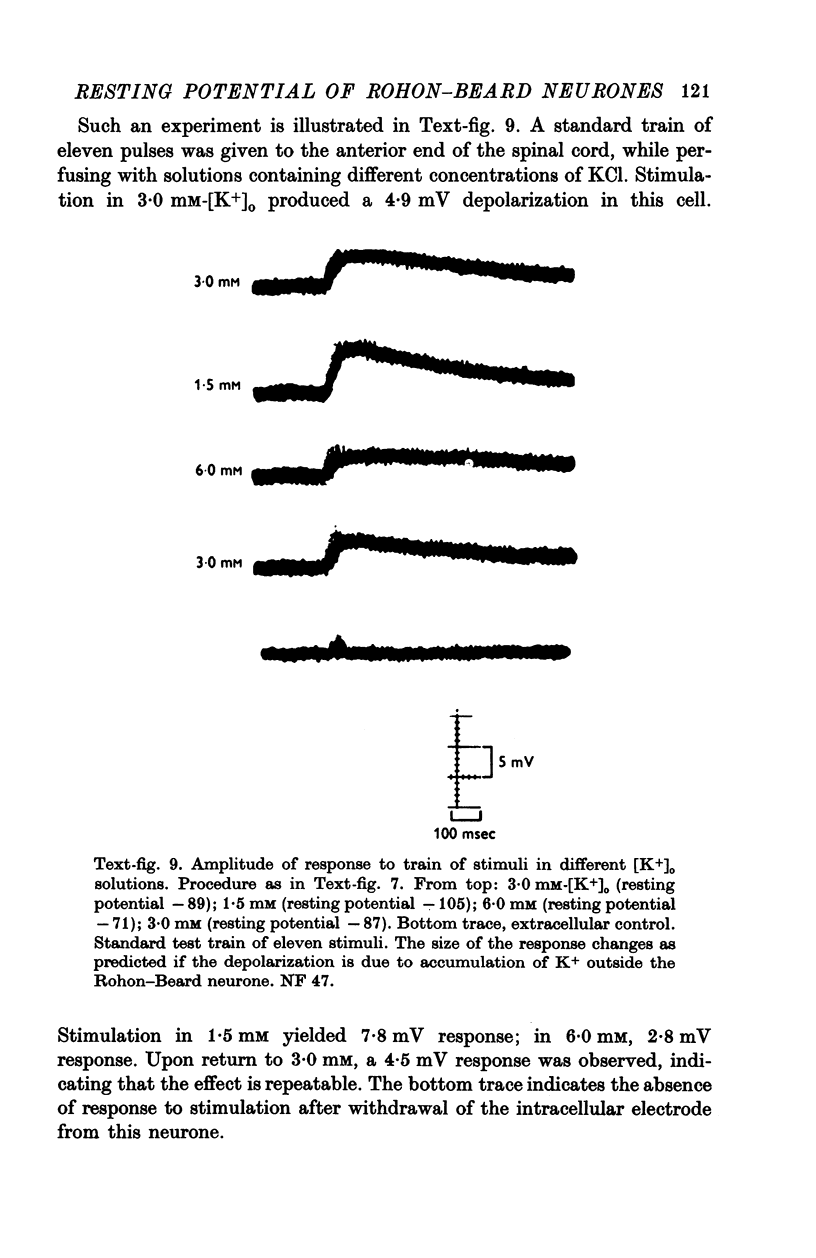
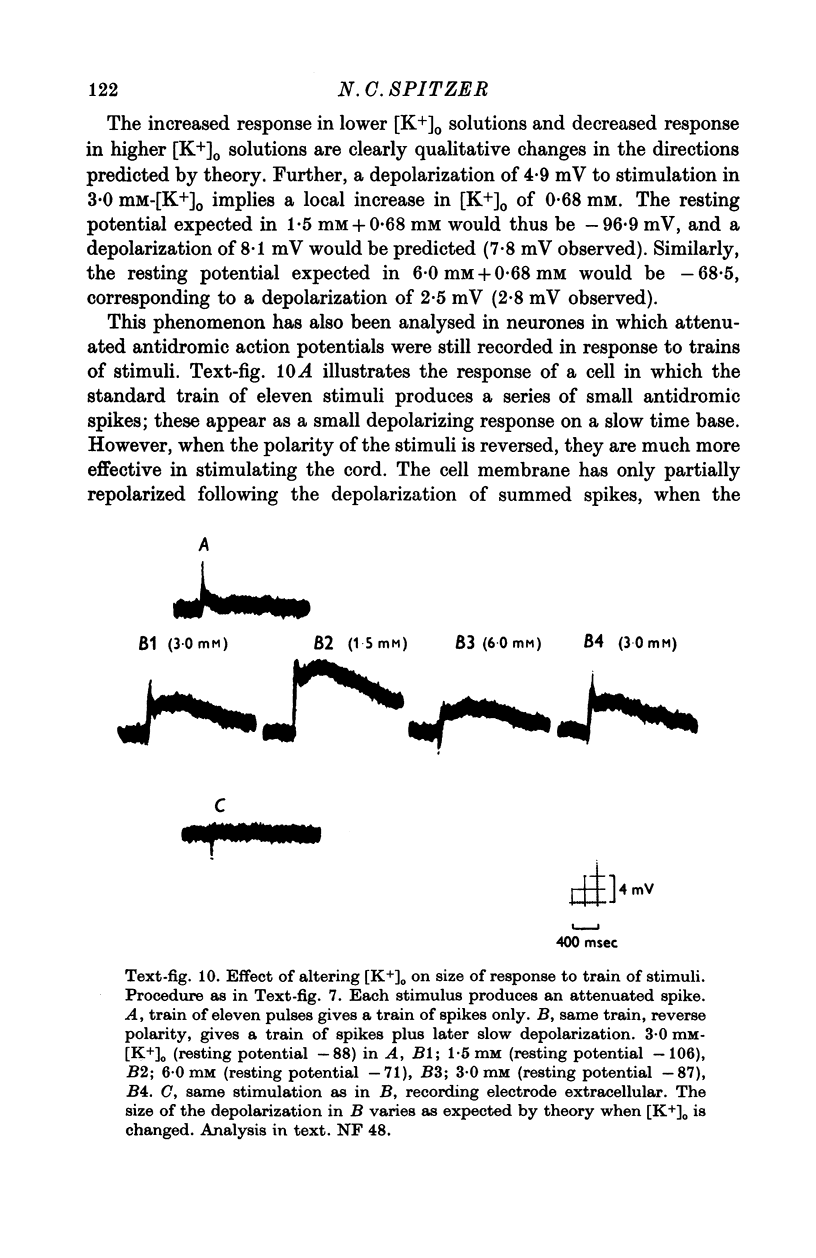
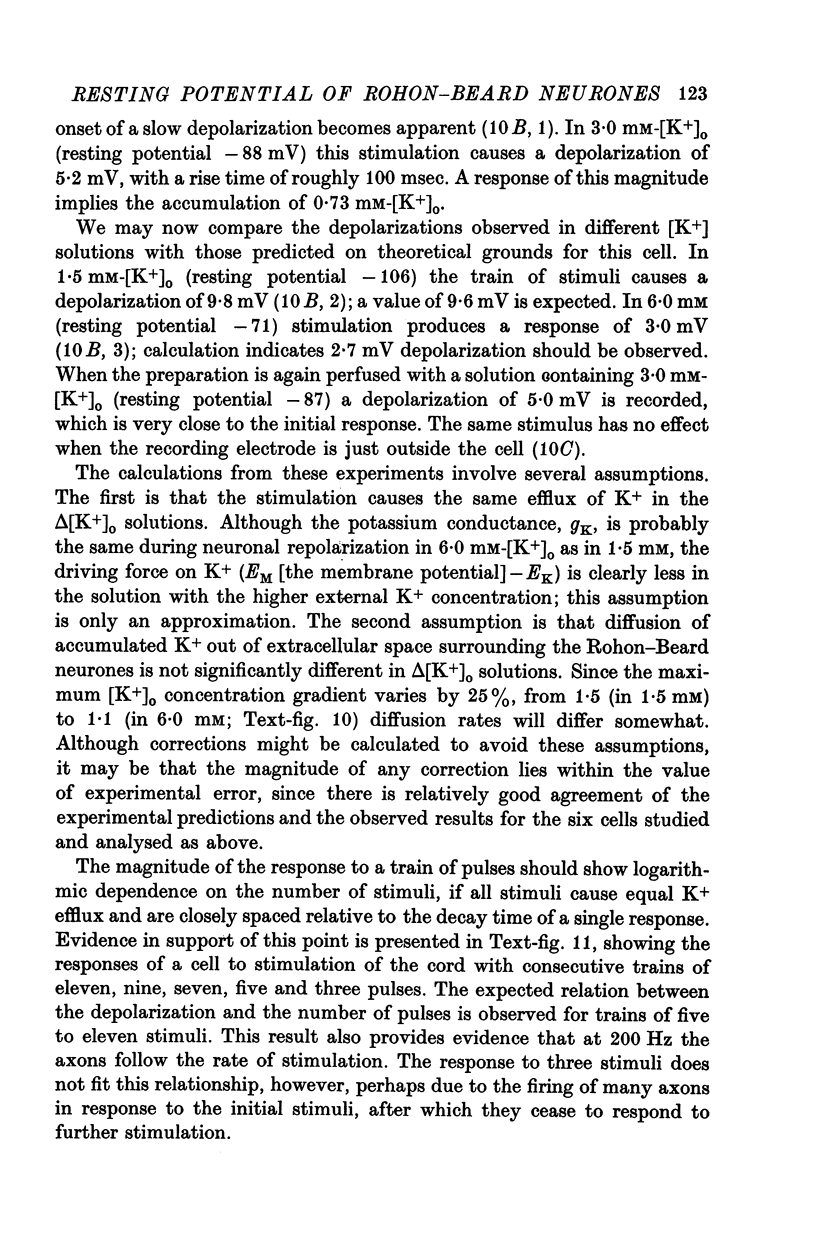
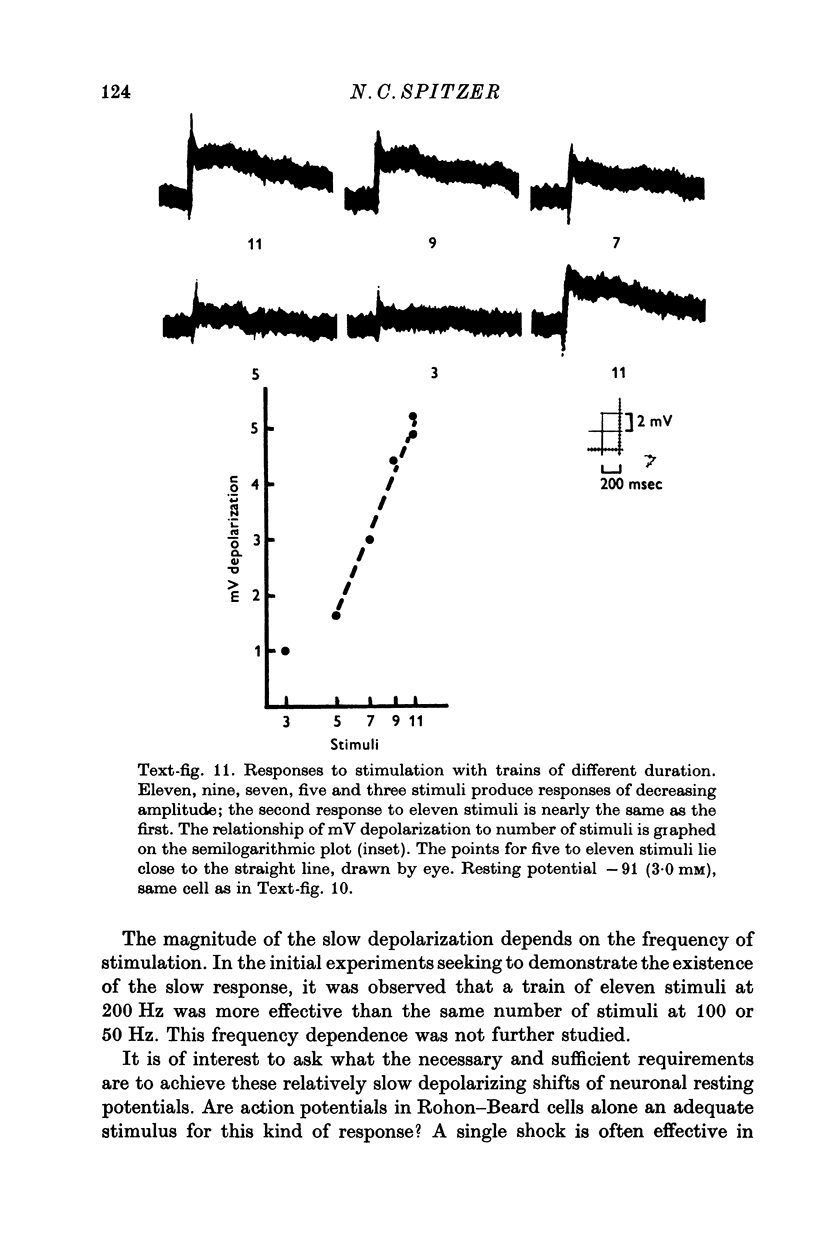
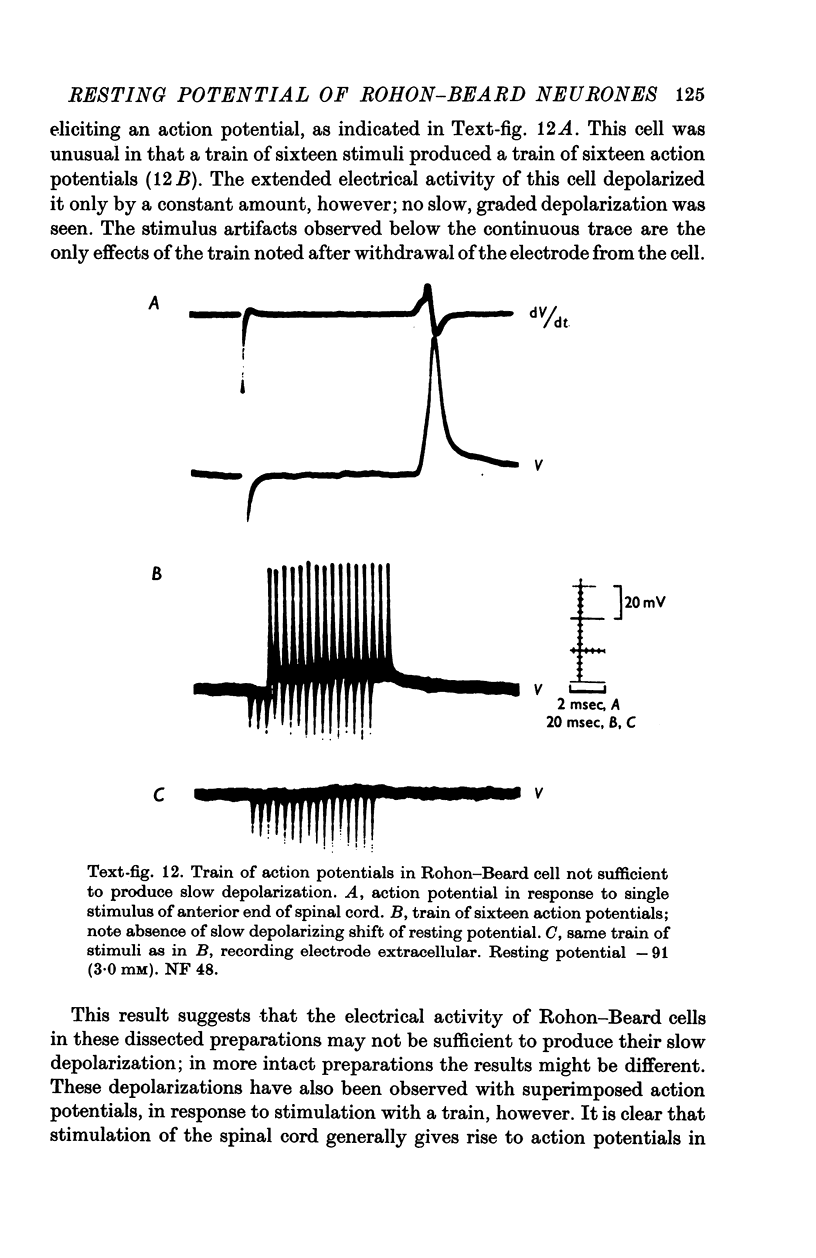
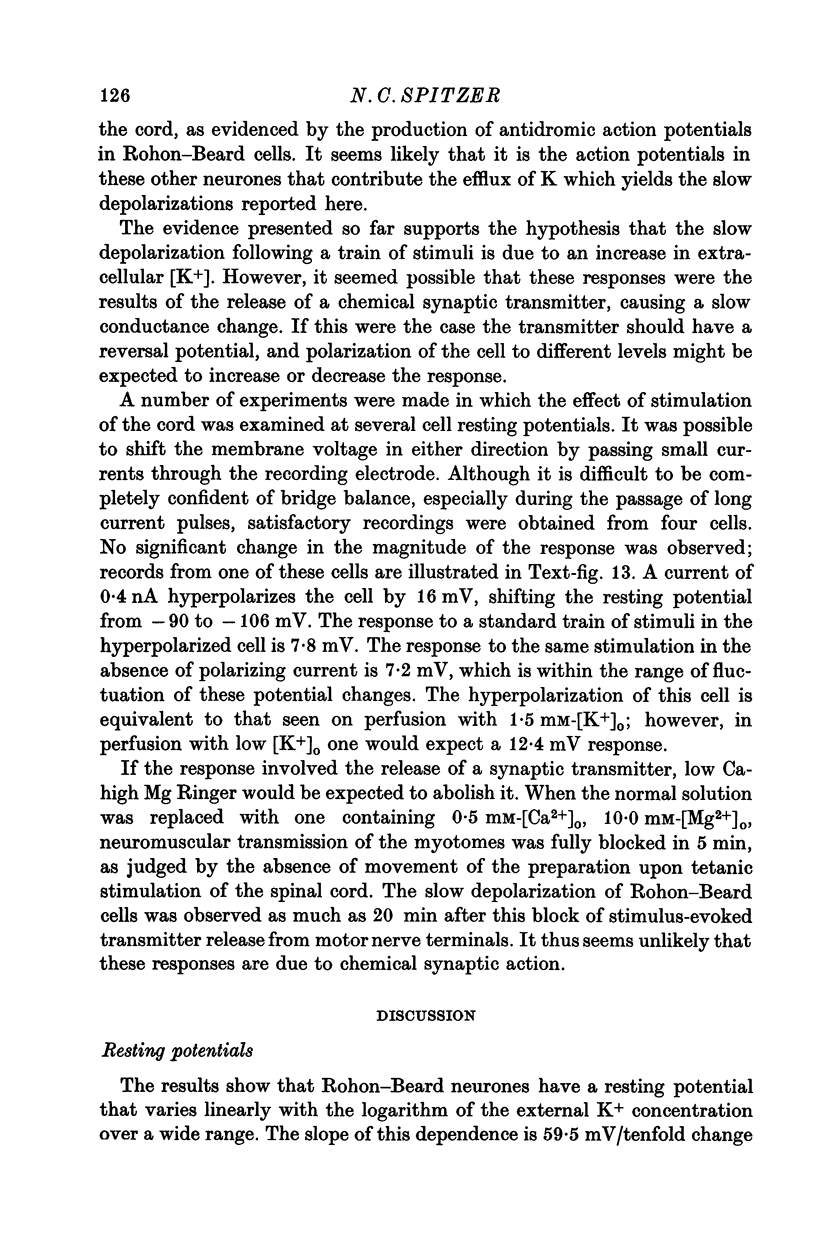
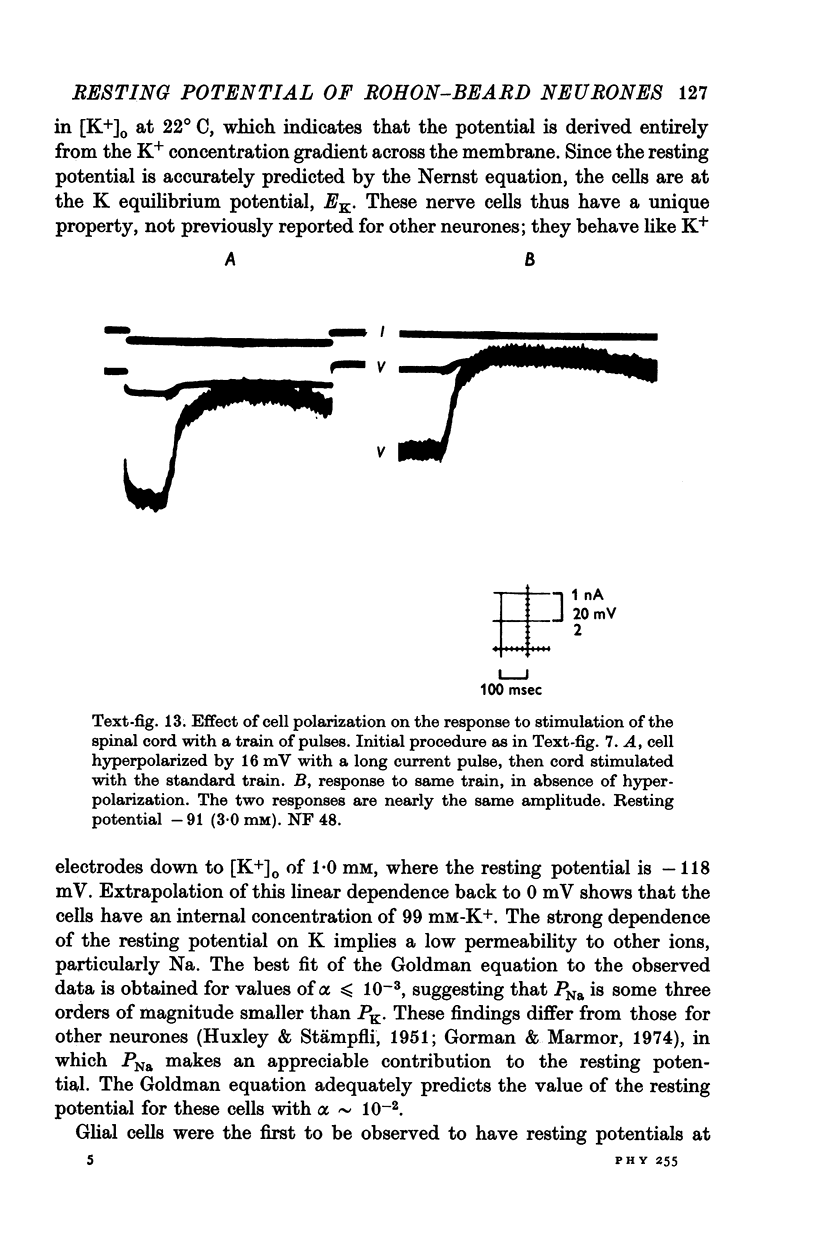
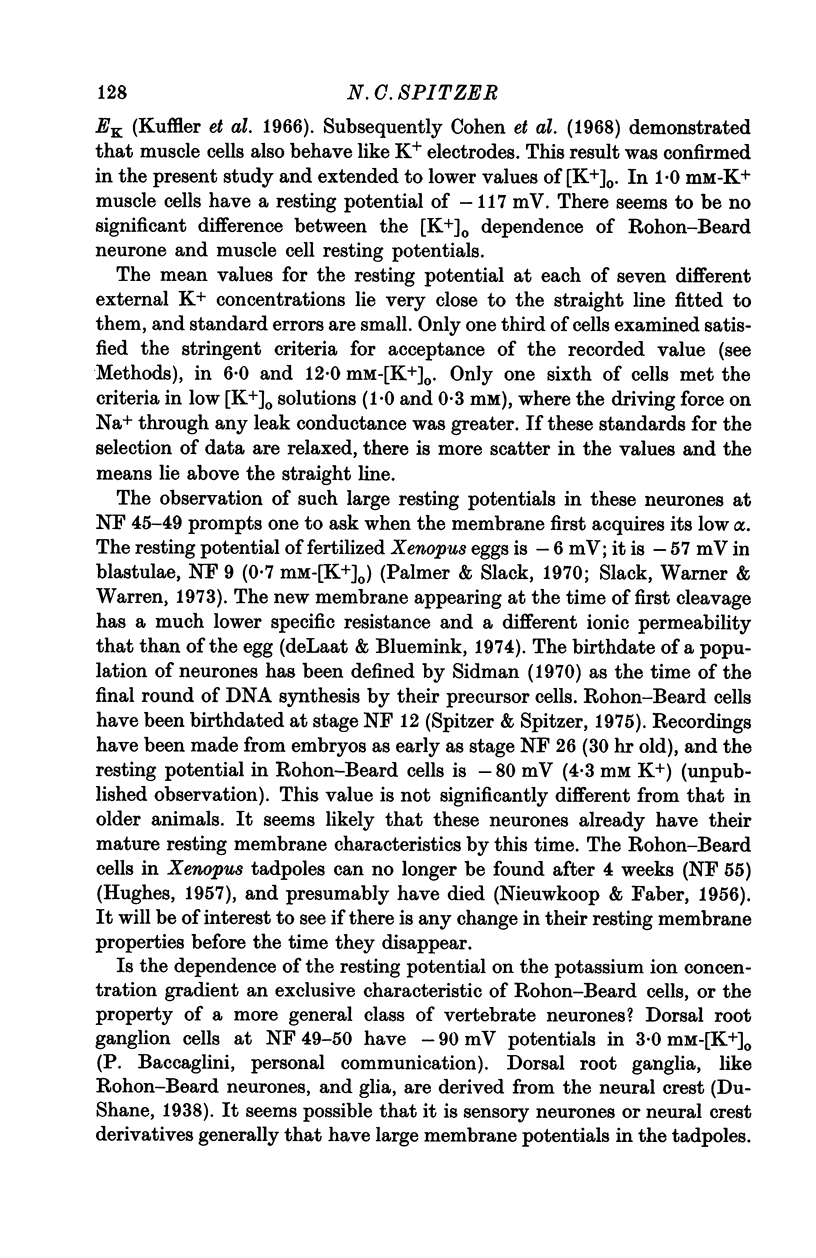
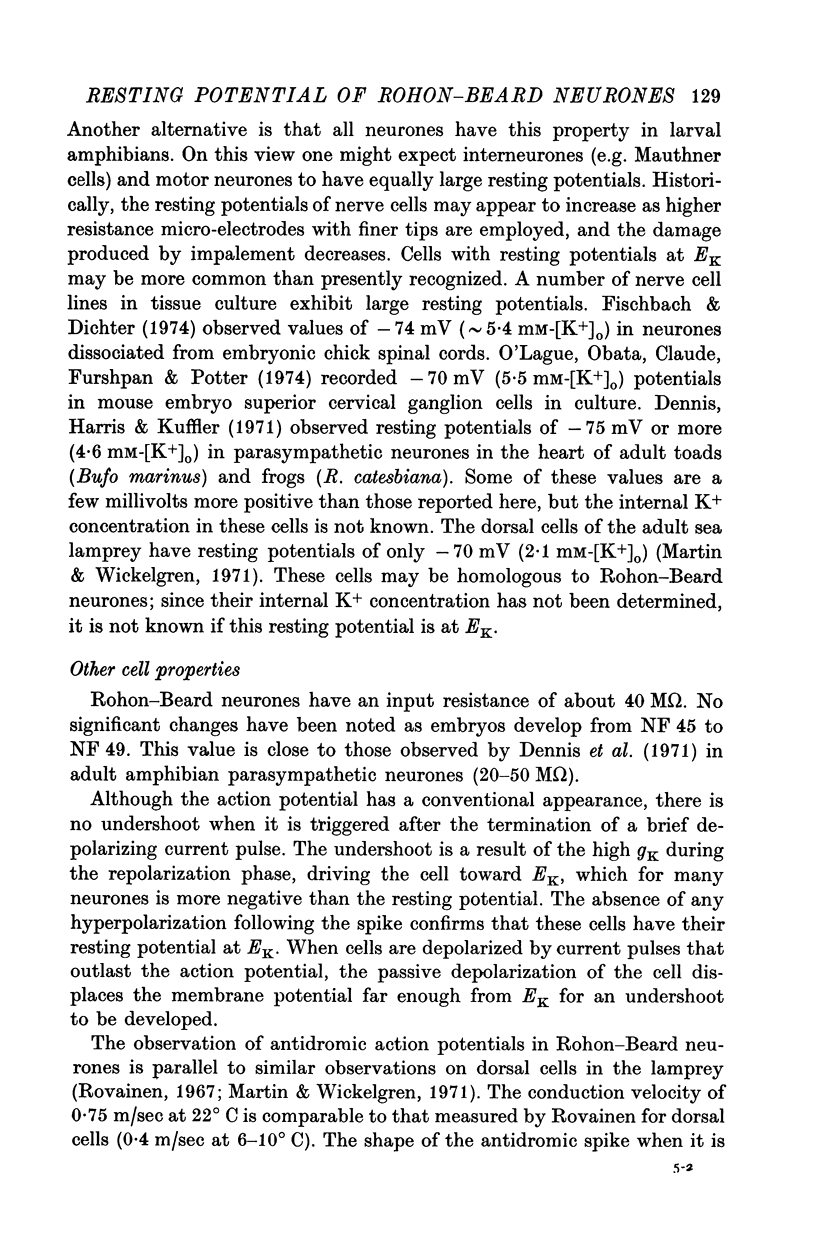
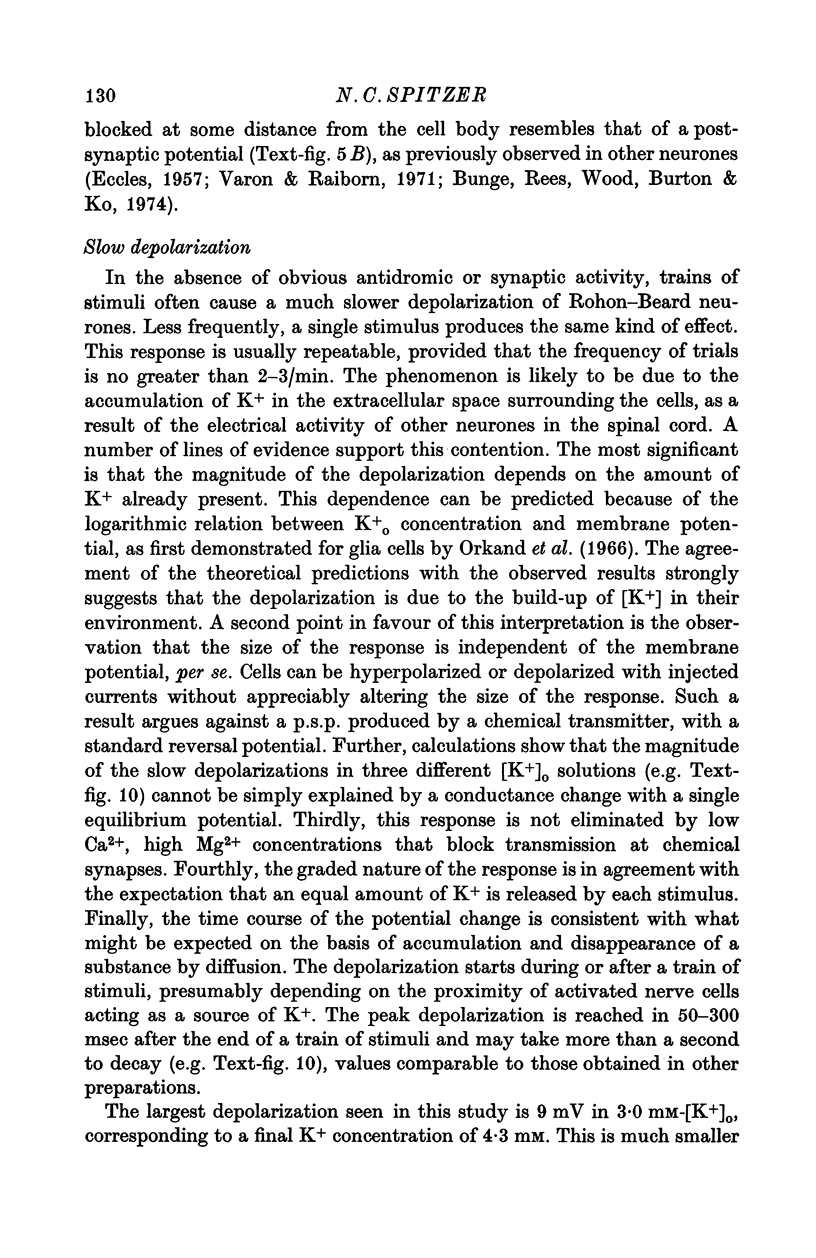
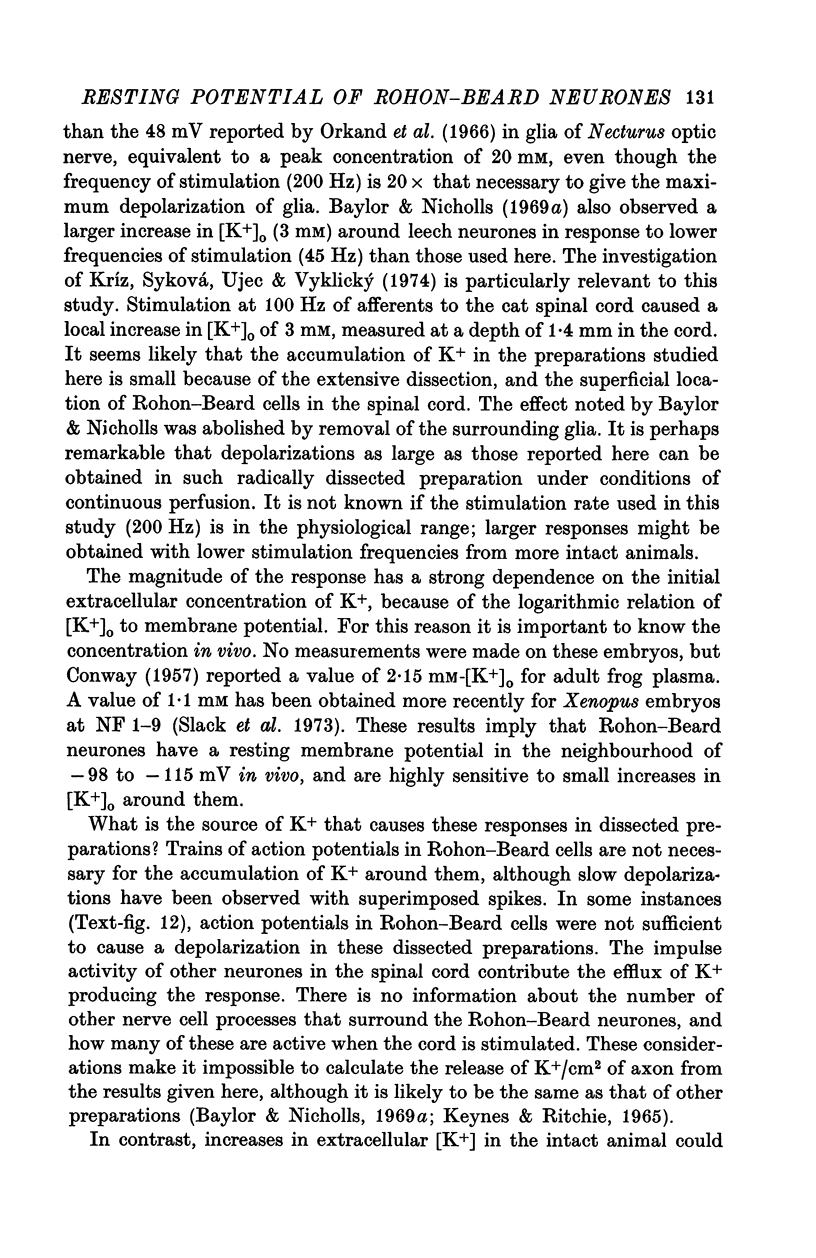
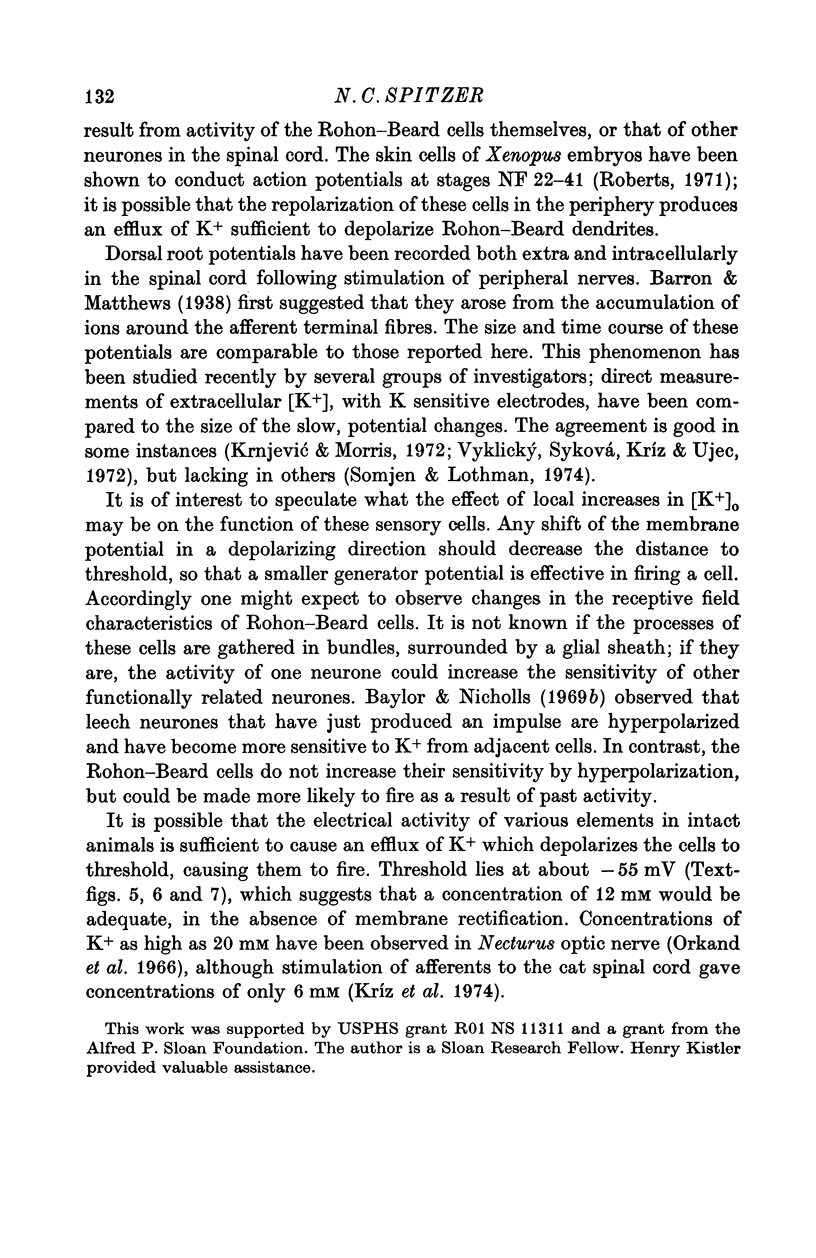
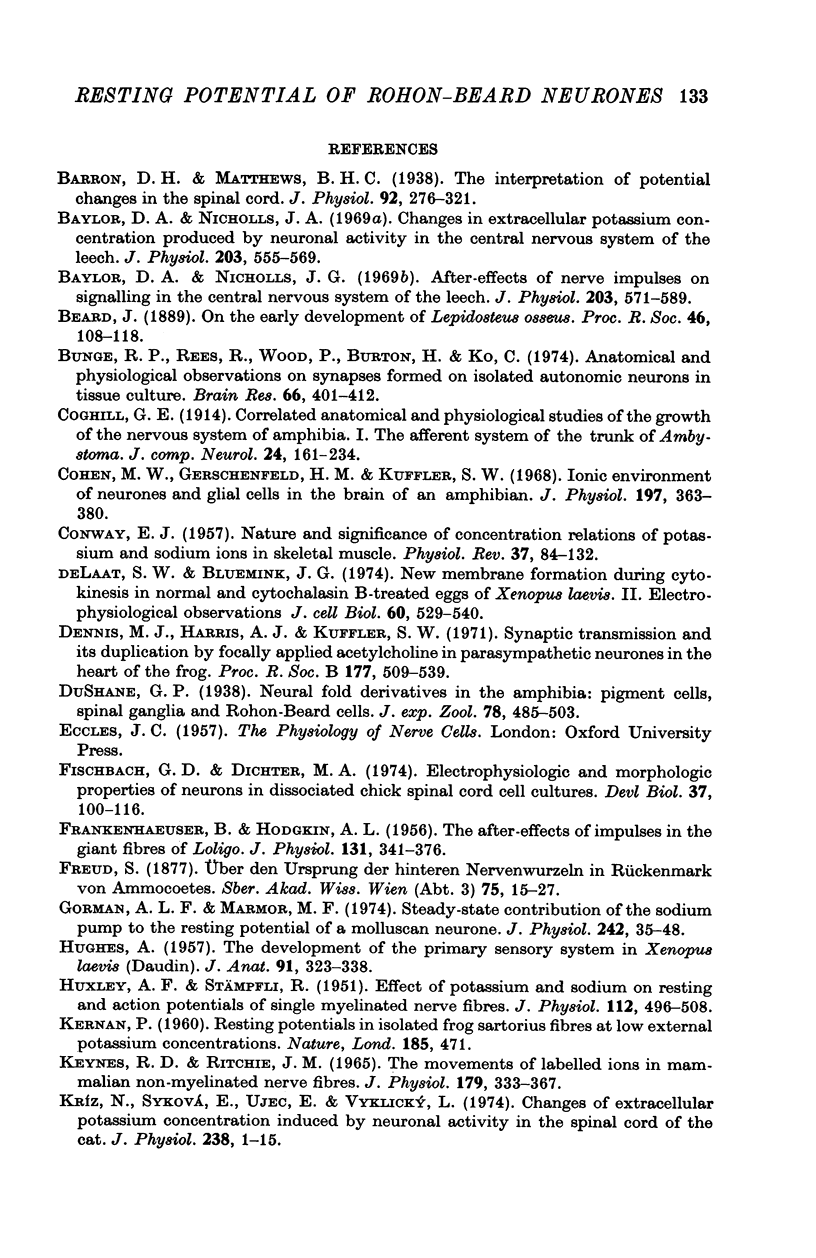
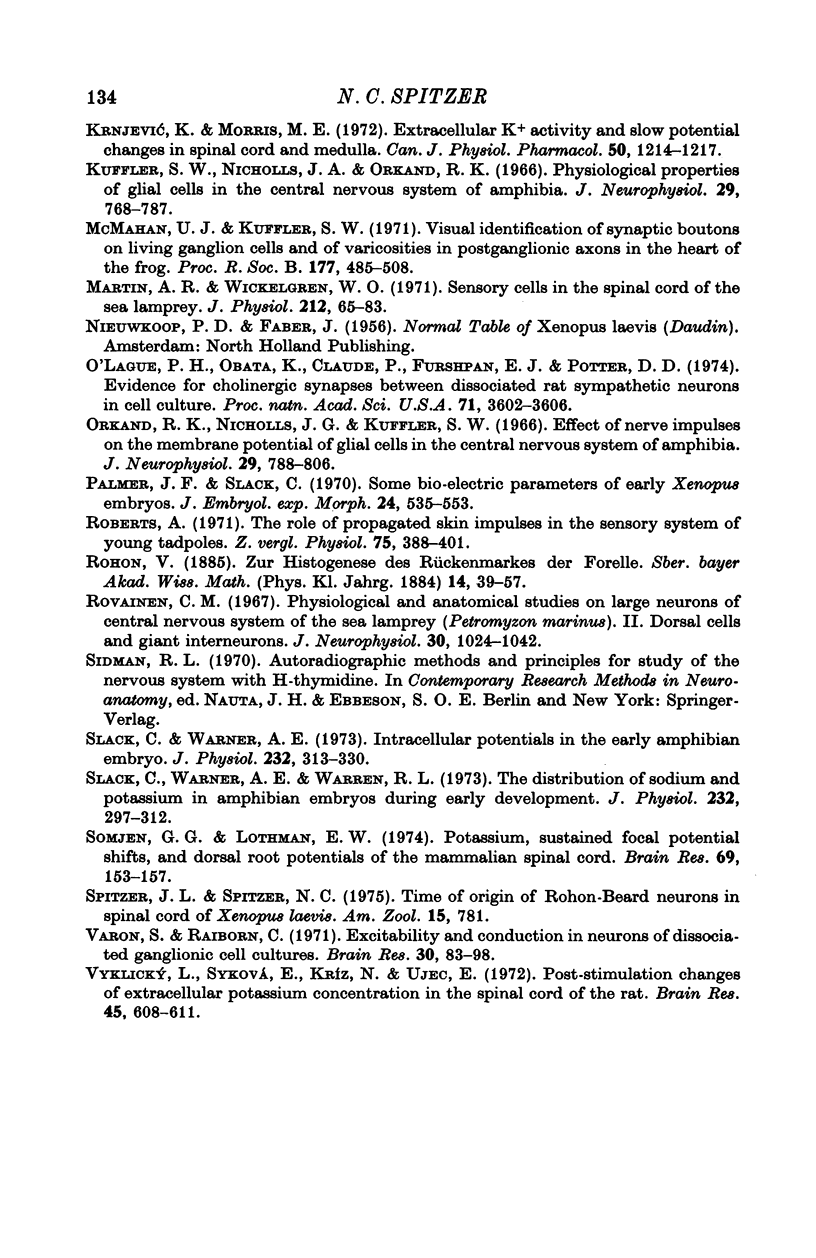
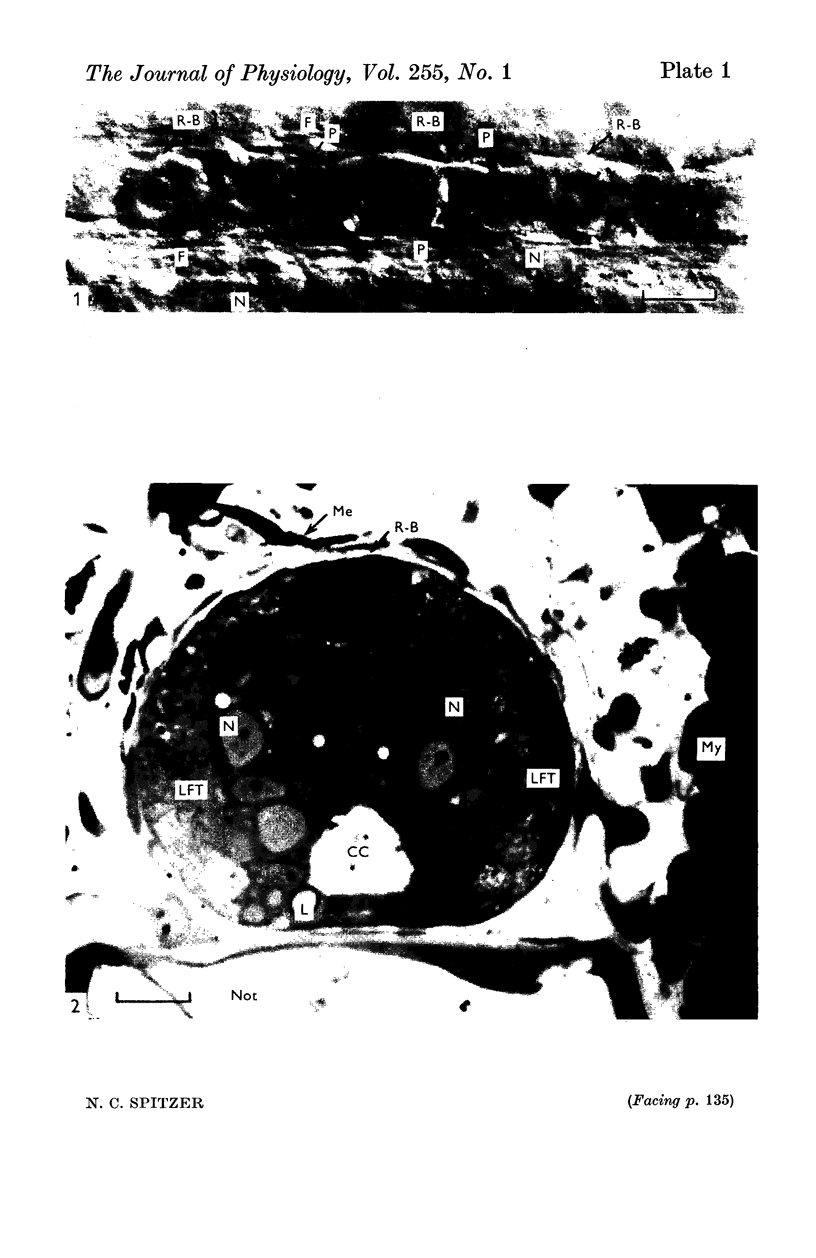
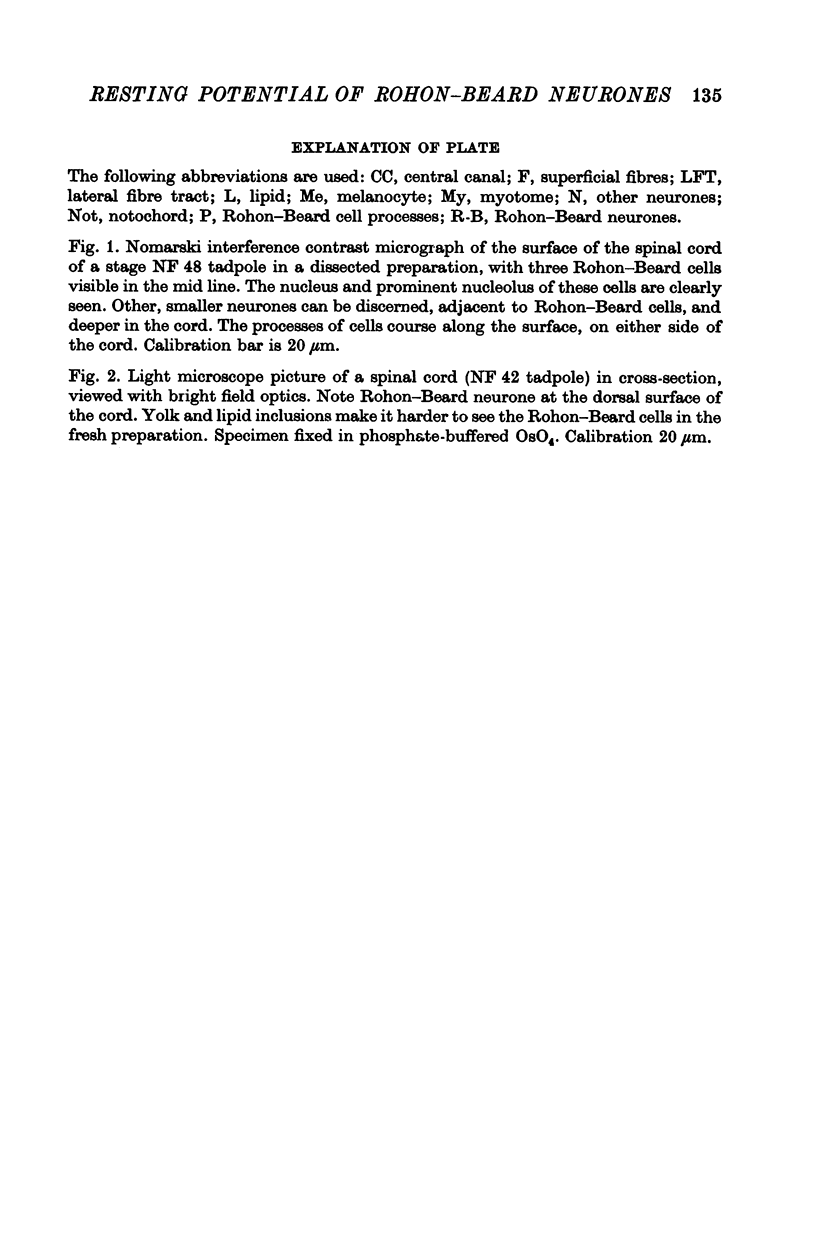
Images in this article
Selected References
These references are in PubMed. This may not be the complete list of references from this article.
- Barron D. H., Matthews B. H. The interpretation of potential changes in the spinal cord. J Physiol. 1938 Apr 14;92(3):276–321. doi: 10.1113/jphysiol.1938.sp003603. [DOI] [PMC free article] [PubMed] [Google Scholar]
- Baylor D. A., Nicholls J. G. After-effects of nerve impulses on signalling in the central nervous system of the leech. J Physiol. 1969 Aug;203(3):571–589. doi: 10.1113/jphysiol.1969.sp008880. [DOI] [PMC free article] [PubMed] [Google Scholar]
- Baylor D. A., Nicholls J. G. Changes in extracellular potassium concentration produced by neuronal activity in the central nervous system of the leech. J Physiol. 1969 Aug;203(3):555–569. doi: 10.1113/jphysiol.1969.sp008879. [DOI] [PMC free article] [PubMed] [Google Scholar]
- CONWAY E. J. Nature and significance of concentration relations of potassium and sodium ions in skeletal muscle. Physiol Rev. 1957 Jan;37(1):84–132. doi: 10.1152/physrev.1957.37.1.84. [DOI] [PubMed] [Google Scholar]
- Cohen M. W., Gerschenfeld H. M., Kuffler S. W. Ionic environment of neurones and glial cells in the brain of an amphibian. J Physiol. 1968 Jul;197(2):363–380. doi: 10.1113/jphysiol.1968.sp008564. [DOI] [PMC free article] [PubMed] [Google Scholar]
- Dennis M. J., Harris A. J., Kuffler S. W. Synaptic transmission and its duplication by focally applied acetylcholine in parasympathetic neurons in the heart of the frog. Proc R Soc Lond B Biol Sci. 1971 Apr 27;177(1049):509–539. doi: 10.1098/rspb.1971.0045. [DOI] [PubMed] [Google Scholar]
- FRANKENHAEUSER B., HODGKIN A. L. The after-effects of impulses in the giant nerve fibres of Loligo. J Physiol. 1956 Feb 28;131(2):341–376. doi: 10.1113/jphysiol.1956.sp005467. [DOI] [PMC free article] [PubMed] [Google Scholar]
- Fischbach G. D., Dichter M. A. Electrophysiologic and morphologic properties of neurons in dissociated chick spinal cord cell cultures. Dev Biol. 1974 Mar;37(1):100–116. doi: 10.1016/0012-1606(74)90172-9. [DOI] [PubMed] [Google Scholar]
- Gorman A. L., Marmor M. F. Steady-state contribution of the sodium pump to the resting potential of a molluscan neurone. J Physiol. 1974 Oct;242(1):35–48. doi: 10.1113/jphysiol.1974.sp010692. [DOI] [PMC free article] [PubMed] [Google Scholar]
- HUGHES A. The development of the primary sensory system in Xenopus laevis (Daudin). J Anat. 1957 Jul;91(3):323–338. [PMC free article] [PubMed] [Google Scholar]
- HUXLEY A. F., STAMPFLI R. Effect of potassium and sodium on resting and action potentials of single myelinated nerve fibers. J Physiol. 1951 Feb;112(3-4):496–508. doi: 10.1113/jphysiol.1951.sp004546. [DOI] [PMC free article] [PubMed] [Google Scholar]
- Keynes R. D., Ritchie J. M. The movements of labelled ions in mammalian non-myelinated nerve fibres. J Physiol. 1965 Jul;179(2):333–367. doi: 10.1113/jphysiol.1965.sp007666. [DOI] [PMC free article] [PubMed] [Google Scholar]
- Krnjević K., Morris M. E. Extracellular K + activity and slow potential changes in spinal cord and medulla. Can J Physiol Pharmacol. 1972 Dec;50(12):1214–1217. doi: 10.1139/y72-177. [DOI] [PubMed] [Google Scholar]
- Kríz N., Syková E., Ujec E., Vyklický L. Changes of extracellular potassium concentration induced by neuronal activity in the sinal cord of the cat. J Physiol. 1974 Apr;238(1):1–15. doi: 10.1113/jphysiol.1974.sp010507. [DOI] [PMC free article] [PubMed] [Google Scholar]
- Kuffler S. W., Nicholls J. G., Orkand R. K. Physiological properties of glial cells in the central nervous system of amphibia. J Neurophysiol. 1966 Jul;29(4):768–787. doi: 10.1152/jn.1966.29.4.768. [DOI] [PubMed] [Google Scholar]
- Martin A. R., Wickelgren W. O. Sensory cells in the spinal cord of the sea lamprey. J Physiol. 1971 Jan;212(1):65–83. doi: 10.1113/jphysiol.1971.sp009310. [DOI] [PMC free article] [PubMed] [Google Scholar]
- McMahan U. J., Kuffler S. W. Visual identification of synaptic boutons on living ganglion cells and of varicosities in postganglionic axons in the heart of the frog. Proc R Soc Lond B Biol Sci. 1971 Apr 27;177(1049):485–508. doi: 10.1098/rspb.1971.0044. [DOI] [PubMed] [Google Scholar]
- O'Lague P. H., Obata K., Claude P., Furshpan E. J., Potter D. D. Evidence for cholinergic synapses between dissociated rat sympathetic neurons in cell culture. Proc Natl Acad Sci U S A. 1974 Sep;71(9):3602–3606. doi: 10.1073/pnas.71.9.3602. [DOI] [PMC free article] [PubMed] [Google Scholar]
- Orkand R. K., Nicholls J. G., Kuffler S. W. Effect of nerve impulses on the membrane potential of glial cells in the central nervous system of amphibia. J Neurophysiol. 1966 Jul;29(4):788–806. doi: 10.1152/jn.1966.29.4.788. [DOI] [PubMed] [Google Scholar]
- Palmer J. F., Slack C. Some bio-electric parameters of early Xenopus embryos. J Embryol Exp Morphol. 1970 Nov;24(3):535–553. [PubMed] [Google Scholar]
- Rovainen C. M. Physiological and anatomical studies on large neurons of central nervous system of the sea lamprey (Petromyzon marinus). II. Dorsal cells and giant interneurons. J Neurophysiol. 1967 Sep;30(5):1024–1042. doi: 10.1152/jn.1967.30.5.1024. [DOI] [PubMed] [Google Scholar]
- Slack C., Warner A. E. Intracellular and intercellular potentials in the early amphibian embryo. J Physiol. 1973 Jul;232(2):313–330. doi: 10.1113/jphysiol.1973.sp010272. [DOI] [PMC free article] [PubMed] [Google Scholar]
- Slack C., Warner A. E., Warren R. L. The distribution of sodium and potassium in amphibian embryos during early development. J Physiol. 1973 Jul;232(2):297–312. doi: 10.1113/jphysiol.1973.sp010271. [DOI] [PMC free article] [PubMed] [Google Scholar]
- Somjen G. G., Lothman E. W. Potassium, sustained focal potential shifts, and dorsal root potentials of the mammalian spinal cord. Brain Res. 1974 Mar 29;69(1):153–157. doi: 10.1016/0006-8993(74)90382-5. [DOI] [PubMed] [Google Scholar]
- Varon S., Raiborn C. Excitability and conduction in neurons of dissociated ganglionic cell cultures. Brain Res. 1971 Jul 9;30(1):83–98. doi: 10.1016/0006-8993(71)90007-2. [DOI] [PubMed] [Google Scholar]
- Vyklicky L., Sykova E., Kriz N., Ujec E. Post-stimulation changes of extracellular potassium concentration in the spinal cord of the rat. Brain Res. 1972 Oct 27;45(2):608–611. doi: 10.1016/0006-8993(72)90492-1. [DOI] [PubMed] [Google Scholar]
- de Laat W. S., Bluemink J. G. New membrane formation during cytokinesis in normal and cytochalasin B-treated eggs of Xenopus laevis. II. Electrophysiological observations. J Cell Biol. 1974 Mar;60(3):529–540. doi: 10.1083/jcb.60.3.529. [DOI] [PMC free article] [PubMed] [Google Scholar]