Abstract
1. The temperature-dependence of the uptake of 24Na and 42K into dog red cells between 38 and 4 degrees C has been investigated. The effects on the cation fluxes of partial dehydration of the cells in hyperosmolar sucrose (50-125 mM) have also been studied. 2. A Hamilton gas-tight syringe was used to pipette accurately reproducible volumes of packed cells which contained in addition to 24Na or 42K either [131I]albumin or [51Cr]EDTA as extracellular markers. 3. At 38 degrees C Na flux (m-equiv/l. isosmolar cell volume. hr) increased from 2-8 +/- 0-1 (n = 8) in cells of normal volume to 226 +/- 8 (n = 8) when the cells were shrunken by 27-4 +/- 0-6% (n = 8) in media containing sucrose (100 mM). K influx remained relatively constant under these conditions. 4. The exchange of 24Na in shrunken cells followed a single exponential time course but about 9% of the intracellular Na apparently did not exchange with 24Na in the bathing medium. 5. The steady-state influx of Na in cells of normal volume was maximal at about 22 degrees C. The temperature dependence of the Na fluxes in shrunken cells was described by an Arrhenius relationship with a change in slope at about 22 degrees C. 6. The K influx in cells of normal volume decreased as the temperature was lowered from 38 degrees C, to about 12 degrees C, at which temperature the flux was at a well defined minimum. Above 12 degrees C, cell shrinkage had hardly any effect on K influx, but below 12 degrees C the influx in shrunken cells was significantly less than in cells of normal volume. 7. The selective increase in Na flux induced by cell shrinkage results from a Na:Na exchange process which cannot be explained in terms of Ussing's (1947) model of carrier-mediated exchange diffusion. 8. The lack of coupling between the effects of temperature and cell volume on the fluxes of Na and K indicates that localized structural changes of lipid-protein complexes specific for Na or K are responsible for the cation transport characteristics of dog red cells, and that phase transitions in the lipids of the cell membrane are unlikely to account for the temperature dependence of the fluxes.
Full text
PDF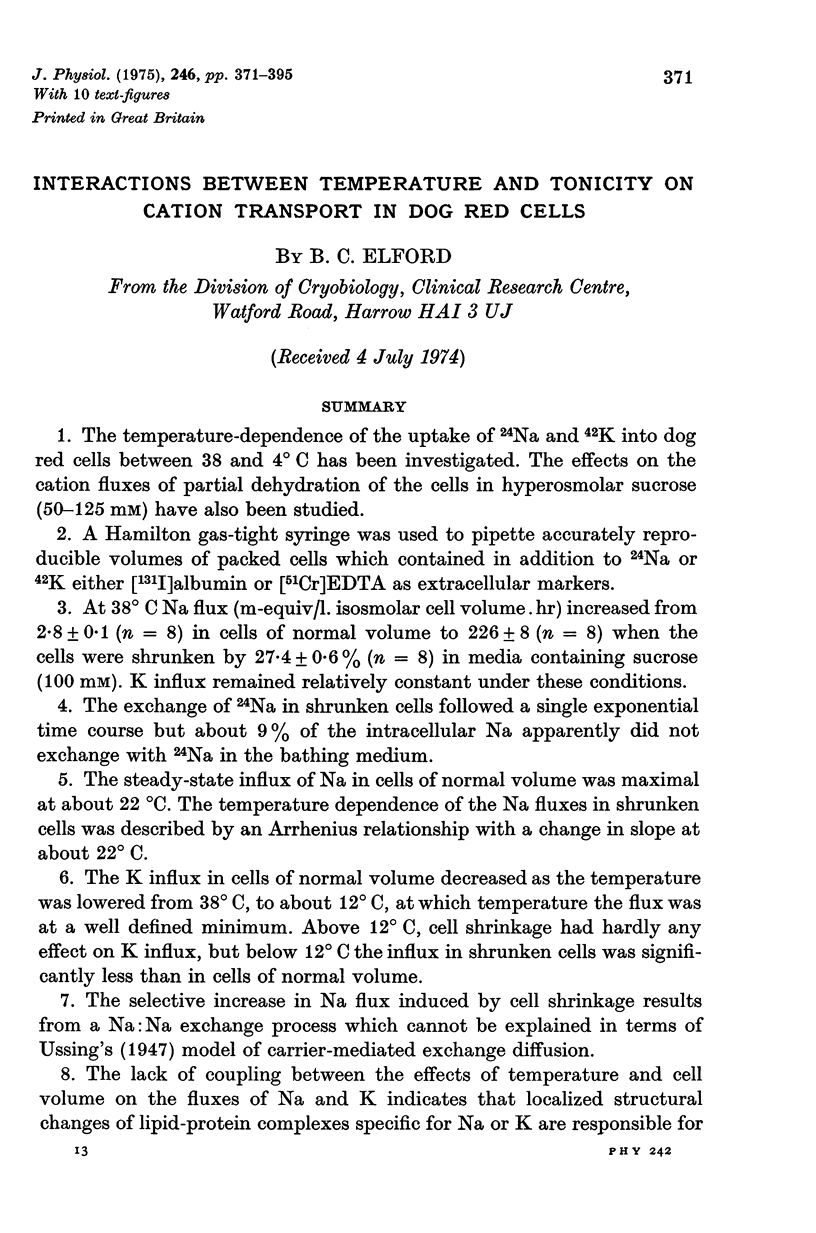
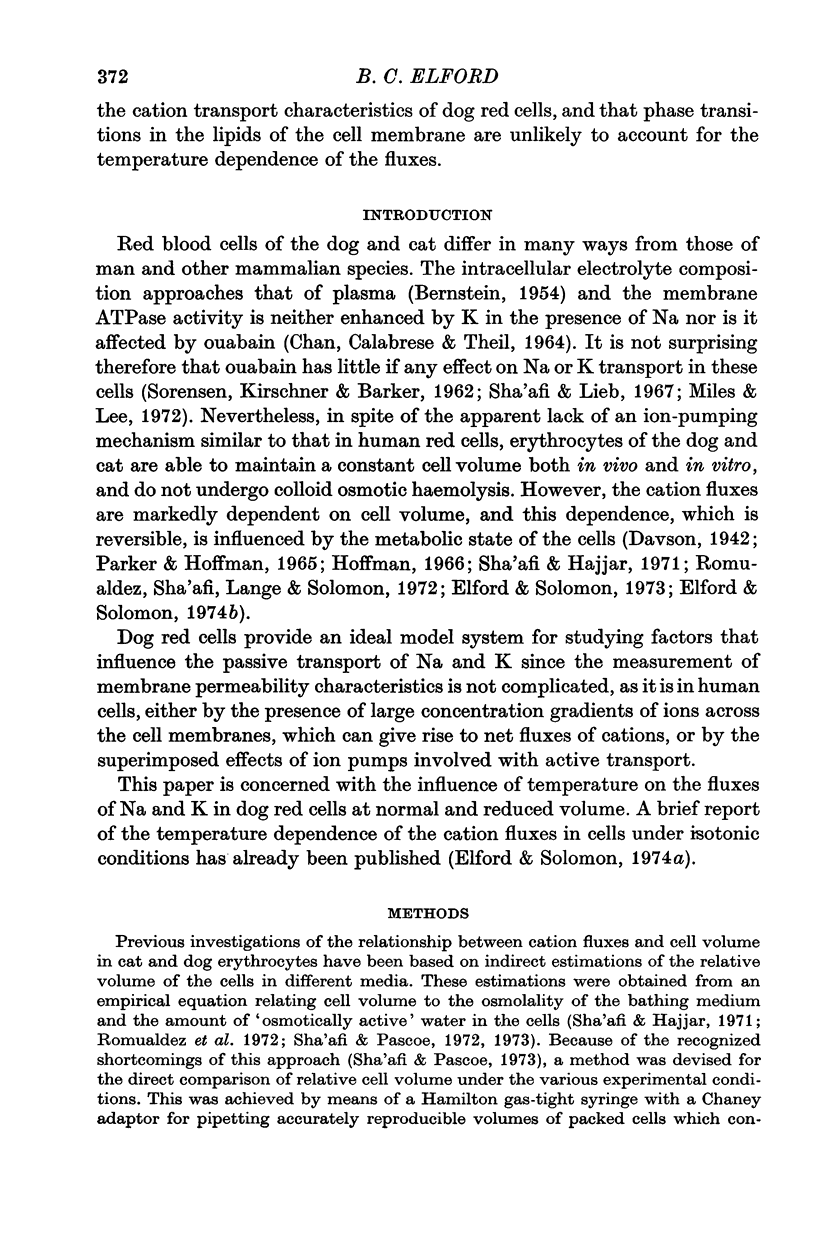
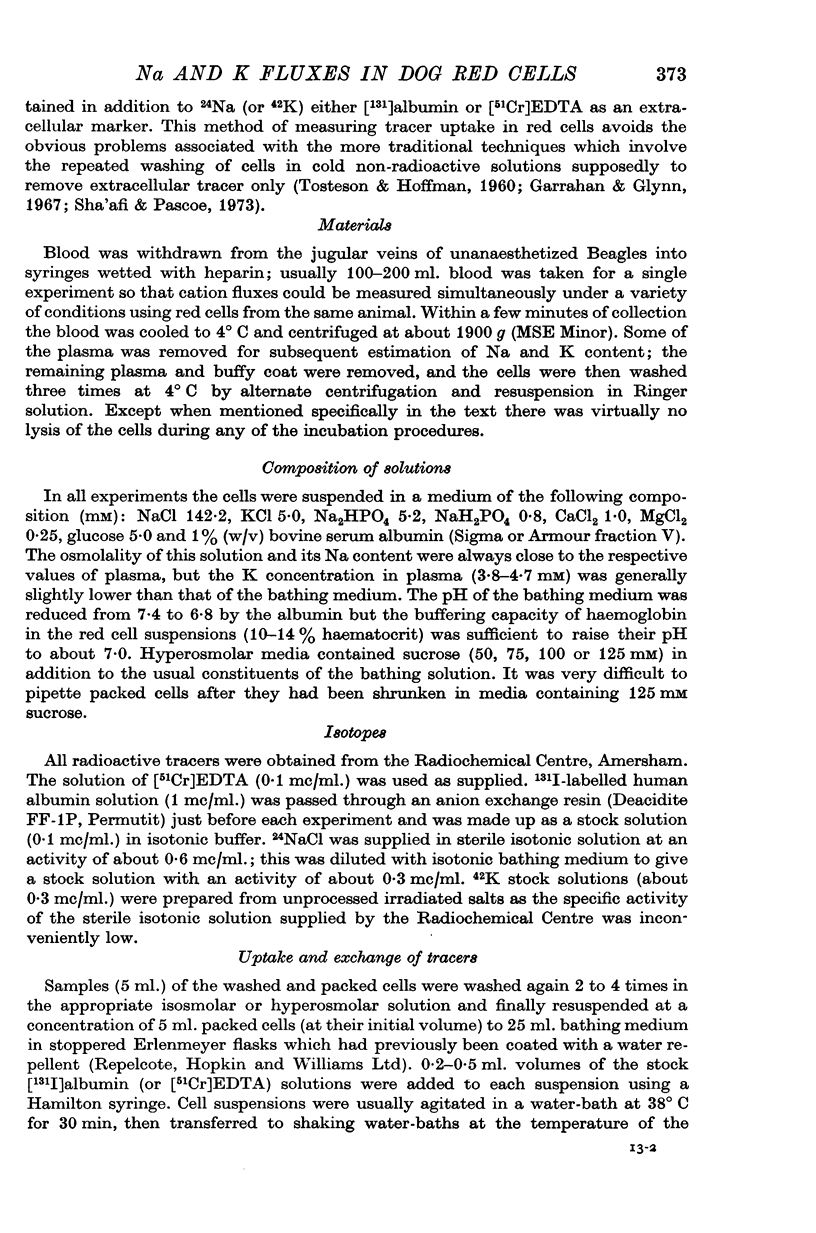
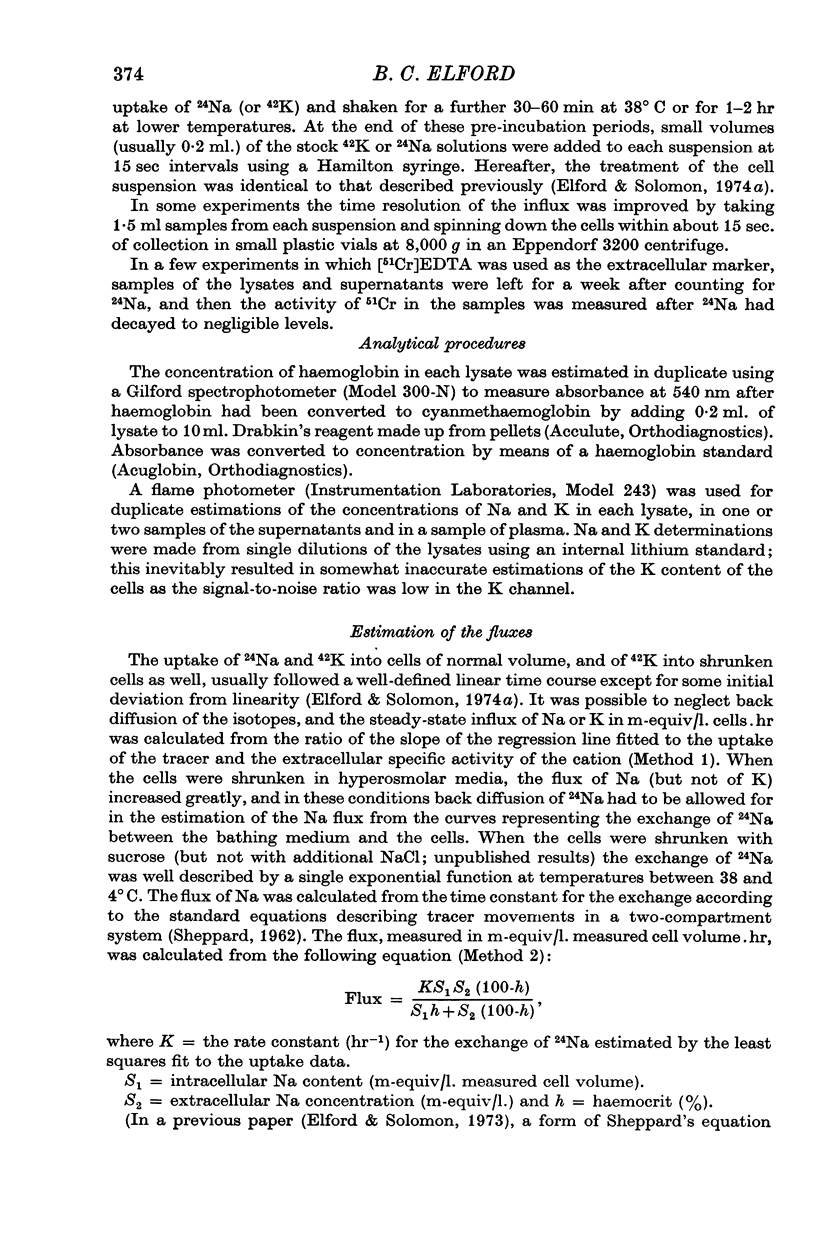
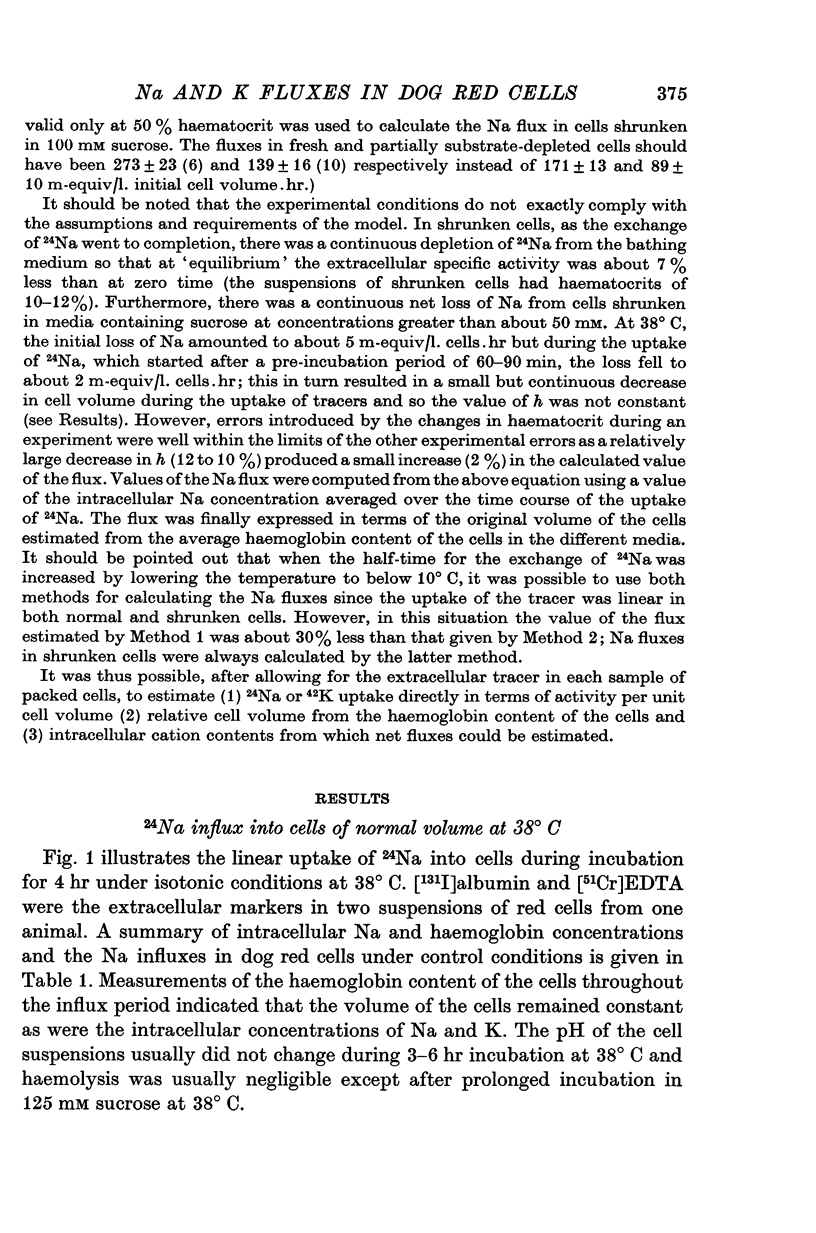
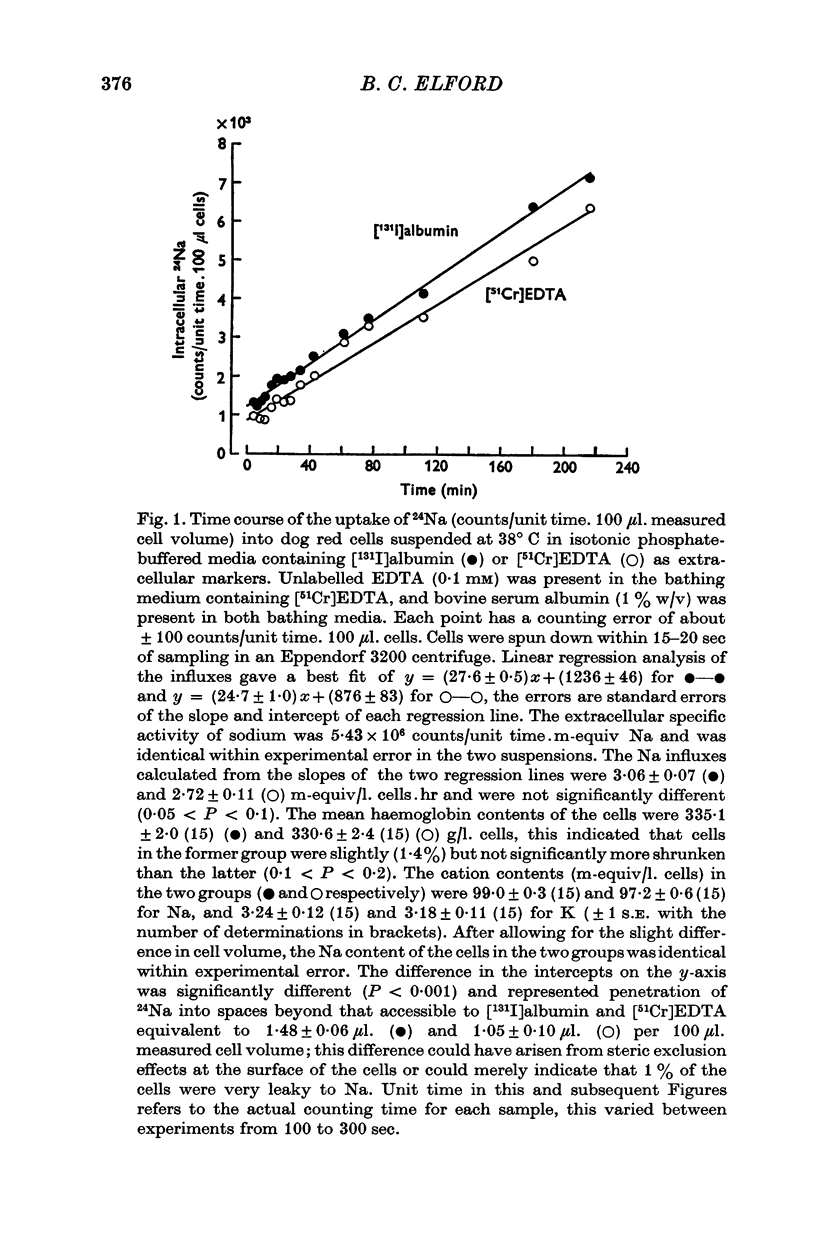
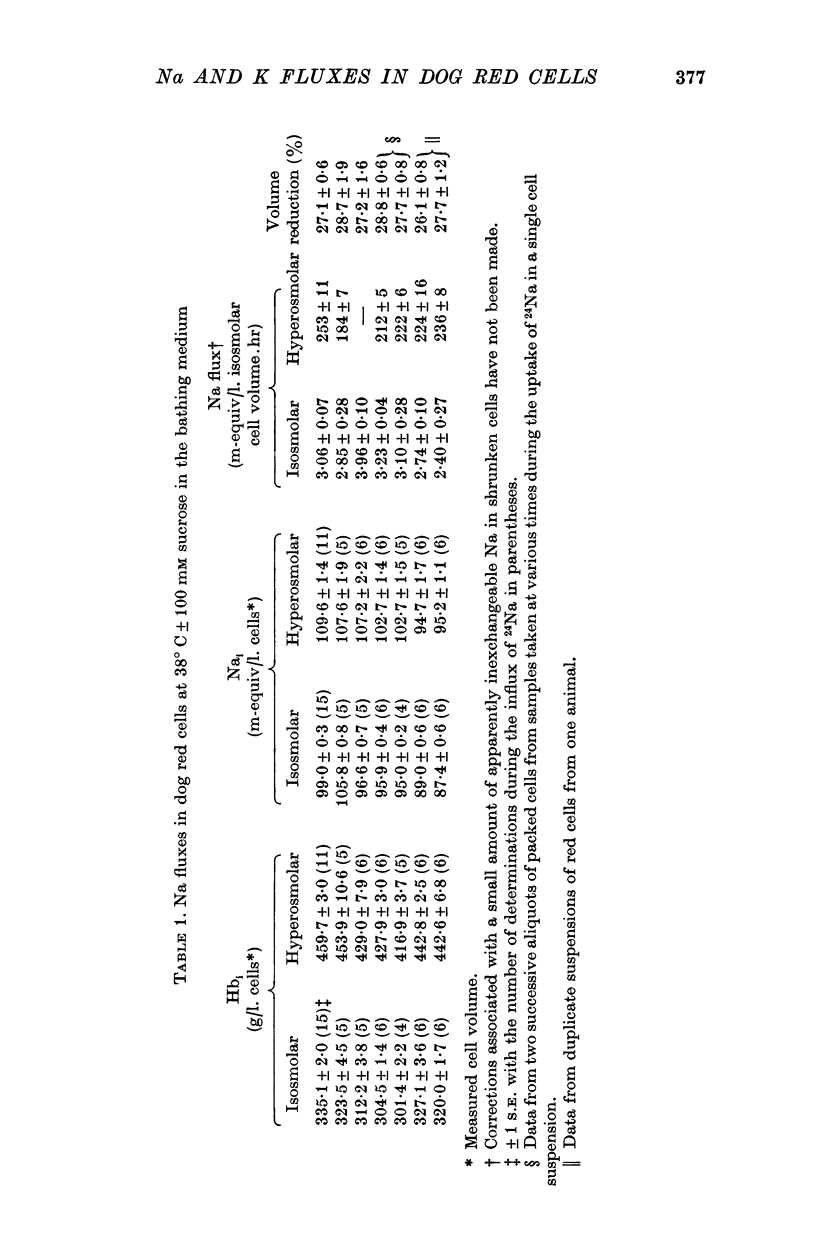
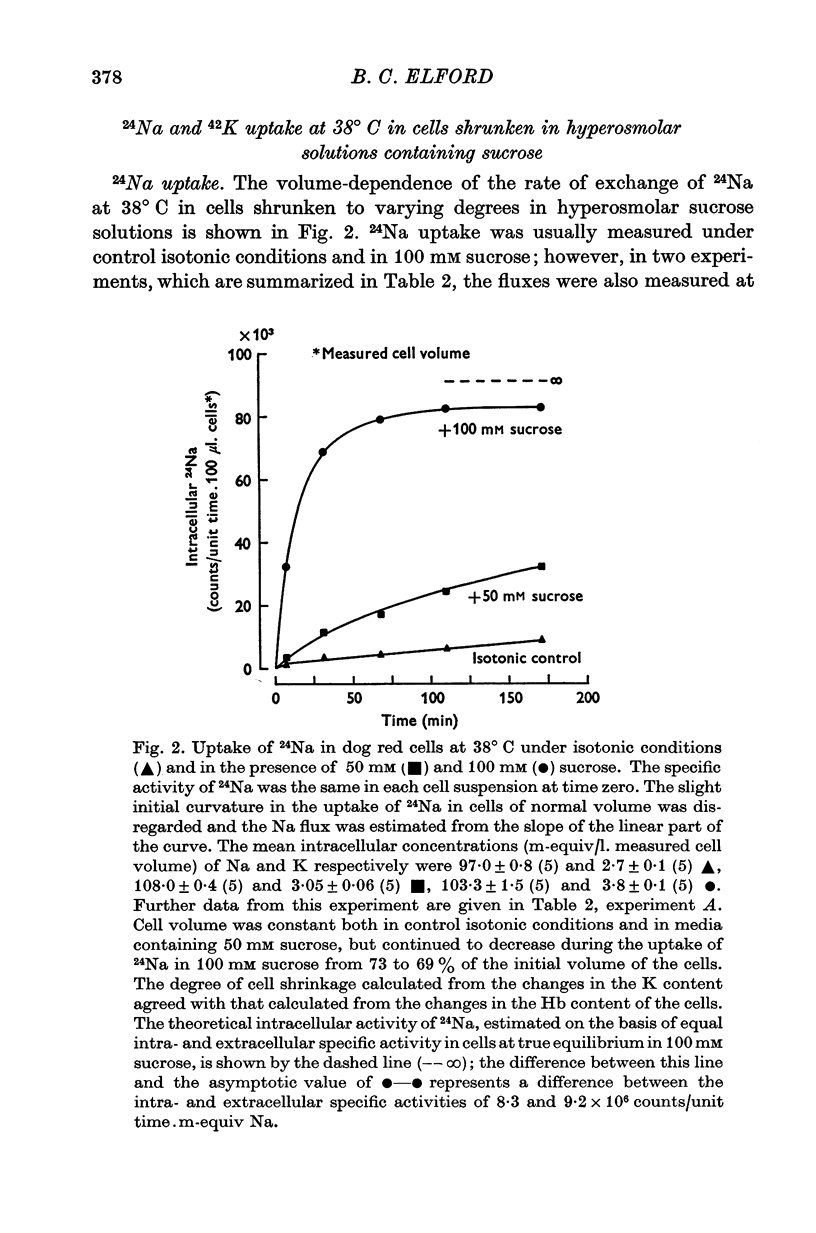
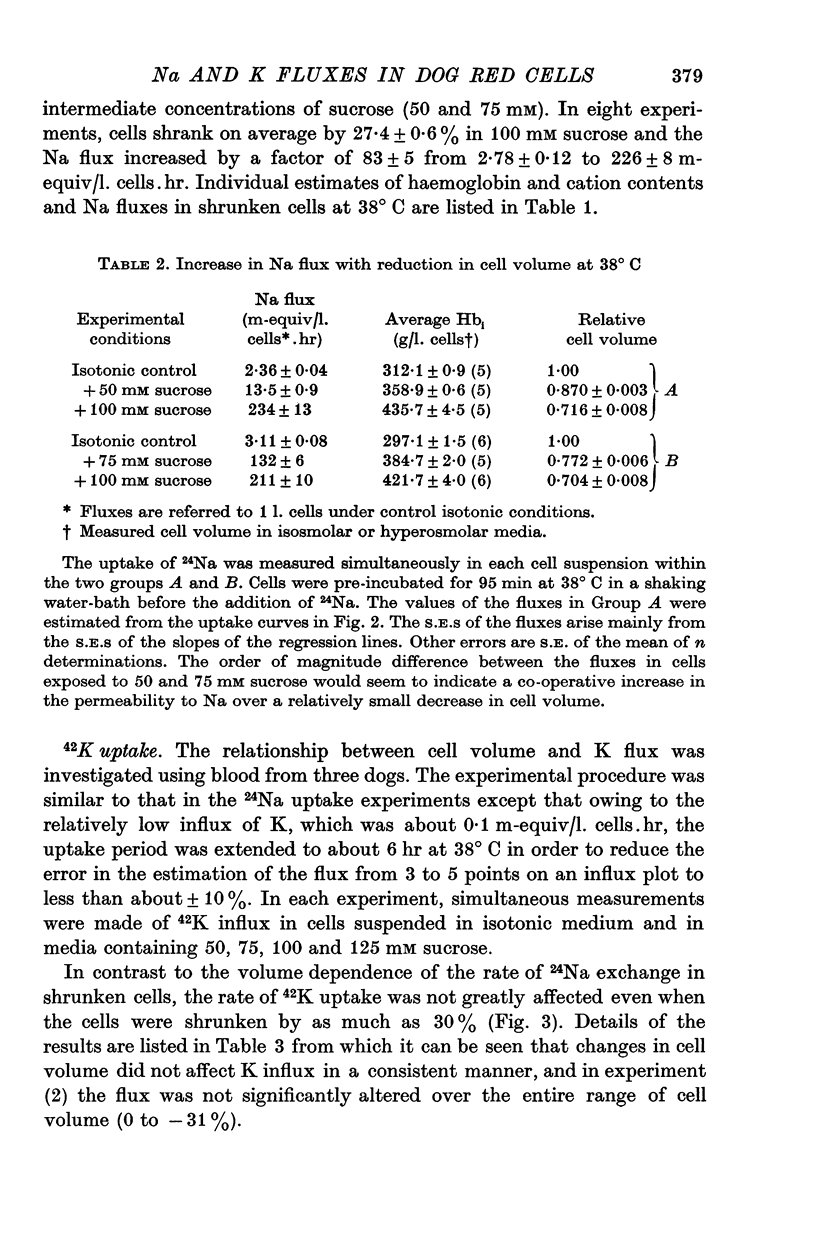
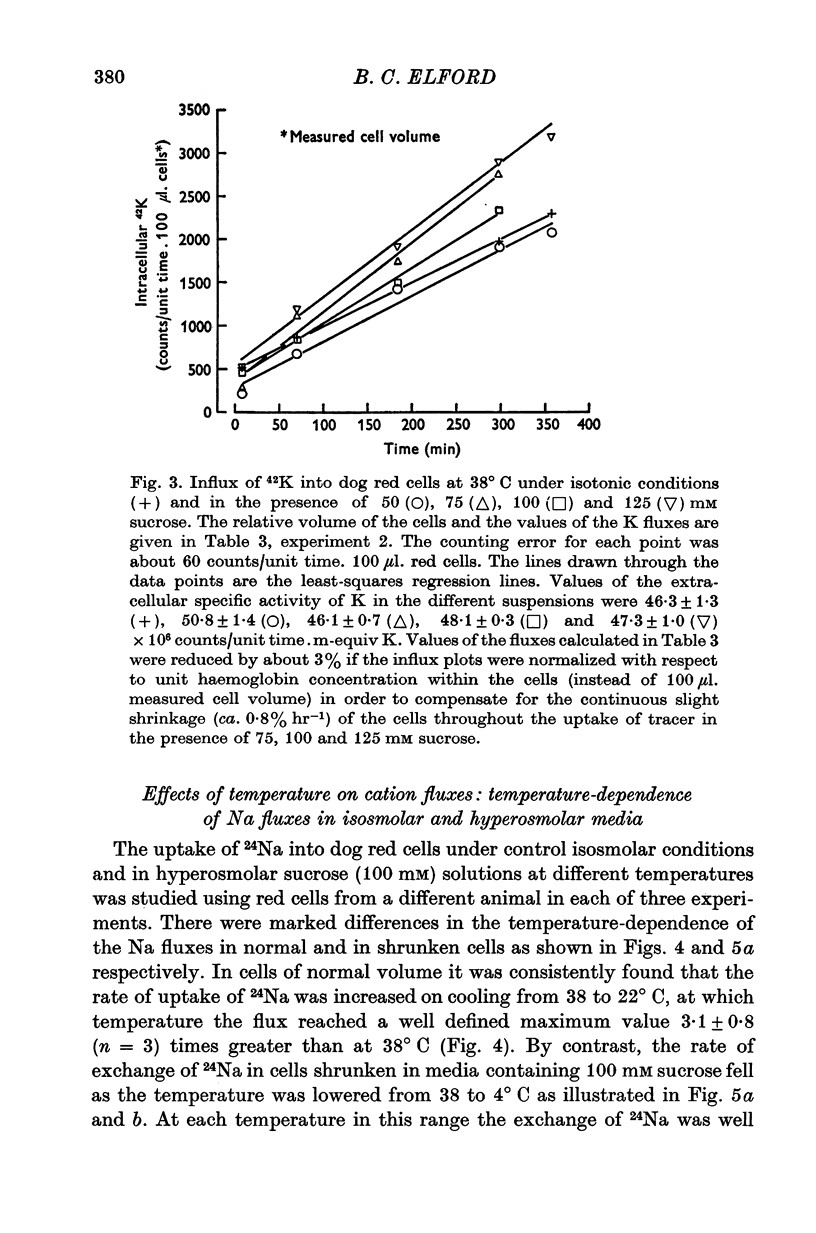
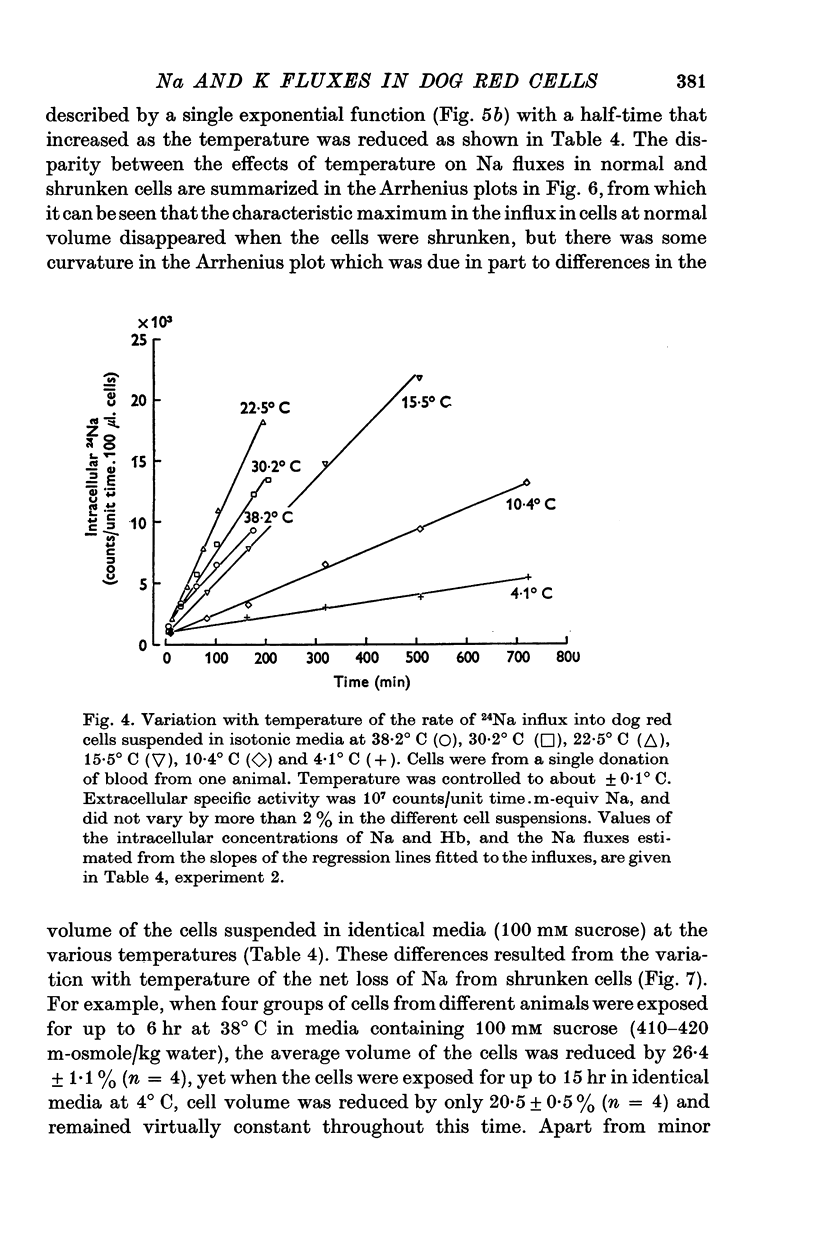
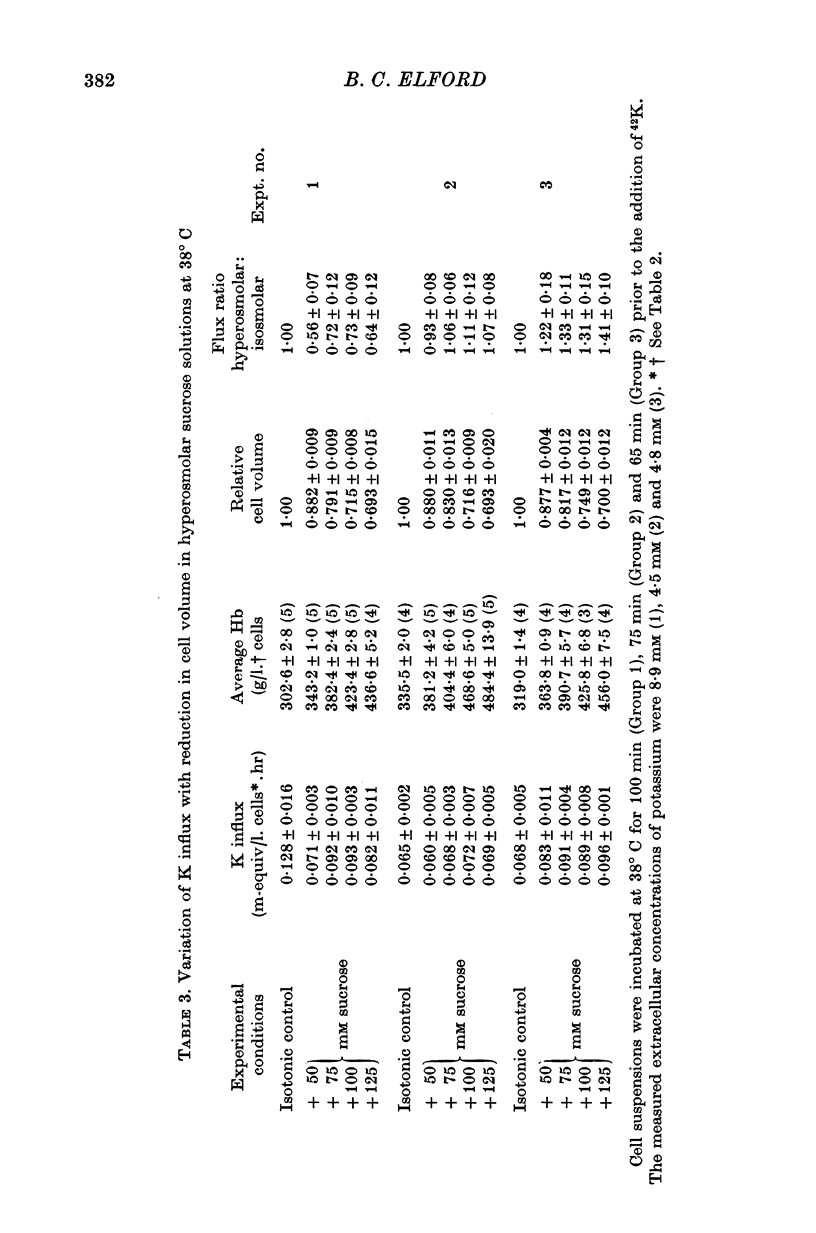
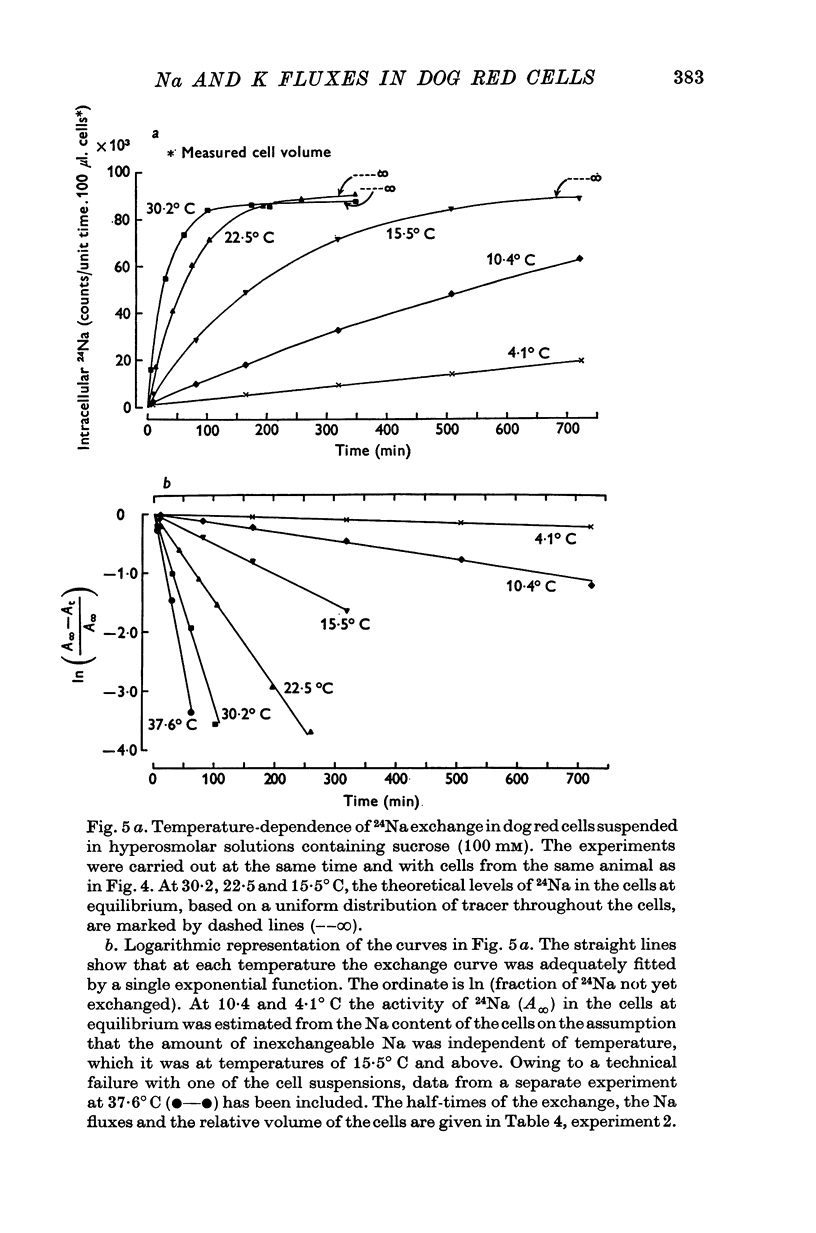
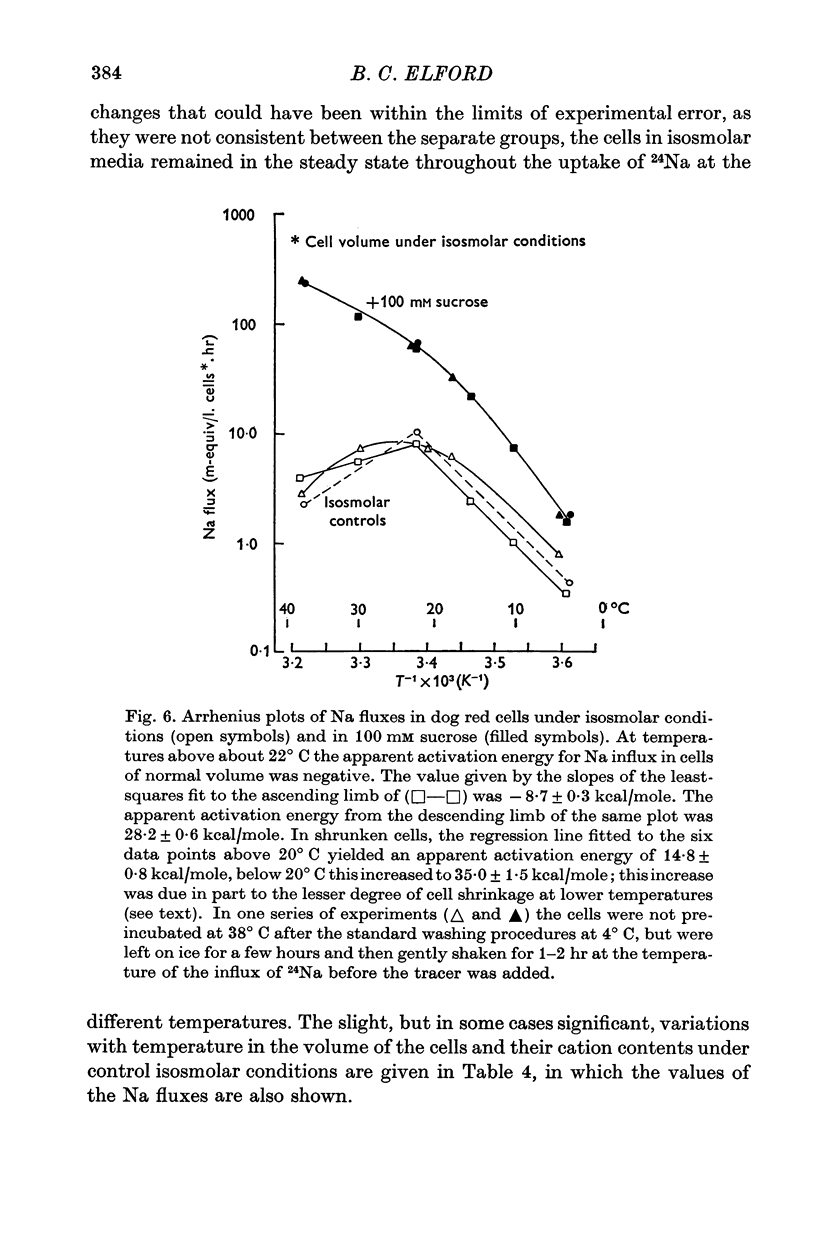
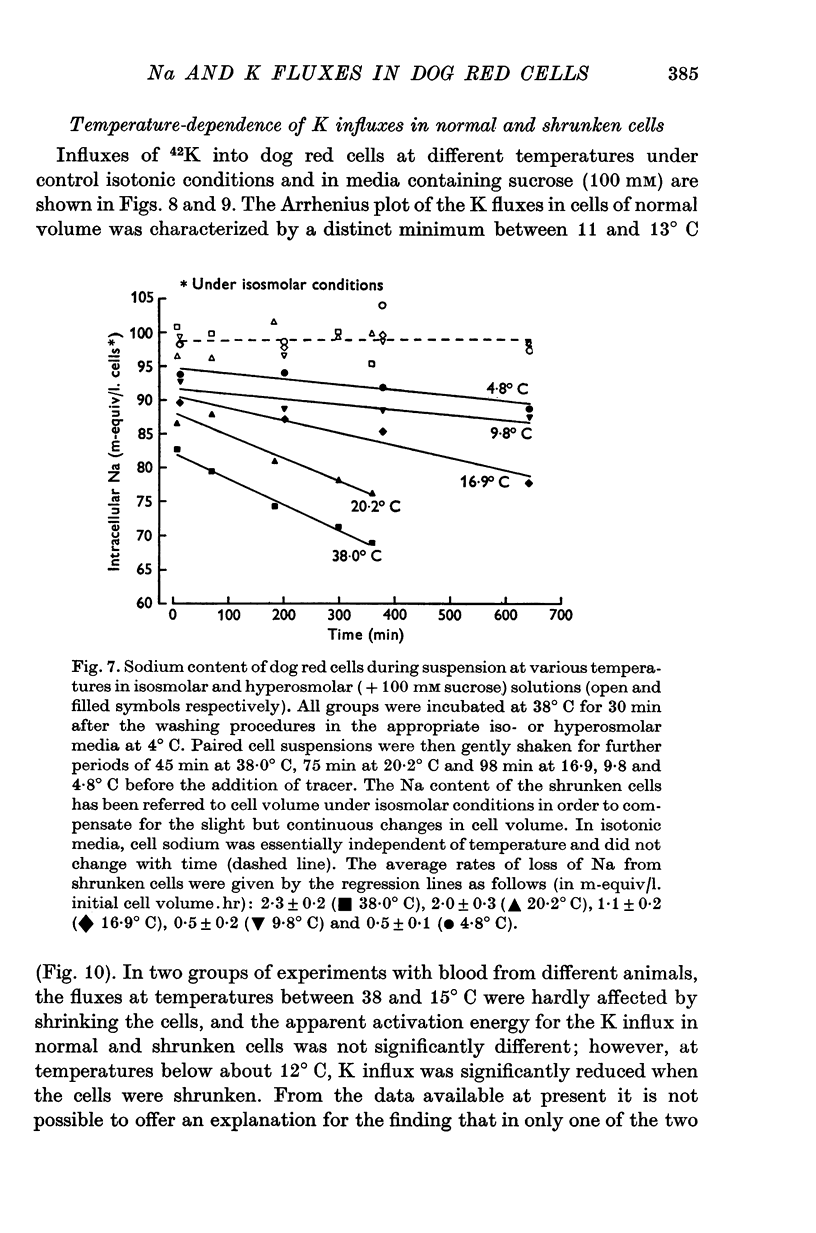
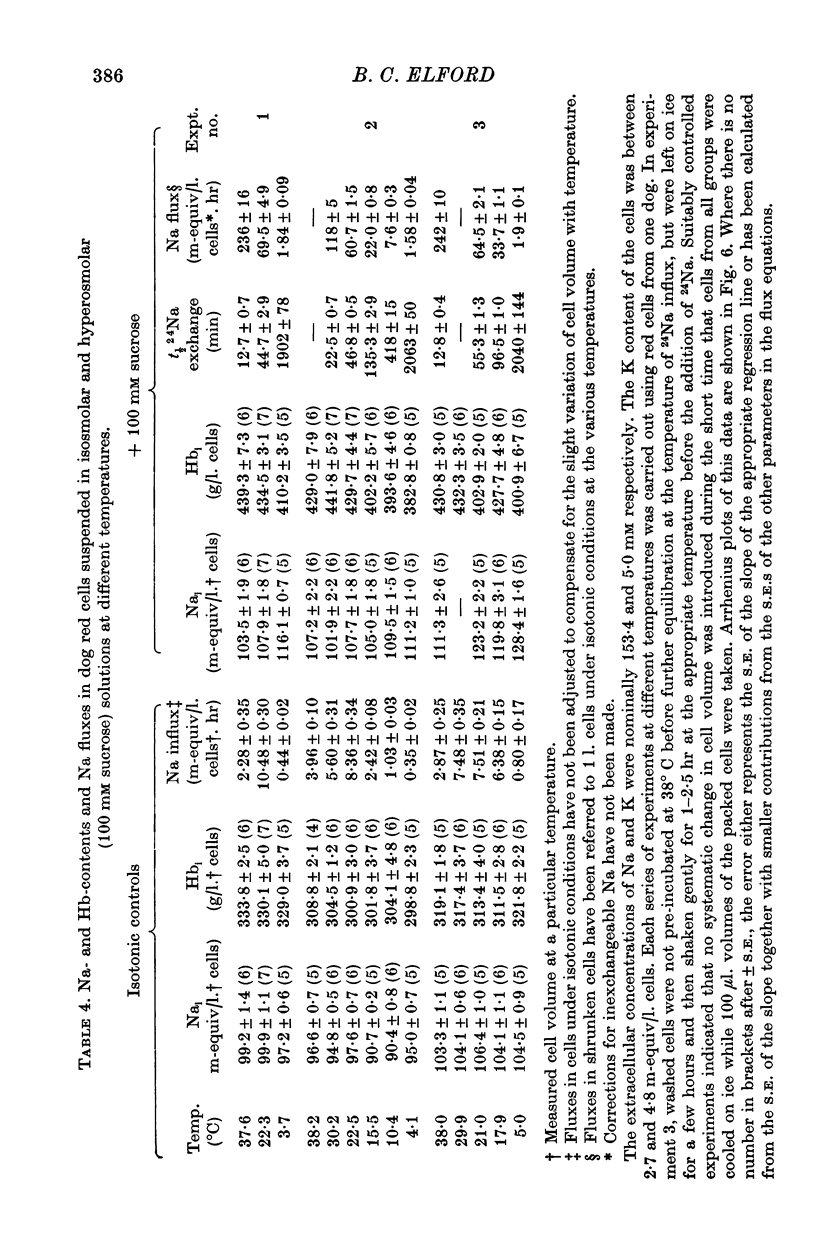
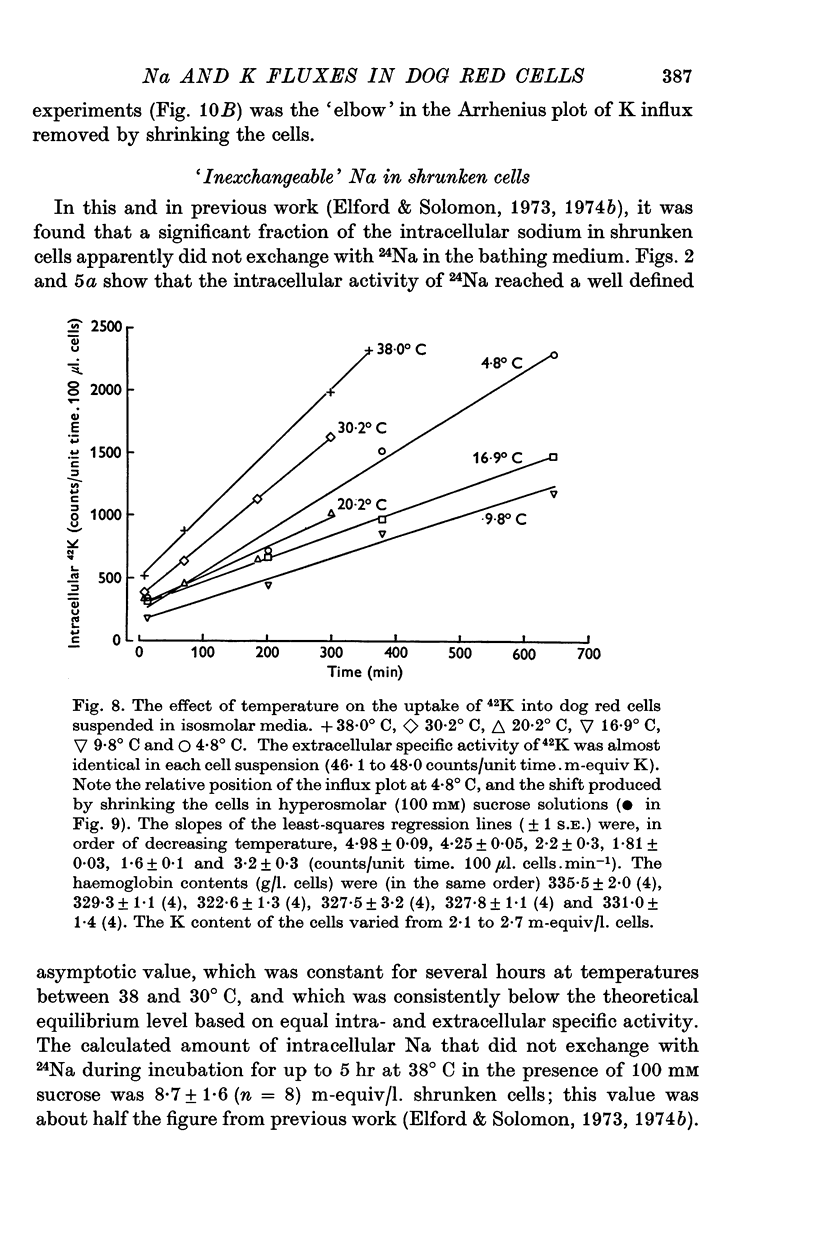
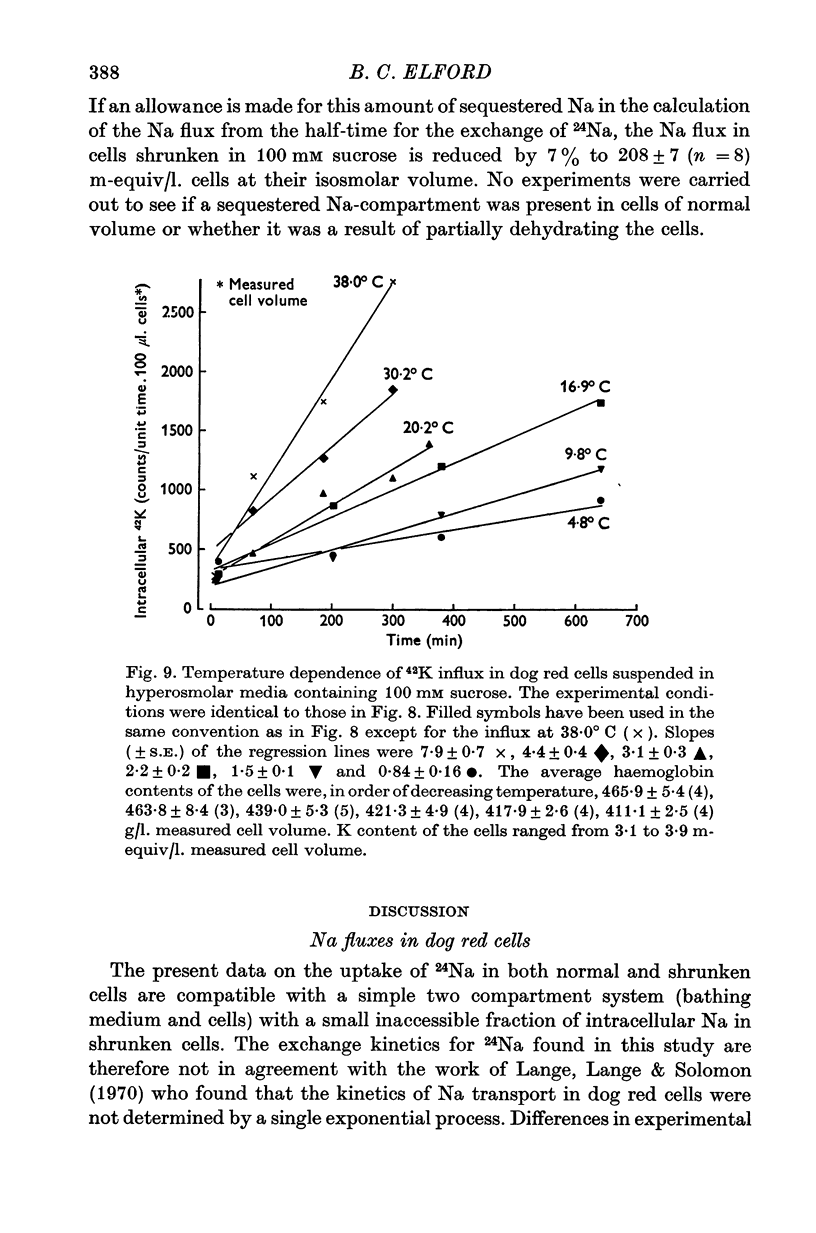
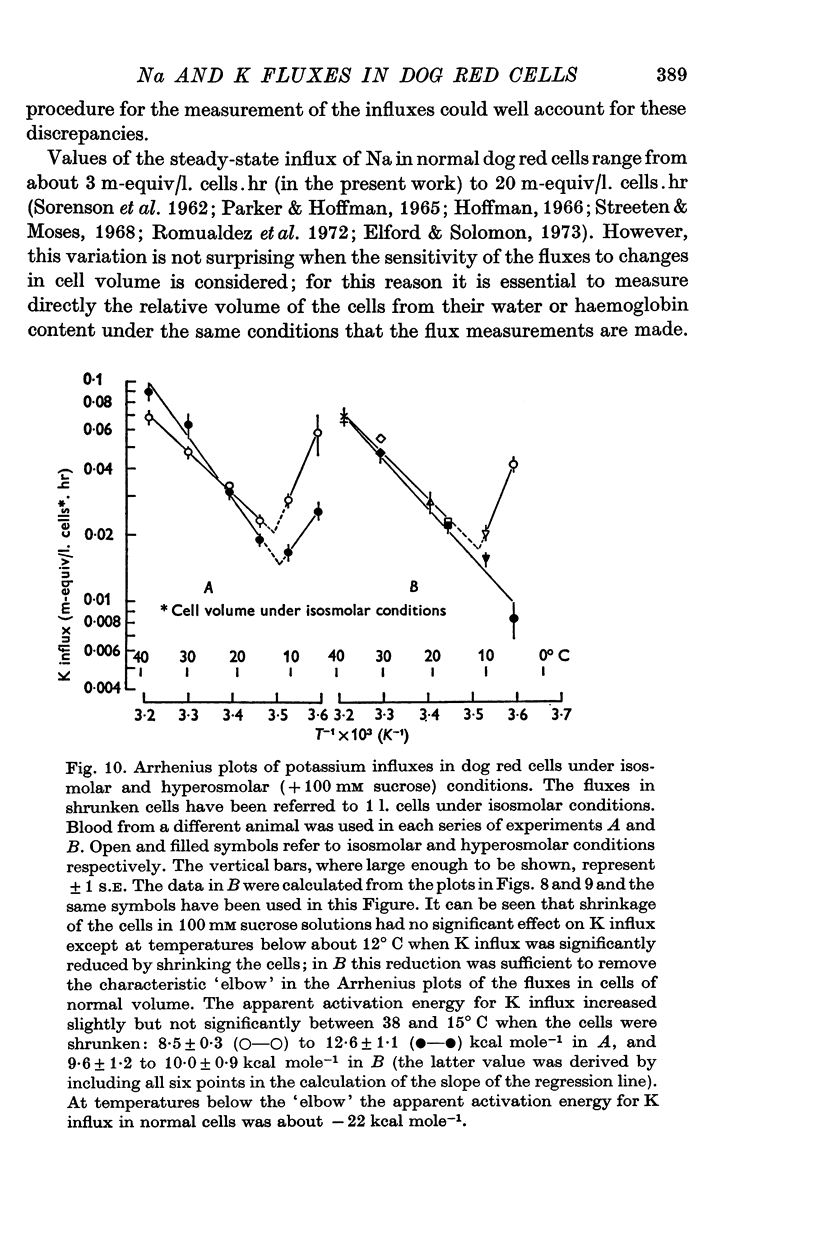
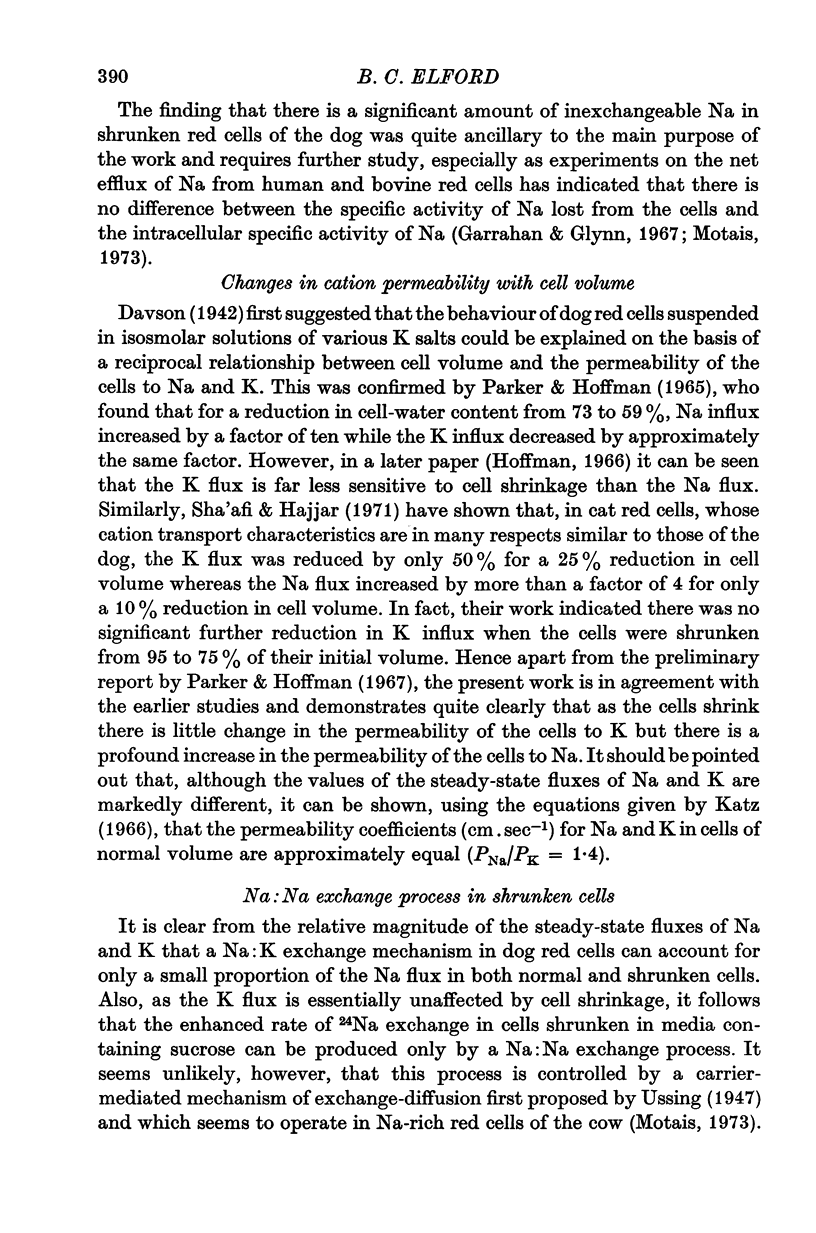
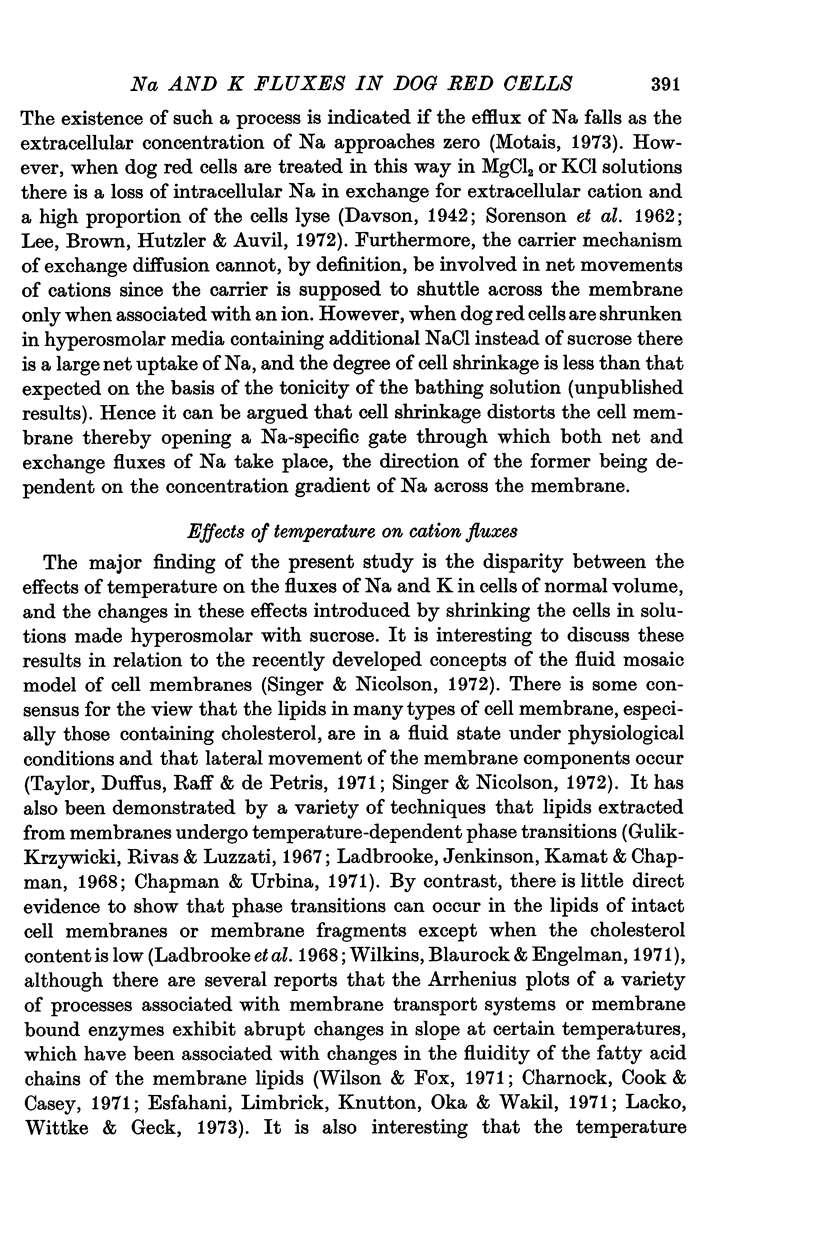
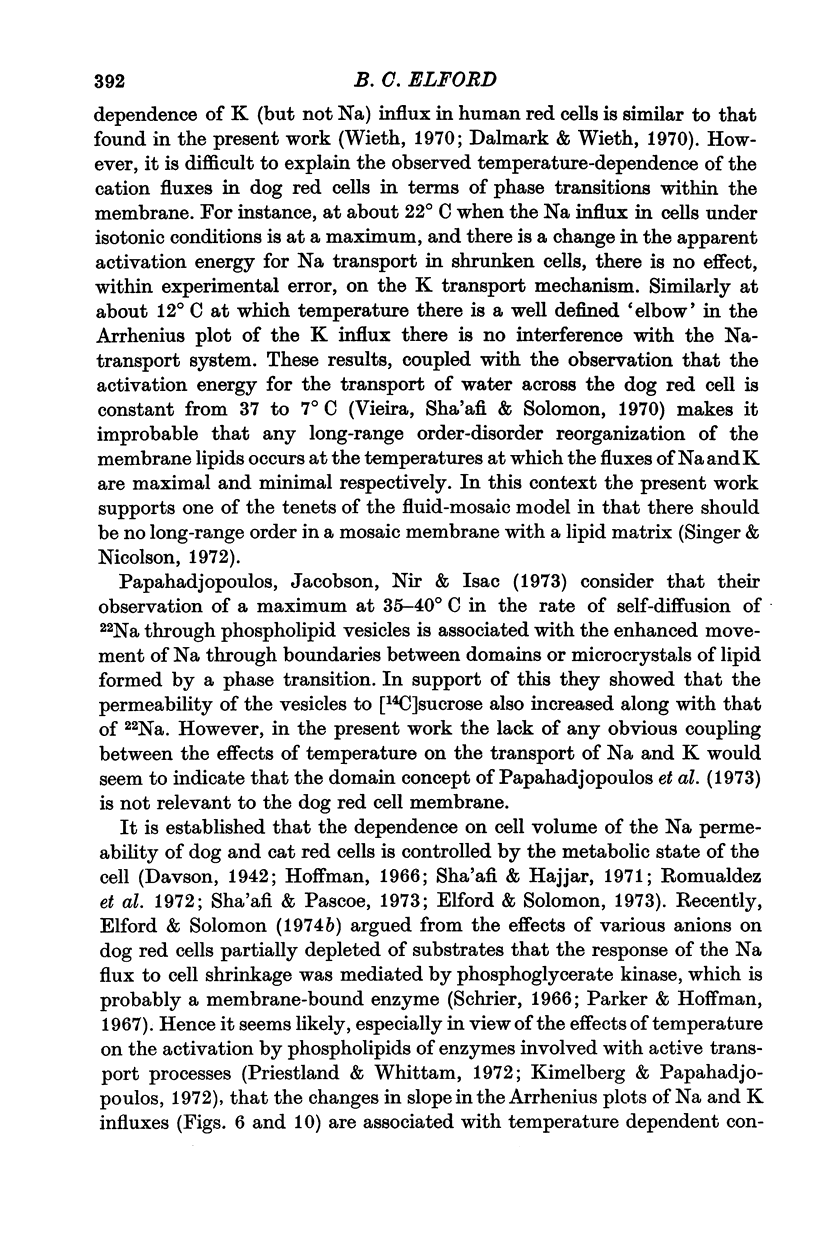
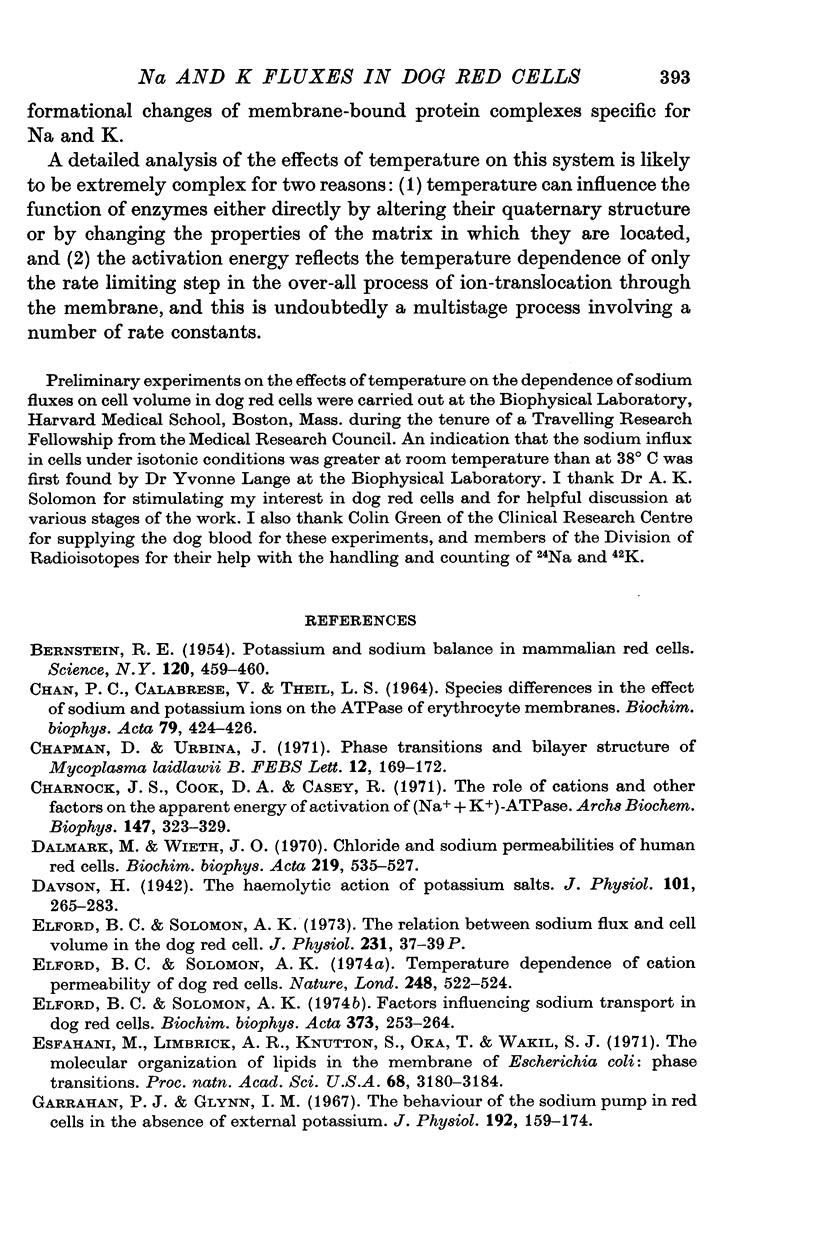
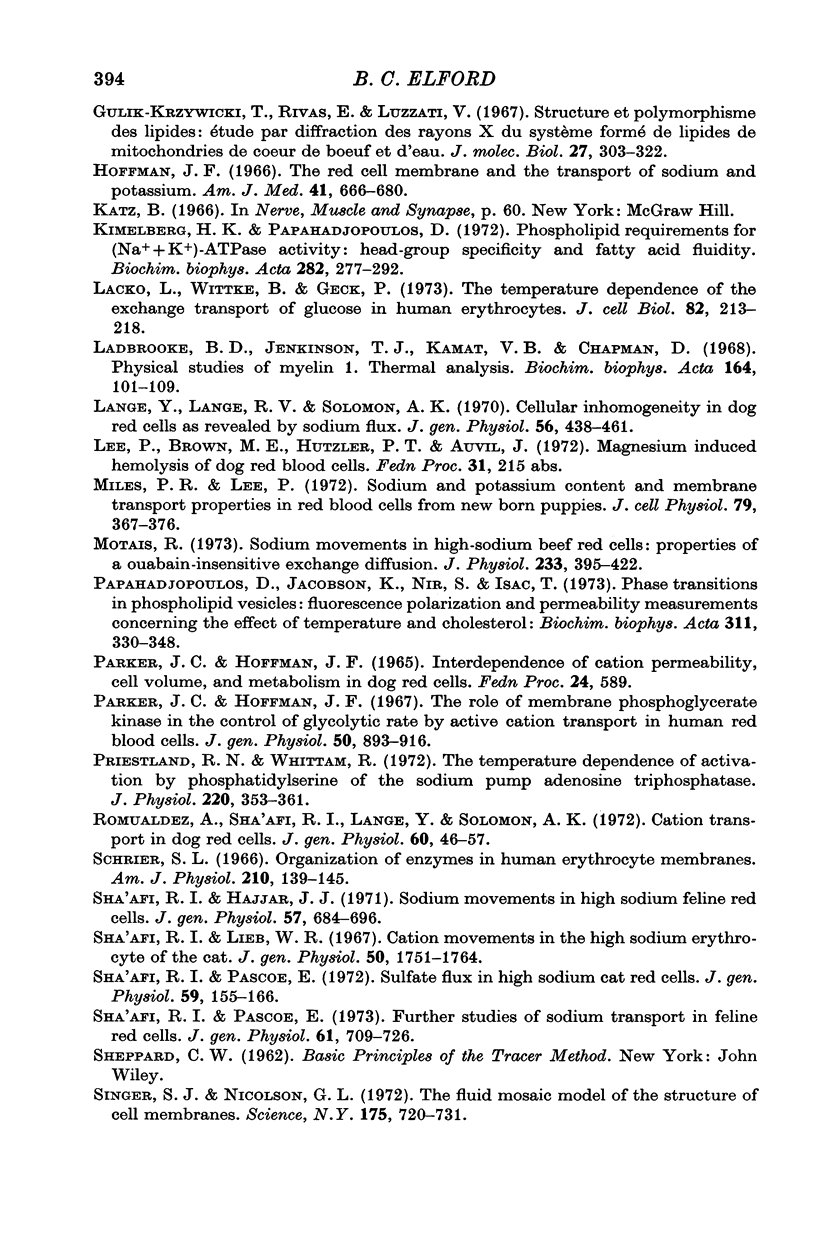
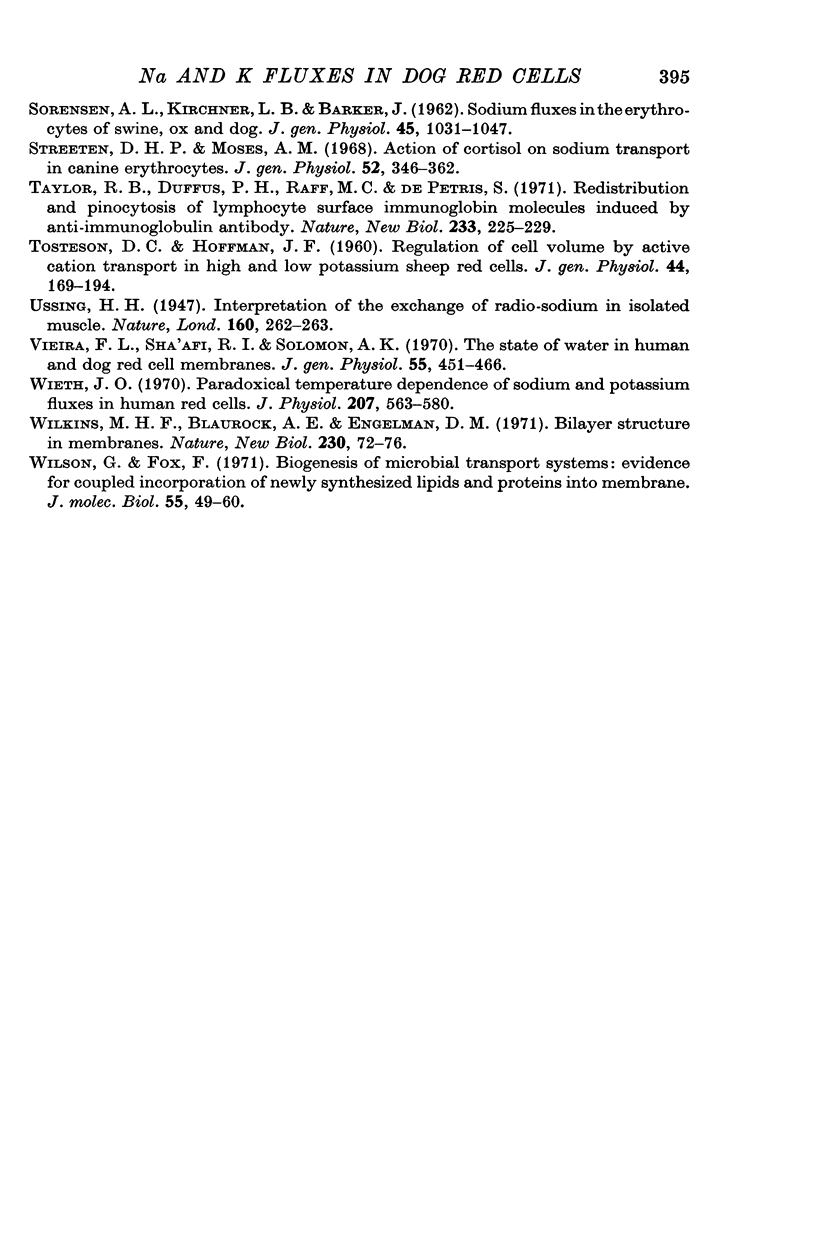
Selected References
These references are in PubMed. This may not be the complete list of references from this article.
- BERNSTEIN R. E. Potassium and sodium balance in mammalian red cells. Science. 1954 Sep 17;120(3116):459–460. doi: 10.1126/science.120.3116.459. [DOI] [PubMed] [Google Scholar]
- CHAN P. C., CALABRESE V., THEIL L. S. SPECIES DIFFERENCES IN THE EFFECT OF SODIUM AND POTASSIUM IONS ON THE ATPASE OF ERYTHROCYTE MEMBRANES. Biochim Biophys Acta. 1964 Mar 30;79:424–426. [PubMed] [Google Scholar]
- Chapman D., Urbina J. Phase transitions and bilayer structure of Mycoplasma laidlawii B. FEBS Lett. 1971 Jan 12;12(3):169–172. doi: 10.1016/0014-5793(71)80060-1. [DOI] [PubMed] [Google Scholar]
- Charnock J. S., Cook D. A., Casey R. The role of cations and other factors on the apparent energy of activation of (Na + + K + )-ATPase. Arch Biochem Biophys. 1971 Nov;147(1):323–329. doi: 10.1016/0003-9861(71)90340-7. [DOI] [PubMed] [Google Scholar]
- Dalmark M., Wieth J. O. Chloride and sodium permeabilities of human red cells. Biochim Biophys Acta. 1970 Dec 1;219(2):525–527. doi: 10.1016/0005-2736(70)90239-7. [DOI] [PubMed] [Google Scholar]
- Davson H. The haemolytic action of potassium salts. J Physiol. 1942 Nov 30;101(3):265–283. doi: 10.1113/jphysiol.1942.sp003981. [DOI] [PMC free article] [PubMed] [Google Scholar]
- Elford B. C., Solomon A. K. Factors influencing Na+ transport in dog red cells. Biochim Biophys Acta. 1974 Dec 10;373(2):253–264. doi: 10.1016/0005-2736(74)90149-7. [DOI] [PubMed] [Google Scholar]
- Elford B. C., Solomon A. K. Temperature dependence of cation permeability of dog red cells. Nature. 1974 Apr 5;248(448):522–524. doi: 10.1038/248522a0. [DOI] [PubMed] [Google Scholar]
- Esfahani M., Limbrick A. R., Knutton S., Oka T., Wakil S. J. The molecular organization of lipids in the membrane of Escherichia coli: phase transitions. Proc Natl Acad Sci U S A. 1971 Dec;68(12):3180–3184. doi: 10.1073/pnas.68.12.3180. [DOI] [PMC free article] [PubMed] [Google Scholar]
- Garrahan P. J., Glynn I. M. The behaviour of the sodium pump in red cells in the absence of external potassium. J Physiol. 1967 Sep;192(1):159–174. doi: 10.1113/jphysiol.1967.sp008294. [DOI] [PMC free article] [PubMed] [Google Scholar]
- Gulik-Krzywicki T., Rivas E., Luzzati V. Structure et polymorphisme des lipides: étude par diffraction des rayons X du systéme formé de lipides de mitochondries de coeur de boeuf et d'eau. J Mol Biol. 1967 Jul 28;27(2):303–322. doi: 10.1016/0022-2836(67)90022-8. [DOI] [PubMed] [Google Scholar]
- Hoffman J. F. The red cell membrane and the transport of sodium and potassium. Am J Med. 1966 Nov;41(5):666–680. doi: 10.1016/0002-9343(66)90029-5. [DOI] [PubMed] [Google Scholar]
- Kimelberg H. K., Papahadjopoulos D. Phospholipid requirements for (Na + + K + )-ATPase activity: head-group specificity and fatty acid fluidity. Biochim Biophys Acta. 1972 Sep 1;282(1):277–292. doi: 10.1016/0005-2736(72)90334-3. [DOI] [PubMed] [Google Scholar]
- Lacko L., Wittke B., Geck P. The temperature dependence of the exchange transport of glucose in human erythrocytes. J Cell Physiol. 1973 Oct;82(2):213–218. doi: 10.1002/jcp.1040820209. [DOI] [PubMed] [Google Scholar]
- Ladbrooke B. D., Jenkinson T. J., Kamat V. B., Chapman D. Physical studies of myelin. I. Thermal analysis. Biochim Biophys Acta. 1968 Sep 2;164(1):101–109. doi: 10.1016/0005-2760(68)90076-3. [DOI] [PubMed] [Google Scholar]
- Lange Y., Lange R. V., Solomon A. K. Cellular inhomogeneity in dog red cells as revealed by sodium flux. J Gen Physiol. 1970 Oct;56(4):438–461. doi: 10.1085/jgp.56.4.438. [DOI] [PMC free article] [PubMed] [Google Scholar]
- Miles P. R., Lee P. Sodium and potassium content and membrane transport properties in red blood cells from newborn puppies. J Cell Physiol. 1972 Jun;79(3):367–376. doi: 10.1002/jcp.1040790307. [DOI] [PubMed] [Google Scholar]
- Motais R. Sodium movements in high-sodium beef red cells: properties of a ouabain-insensitive exchange diffusion. J Physiol. 1973 Sep;233(2):395–422. doi: 10.1113/jphysiol.1973.sp010314. [DOI] [PMC free article] [PubMed] [Google Scholar]
- Papahadjopoulos D., Jacobson K., Nir S., Isac T. Phase transitions in phospholipid vesicles. Fluorescence polarization and permeability measurements concerning the effect of temperature and cholesterol. Biochim Biophys Acta. 1973 Jul 6;311(3):330–348. doi: 10.1016/0005-2736(73)90314-3. [DOI] [PubMed] [Google Scholar]
- Parker J. C., Hoffman J. F. The role of membrane phosphoglycerate kinase in the control of glycolytic rate by active cation transport in human red blood cells. J Gen Physiol. 1967 Mar;50(4):893–916. doi: 10.1085/jgp.50.4.893. [DOI] [PMC free article] [PubMed] [Google Scholar]
- Priestland R. N., Whittam R. The temperature dependence of activation by phosphatidylserine of the sodium pump adenosine triphosphatase. J Physiol. 1972 Jan;220(2):353–361. doi: 10.1113/jphysiol.1972.sp009711. [DOI] [PMC free article] [PubMed] [Google Scholar]
- Romualdez A., Sha'afi R. I., Lange Y., Solomon A. K. Cation transport in dog red cells. J Gen Physiol. 1972 Jul;60(1):46–57. doi: 10.1085/jgp.60.1.46. [DOI] [PMC free article] [PubMed] [Google Scholar]
- SORENSON A. L., KIRSCHNER L. B., BARKER J. Sodium fluxes in the erythrocytes of swine, ox, and dog. J Gen Physiol. 1962 Jul;45:1031–1047. doi: 10.1085/jgp.45.6.1031. [DOI] [PMC free article] [PubMed] [Google Scholar]
- Schrier S. L. Organization of enzymes in human erythrocyte membranes. Am J Physiol. 1966 Jan;210(1):139–145. doi: 10.1152/ajplegacy.1966.210.1.139. [DOI] [PubMed] [Google Scholar]
- Sha'afi R. I., Hajjar J. J. Sodium movement in high sodium feline red cells. J Gen Physiol. 1971 Jun;57(6):684–696. doi: 10.1085/jgp.57.6.684. [DOI] [PMC free article] [PubMed] [Google Scholar]
- Sha'afi R. I., Lieb W. R. Cation movements in the high sodium erythrocyte of the cat. J Gen Physiol. 1967 Jul;50(6):1751–1764. doi: 10.1085/jgp.50.6.1751. [DOI] [PMC free article] [PubMed] [Google Scholar]
- Sha'afi R. I., Pascoe E. Further studies of sodium transport in feline red cells. J Gen Physiol. 1973 Jun;61(6):709–726. doi: 10.1085/jgp.61.6.709. [DOI] [PMC free article] [PubMed] [Google Scholar]
- Sha'afi R. I., Pascoe E. Sulfate flux in high sodium cat red cells. J Gen Physiol. 1972 Feb;59(2):155–166. doi: 10.1085/jgp.59.2.155. [DOI] [PMC free article] [PubMed] [Google Scholar]
- Singer S. J., Nicolson G. L. The fluid mosaic model of the structure of cell membranes. Science. 1972 Feb 18;175(4023):720–731. doi: 10.1126/science.175.4023.720. [DOI] [PubMed] [Google Scholar]
- Streeten D. H., Moses A. M. Action of cortisol on sodium transport in canine erythrocytes. J Gen Physiol. 1968 Aug;52(2):346–362. doi: 10.1085/jgp.52.2.346. [DOI] [PMC free article] [PubMed] [Google Scholar]
- TOSTESON D. C., HOFFMAN J. F. Regulation of cell volume by active cation transport in high and low potassium sheep red cells. J Gen Physiol. 1960 Sep;44:169–194. doi: 10.1085/jgp.44.1.169. [DOI] [PMC free article] [PubMed] [Google Scholar]
- Vieira F. L., Sha'afi R. I., Solomon A. K. The state of water in human and dog red cell membranes. J Gen Physiol. 1970 Apr;55(4):451–466. doi: 10.1085/jgp.55.4.451. [DOI] [PMC free article] [PubMed] [Google Scholar]
- Wieth J. O. Paradoxical temperature dependence of sodium and potassium fluxes in human red cells. J Physiol. 1970 May;207(3):563–580. doi: 10.1113/jphysiol.1970.sp009081. [DOI] [PMC free article] [PubMed] [Google Scholar]
- Wilkins M. H., Blaurock A. E., Engelman D. M. Bilayer structure in membranes. Nat New Biol. 1971 Mar 17;230(11):72–76. doi: 10.1038/newbio230072a0. [DOI] [PubMed] [Google Scholar]
- Wilson G., Fox C. F. Biogenesis of microbial transport systems: evidnce for coupled incorporation of newly synthesized lipids and proteins into membrane. J Mol Biol. 1971 Jan 14;55(1):49–60. doi: 10.1016/0022-2836(71)90280-4. [DOI] [PubMed] [Google Scholar]