Abstract
Purposeful switching among different conformational states exerts self-control in the construction and action of protein assemblies. Quasi-equivalence, conceived to explain icosahedral virus structure, arises by differentiation of identical protein subunits into different conformations that conserve essential bonding specificity. Mechanical models designed to represent the energy distribution in the structure, rather than just the arrangement of matter, are used to explore flexibility and self-controlled movements in virus particles. Information about the assembly of bacterial flagella, actin, tobacco mosaic virus and the T4 bacteriophage tail structure show that assembly can be controlled by switching the subunits from an inactive, unsociable form to an active, associable form. Energy to drive this change is provided by the intersubunit bonding in the growing structure; this self-control of assembly by conformational switching is called "autostery", by homology with allostery. A mechanical model of the contractile T4 tail sheath has been constructed to demonstrate how self-controlled activation of a latent bonding potential can drive a purposeful movement. The gradient of quasi-equivalent conformations modelled in the contracting tail sheath has suggested a workable mechanism for self-determination of tail tube length. Concerted action by assemblies of identical proteins may often depend on individually differentiated movements.
Full text
PDF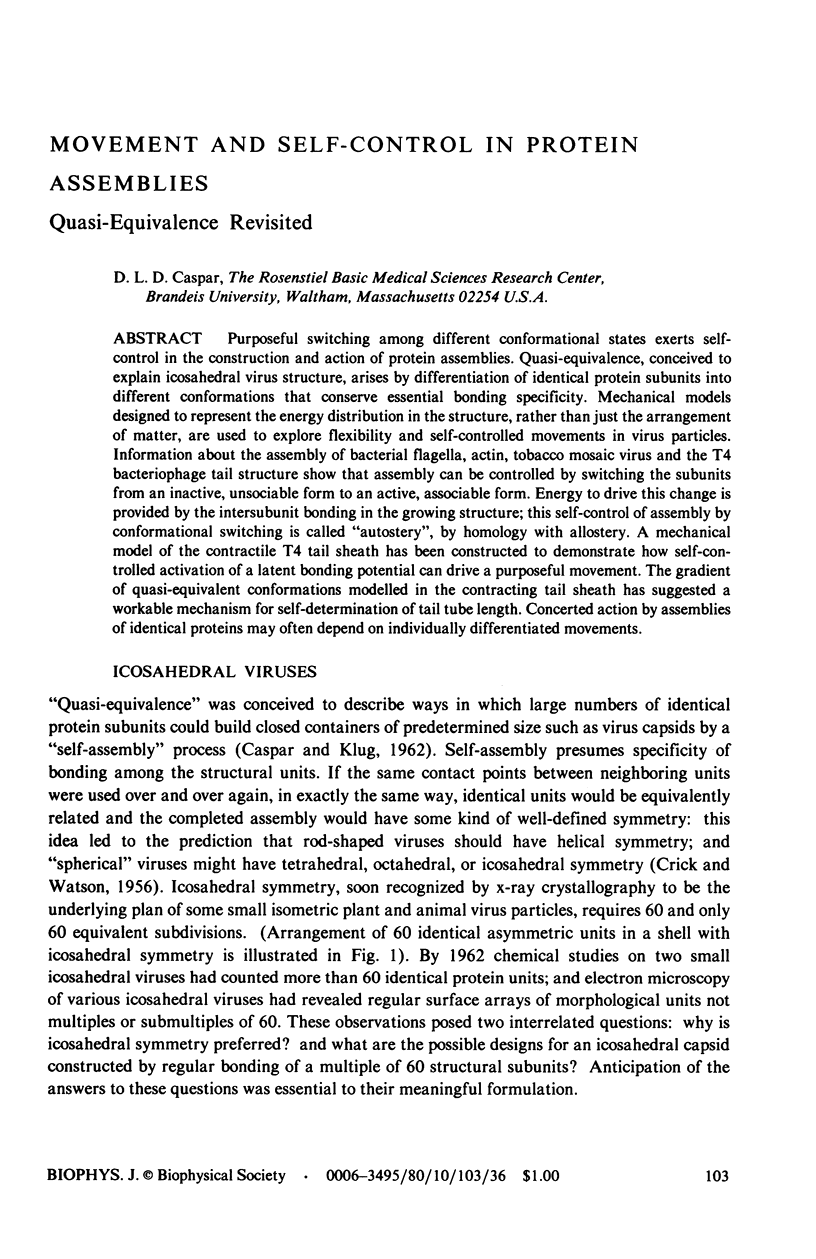
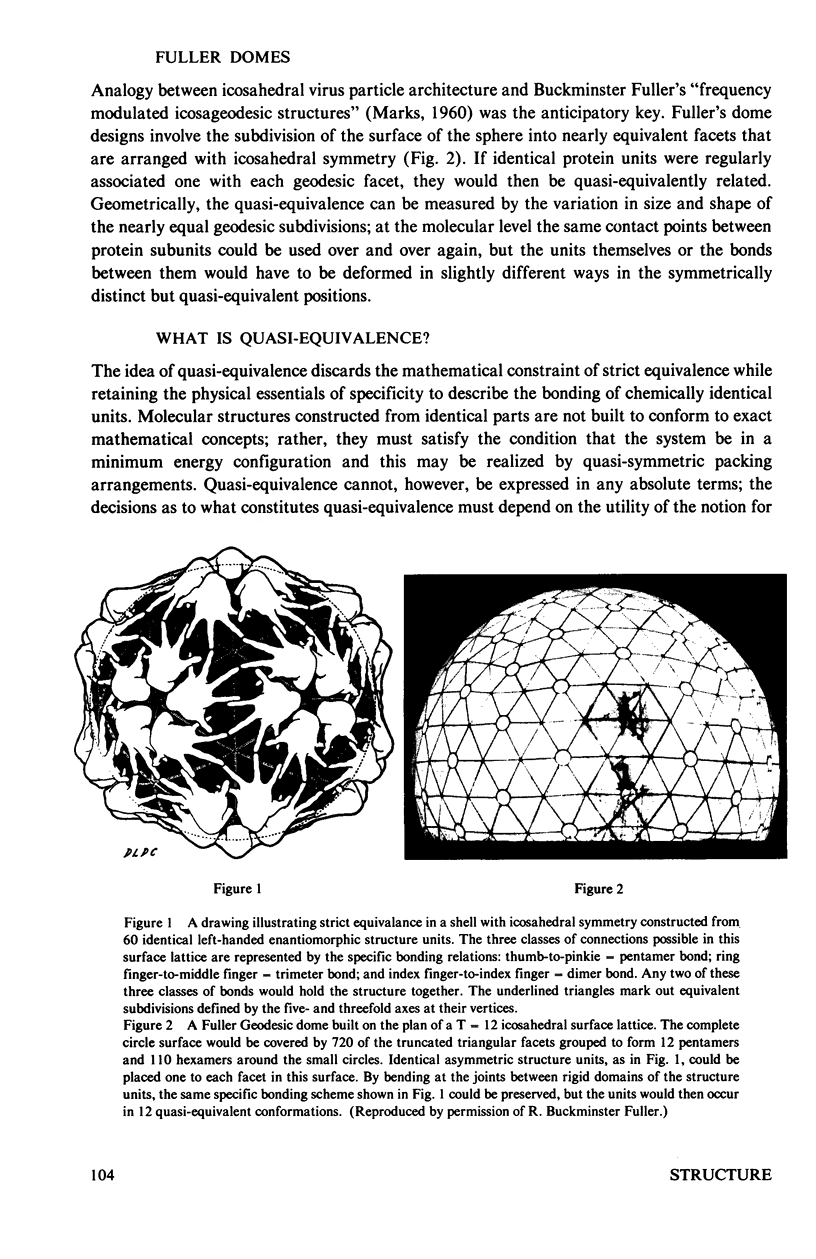
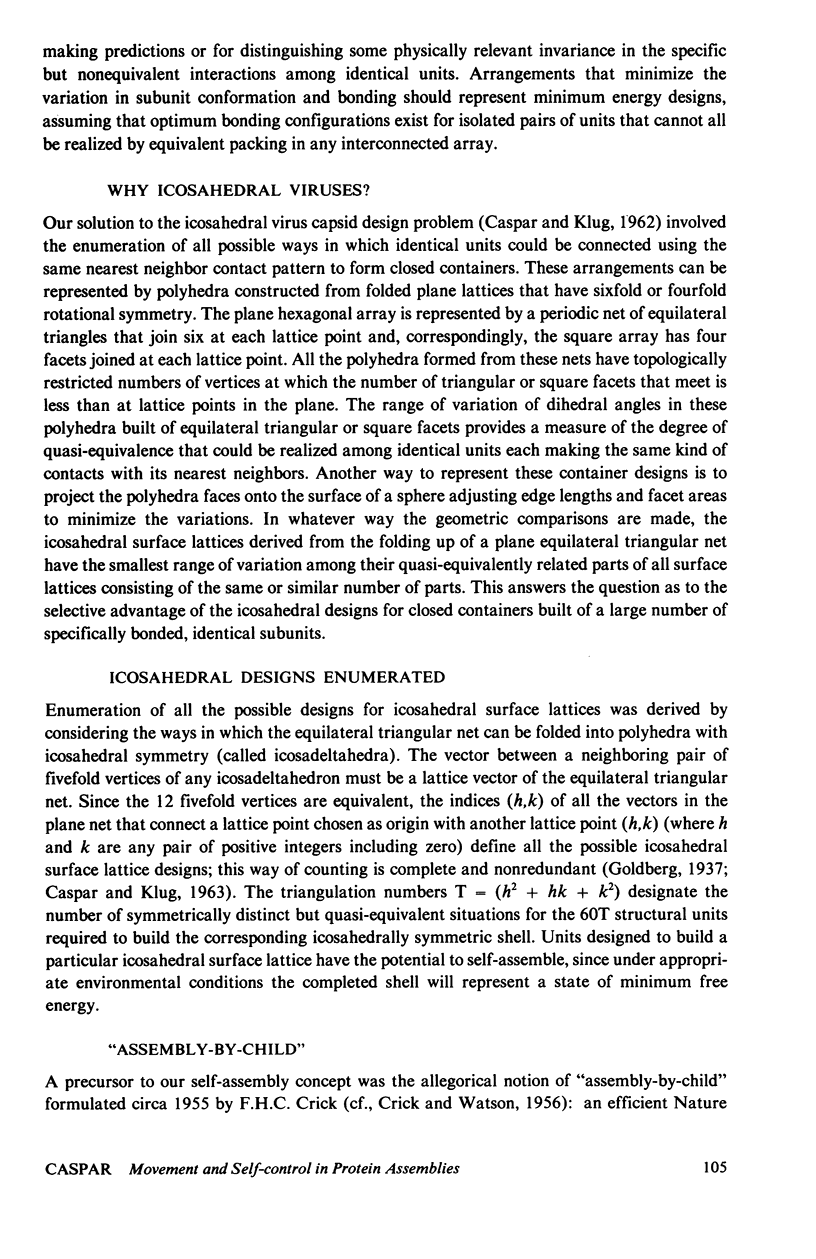
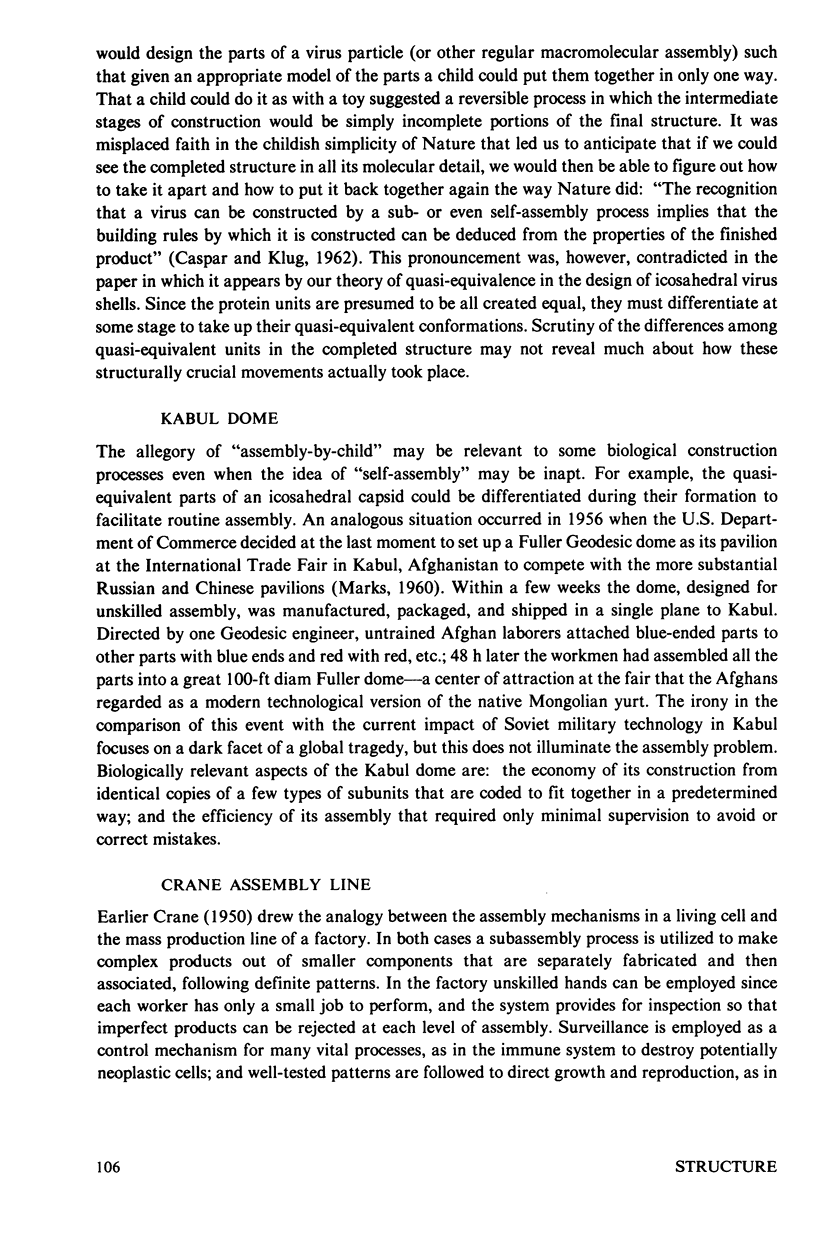
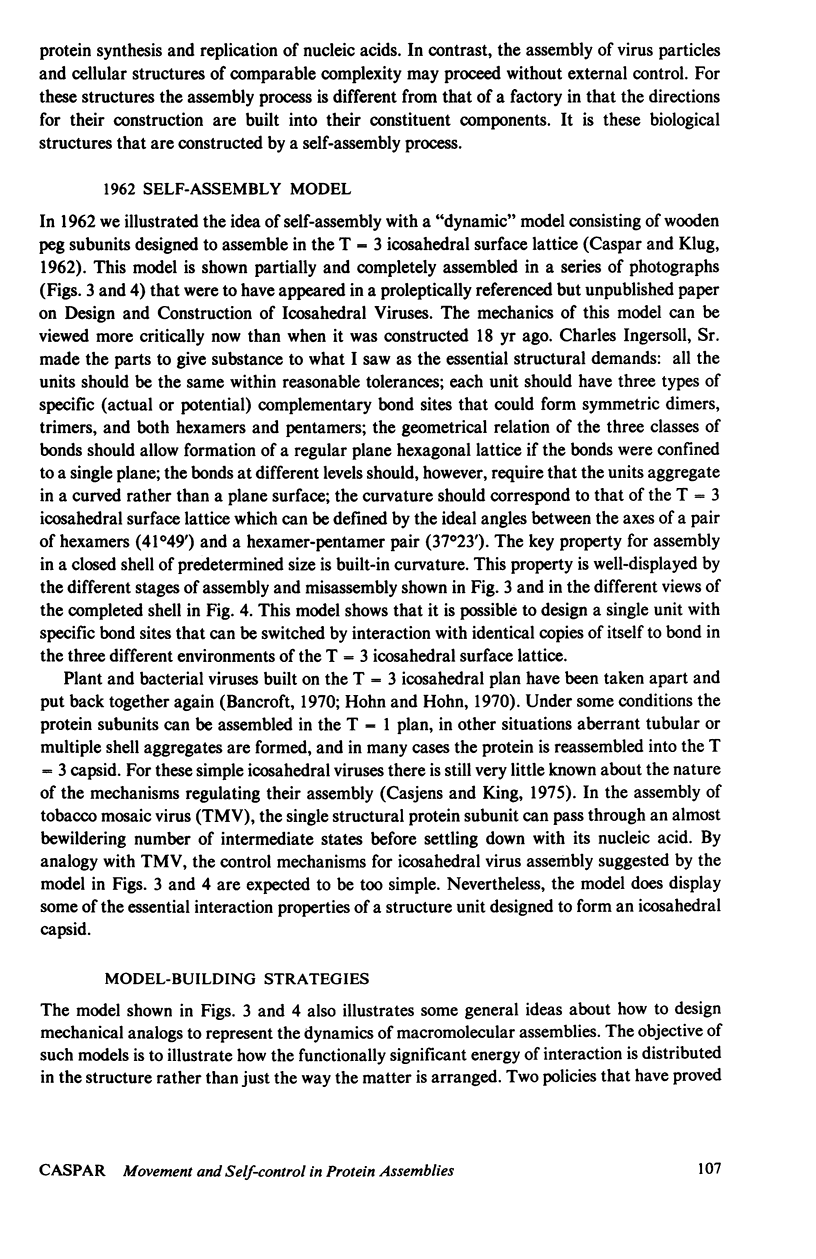
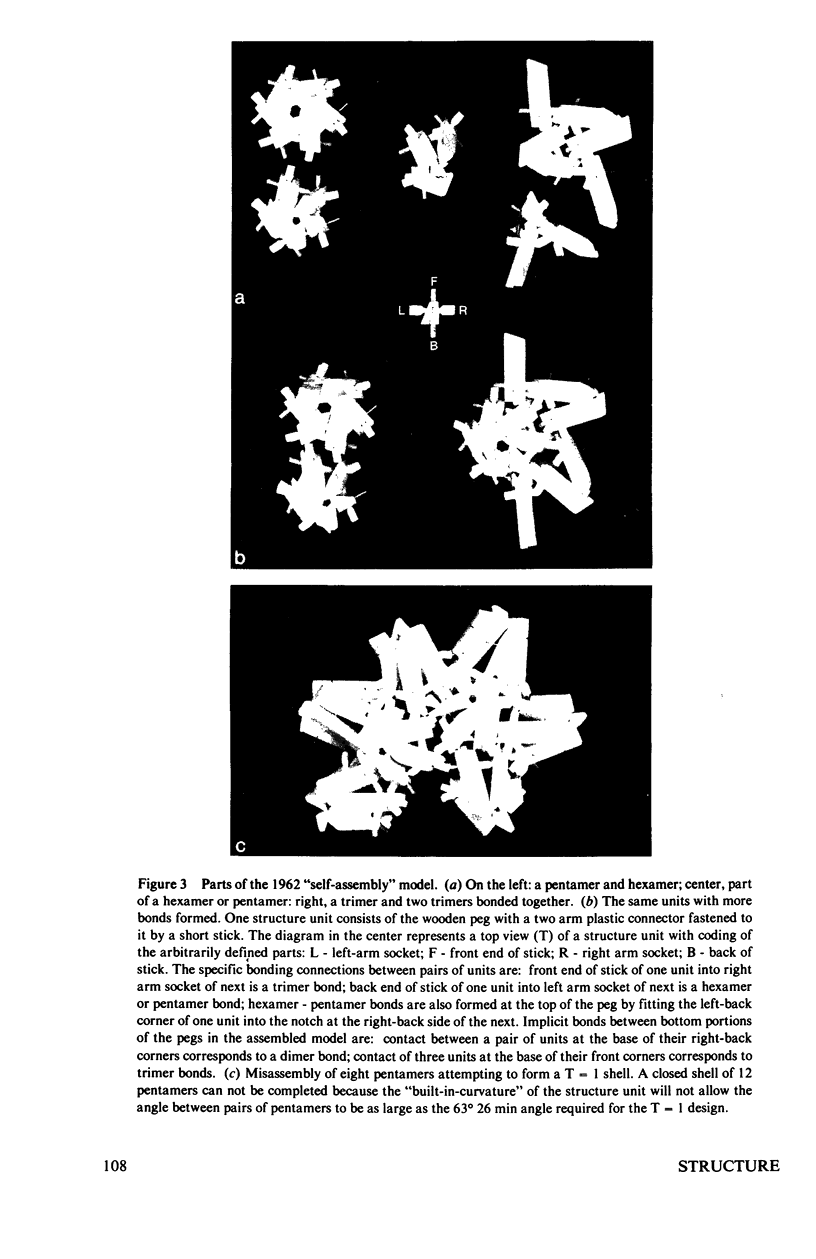
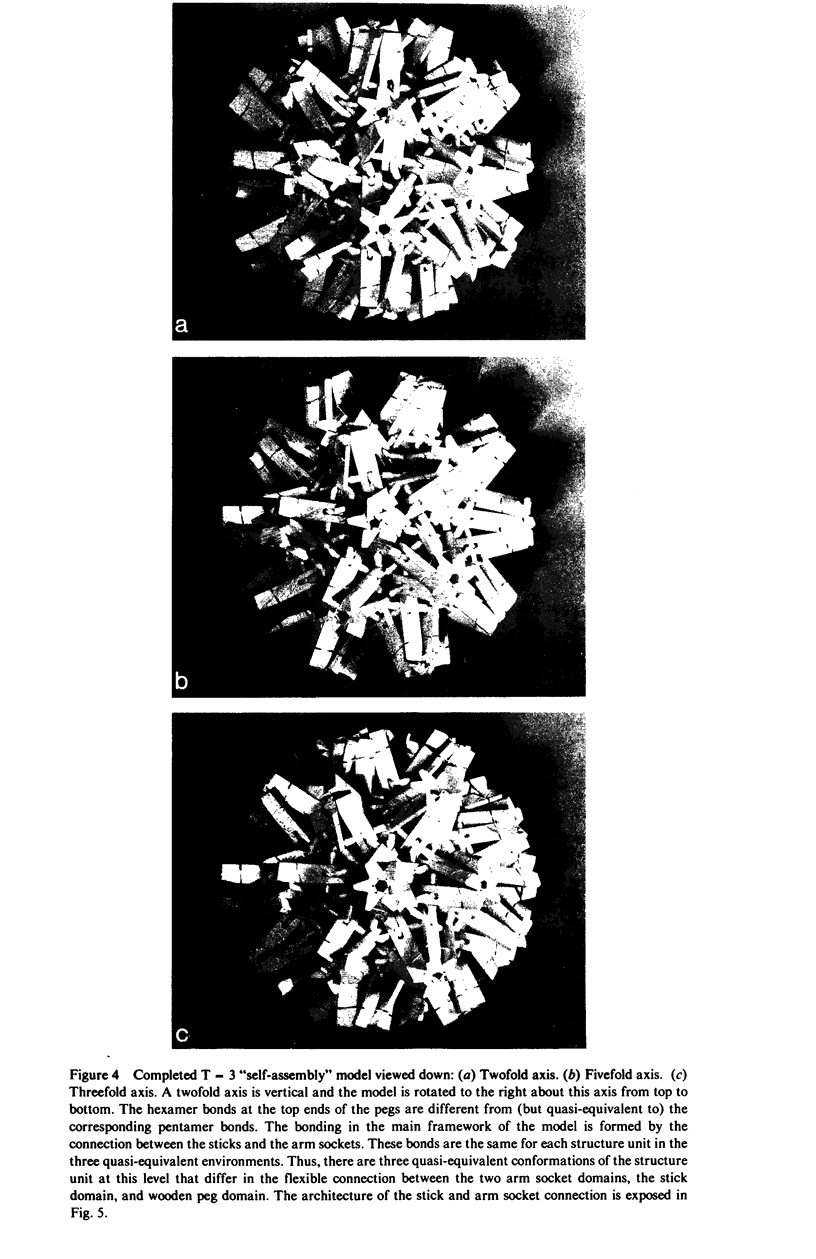
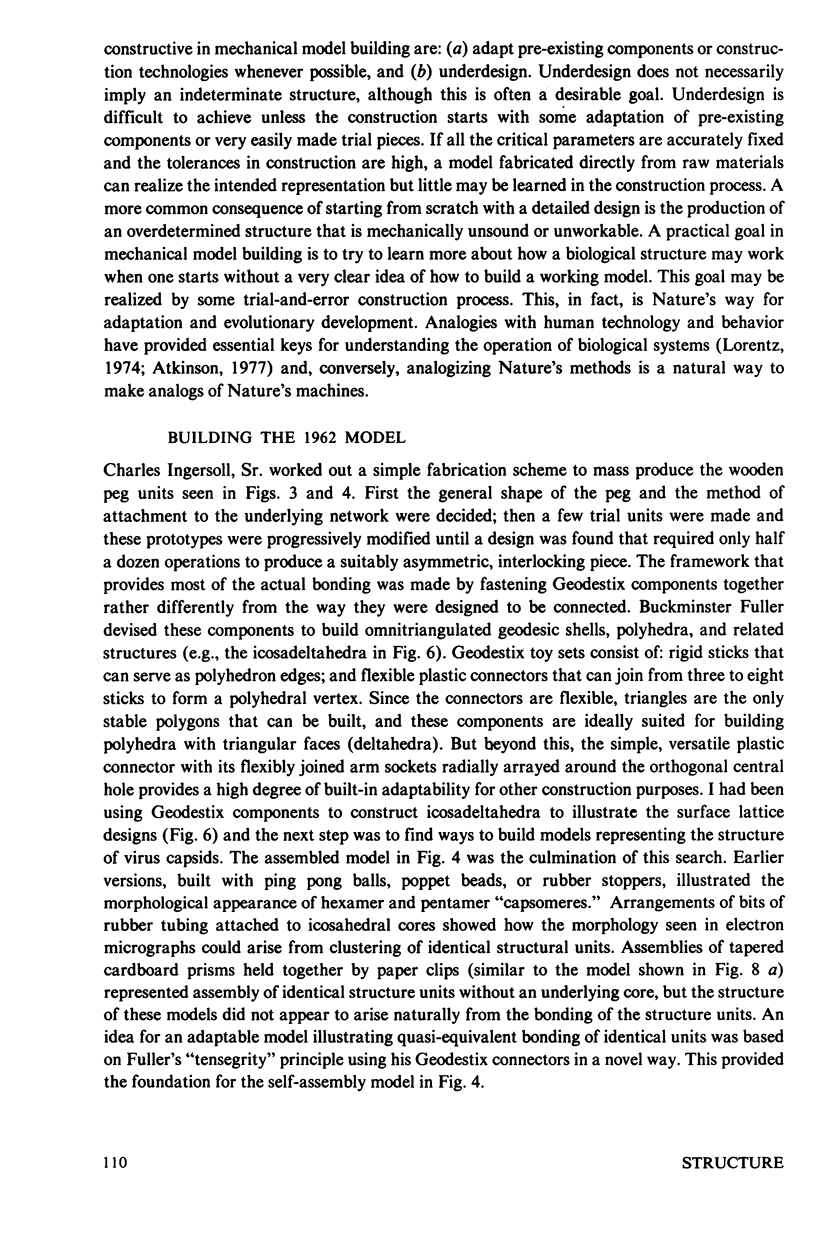
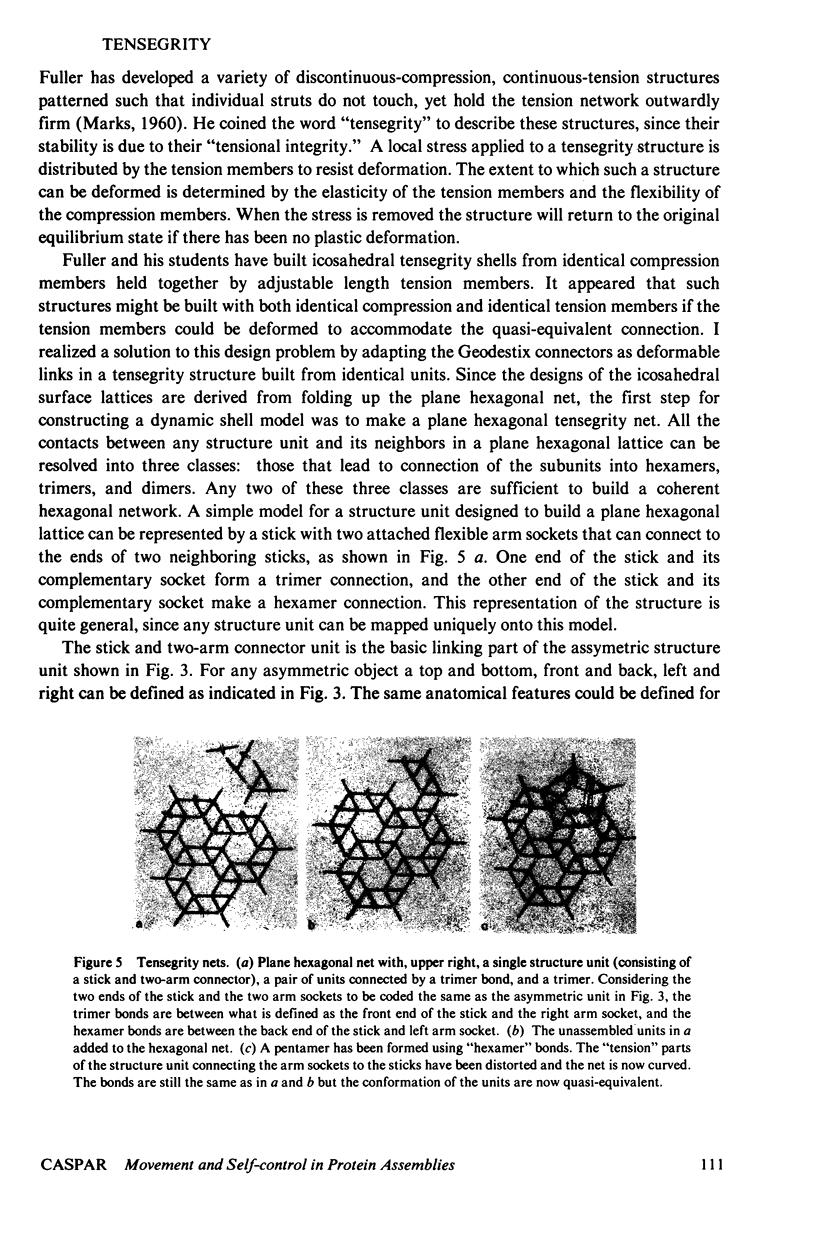
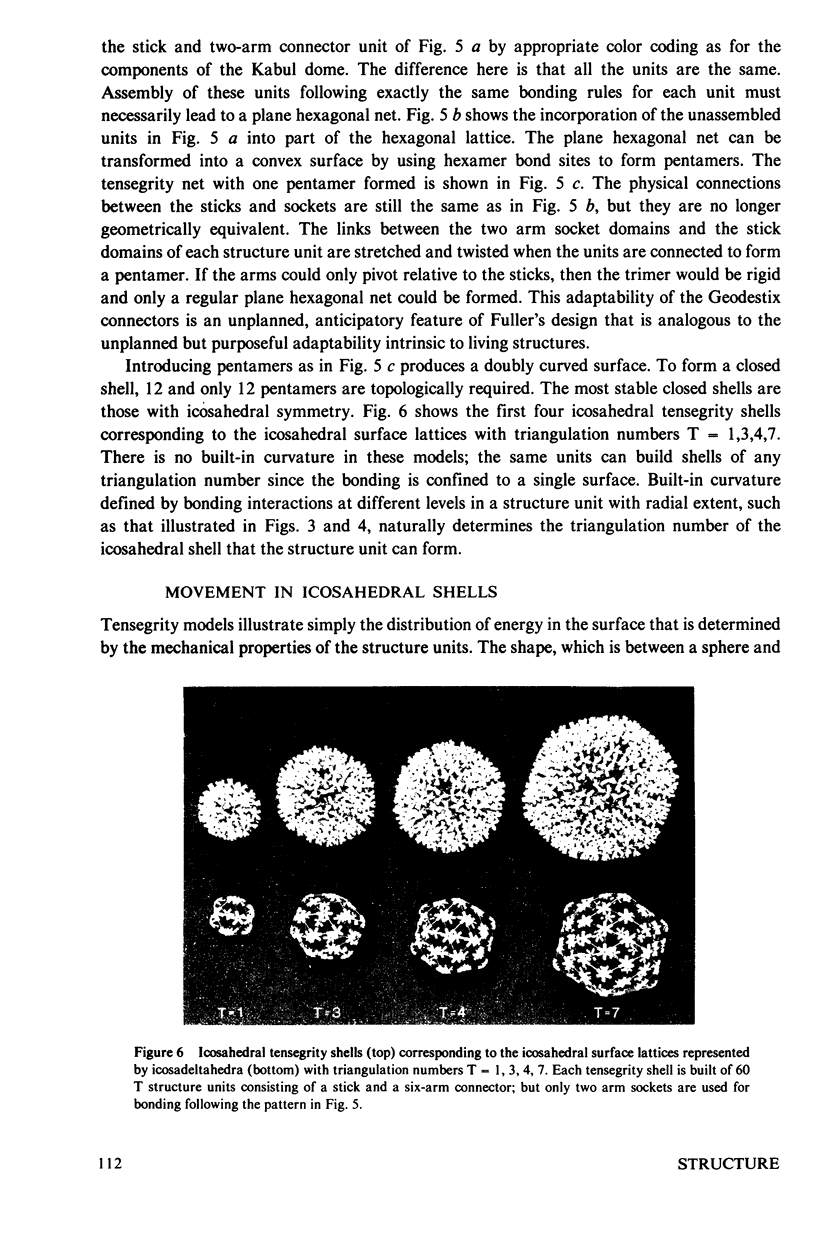
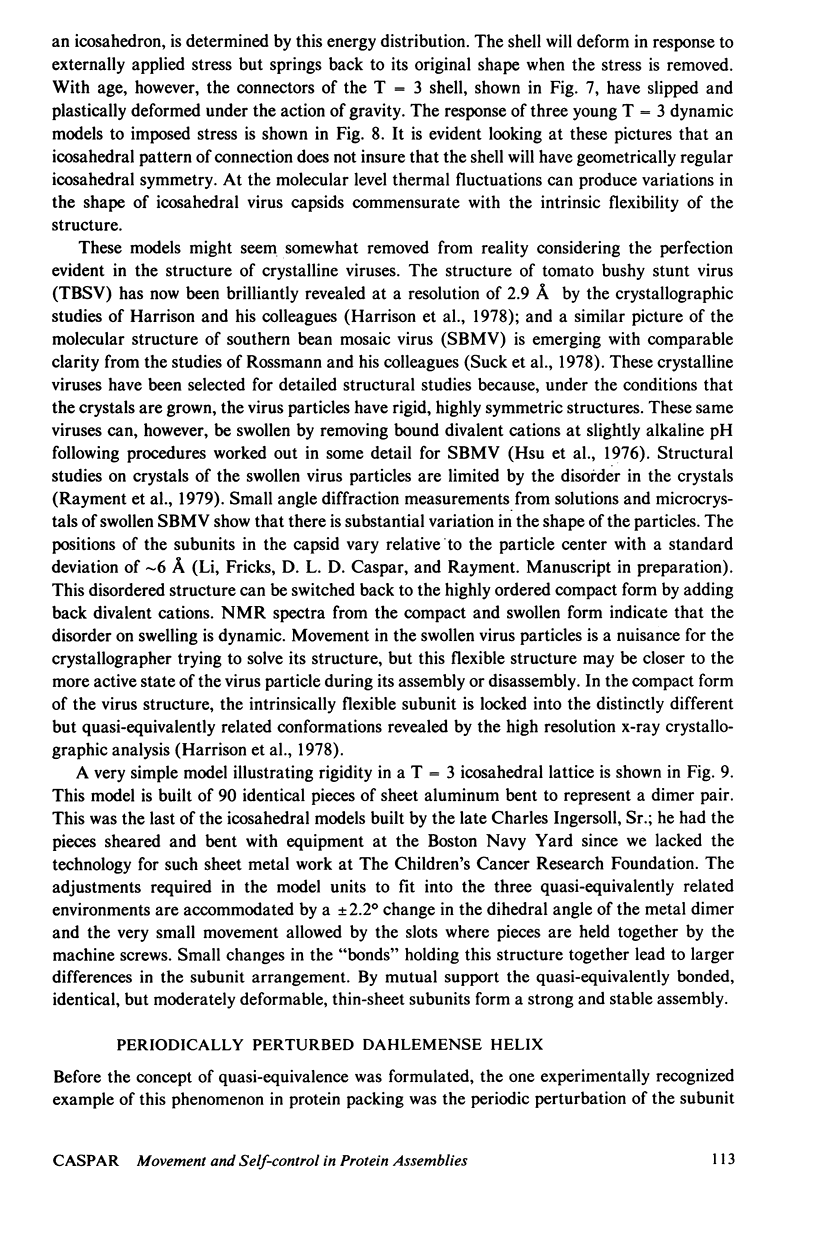
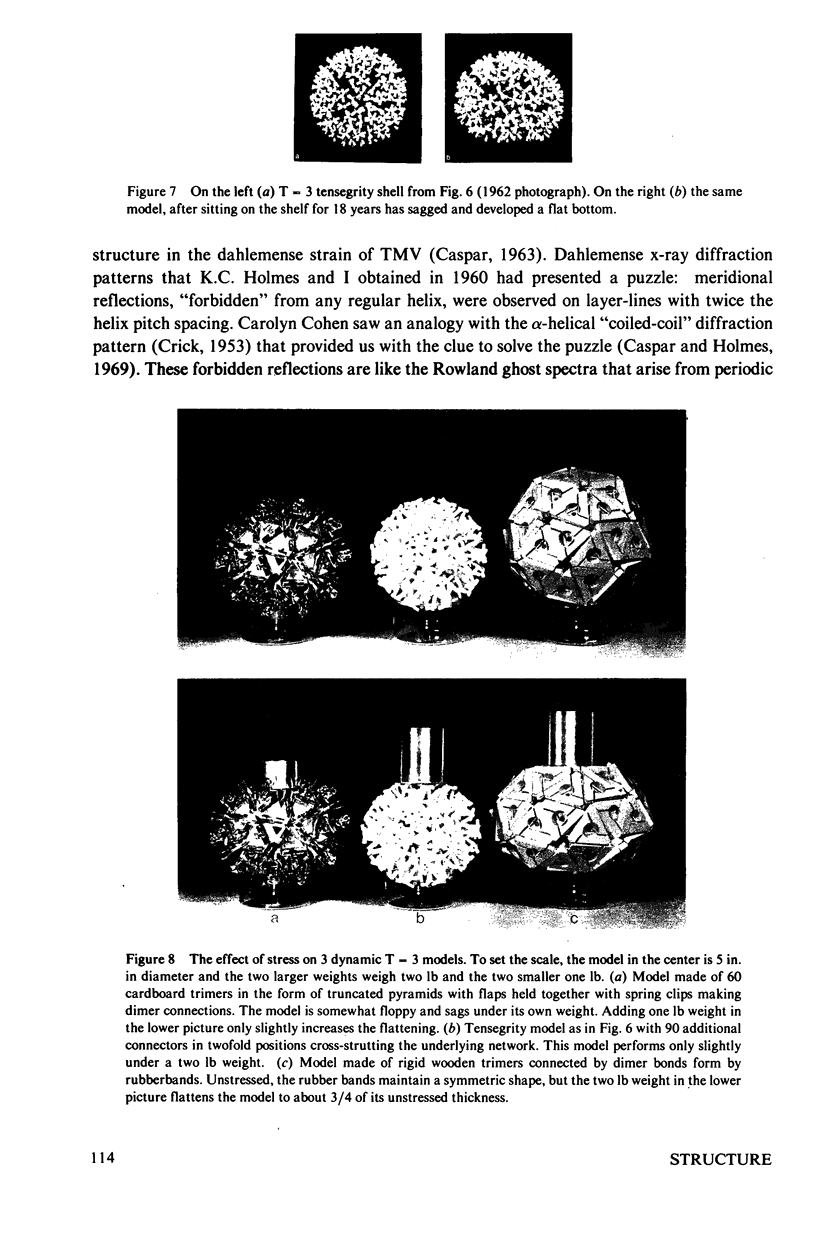
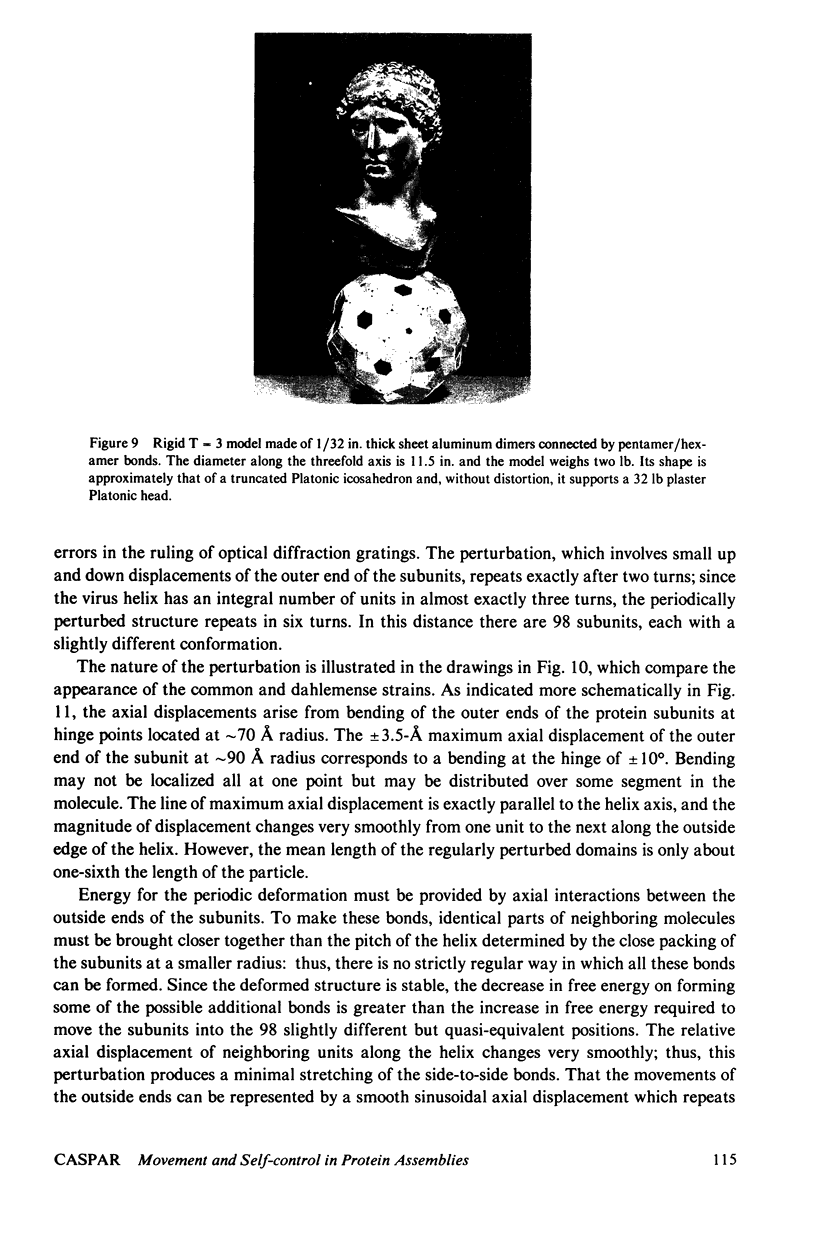
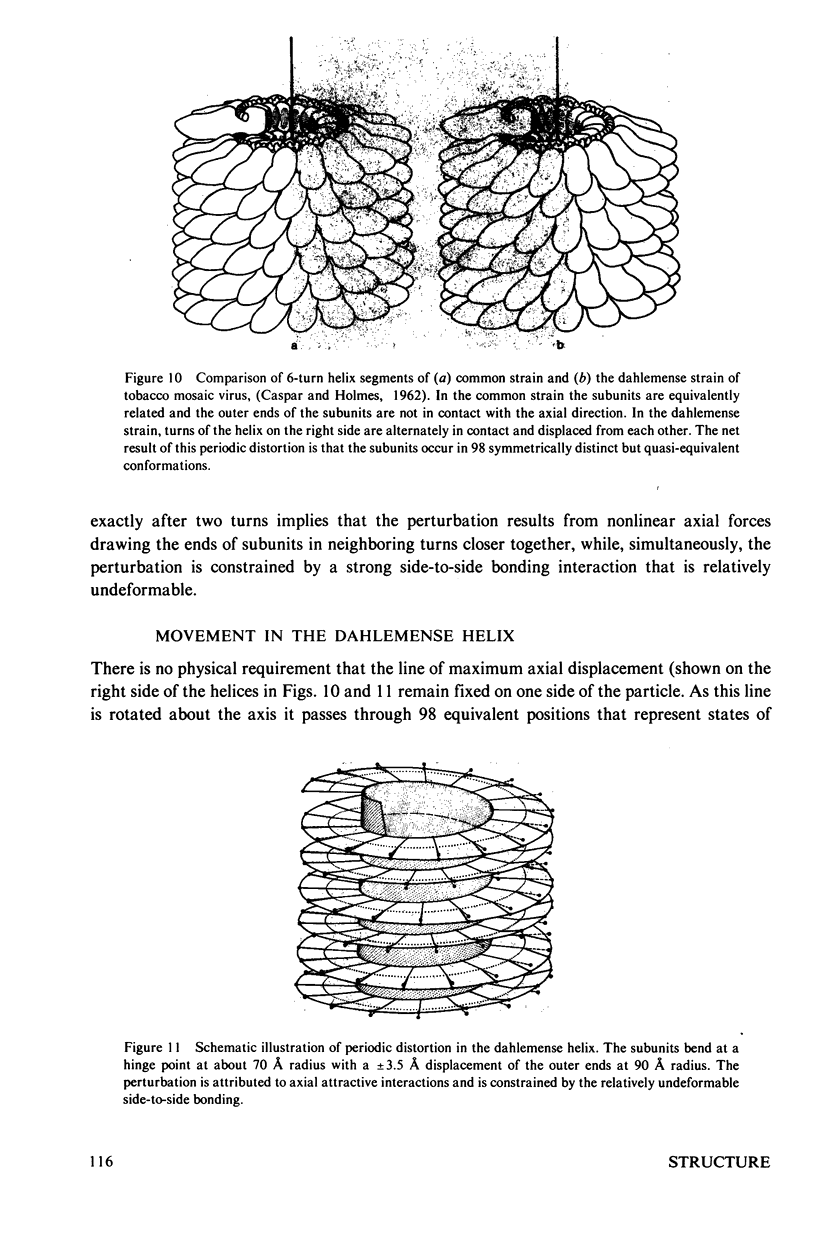
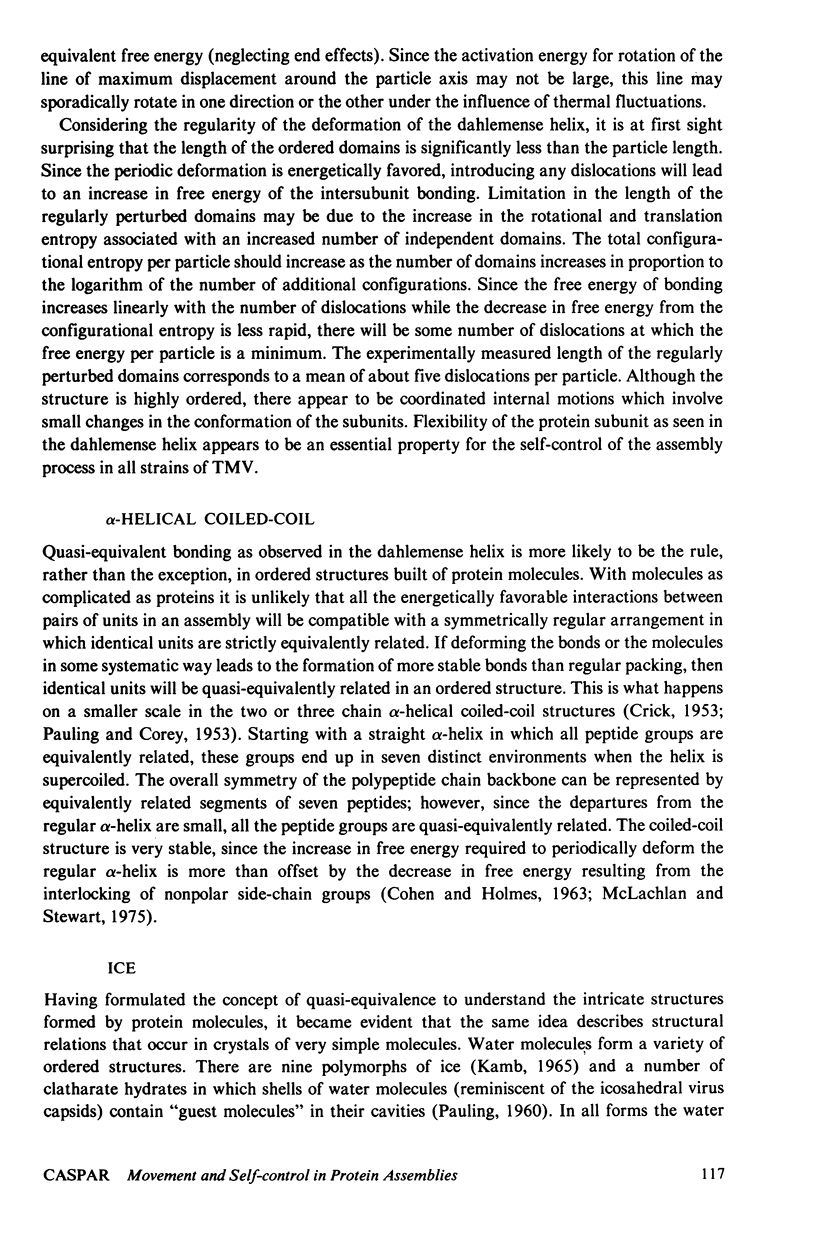
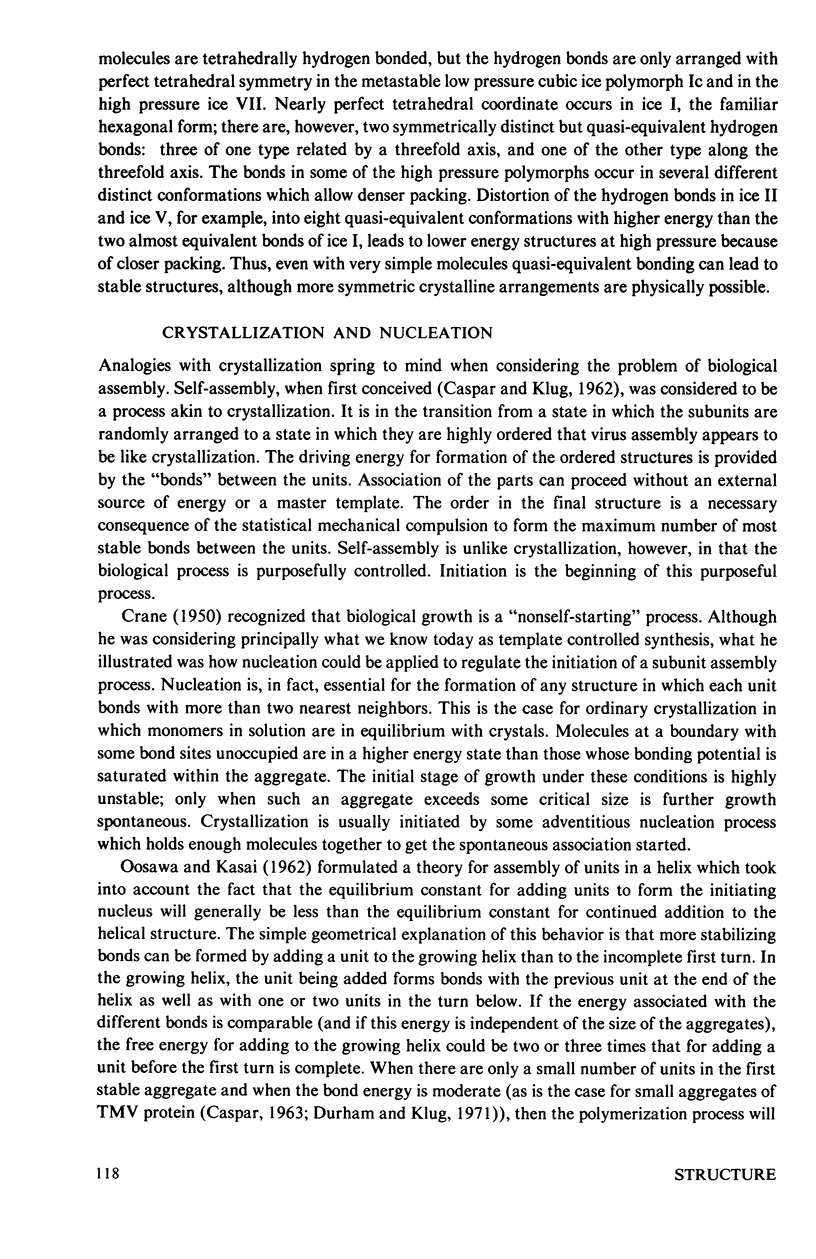
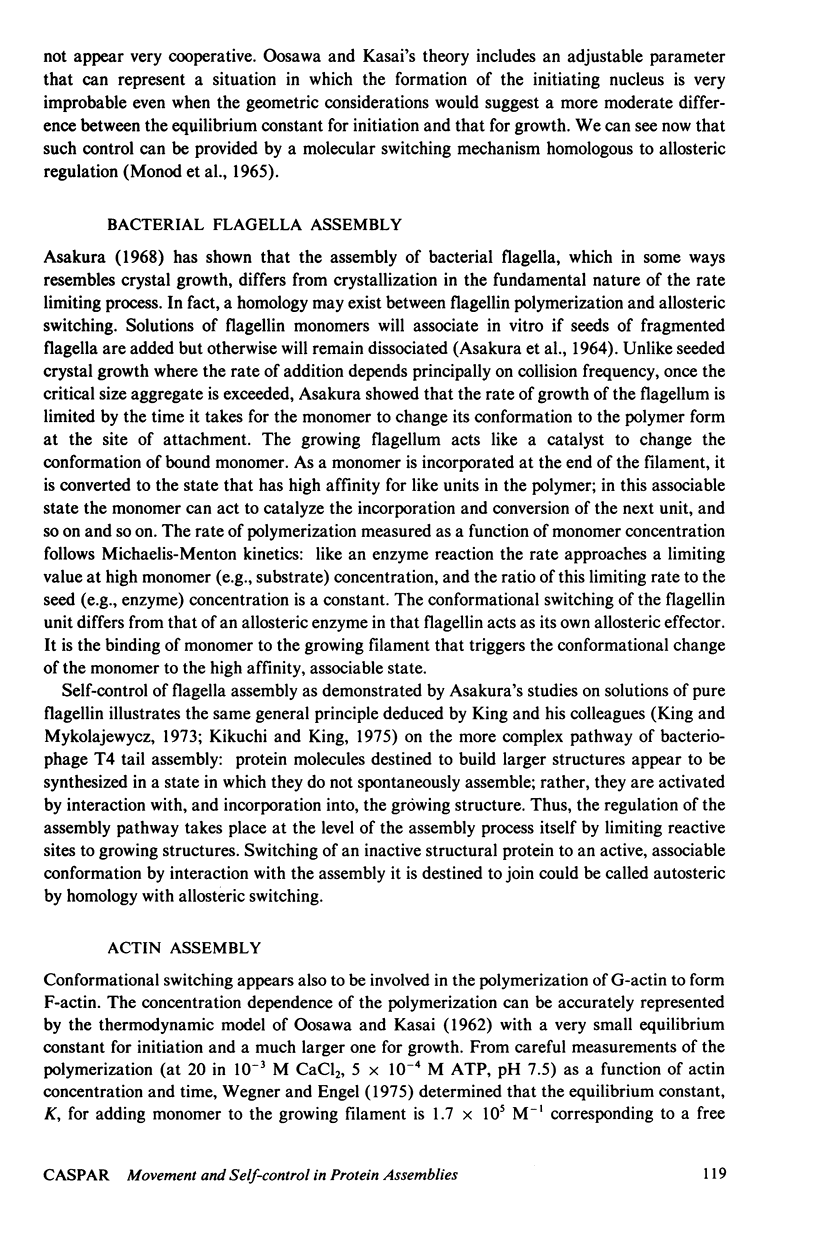
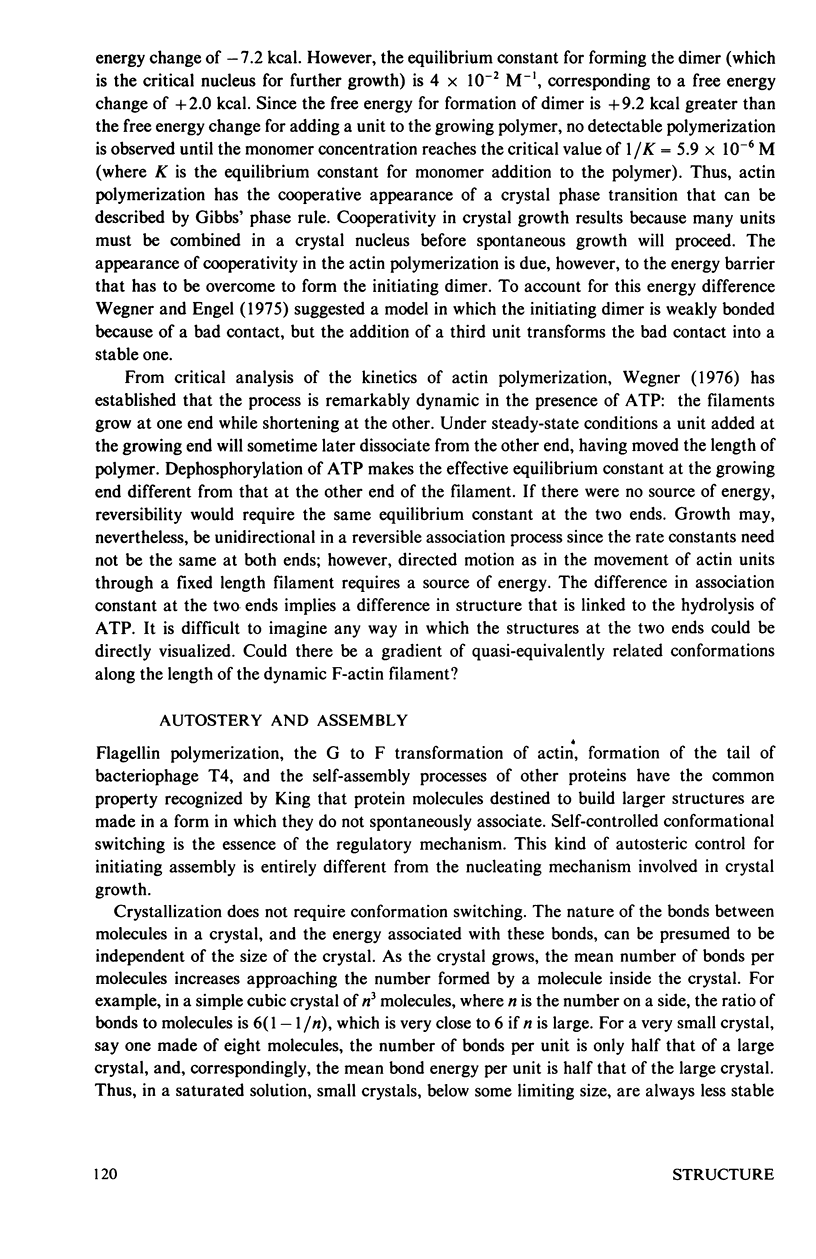
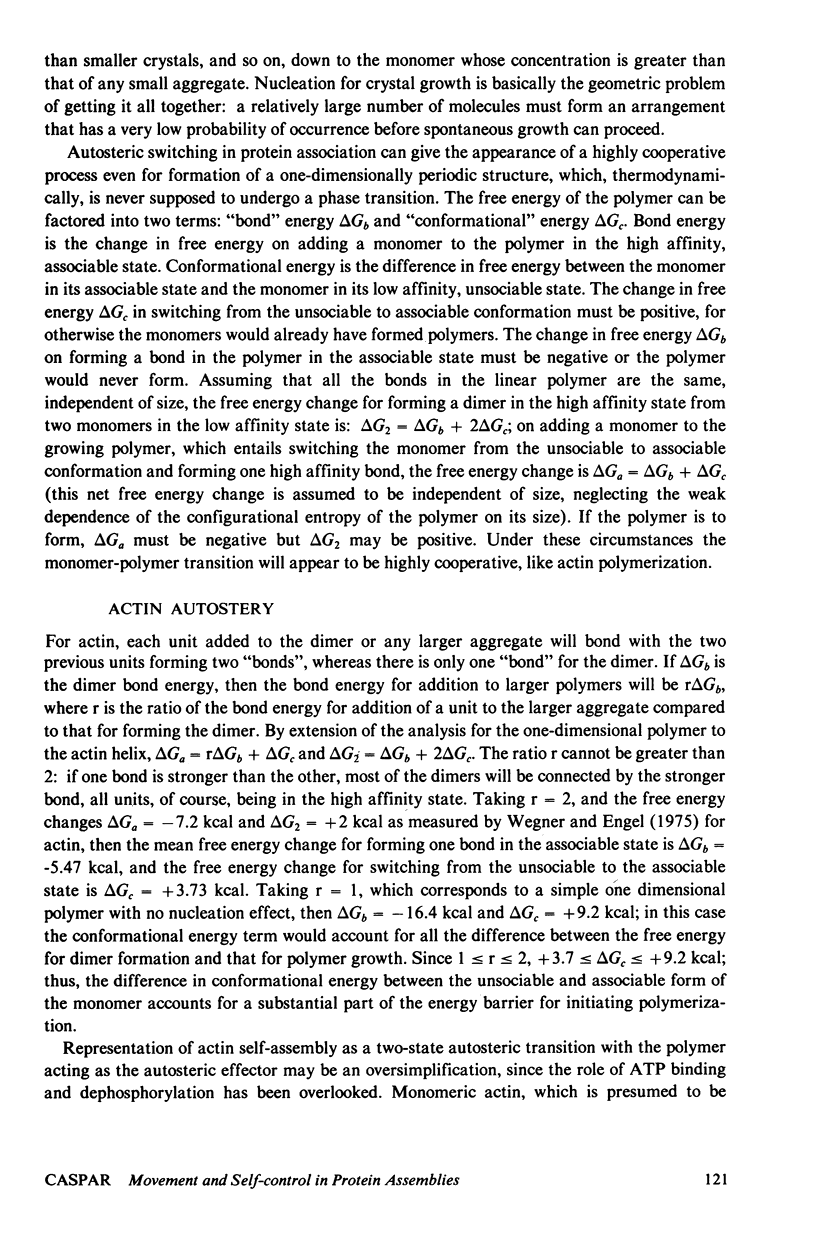
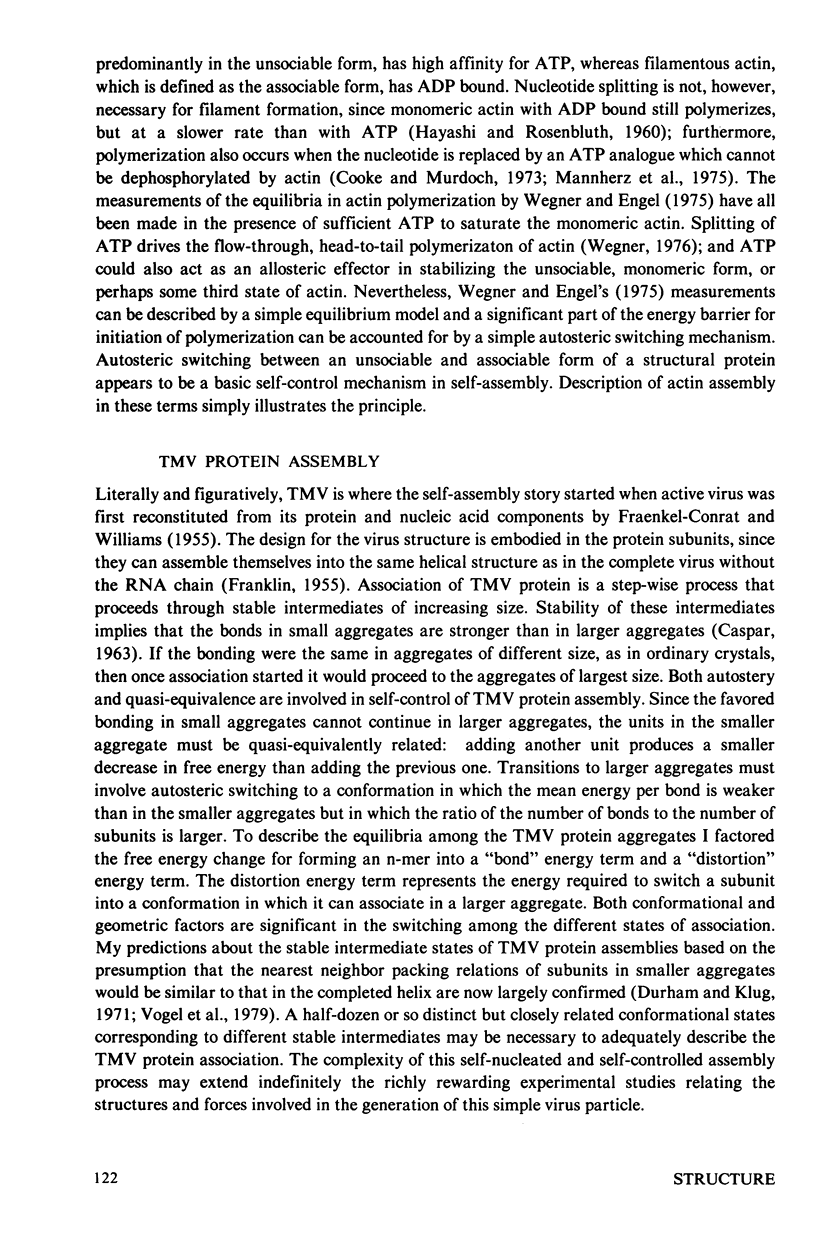
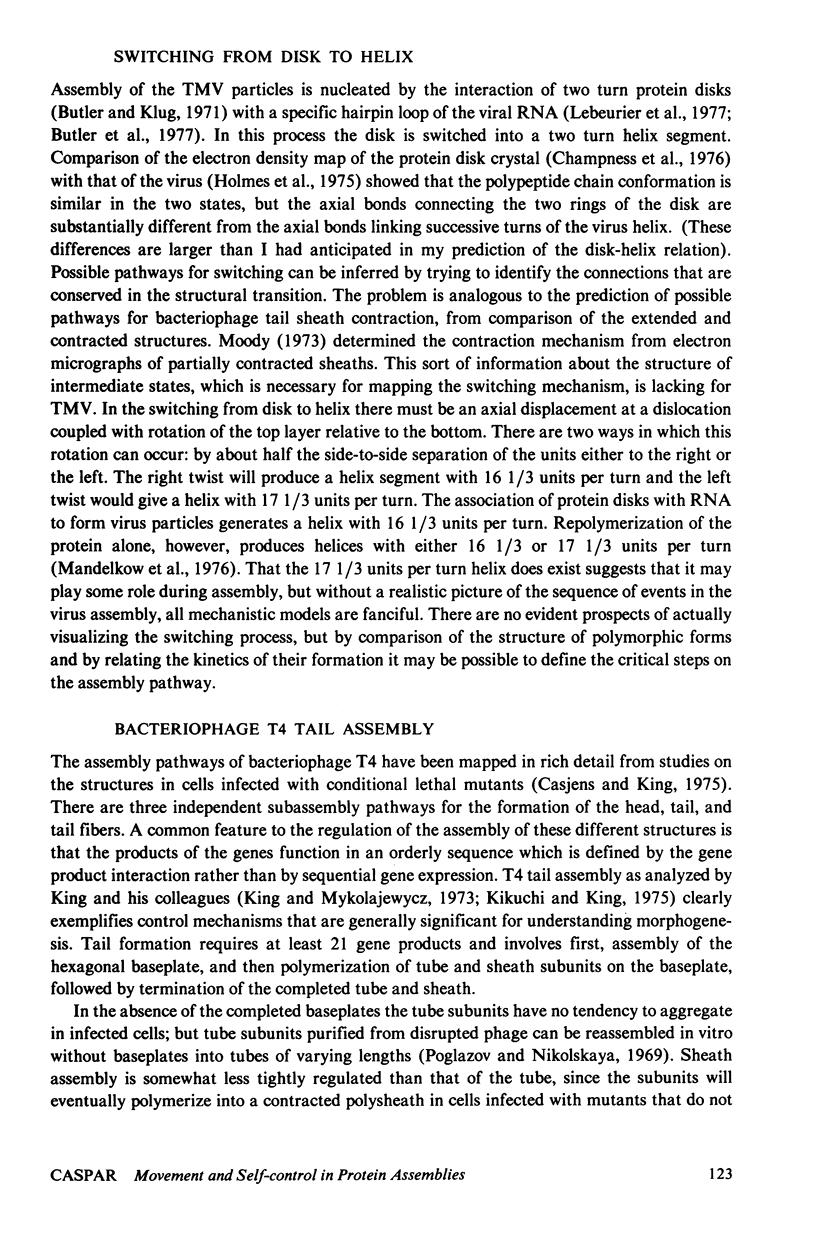
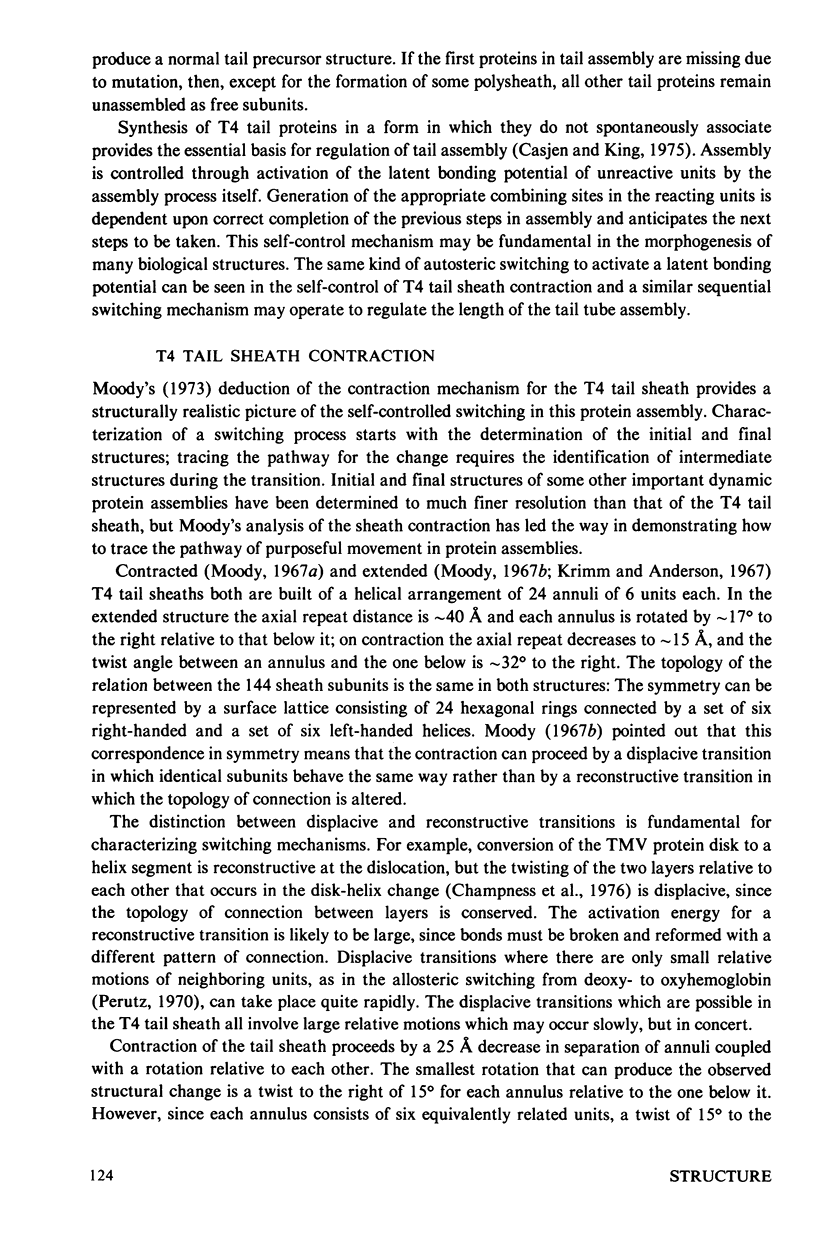
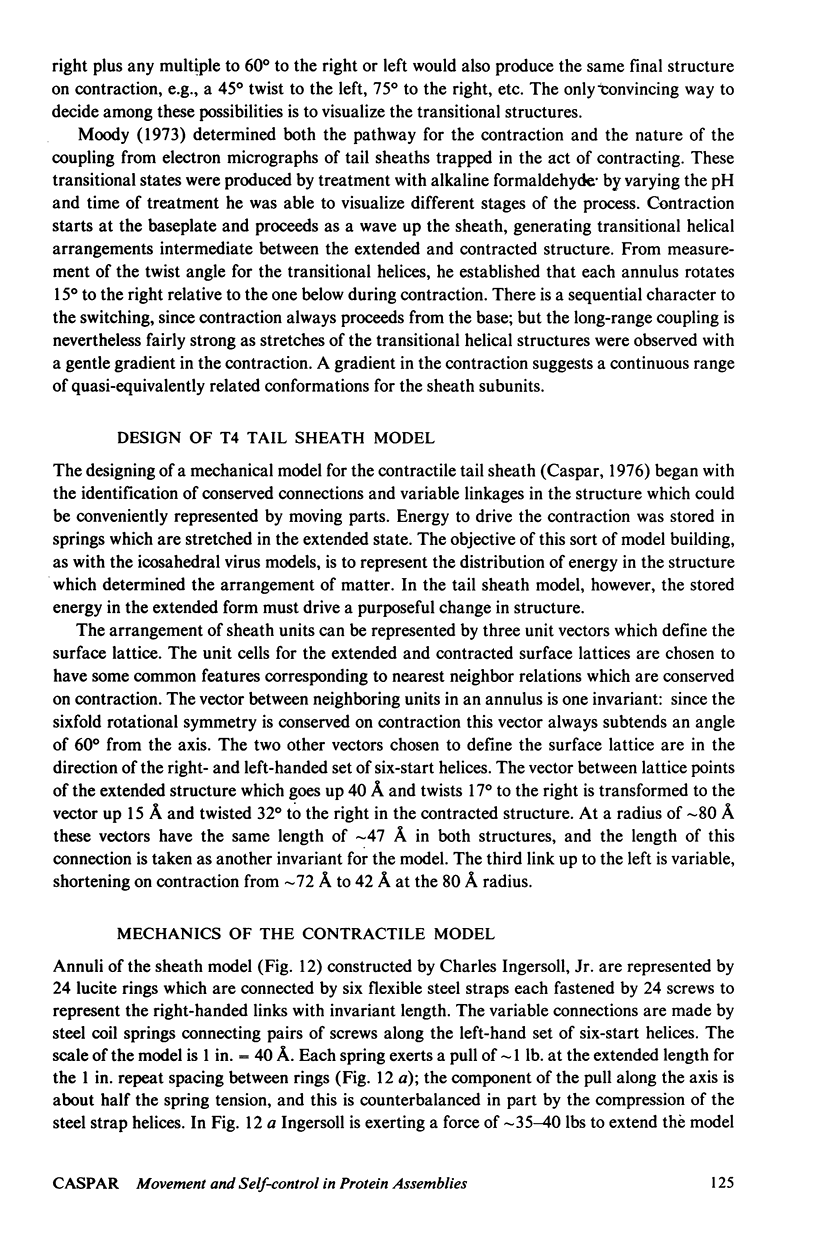
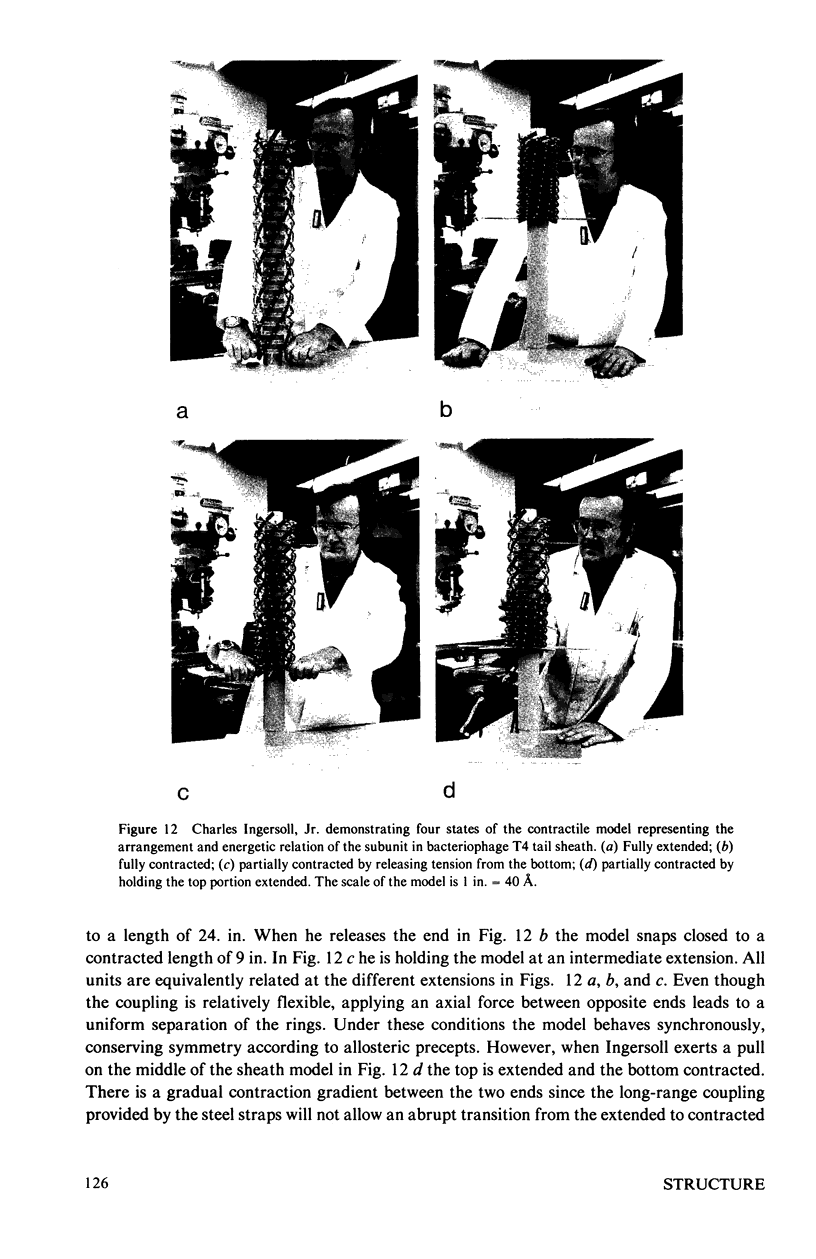
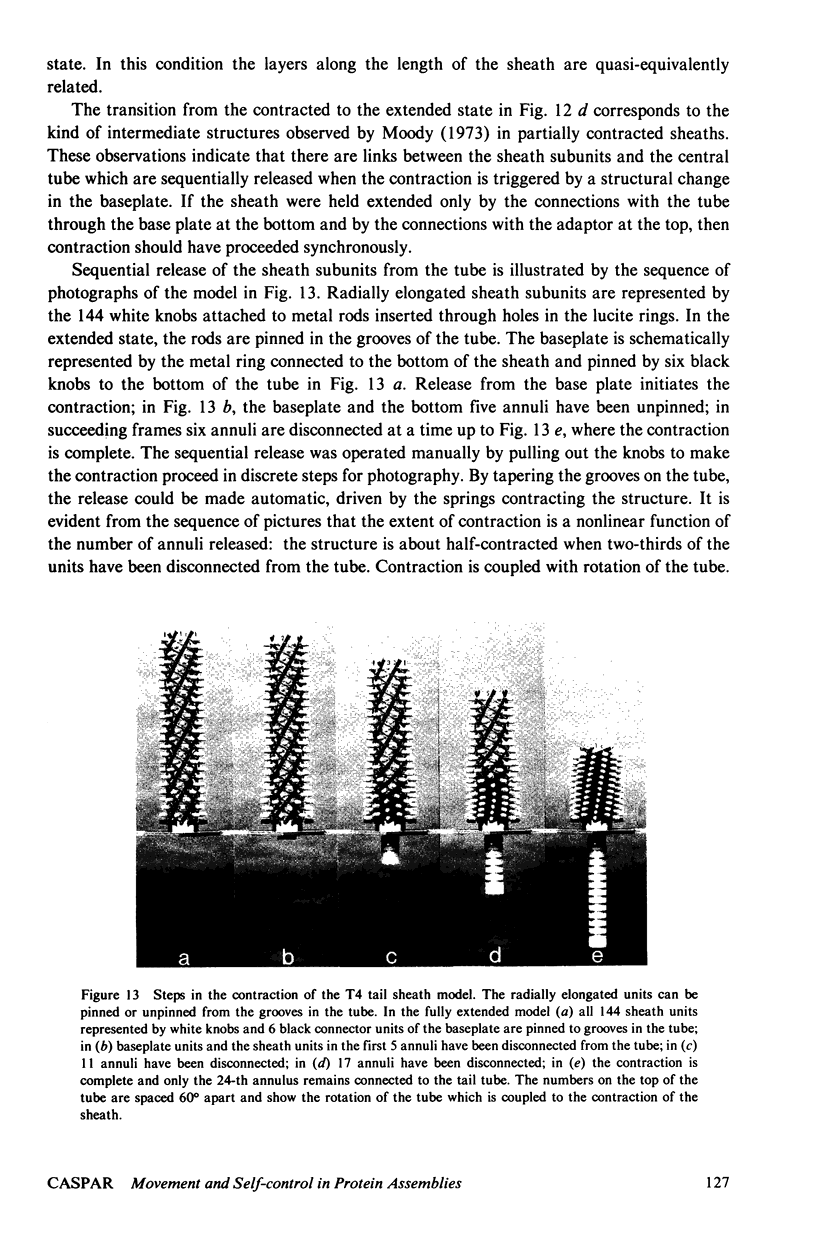
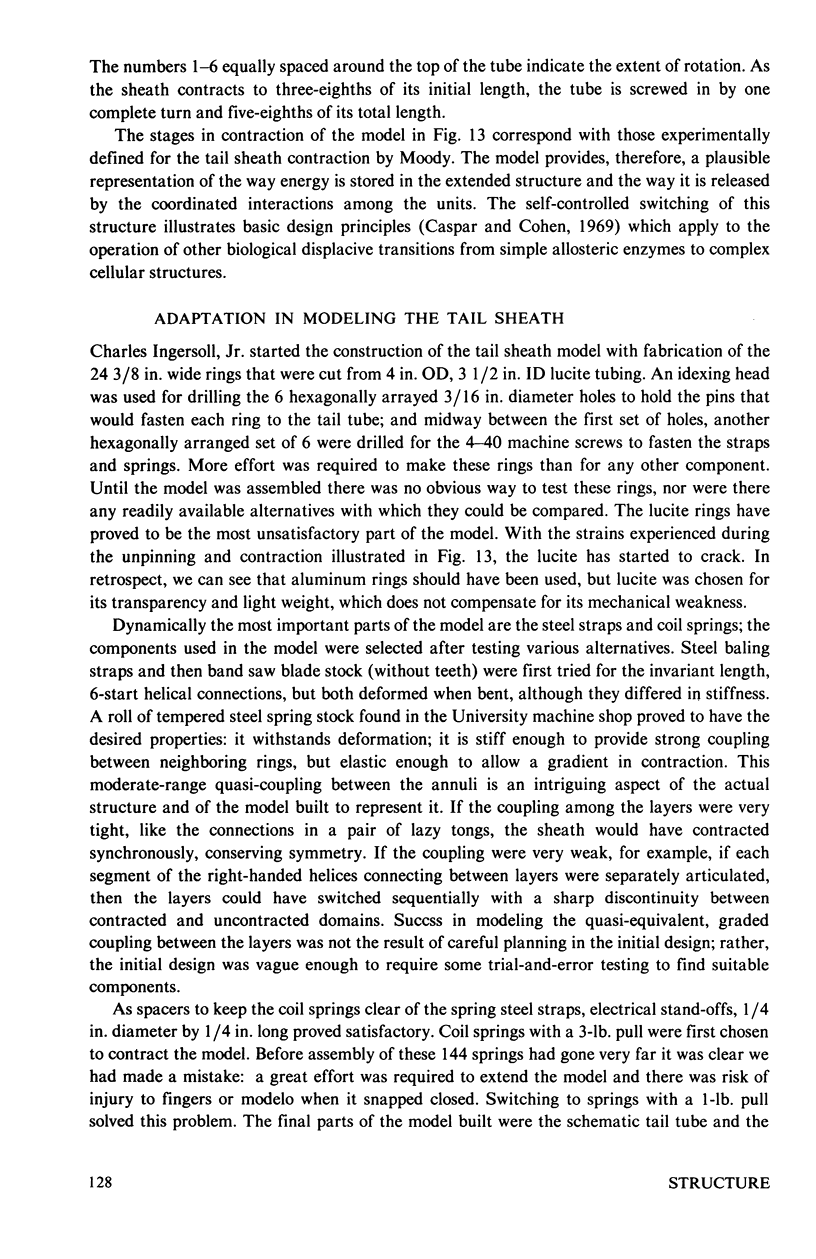
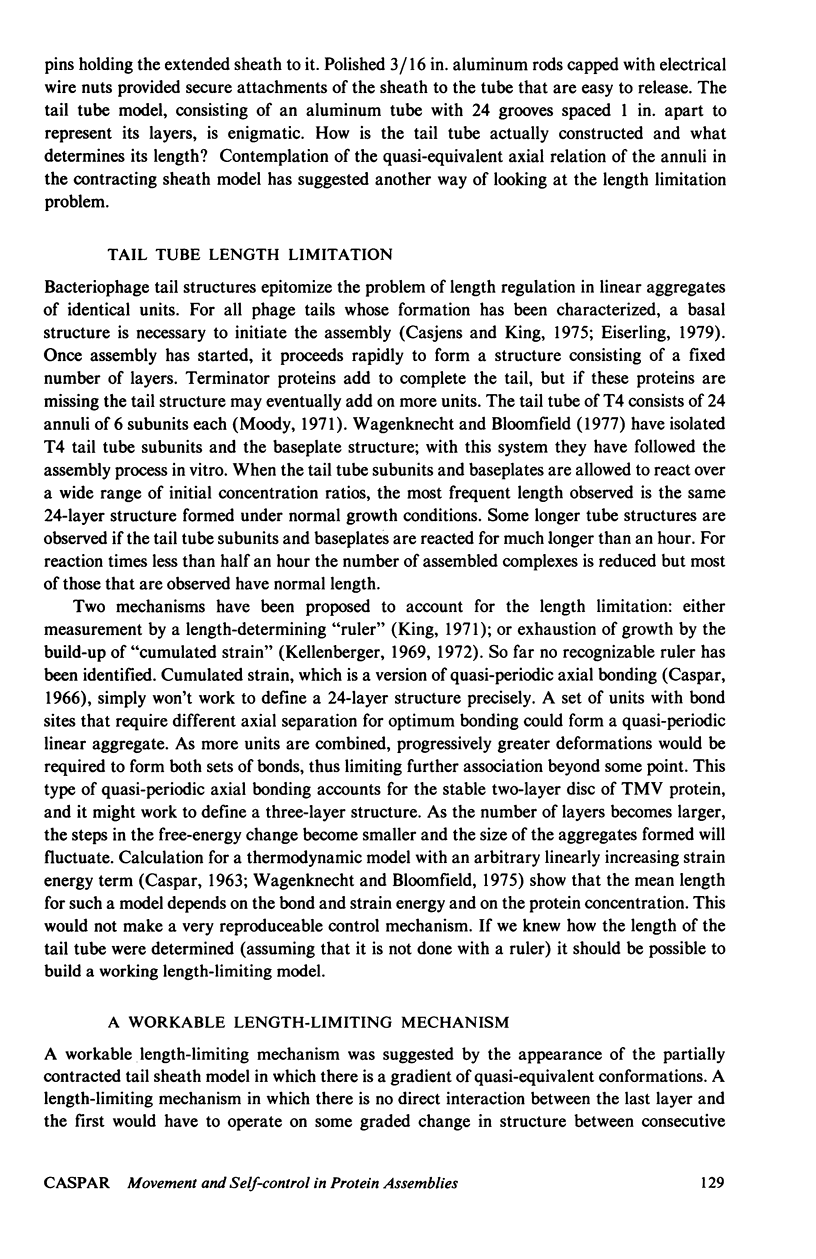
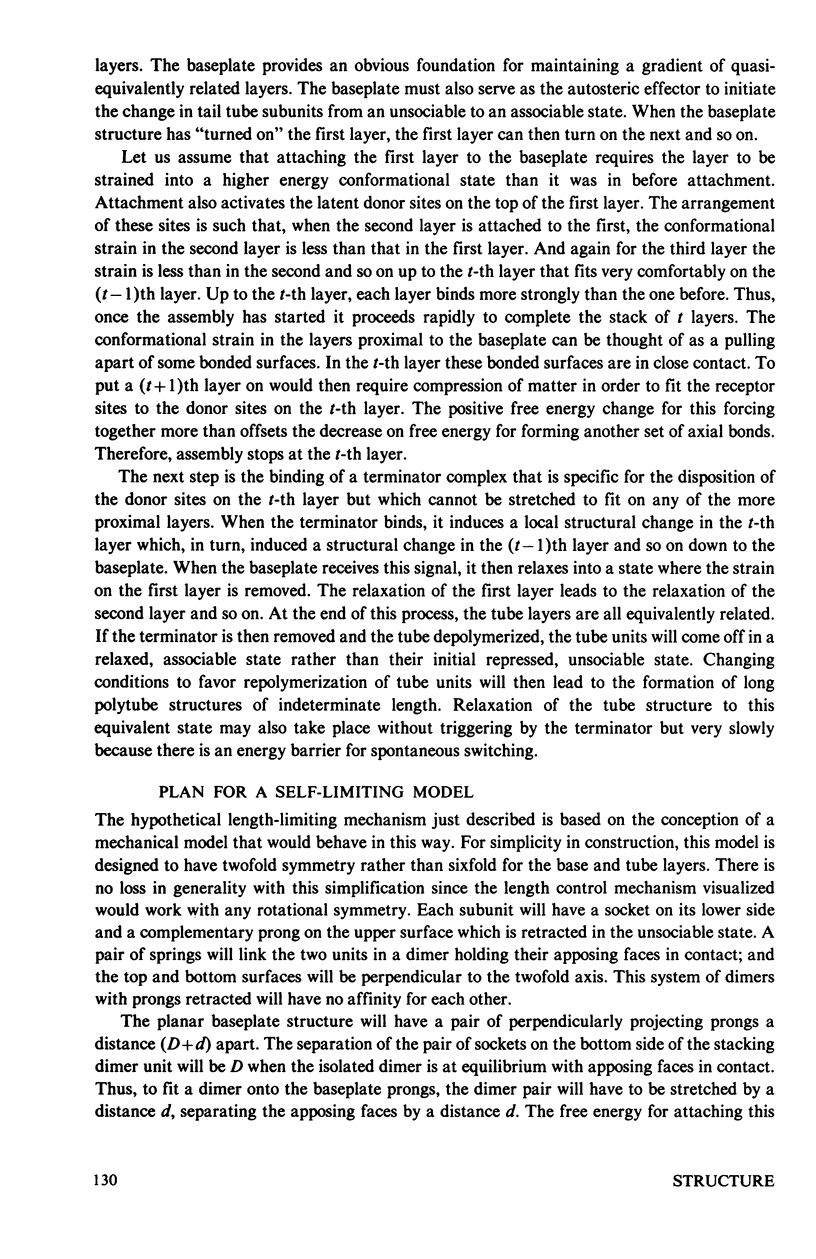
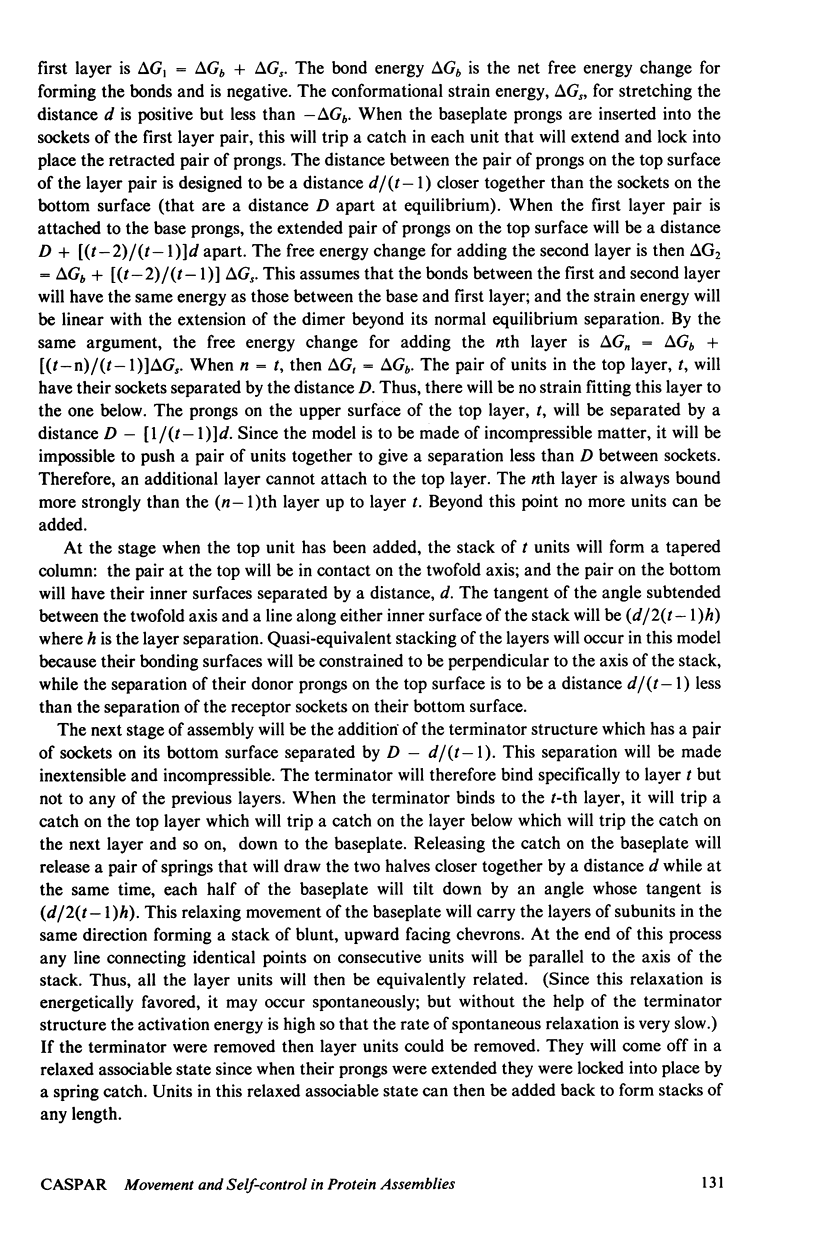
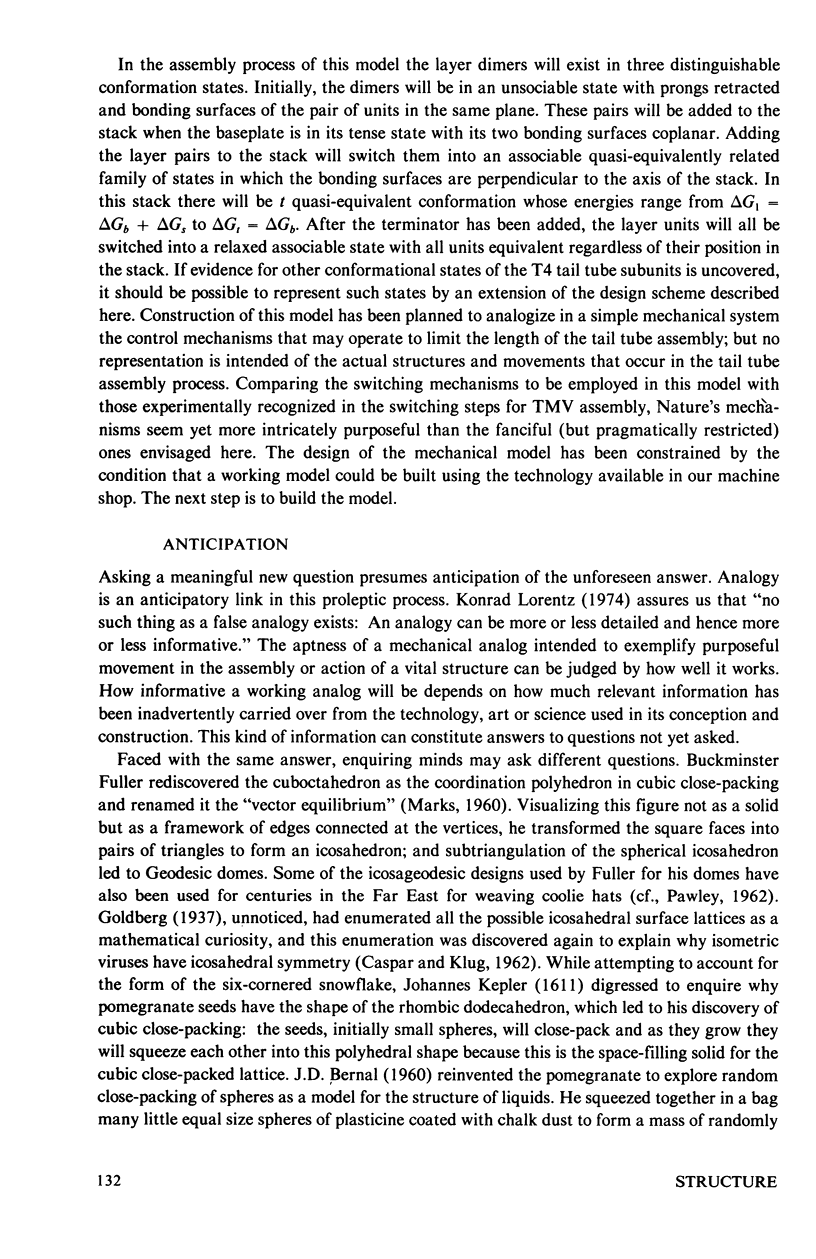
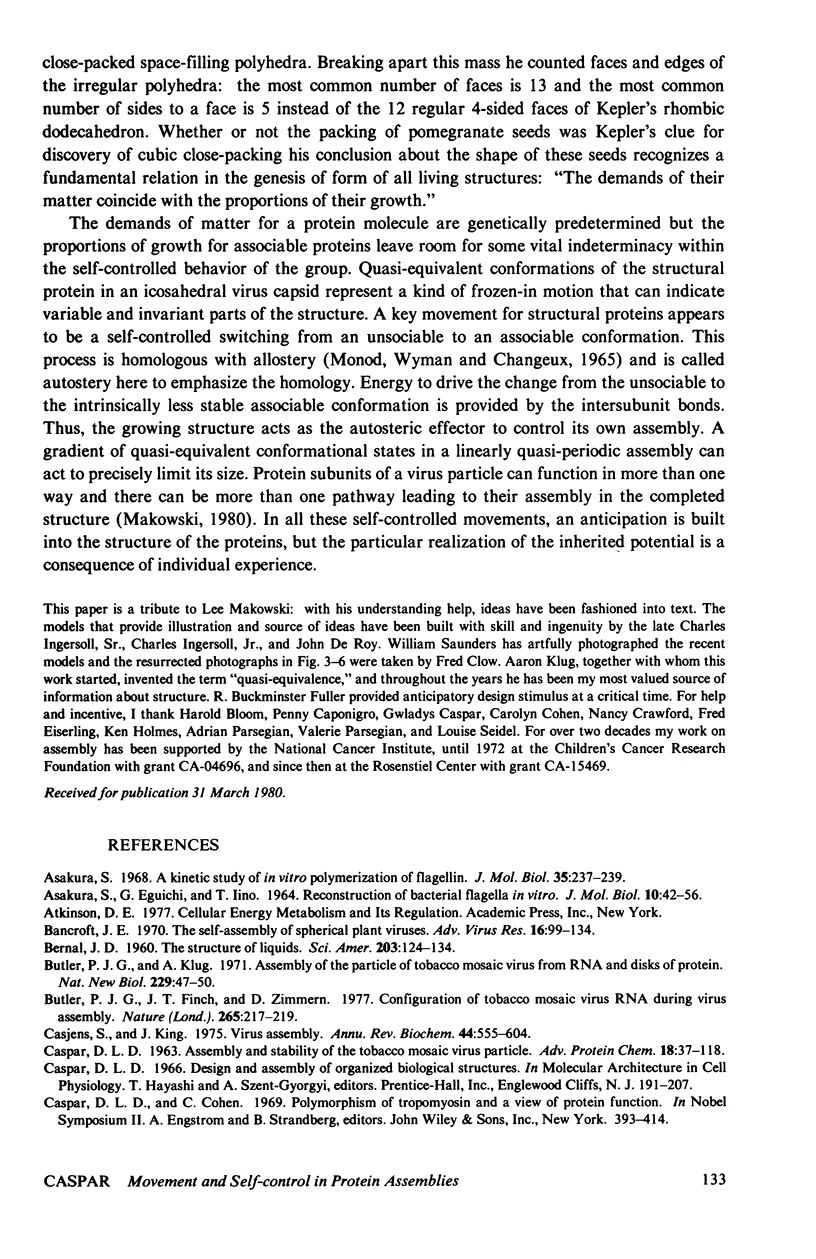
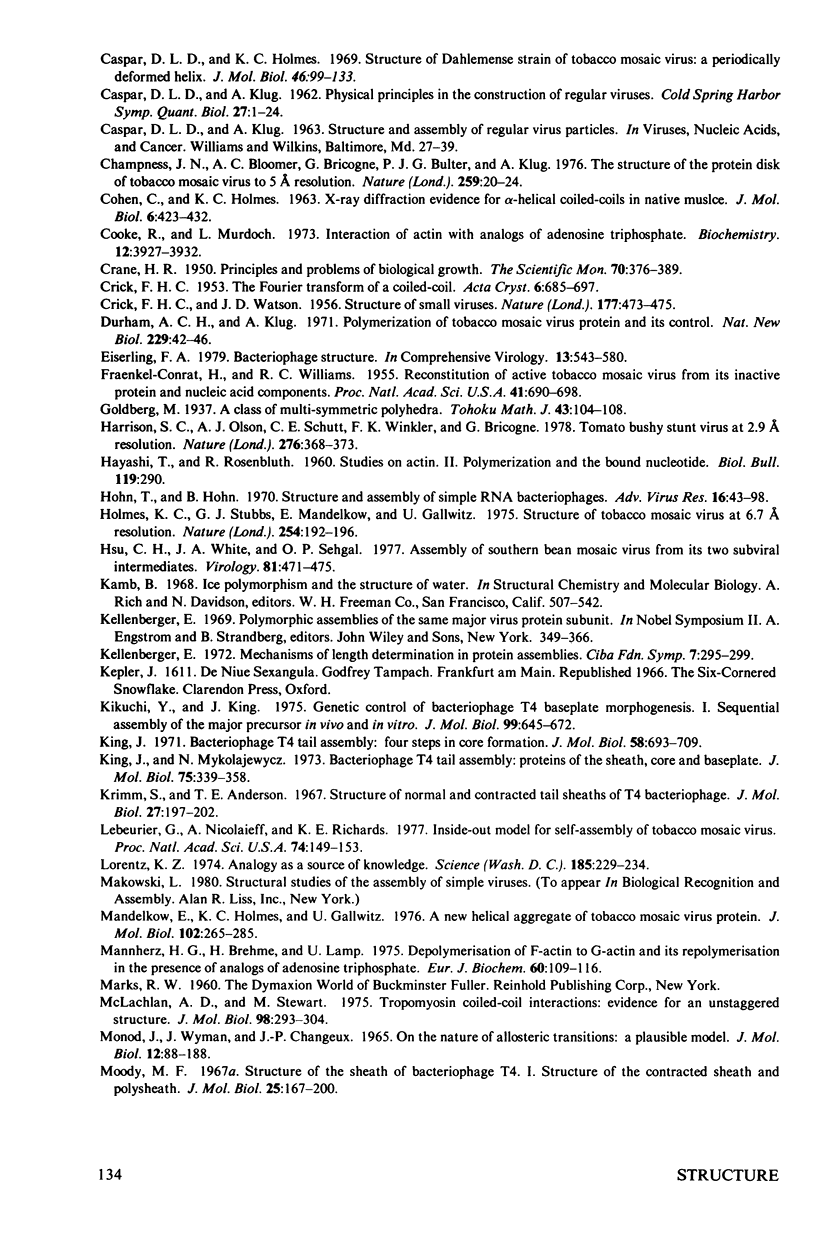
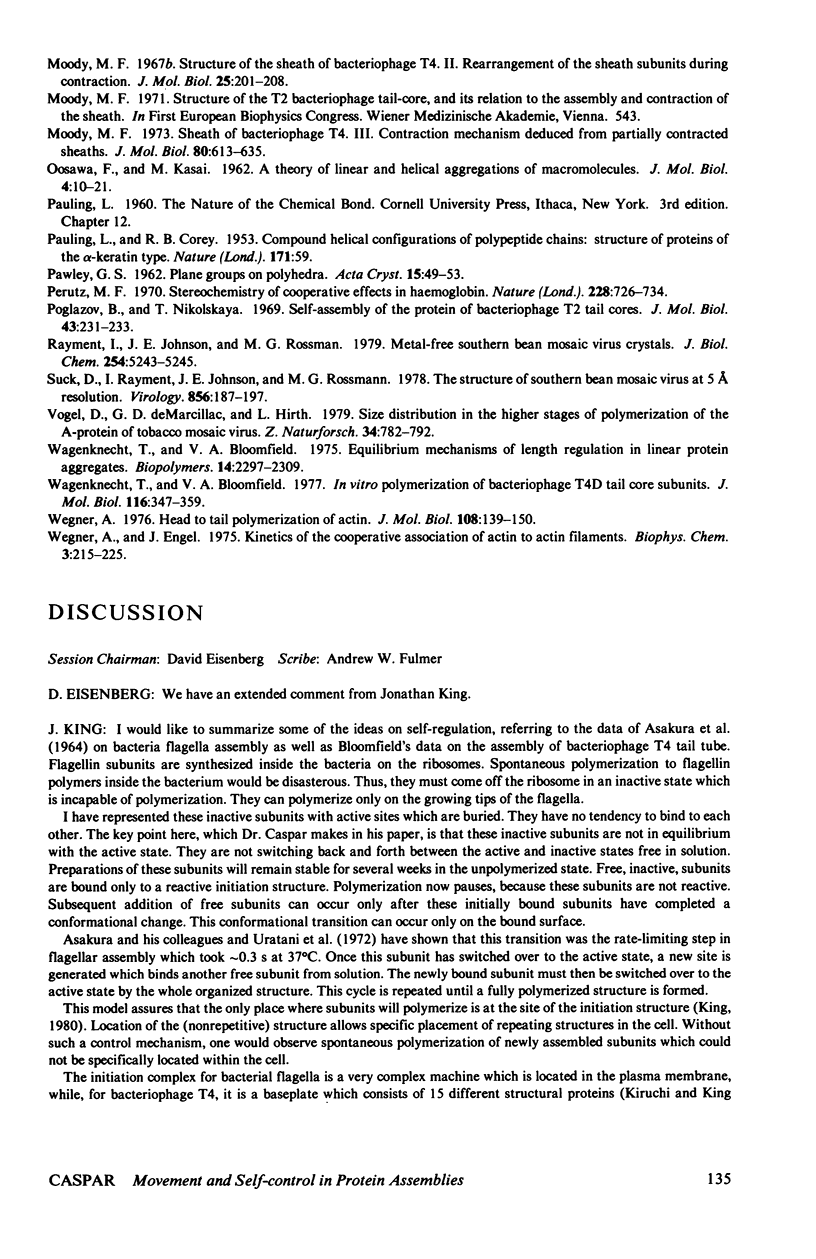
Images in this article
Selected References
These references are in PubMed. This may not be the complete list of references from this article.
- ASAKURA S., EGUCHI G., IINO T. RECONSTITUTION OF BACTERIAL FLAGELLA IN VITRO. J Mol Biol. 1964 Oct;10:42–56. doi: 10.1016/s0022-2836(64)80026-7. [DOI] [PubMed] [Google Scholar]
- Asakura S. A kinetic study of in vitro polymerization of flagellin. J Mol Biol. 1968 Jul 14;35(1):237–239. doi: 10.1016/s0022-2836(68)80051-8. [DOI] [PubMed] [Google Scholar]
- Bancroft J. B. The self-assembly of spherical plant viruses. Adv Virus Res. 1970;16:99–134. doi: 10.1016/s0065-3527(08)60022-6. [DOI] [PubMed] [Google Scholar]
- Butler P. J., Finch J. T., Zimmern D. Configuration of tobacco mosaic virus, RNA during virus assembly. Nature. 1977 Jan 20;265(5591):217–219. doi: 10.1038/265217a0. [DOI] [PubMed] [Google Scholar]
- Butler P. J., Klug A. Assembly of the particle of tobacco mosaic virus from RNA and disks of protein. Nat New Biol. 1971 Jan 13;229(2):47–50. doi: 10.1038/newbio229047a0. [DOI] [PubMed] [Google Scholar]
- CASPAR D. L. ASSEMBLY AND STABILITY OF THE TOBACCO MOSAIC VIRUS PARTICLE. Adv Protein Chem. 1963;18:37–121. doi: 10.1016/s0065-3233(08)60268-5. [DOI] [PubMed] [Google Scholar]
- CASPAR D. L., KLUG A. Physical principles in the construction of regular viruses. Cold Spring Harb Symp Quant Biol. 1962;27:1–24. doi: 10.1101/sqb.1962.027.001.005. [DOI] [PubMed] [Google Scholar]
- COHEN C., HOLMES K. C. X-ray diffraction evidence for alpha-helical coiled-coils in native muscle. J Mol Biol. 1963 May;6:423–432. doi: 10.1016/s0022-2836(63)80053-4. [DOI] [PubMed] [Google Scholar]
- CRICK F. H., WATSON J. D. Structure of small viruses. Nature. 1956 Mar 10;177(4506):473–475. doi: 10.1038/177473a0. [DOI] [PubMed] [Google Scholar]
- Casjens S., King J. Virus assembly. Annu Rev Biochem. 1975;44:555–611. doi: 10.1146/annurev.bi.44.070175.003011. [DOI] [PubMed] [Google Scholar]
- Caspar D. L., Holmes K. C. Structure of dahlemense strain of tobacco mosaic virus: a periodically deformed helix. J Mol Biol. 1969 Nov 28;46(1):99–133. doi: 10.1016/0022-2836(69)90060-6. [DOI] [PubMed] [Google Scholar]
- Champness J. N., Bloomer A. C., Bricogne G., Butler P. G., Klug A. The structure of the protein disk of tobacco mosaic virus to 5A resolution. Nature. 1976 Jan 1;259(5538):20–24. doi: 10.1038/259020a0. [DOI] [PubMed] [Google Scholar]
- Cooke R., Murdoch L. Interaction of actin with analogs of adenosine triphosphate. Biochemistry. 1973 Sep 25;12(20):3927–3932. doi: 10.1021/bi00744a022. [DOI] [PubMed] [Google Scholar]
- Durham A. C., Klug A. Polymerization of tobacco mosaic virus protein and its control. Nat New Biol. 1971 Jan 13;229(2):42–46. doi: 10.1038/newbio229042a0. [DOI] [PubMed] [Google Scholar]
- Fraenkel-Conrat H., Williams R. C. RECONSTITUTION OF ACTIVE TOBACCO MOSAIC VIRUS FROM ITS INACTIVE PROTEIN AND NUCLEIC ACID COMPONENTS. Proc Natl Acad Sci U S A. 1955 Oct 15;41(10):690–698. doi: 10.1073/pnas.41.10.690. [DOI] [PMC free article] [PubMed] [Google Scholar]
- Hohn T., Hohn B. Structure and assembly of simple RNA bacteriophages. Adv Virus Res. 1970;16:43–98. doi: 10.1016/s0065-3527(08)60021-4. [DOI] [PubMed] [Google Scholar]
- Holmes K. C., Stubbs G. J., Mandelkow E., Gallwitz U. Structure of tobacco mosaic virus at 6.7 å resolution. Nature. 1975 Mar 20;254(5497):192–196. doi: 10.1038/254192a0. [DOI] [PubMed] [Google Scholar]
- Hsu C. H., White J. A., Sehgal O. P. Assembly of southern bean mosaic virus from its two subviral intermediates. Virology. 1977 Sep;81(2):471–475. doi: 10.1016/0042-6822(77)90162-3. [DOI] [PubMed] [Google Scholar]
- Kikuchi Y., King J. Genetic control of bacteriophage T4 baseplate morphogenesis. I. Sequential assembly of the major precursor, in vivo and in vitro. J Mol Biol. 1975 Dec 25;99(4):645–672. doi: 10.1016/s0022-2836(75)80178-1. [DOI] [PubMed] [Google Scholar]
- King J. Bacteriophage T4 tail assembly: four steps in core formation. J Mol Biol. 1971 Jun 28;58(3):693–709. doi: 10.1016/0022-2836(71)90034-9. [DOI] [PubMed] [Google Scholar]
- King J., Mykolajewycz N. Bacteriophage T4 tail assembly: proteins of the sheath, core and baseplate. J Mol Biol. 1973 Apr 5;75(2):339–358. doi: 10.1016/0022-2836(73)90025-9. [DOI] [PubMed] [Google Scholar]
- Krimm S., Anderson T. F. Structure of normal and contracted tail sheaths of T4 bacteriophage. J Mol Biol. 1967 Jul 28;27(2):197–202. doi: 10.1016/0022-2836(67)90015-0. [DOI] [PubMed] [Google Scholar]
- Lebeurier G., Nicolaieff A., Richards K. E. Inside-out model for self-assembly of tobacco mosaic virus. Proc Natl Acad Sci U S A. 1977 Jan;74(1):149–153. doi: 10.1073/pnas.74.1.149. [DOI] [PMC free article] [PubMed] [Google Scholar]
- MONOD J., WYMAN J., CHANGEUX J. P. ON THE NATURE OF ALLOSTERIC TRANSITIONS: A PLAUSIBLE MODEL. J Mol Biol. 1965 May;12:88–118. doi: 10.1016/s0022-2836(65)80285-6. [DOI] [PubMed] [Google Scholar]
- Mandelkow E., Holmes K. C., Gallwitz U. A new helical aggregate of tobacco mosaic virus protein. J Mol Biol. 1976 Apr 5;102(2):265–285. doi: 10.1016/s0022-2836(76)80053-8. [DOI] [PubMed] [Google Scholar]
- Mannherz H. G., Brehme H., Lamp U. Depolymerisation of F-actin to G-actin and its repolymerisation in the presence of analogs of adenosine triphosphate. Eur J Biochem. 1975 Dec 1;60(1):109–116. doi: 10.1111/j.1432-1033.1975.tb20981.x. [DOI] [PubMed] [Google Scholar]
- McLachlan A. D., Stewart M. Tropomyosin coiled-coil interactions: evidence for an unstaggered structure. J Mol Biol. 1975 Oct 25;98(2):293–304. doi: 10.1016/s0022-2836(75)80119-7. [DOI] [PubMed] [Google Scholar]
- Moody M. F. Sheath of bacteriophage T4. 3. Contraction mechanism deduced from partially contracted sheaths. J Mol Biol. 1973 Nov 15;80(4):613–635. doi: 10.1016/0022-2836(73)90200-3. [DOI] [PubMed] [Google Scholar]
- Moody M. F. Structure of the sheath of bacteriophage T4. I. Structure of the contracted sheath and polysheath. J Mol Biol. 1967 Apr 28;25(2):167–200. doi: 10.1016/0022-2836(67)90136-2. [DOI] [PubMed] [Google Scholar]
- Moody M. F. Structure of the sheath of bacteriophage T4. II. Rearrangement of the sheath subunits during contraction. J Mol Biol. 1967 Apr 28;25(2):201–208. doi: 10.1016/0022-2836(67)90137-4. [DOI] [PubMed] [Google Scholar]
- OOSAWA F., KASAI M. A theory of linear and helical aggregations of macromolecules. J Mol Biol. 1962 Jan;4:10–21. doi: 10.1016/s0022-2836(62)80112-0. [DOI] [PubMed] [Google Scholar]
- Perutz M. F. Stereochemistry of cooperative effects in haemoglobin. Nature. 1970 Nov 21;228(5273):726–739. doi: 10.1038/228726a0. [DOI] [PubMed] [Google Scholar]
- Poglazov B. F., Nikolskaya T. I. Self-assembly of the protein of bacteriophage T2 tail cores. J Mol Biol. 1969 Jul 14;43(1):231–233. doi: 10.1016/0022-2836(69)90094-1. [DOI] [PubMed] [Google Scholar]
- Rayment I., Johnson J. E., Rossmann M. G. Metal-free southern bean mosaic virus crystals. J Biol Chem. 1979 Jun 25;254(12):5243–5245. [PubMed] [Google Scholar]
- Suck D., Rayment I., Johnson J. E., Rossmann M. G. The structure of southern bean mosaic virus at 5 A resolution. Virology. 1978 Mar;85(1):187–197. doi: 10.1016/0042-6822(78)90423-3. [DOI] [PubMed] [Google Scholar]
- Wagenknecht T., Bloomfield V. A. In vitro polymerization of bacteriophage T4D tail core subunits. J Mol Biol. 1977 Nov 5;116(3):347–359. doi: 10.1016/0022-2836(77)90074-2. [DOI] [PubMed] [Google Scholar]
- Wegner A., Engel J. Kinetics of the cooperative association of actin to actin filaments. Biophys Chem. 1975 Jul;3(3):215–225. doi: 10.1016/0301-4622(75)80013-5. [DOI] [PubMed] [Google Scholar]
- Wegner A. Head to tail polymerization of actin. J Mol Biol. 1976 Nov;108(1):139–150. doi: 10.1016/s0022-2836(76)80100-3. [DOI] [PubMed] [Google Scholar]