Abstract
Steady-state and lifetime-resolved fluorescence anisotropy measurements of protein fluorescence were used to investigate the depolarizing motions of tryptophan residues in proteins. Lifetime resolution was achieved by oxygen quenching. The proteins investigated were carbonic anhydrase, carboxypeptidase A, alpha-chymotrypsin, trypsin, pepsin, and bovine and human serum albumin. When corrected for overall protein rotation, the steady state anisotropies indicate that, on the average, the tryptophan residues in these proteins rotate 29 degrees +/- 6 degrees during the unquenched excited state lifetimes of these proteins, which range from 1.7 to 6.1 ns. The lifetime-resolved anisotropies reveal correlation times for these displacements ranging from 1 to 12 ns. On the average these correlation times are tenfold shorter than that expected for overall protein rotation. We conclude that the tryptophan residues in these proteins display remarkable freedom of motion within the protein matrix, which implies that these matrices are highly flexible on the nanosecond time scale.
Full text
PDF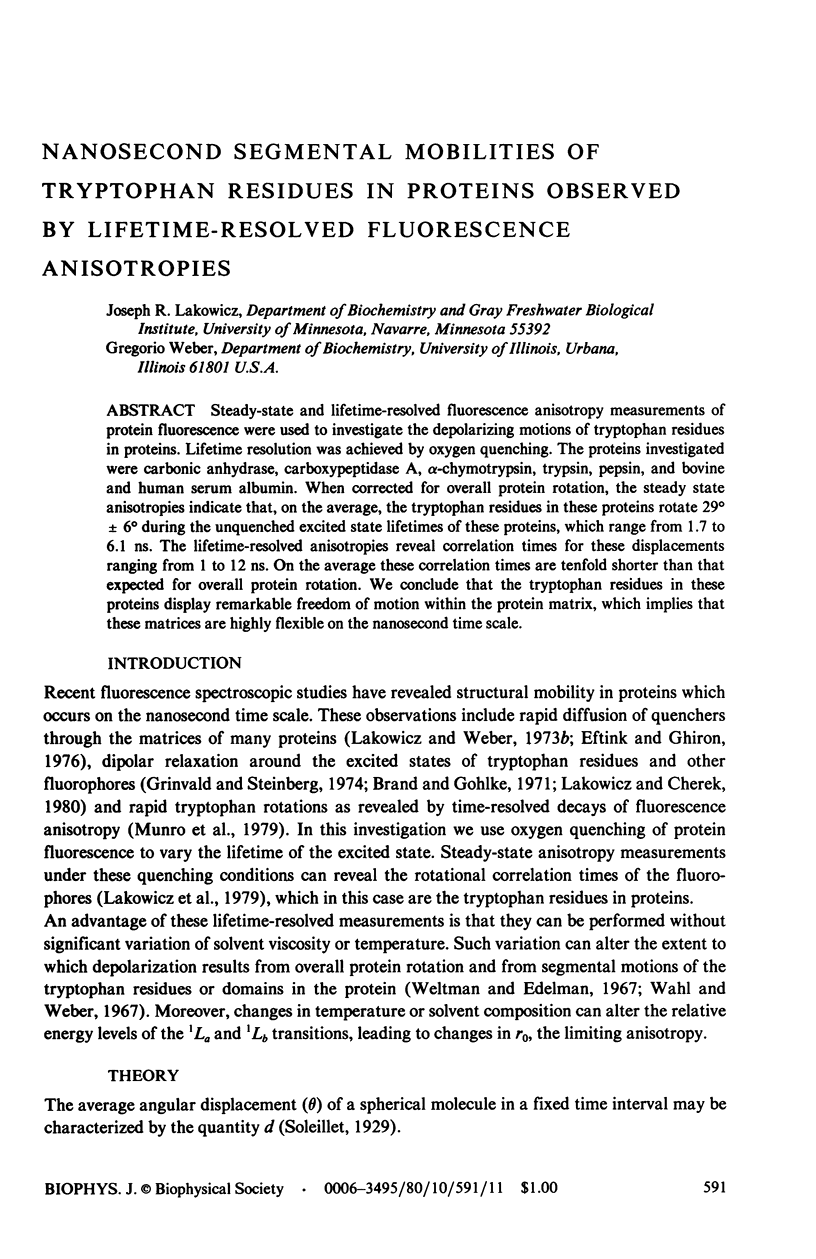
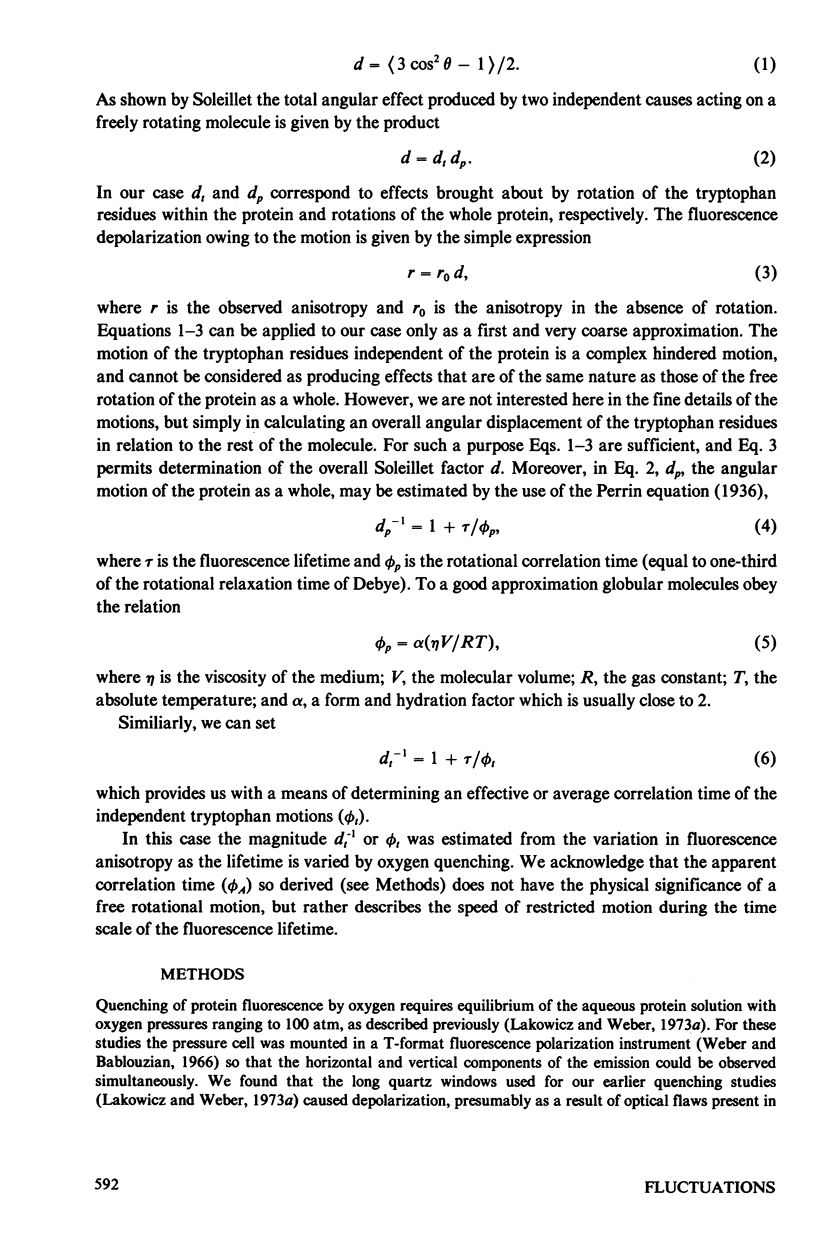
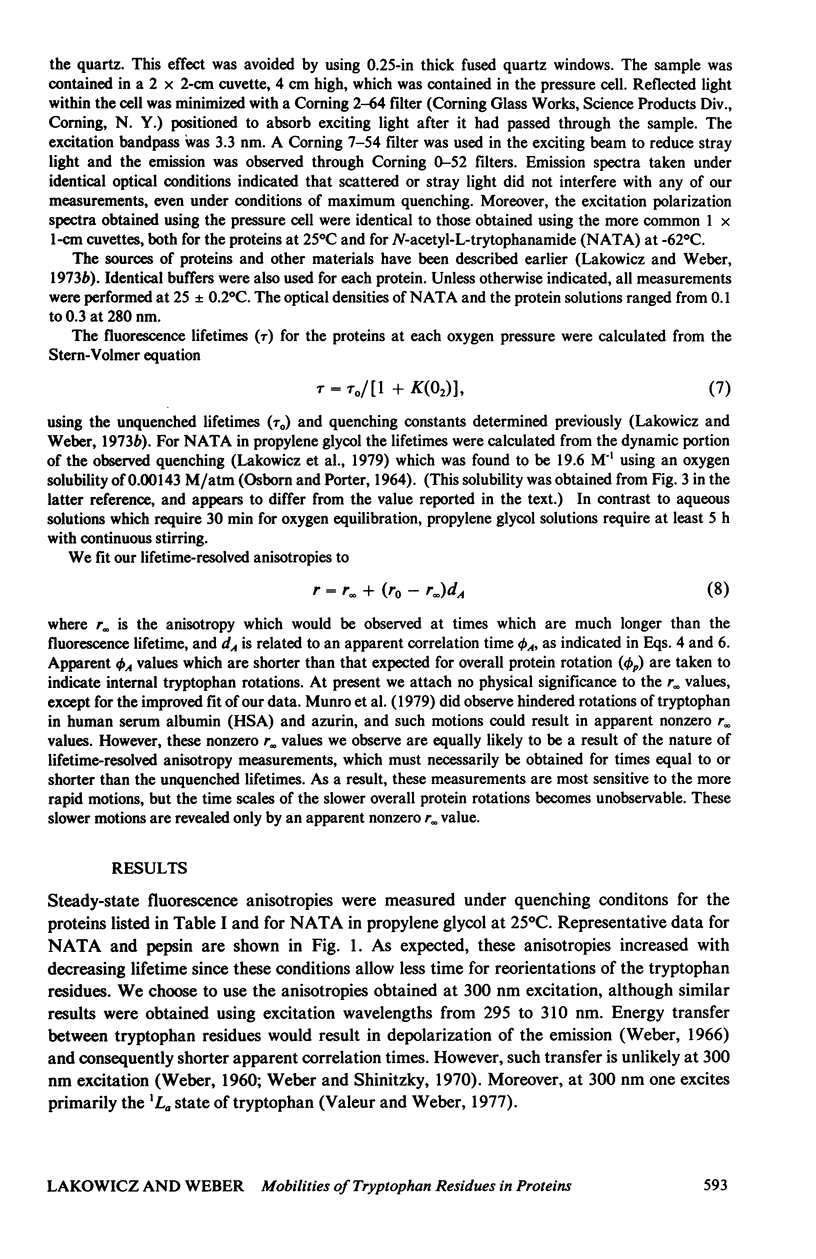
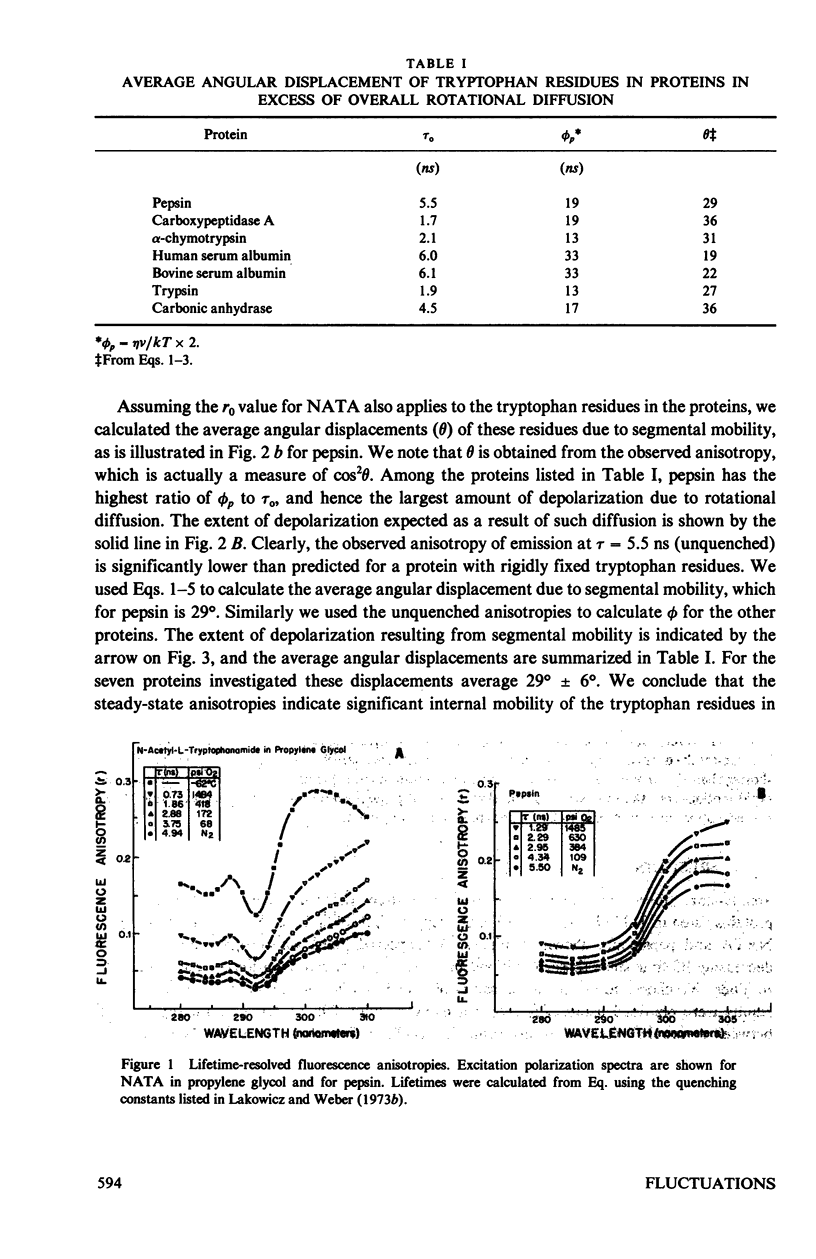
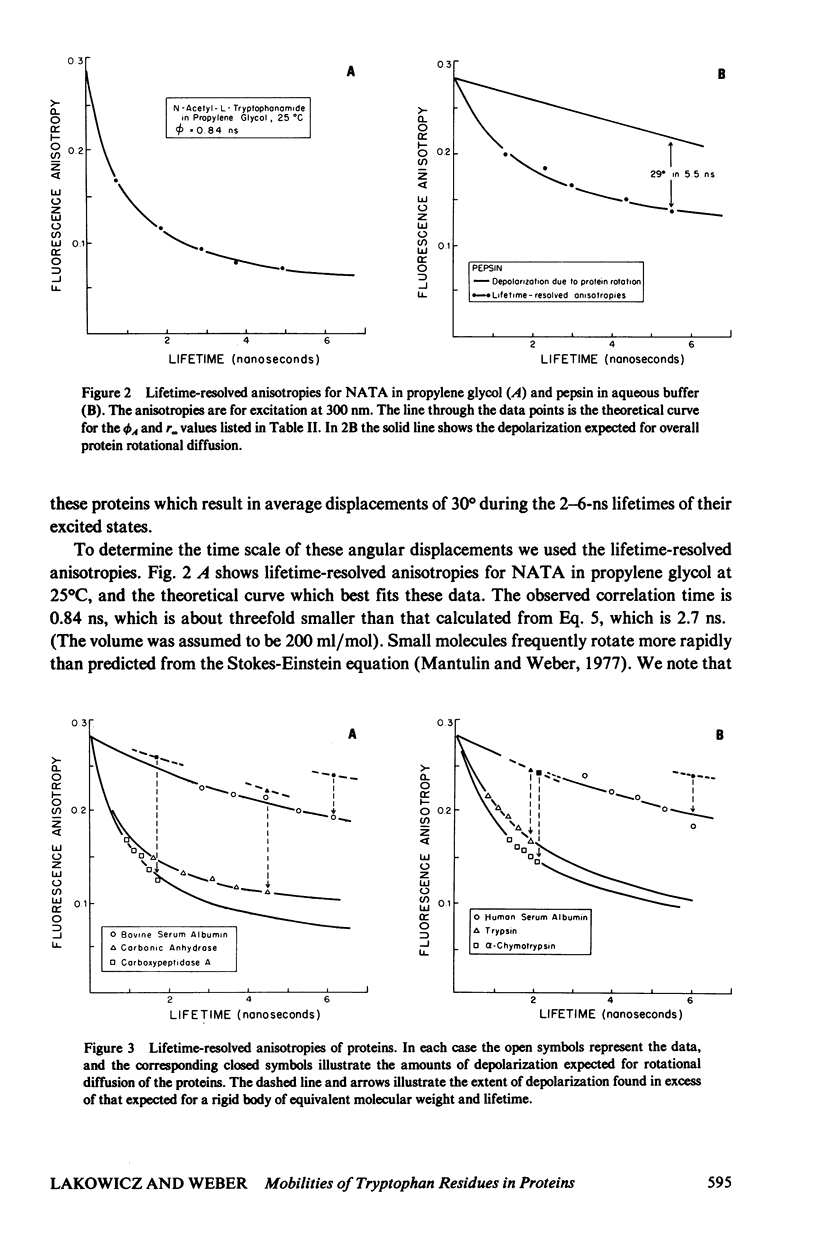
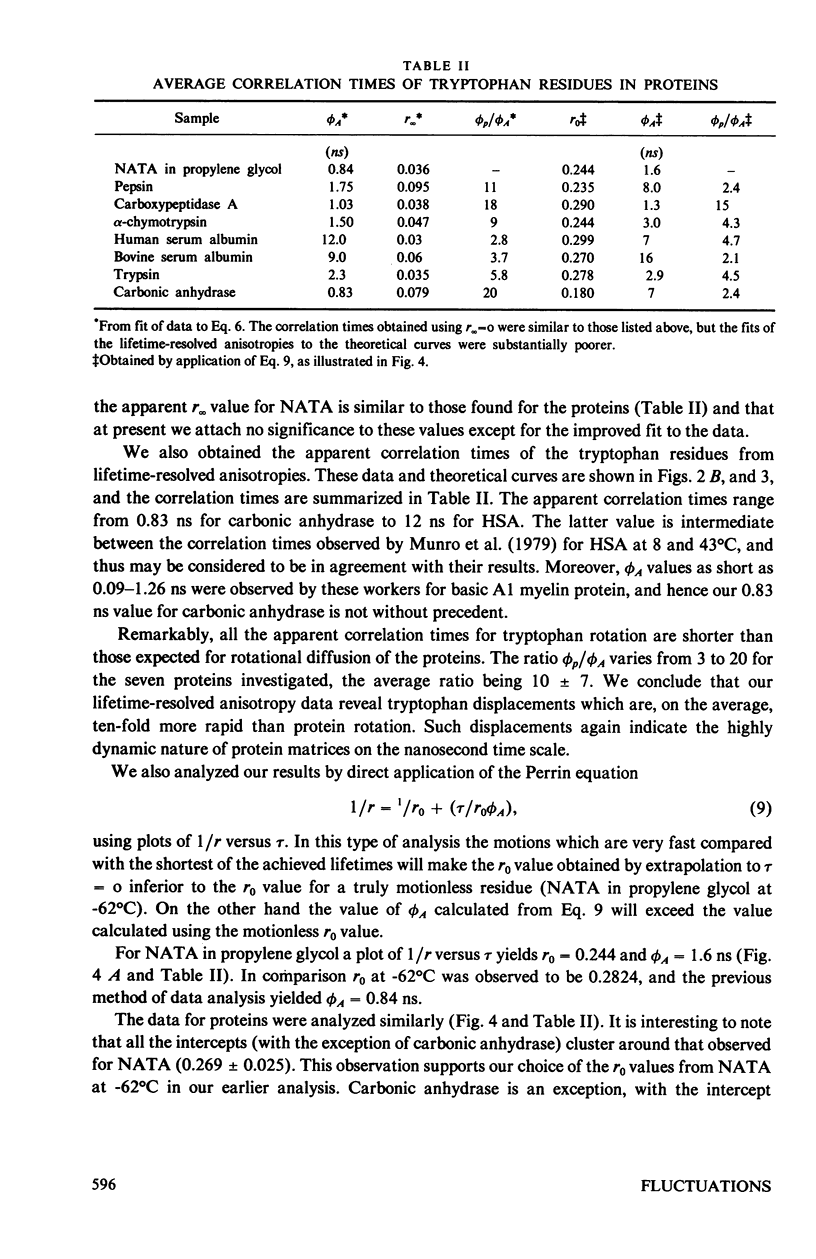
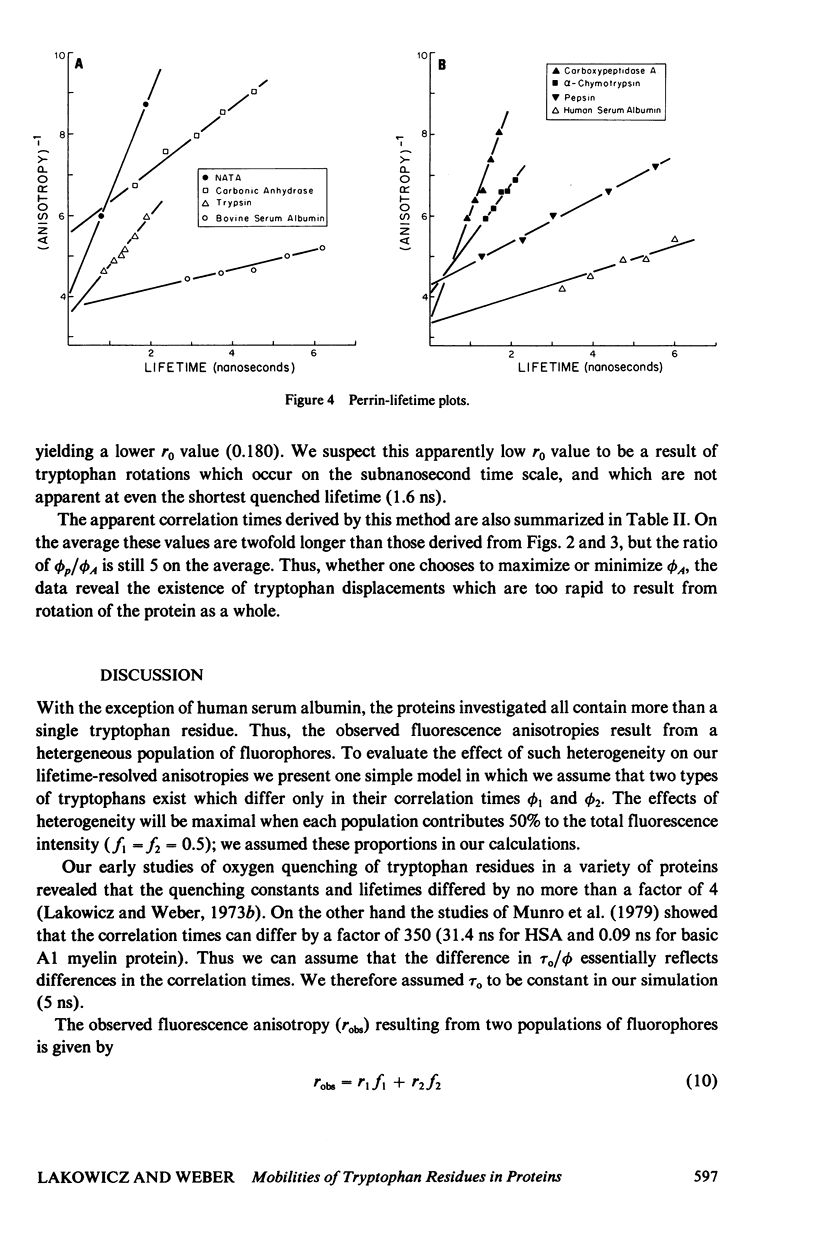
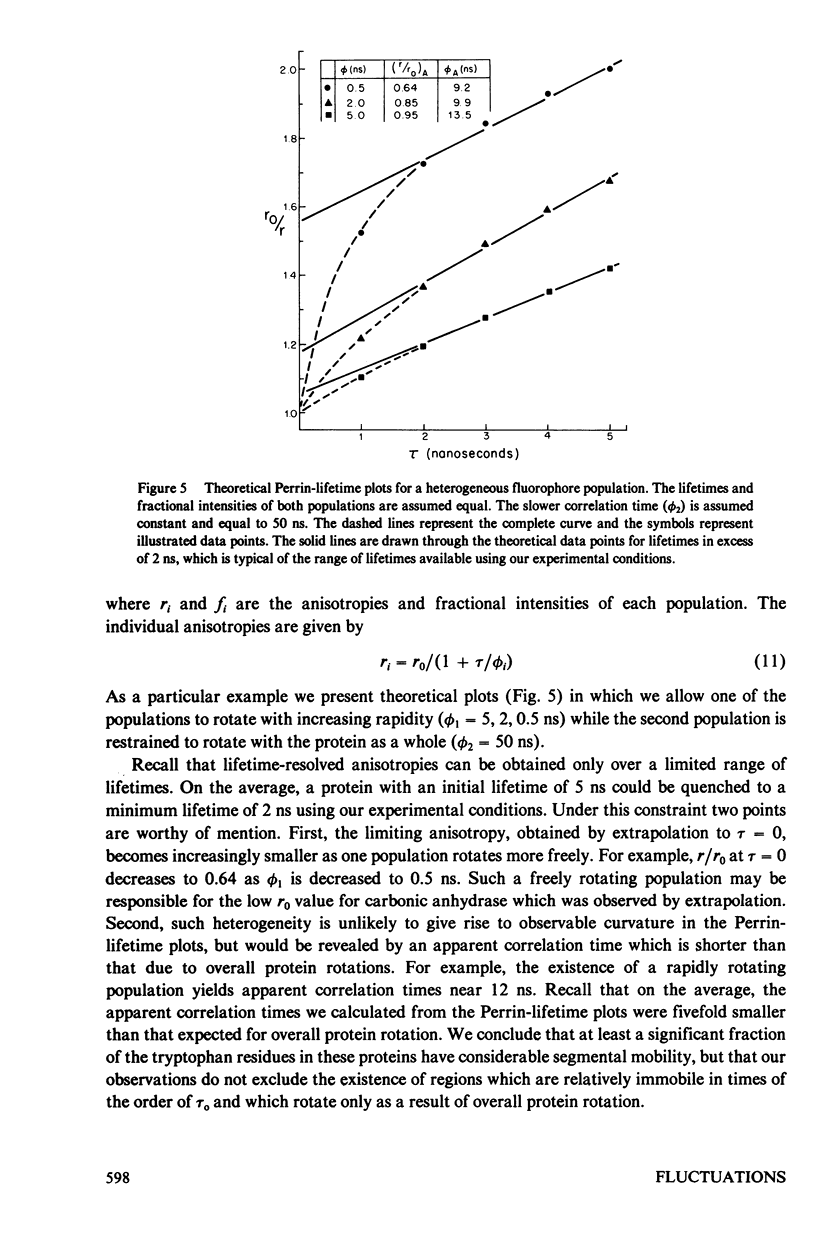
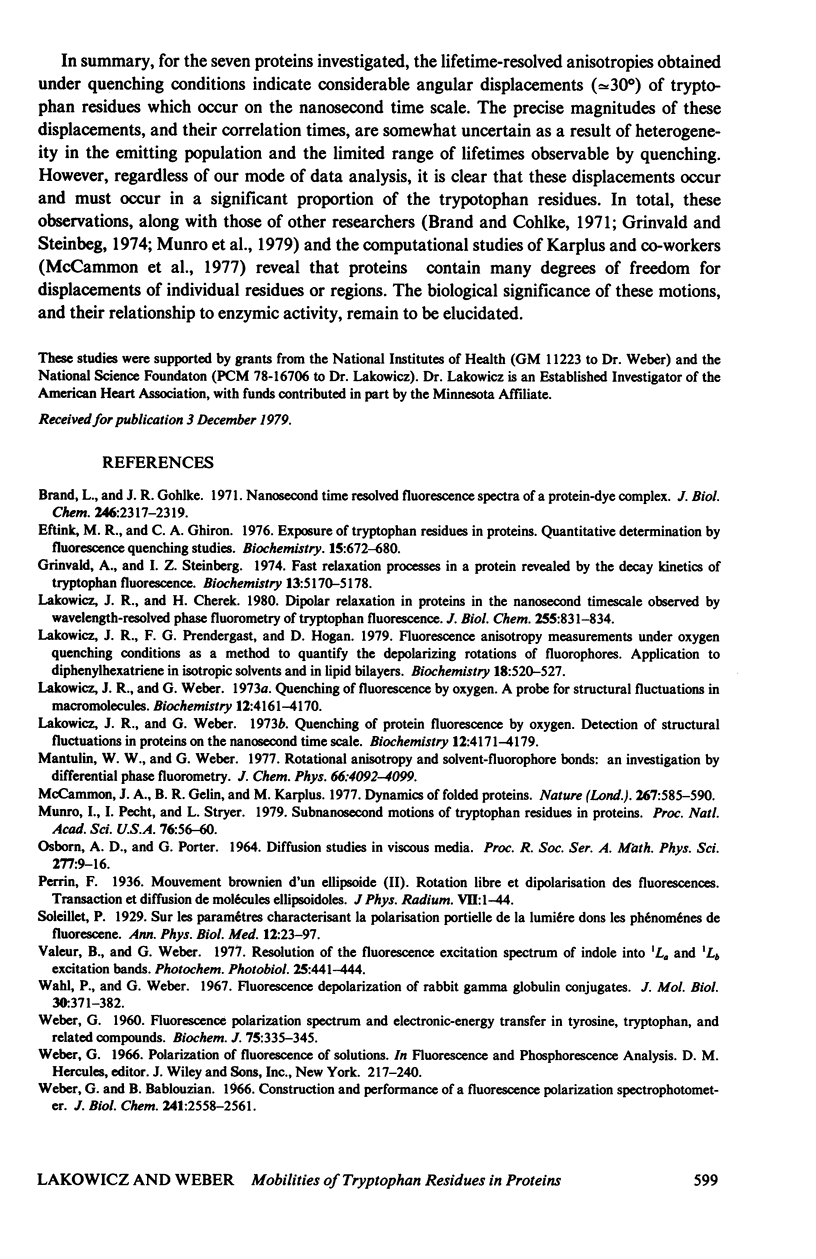
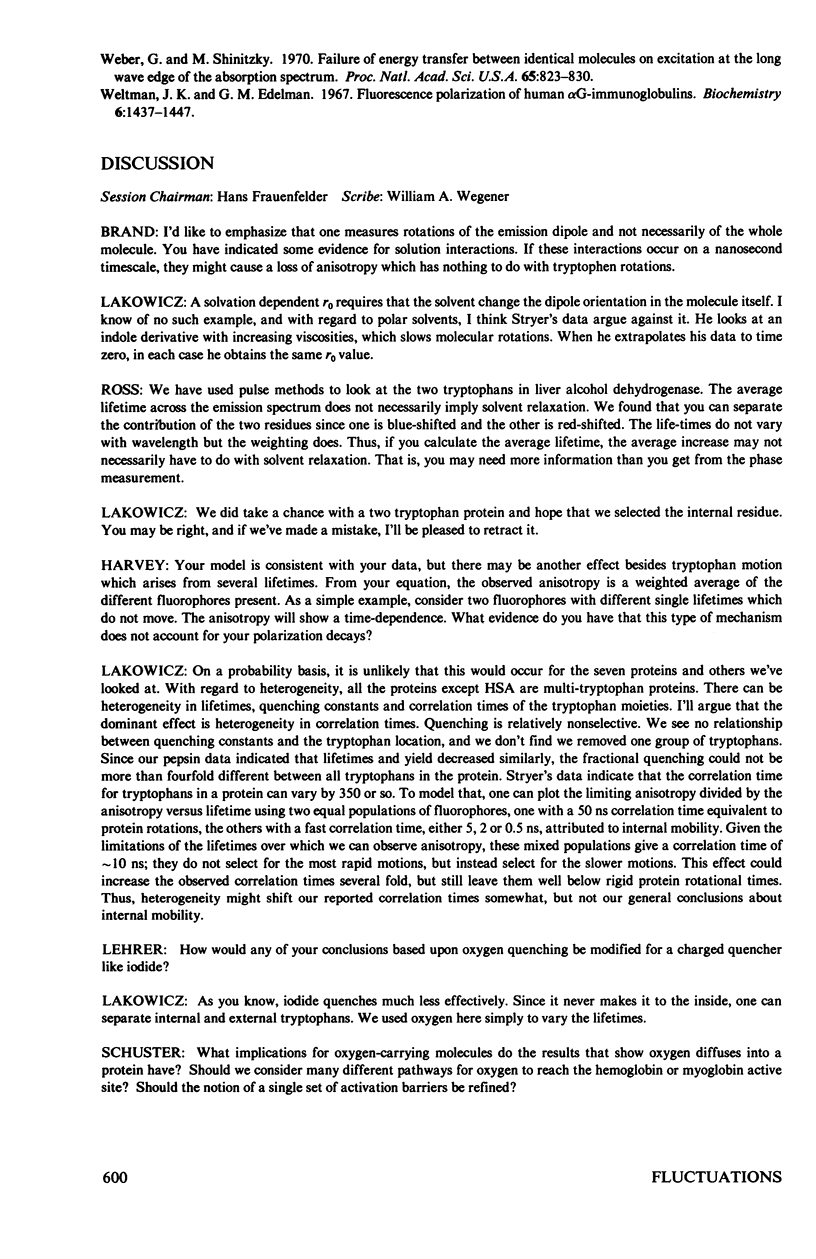
Selected References
These references are in PubMed. This may not be the complete list of references from this article.
- Brand L., Gohlke J. R. Nanosecond time-resolved fluorescence spectra of a protein-dye complex. J Biol Chem. 1971 Apr 10;246(7):2317–2319. [PubMed] [Google Scholar]
- Eftink M. R., Ghiron C. A. Exposure of tryptophanyl residues in proteins. Quantitative determination by fluorescence quenching studies. Biochemistry. 1976 Feb 10;15(3):672–680. doi: 10.1021/bi00648a035. [DOI] [PubMed] [Google Scholar]
- Grinvald A., Steinberg I. Z. Fast relaxation processes inn a protein revealed by the decay kinetics of tryptophan fluorescence. Biochemistry. 1974 Dec 3;13(25):5170–5178. doi: 10.1021/bi00722a019. [DOI] [PubMed] [Google Scholar]
- Lakowicz J. R., Cherek H. Dipolar relaxation in proteins on the nanosecond timescale observed by wavelength-resolved phase fluorometry of tryptophan fluorescence. J Biol Chem. 1980 Feb 10;255(3):831–834. [PubMed] [Google Scholar]
- Lakowicz J. R., Prendergast F. G., Hogen D. Fluorescence anisotropy measurements under oxygen quenching conditions as a method to quantify the depolarizing rotations of fluorophores. Application to diphenylhexatriene in isotropic solvents and in lipid bilayers. Biochemistry. 1979 Feb 6;18(3):520–527. doi: 10.1021/bi00570a022. [DOI] [PMC free article] [PubMed] [Google Scholar]
- Lakowicz J. R., Weber G. Quenching of fluorescence by oxygen. A probe for structural fluctuations in macromolecules. Biochemistry. 1973 Oct 9;12(21):4161–4170. doi: 10.1021/bi00745a020. [DOI] [PMC free article] [PubMed] [Google Scholar]
- Lakowicz J. R., Weber G. Quenching of protein fluorescence by oxygen. Detection of structural fluctuations in proteins on the nanosecond time scale. Biochemistry. 1973 Oct 9;12(21):4171–4179. doi: 10.1021/bi00745a021. [DOI] [PMC free article] [PubMed] [Google Scholar]
- McCammon J. A., Gelin B. R., Karplus M. Dynamics of folded proteins. Nature. 1977 Jun 16;267(5612):585–590. doi: 10.1038/267585a0. [DOI] [PubMed] [Google Scholar]
- Munro I., Pecht I., Stryer L. Subnanosecond motions of tryptophan residues in proteins. Proc Natl Acad Sci U S A. 1979 Jan;76(1):56–60. doi: 10.1073/pnas.76.1.56. [DOI] [PMC free article] [PubMed] [Google Scholar]
- Valeur B., Weber G. Resolution of the fluorescence excitation spectrum of indole into the 1La and 1Lb excitation bands. Photochem Photobiol. 1977 May;25(5):441–444. doi: 10.1111/j.1751-1097.1977.tb09168.x. [DOI] [PubMed] [Google Scholar]
- WEBER G. Fluorescence-polarization spectrum and electronic-energy transfer in tyrosine, tryptophan and related compounds. Biochem J. 1960 May;75:335–345. doi: 10.1042/bj0750335. [DOI] [PMC free article] [PubMed] [Google Scholar]
- Wahl P., Weber G. Fluorescence depolarization of rabbit gamma globulin conjugates. J Mol Biol. 1967 Dec 14;30(2):371–382. doi: 10.1016/s0022-2836(67)80045-7. [DOI] [PubMed] [Google Scholar]
- Weber G., Bablouzian B. Construction and performance of a fluorescence polarization spectrophotometer. J Biol Chem. 1966 Jun 10;241(11):2558–2561. [PubMed] [Google Scholar]
- Weber G., Shinitzky M. Failure of Energy Transfer between Identical Aromatic Molecules on Excitation at the Long Wave Edge of the Absorption Spectrum. Proc Natl Acad Sci U S A. 1970 Apr;65(4):823–830. doi: 10.1073/pnas.65.4.823. [DOI] [PMC free article] [PubMed] [Google Scholar]
- Weltman J. K., Edelman G. M. Fluorescence polarization of human gamma-G-immunoglobulins. Biochemistry. 1967 May;6(5):1437–1447. doi: 10.1021/bi00857a028. [DOI] [PubMed] [Google Scholar]