Abstract
The strongest myosin-related features in the low-angle axial x-ray diffraction pattern of resting frog sartorius muscle are the meridional reflections corresponding to axial spacings of 21.4 and 14.3 nm, and the first layer line, at a spacing 42.9 nm. During tetanus the intensities of the first layer line and the 21.4-nm meridional decrease by 62 and 80% respectively, but, when the muscle is fresh, the 14.3-nm meridional intensity rises by 13%, although it shows a decrease when the muscle is fatigued. The large change in the intensity of the 21.4-nm meridional reflection suggests that the projected myosin cross-bridge density onto the thick filament axis changes during contraction. The model proposed by Bennett (Ph.D. Thesis, University of London, 1977) in which successive cross-bridge levels are at 0,3/8, and 5/8 of the 42.9-nm axial repeat in the resting muscle, passing to 0, 1/3, and 2/3 in the contracting state, can explain why the 21.4-nm reflection decreases in intensity while the 14.3-nm increases when the muscle is activated. The model predicts a rather larger increase of the 14.3-nm reflection intensity during contraction than that observed, but the discrepancy may be removed if a small change of shape or tilt of the cross-bridges relative to the thick filament axis is introduced. The decrease of the intensity of the first layer line indicates that the cross-bridges become disordered in the plane perpendicular to the filament axis.
Full text
PDF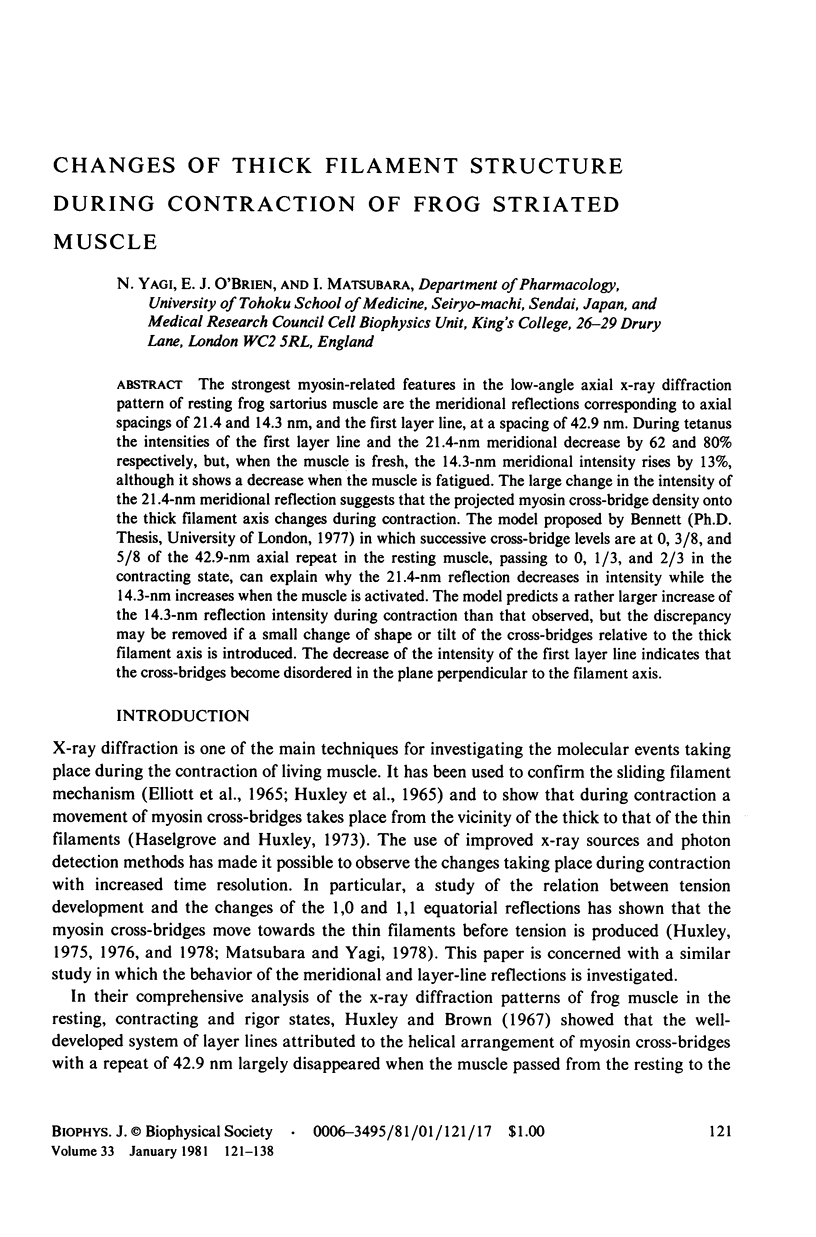
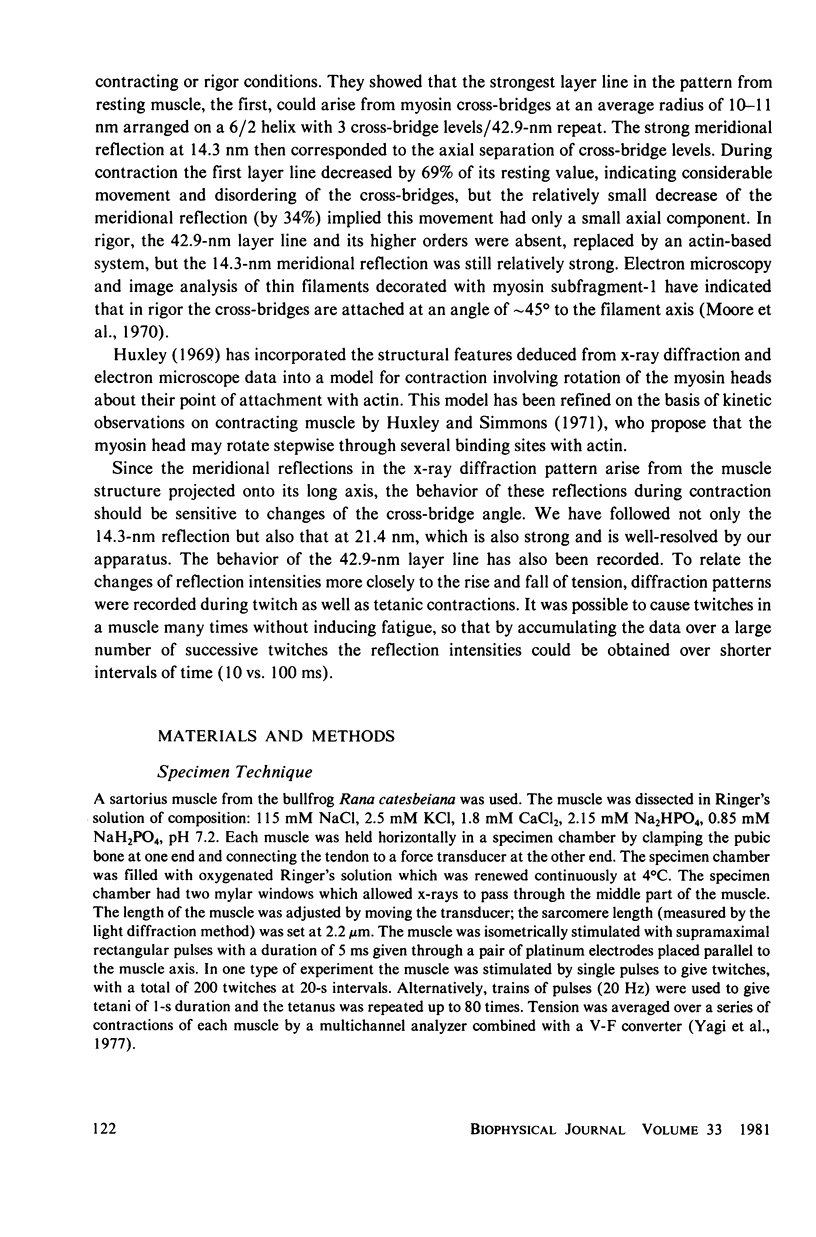
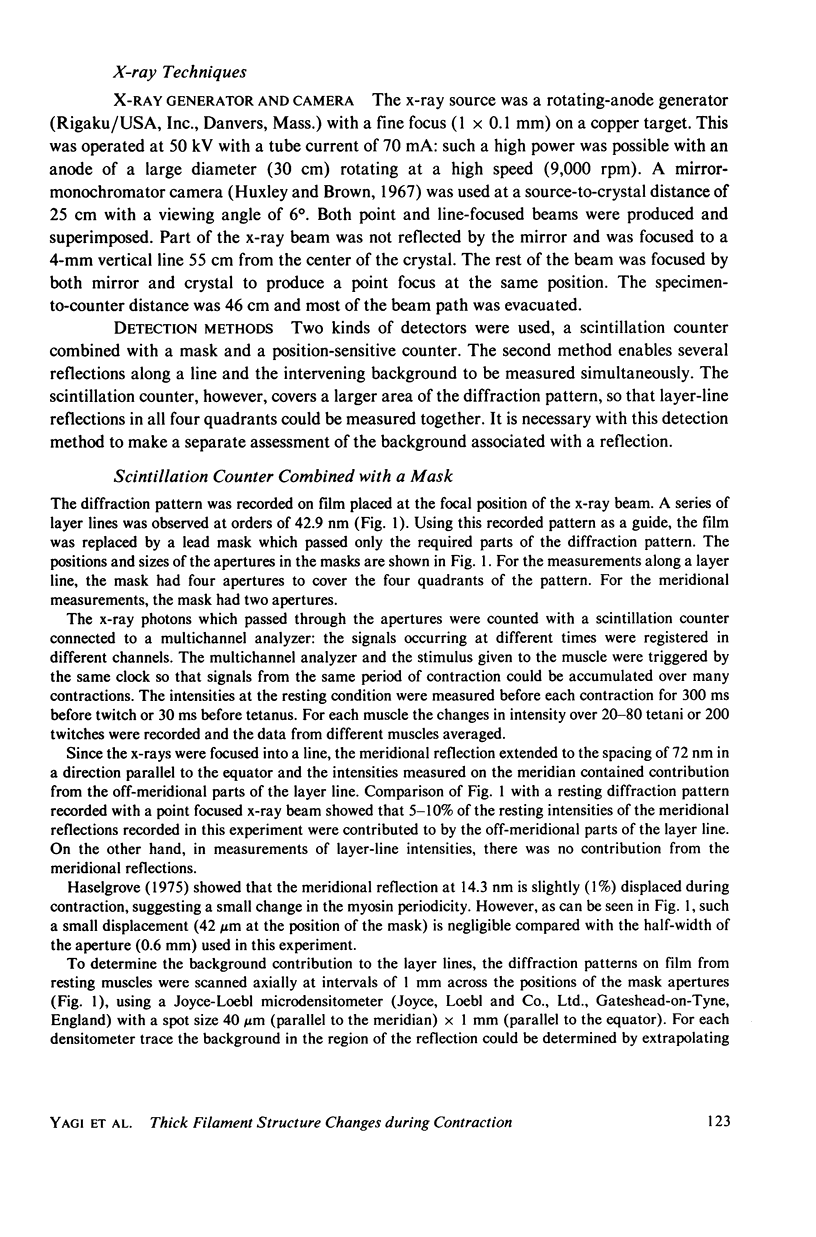
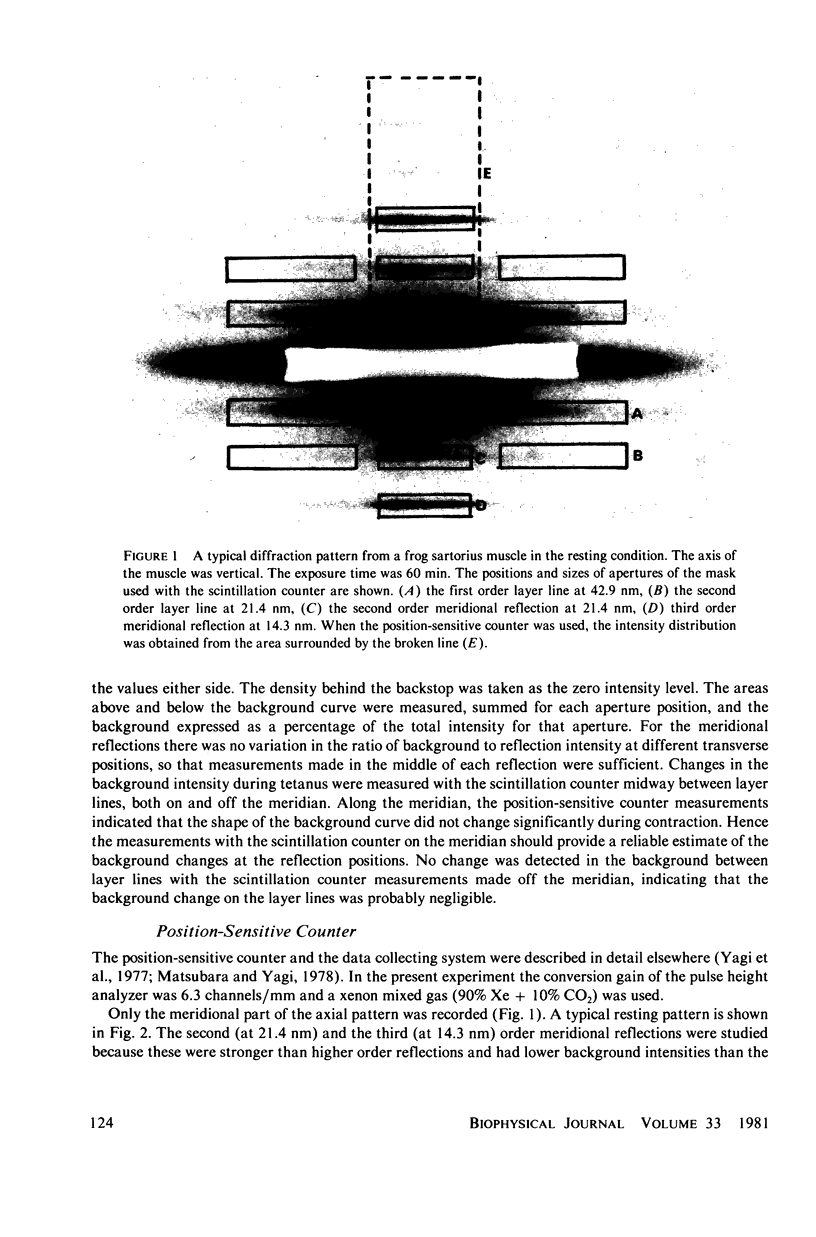
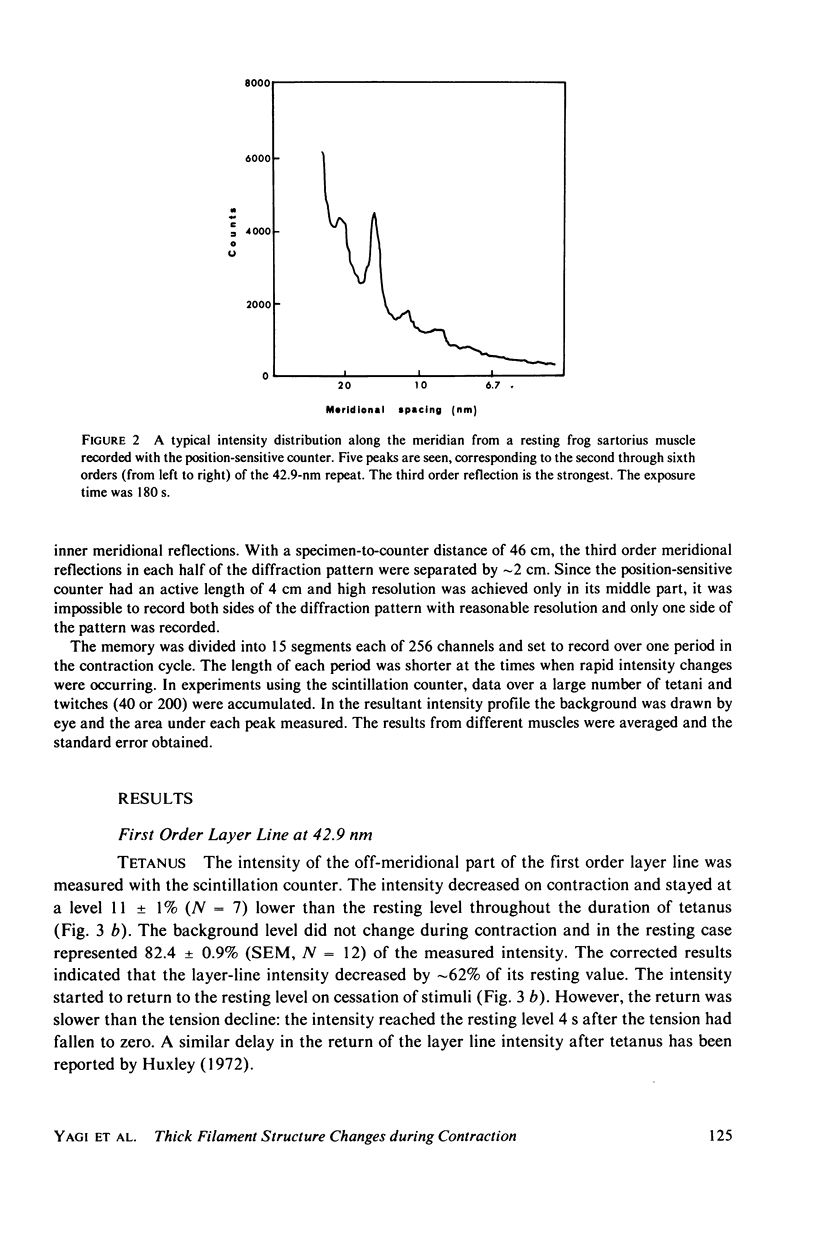
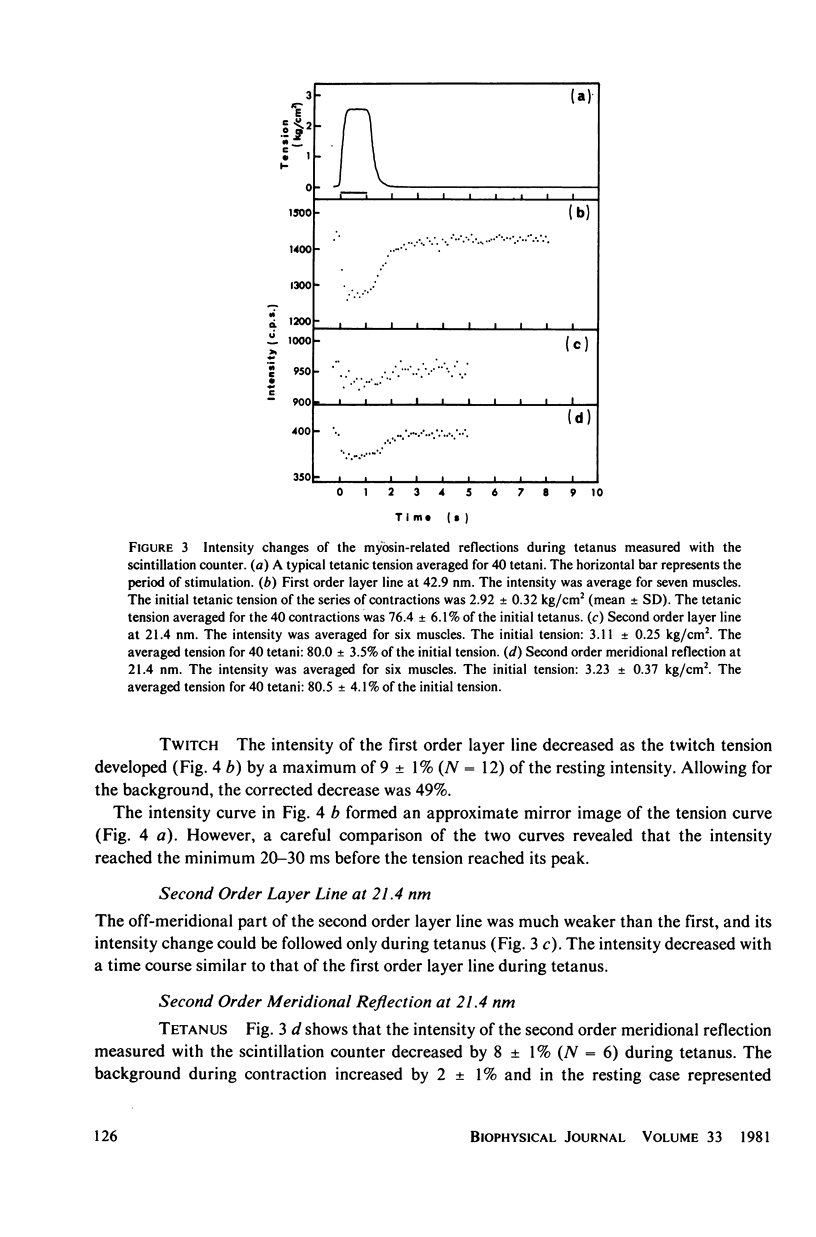
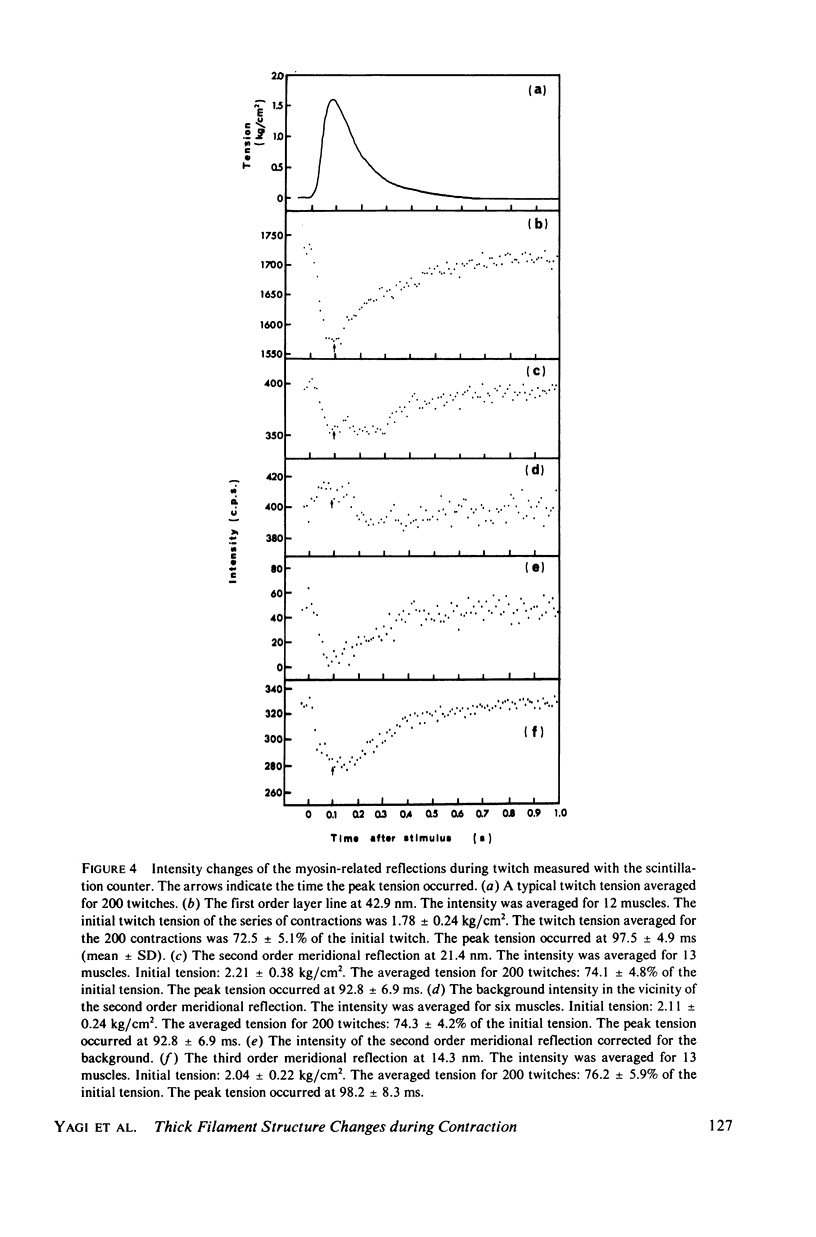
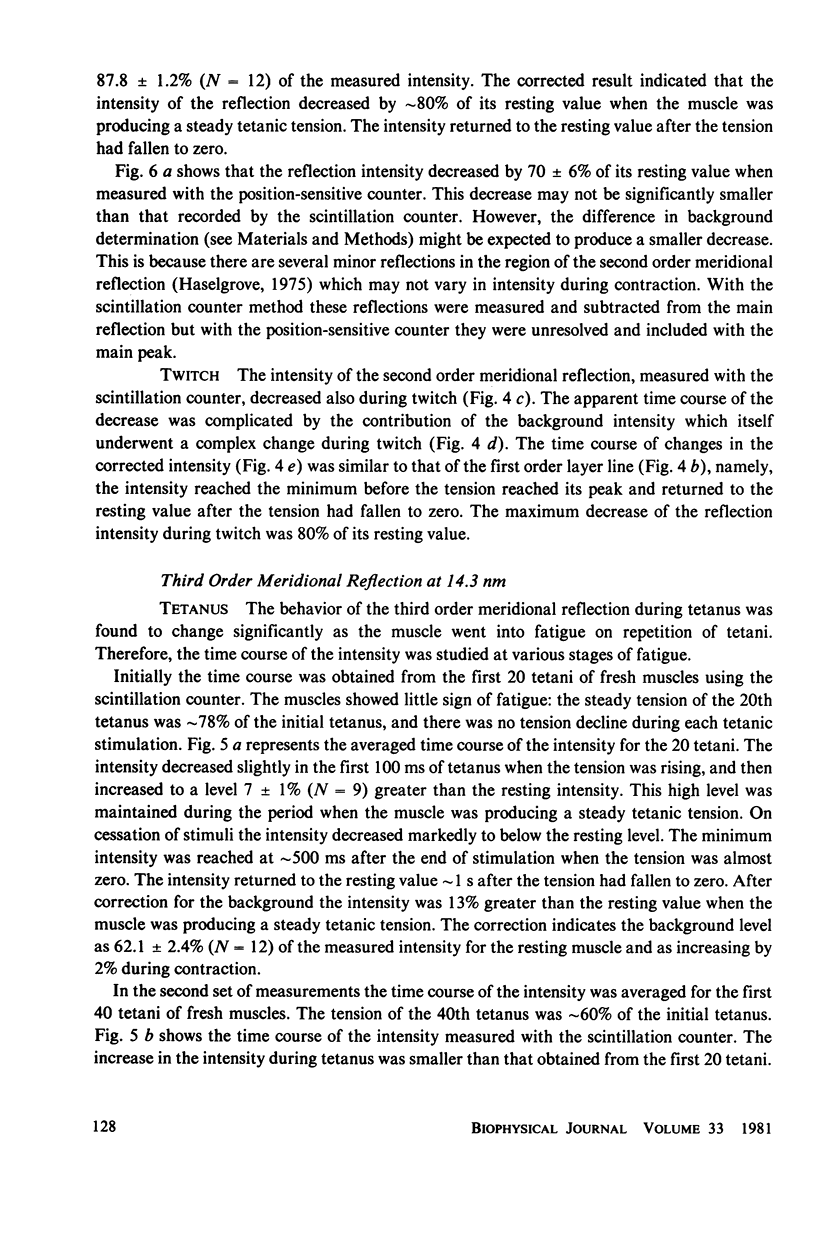
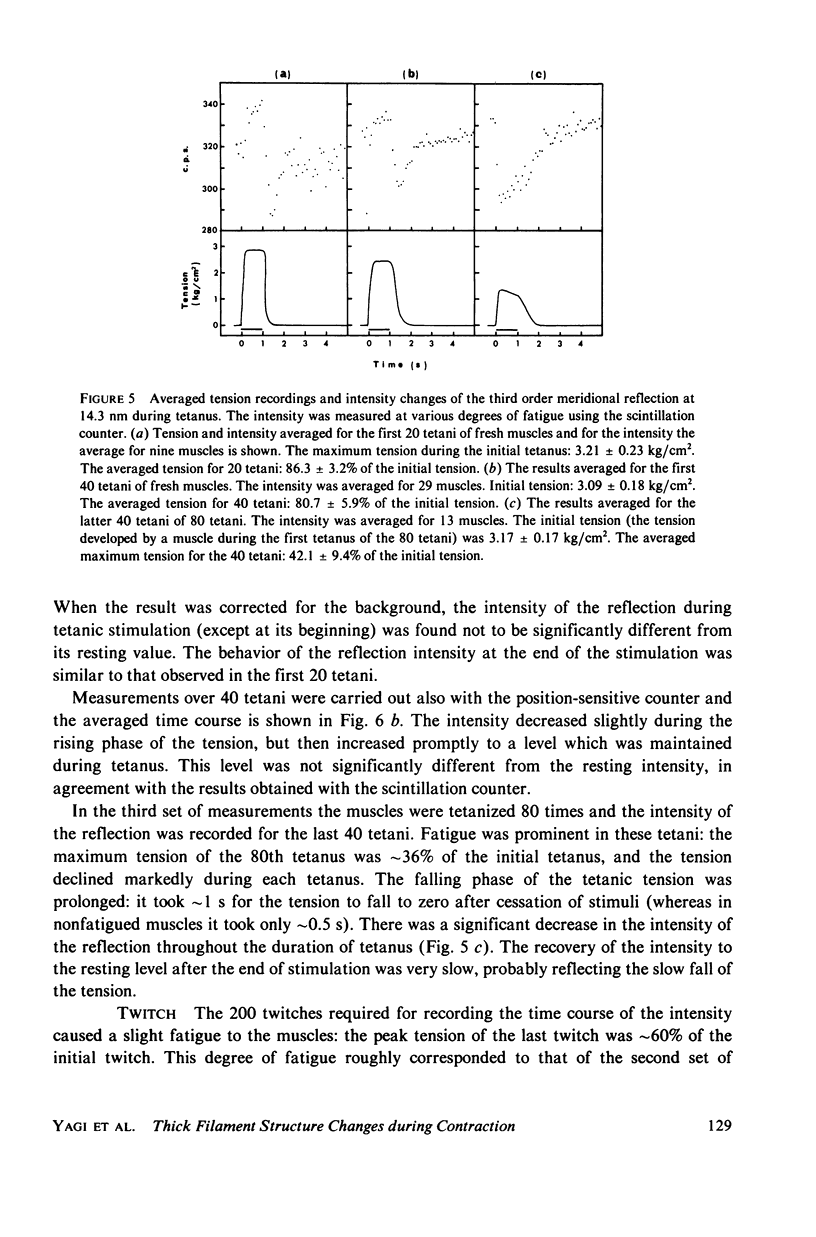
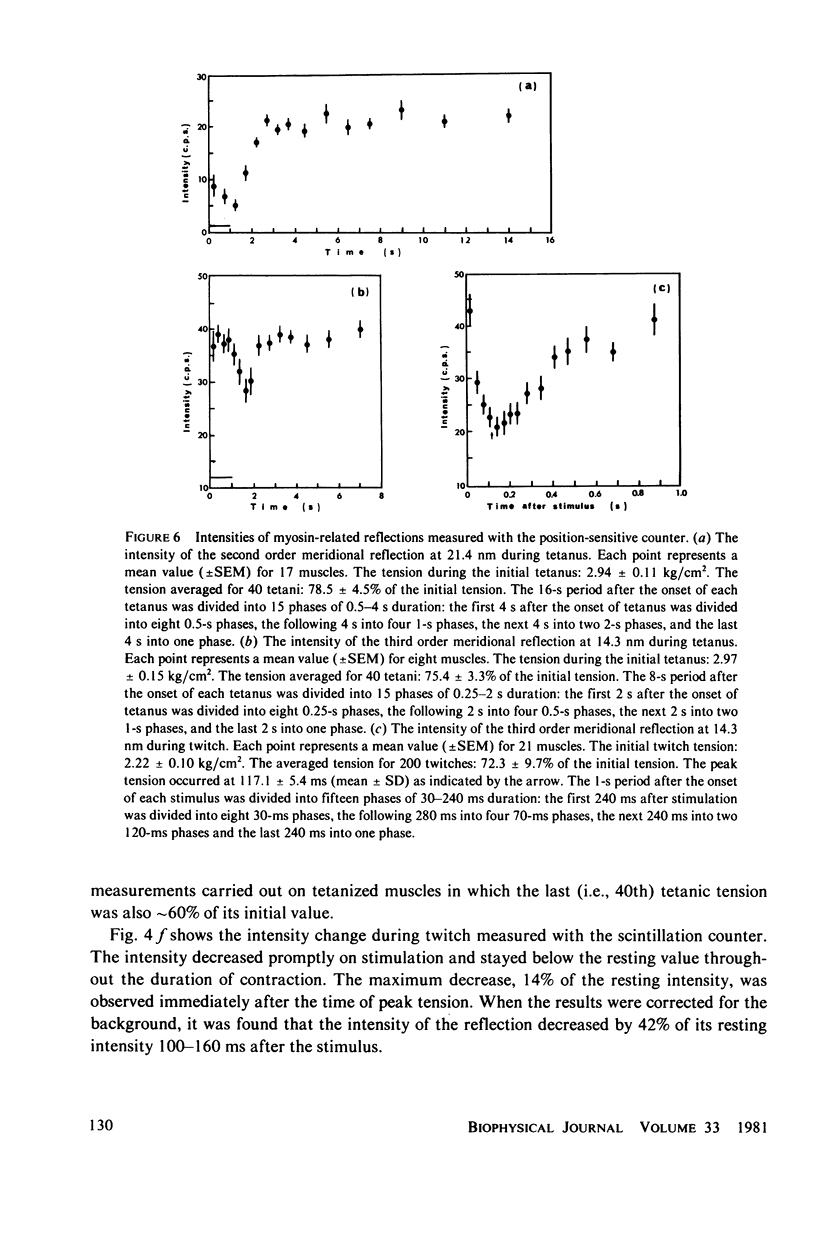
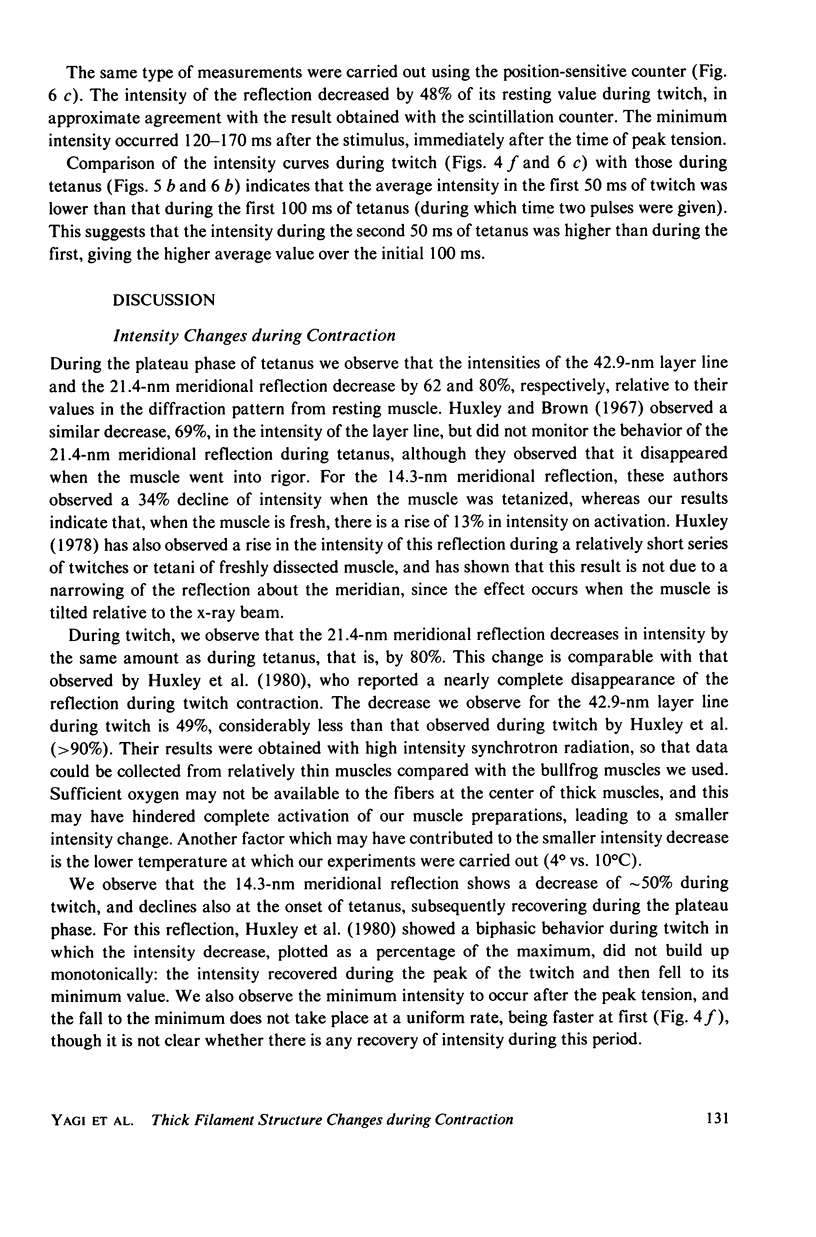
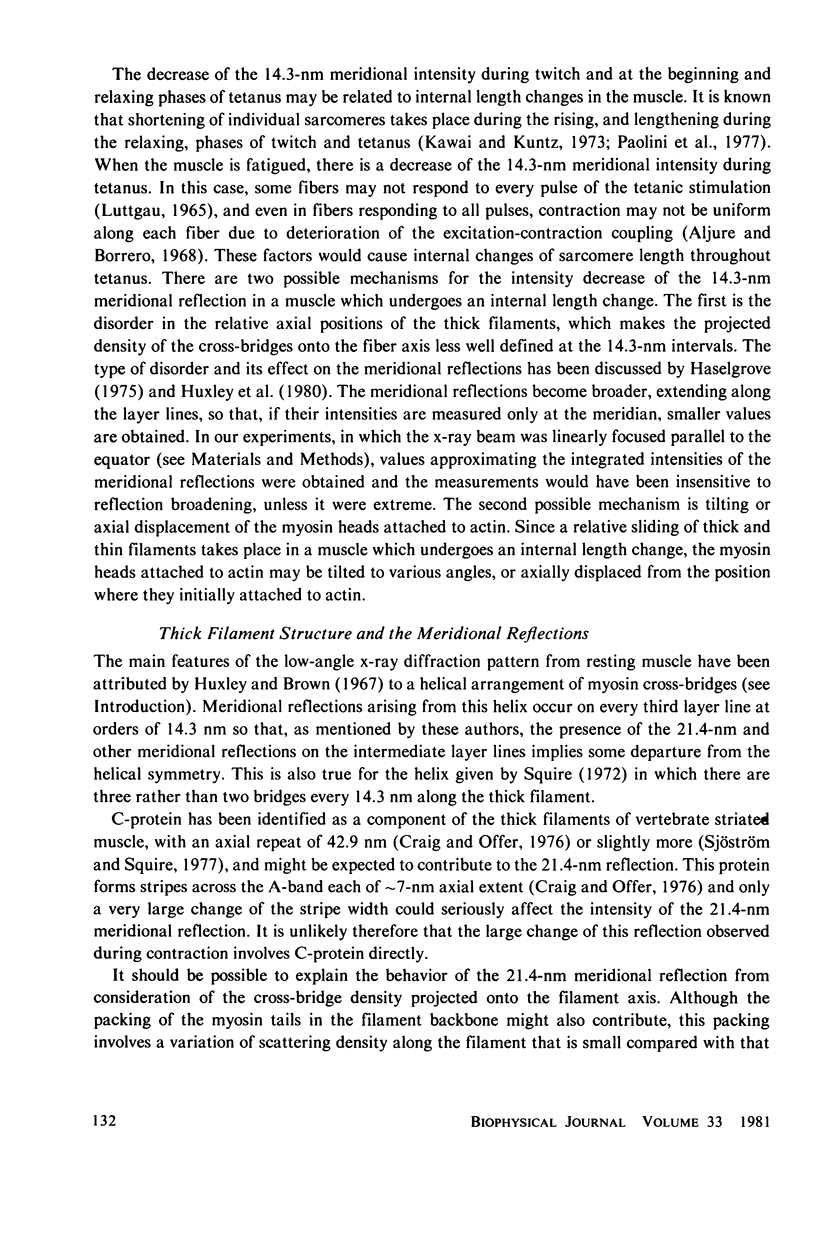
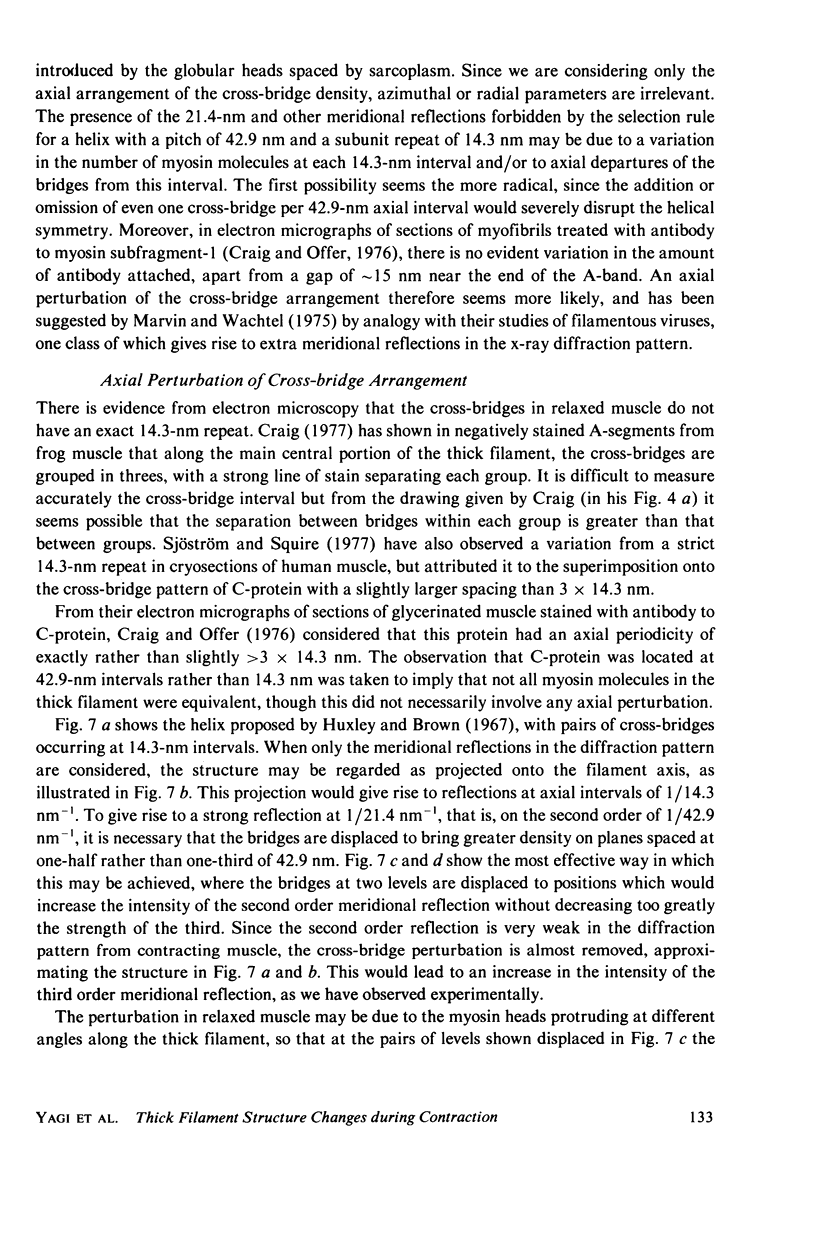
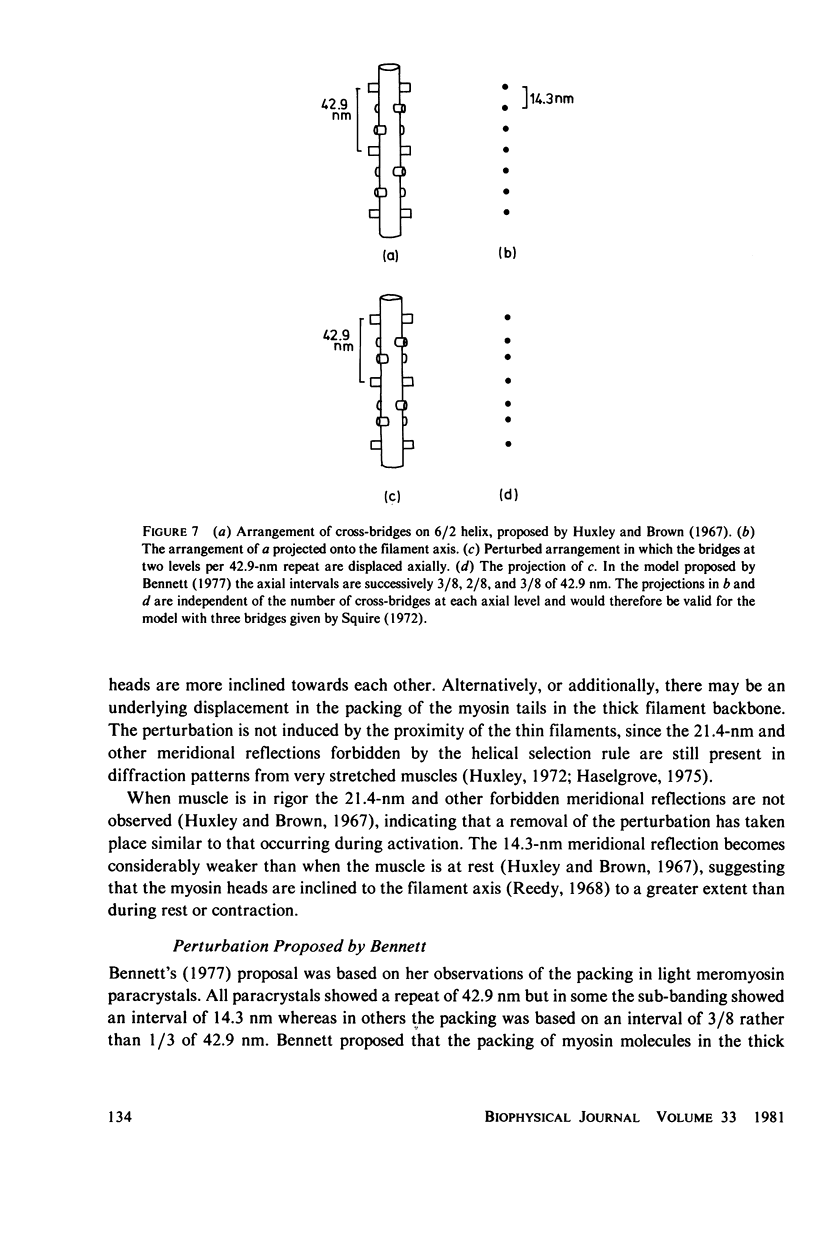
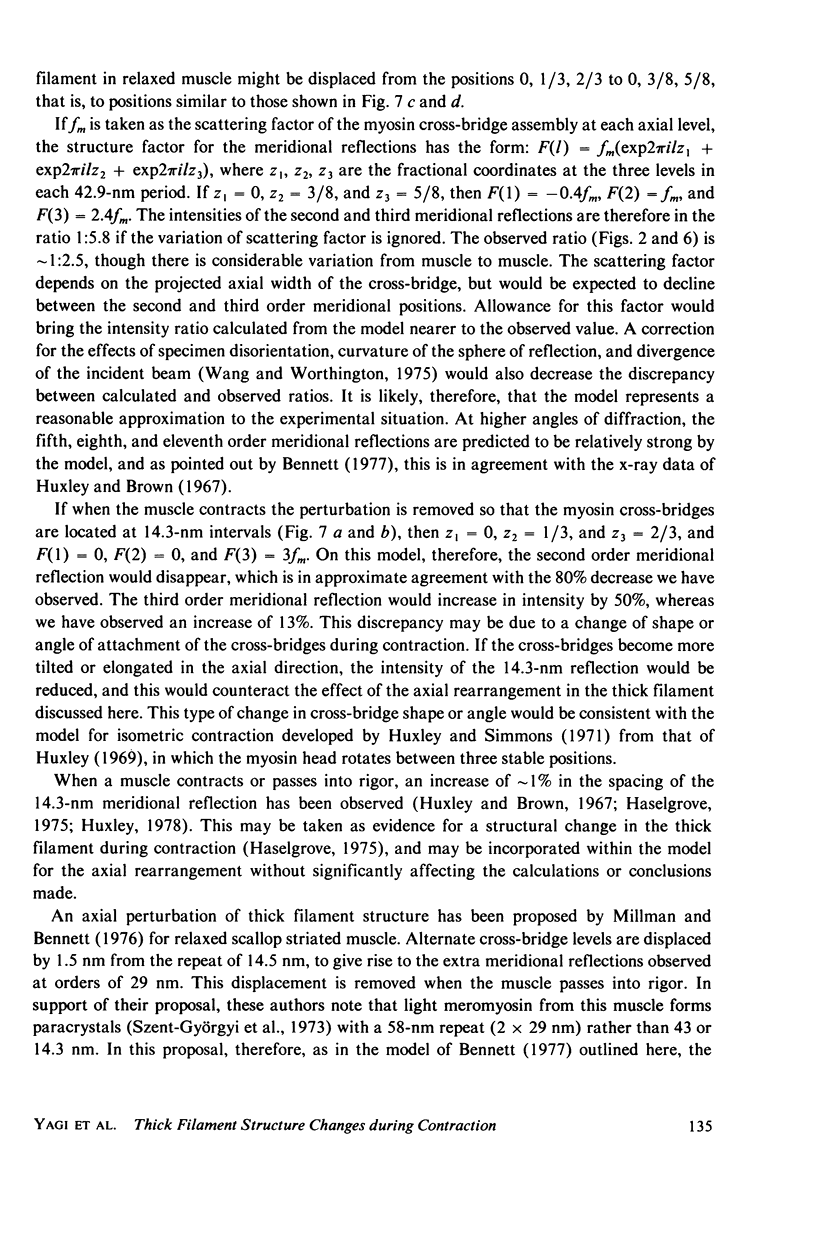
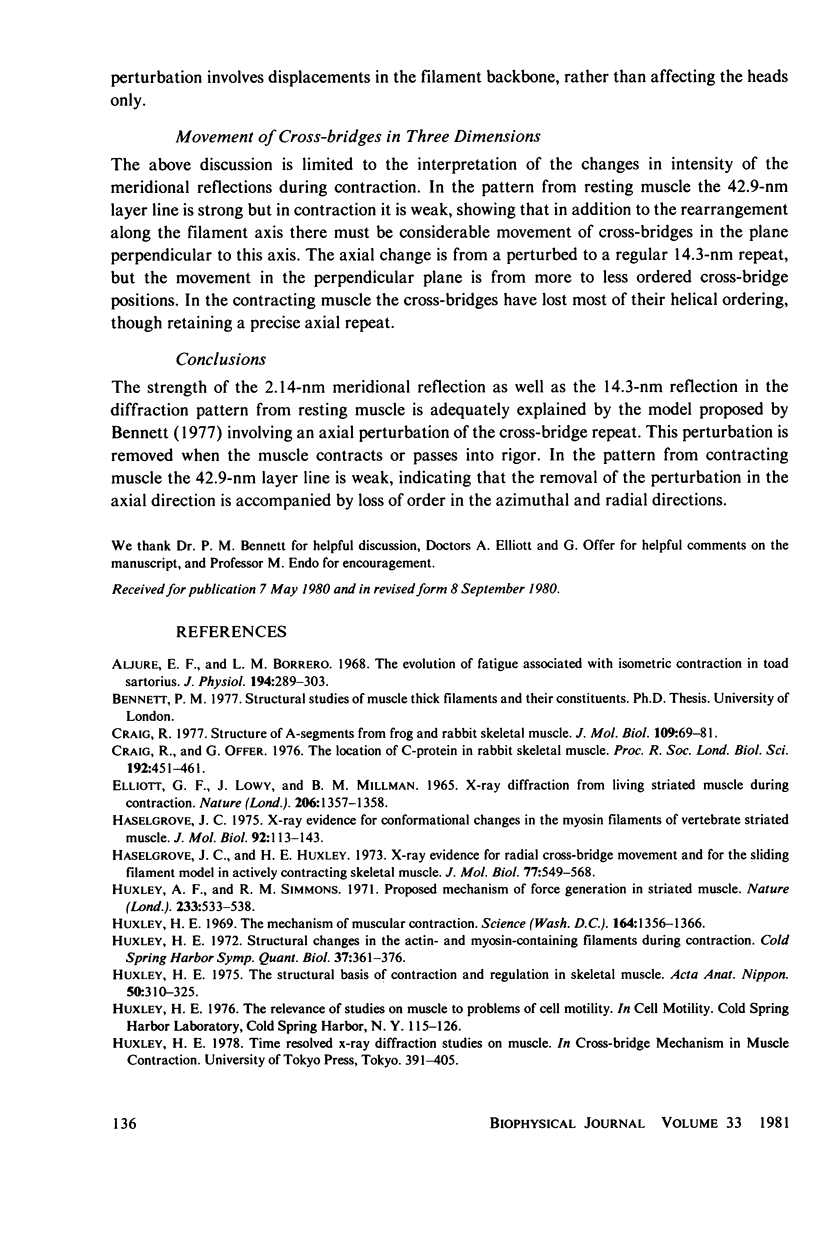
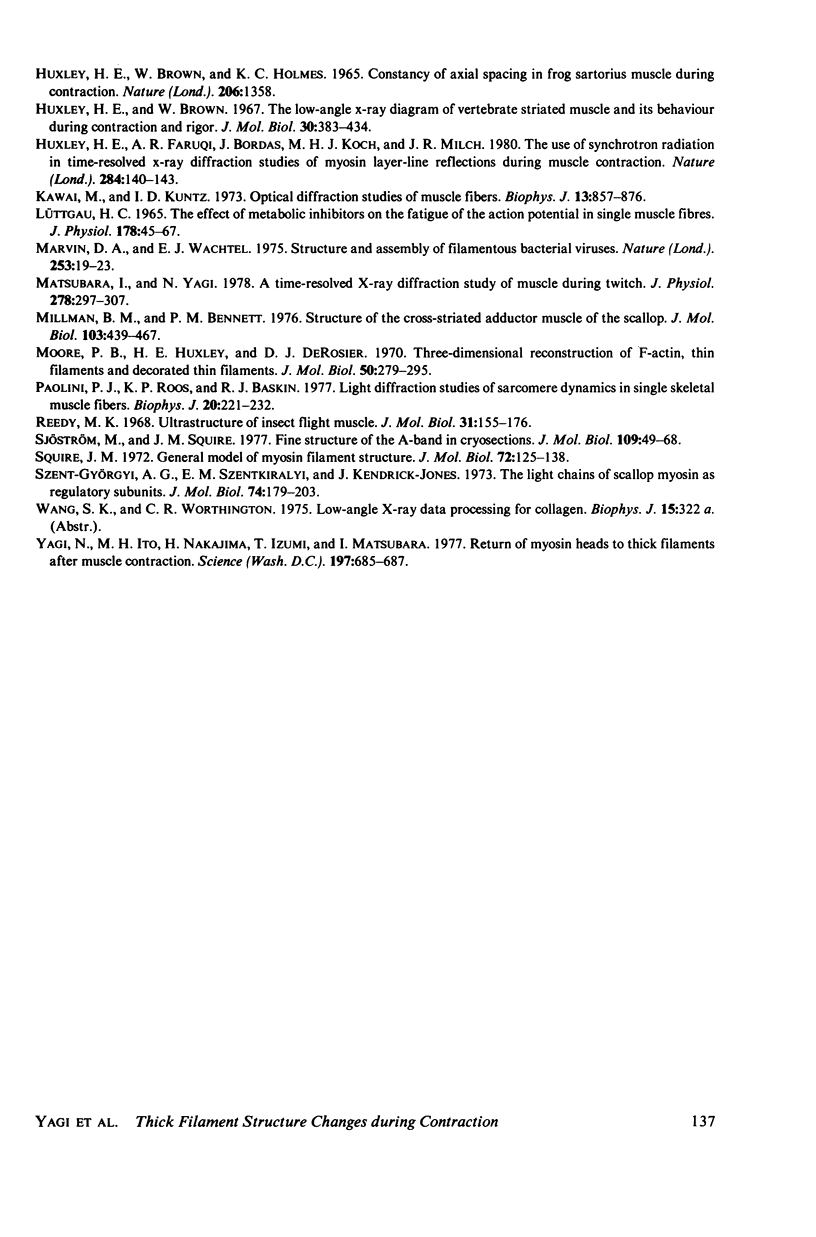
Images in this article
Selected References
These references are in PubMed. This may not be the complete list of references from this article.
- Aljure E. F., Borrero L. M. The evolution of fatigue associated with isometric contraction in toad sartorius. J Physiol. 1968 Feb;194(2):289–303. doi: 10.1113/jphysiol.1968.sp008408. [DOI] [PMC free article] [PubMed] [Google Scholar]
- Craig R., Offer G. The location of C-protein in rabbit skeletal muscle. Proc R Soc Lond B Biol Sci. 1976 Mar 16;192(1109):451–461. doi: 10.1098/rspb.1976.0023. [DOI] [PubMed] [Google Scholar]
- Craig R. Structure of A-segments from frog and rabbit skeletal muscle. J Mol Biol. 1977 Jan 5;109(1):69–81. doi: 10.1016/s0022-2836(77)80046-6. [DOI] [PubMed] [Google Scholar]
- Elliott G. F., Lowy J., Millman B. M. X-ray diffraction from living striated muscle during contraction. Nature. 1965 Jun 26;206(991):1357–1358. doi: 10.1038/2061357a0. [DOI] [PubMed] [Google Scholar]
- Haselgrove J. C., Huxley H. E. X-ray evidence for radial cross-bridge movement and for the sliding filament model in actively contracting skeletal muscle. J Mol Biol. 1973 Jul 15;77(4):549–568. doi: 10.1016/0022-2836(73)90222-2. [DOI] [PubMed] [Google Scholar]
- Haselgrove J. C. X-ray evidence for conformational changes in the myosin filaments of vertebrate striated muscle. J Mol Biol. 1975 Feb 15;92(1):113–143. doi: 10.1016/0022-2836(75)90094-7. [DOI] [PubMed] [Google Scholar]
- Huxley A. F., Simmons R. M. Proposed mechanism of force generation in striated muscle. Nature. 1971 Oct 22;233(5321):533–538. doi: 10.1038/233533a0. [DOI] [PubMed] [Google Scholar]
- Huxley H. E., Brown W., Holmes K. C. Constancy of axial spacings in frog sartorius muscle during contraction. Nature. 1965 Jun 26;206(991):1358–1358. doi: 10.1038/2061358a0. [DOI] [PubMed] [Google Scholar]
- Huxley H. E., Brown W. The low-angle x-ray diagram of vertebrate striated muscle and its behaviour during contraction and rigor. J Mol Biol. 1967 Dec 14;30(2):383–434. doi: 10.1016/s0022-2836(67)80046-9. [DOI] [PubMed] [Google Scholar]
- Huxley H. E., Faruqi A. R., Bordas J., Koch M. H., Milch J. R. The use of synchrotron radiation in time-resolved X-ray diffraction studies of myosin layer-line reflections during muscle contraction. Nature. 1980 Mar 13;284(5752):140–143. doi: 10.1038/284140a0. [DOI] [PubMed] [Google Scholar]
- Huxley H. E. The mechanism of muscular contraction. Science. 1969 Jun 20;164(3886):1356–1365. doi: 10.1126/science.164.3886.1356. [DOI] [PubMed] [Google Scholar]
- Huxley H. E. The structural basis of contraction and regulation in skeletal muscle. Kaibogaku Zasshi. 1975 Dec;50(6):310–325. [PubMed] [Google Scholar]
- Kawai M., Kuntz I. D. Optical diffraction studies of muscle fibers. Biophys J. 1973 Sep;13(9):857–876. doi: 10.1016/S0006-3495(73)86031-X. [DOI] [PMC free article] [PubMed] [Google Scholar]
- LUETTGAU H. C. THE EFFECT OF METABOLIC INHIBITORS ON THE FATIGUE OF THE ACTION POTENTIAL IN SINGLE MUSCLE FIBRES. J Physiol. 1965 May;178:45–67. doi: 10.1113/jphysiol.1965.sp007613. [DOI] [PMC free article] [PubMed] [Google Scholar]
- Marvin D. A., Wachtel E. J. Structure and assembly of filamentous bacterial viruses. Nature. 1975 Jan 3;253(5486):19–23. doi: 10.1038/253019a0. [DOI] [PubMed] [Google Scholar]
- Matsubara I., Yagi N. A time-resolved X-ray diffraction study of muscle during twitch. J Physiol. 1978 May;278:297–307. doi: 10.1113/jphysiol.1978.sp012305. [DOI] [PMC free article] [PubMed] [Google Scholar]
- Millman B. M., Bennett P. M., Bennett P. M. Structure of the cross-striated adductor muscle of the scallop. J Mol Biol. 1976 May 25;103(3):439–467. doi: 10.1016/0022-2836(76)90212-6. [DOI] [PubMed] [Google Scholar]
- Moore P. B., Huxley H. E., DeRosier D. J. Three-dimensional reconstruction of F-actin, thin filaments and decorated thin filaments. J Mol Biol. 1970 Jun 14;50(2):279–295. doi: 10.1016/0022-2836(70)90192-0. [DOI] [PubMed] [Google Scholar]
- Paolini P. J., Roos K. P., Baskin R. J. Light diffraction studies of sarcomere dynamics in single skeletal muscle fibers. Biophys J. 1977 Nov;20(2):221–232. doi: 10.1016/S0006-3495(77)85545-8. [DOI] [PMC free article] [PubMed] [Google Scholar]
- Reedy M. K. Ultrastructure of insect flight muscle. I. Screw sense and structural grouping in the rigor cross-bridge lattice. J Mol Biol. 1968 Jan 28;31(2):155–176. doi: 10.1016/0022-2836(68)90437-3. [DOI] [PubMed] [Google Scholar]
- Sjöström M., Squire J. M. Fine structure of the A-band in cryo-sections. The structure of the A-band of human skeletal muscle fibres from ultra-thin cryo-sections negatively stained. J Mol Biol. 1977 Jan 5;109(1):49–68. doi: 10.1016/s0022-2836(77)80045-4. [DOI] [PubMed] [Google Scholar]
- Squire J. M. General model of myosin filament structure. II. Myosin filaments and cross-bridge interactions in vertebrate striated and insect flight muscles. J Mol Biol. 1972 Dec 14;72(1):125–138. doi: 10.1016/0022-2836(72)90074-5. [DOI] [PubMed] [Google Scholar]
- Szent-Györgyi A. G., Szentkiralyi E. M., Kendrick-Jonas J. The light chains of scallop myosin as regulatory subunits. J Mol Biol. 1973 Feb 25;74(2):179–203. doi: 10.1016/0022-2836(73)90106-x. [DOI] [PubMed] [Google Scholar]
- Yagi N., Ito M. H., Nakajima H., Izumi T., Matsubara I. Return of myosin heads to thick filaments after muscle contraction. Science. 1977 Aug 12;197(4304):685–687. doi: 10.1126/science.301660. [DOI] [PubMed] [Google Scholar]