Abstract
The self-assembly and nucleation of two-dimensional polymers is described by a theory based on a model of rigid subunits and bonds and simple principles of thermodynamics. The key point in the theory is to separate as an explicit parameter the free energy, primarily attributed to the entropy of the free subunit, that is required to immobilize a subunit in the polymer. Quantitative relations for the association of a subunit forming a longitudinal bond, a lateral bone, or both together are obtained, which demonstrate the basis and magnitude of cooperativity. The same formalism leads to a quantitative estimate for th concentration of the small polymers that are important intermediates in nucleation. It is shown that, if the concentration of free subunits is below a certain "critical supersaturation," the concentration of some essential intermediates is too low to support any significant assembly and nucleation is blocked. If the subunit concentration is above the critical supersaturation, all of the small intermediates are sufficiently stable to form and grow spontaneously. The theory predicts a critical supersaturation of 3.5 to 7 (the ratio of subunit concentration to the equilibrium solubility) for parameters appropriate to assembly of the microtubule wall. Experimentally, nucleation and assembly of microtubules is obtained at somewhat lower concentrations, 1.5 to 3 times the equilibrium solubility. Special mechanisms that could stabilize small polymers and facilitate nucleation of microtubule assembly are suggested.
Full text
PDF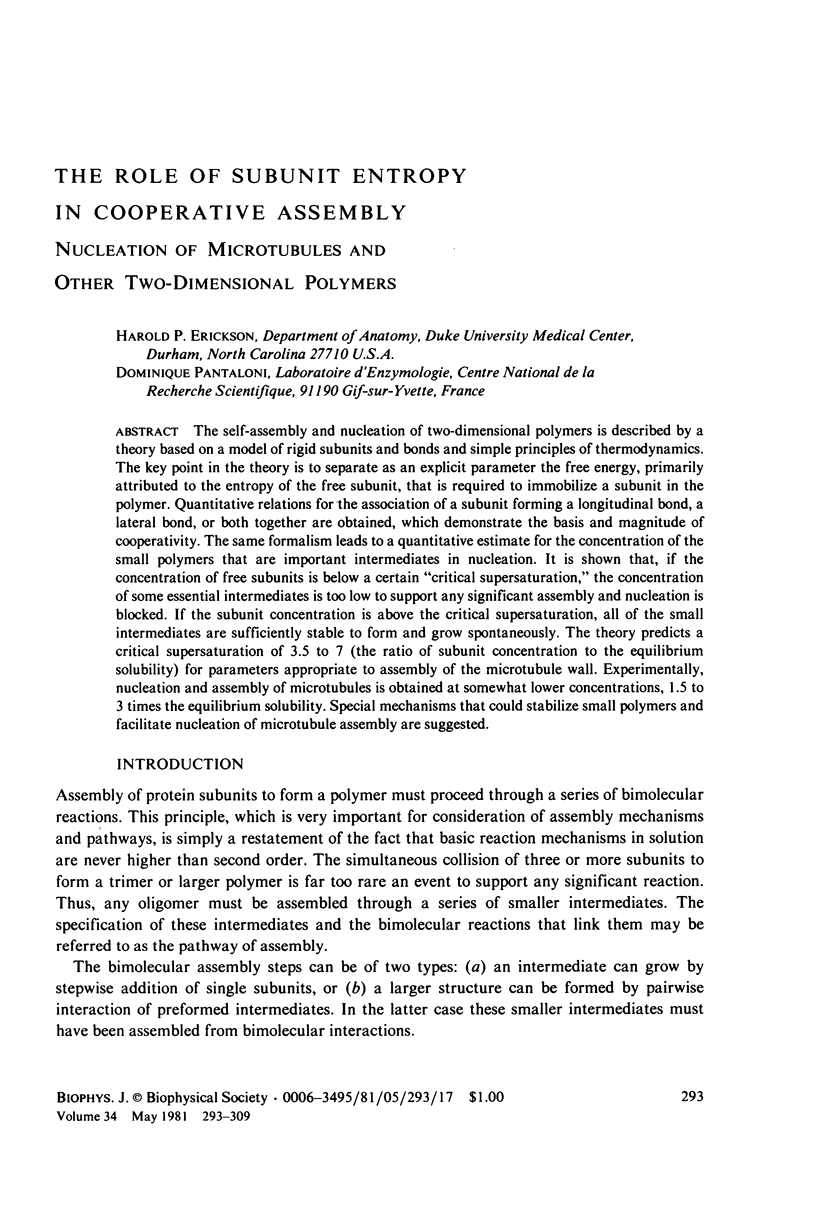
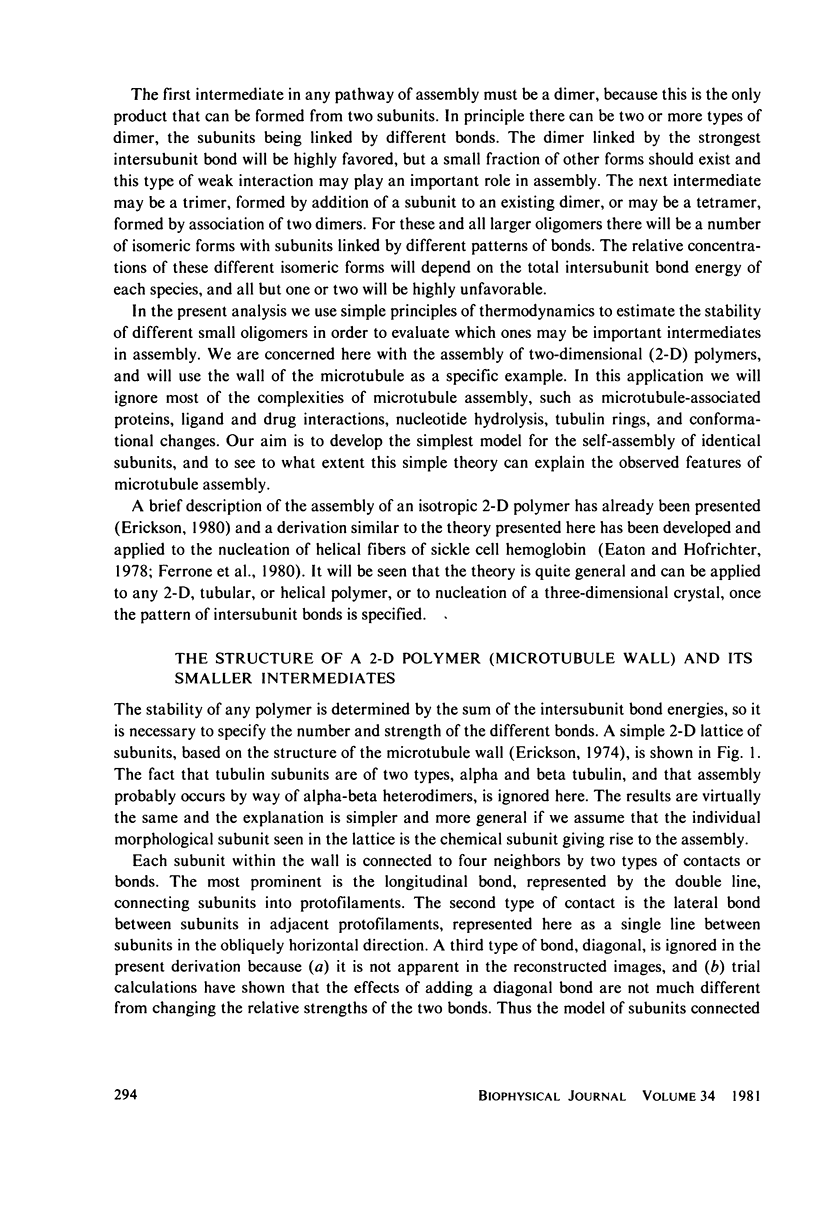
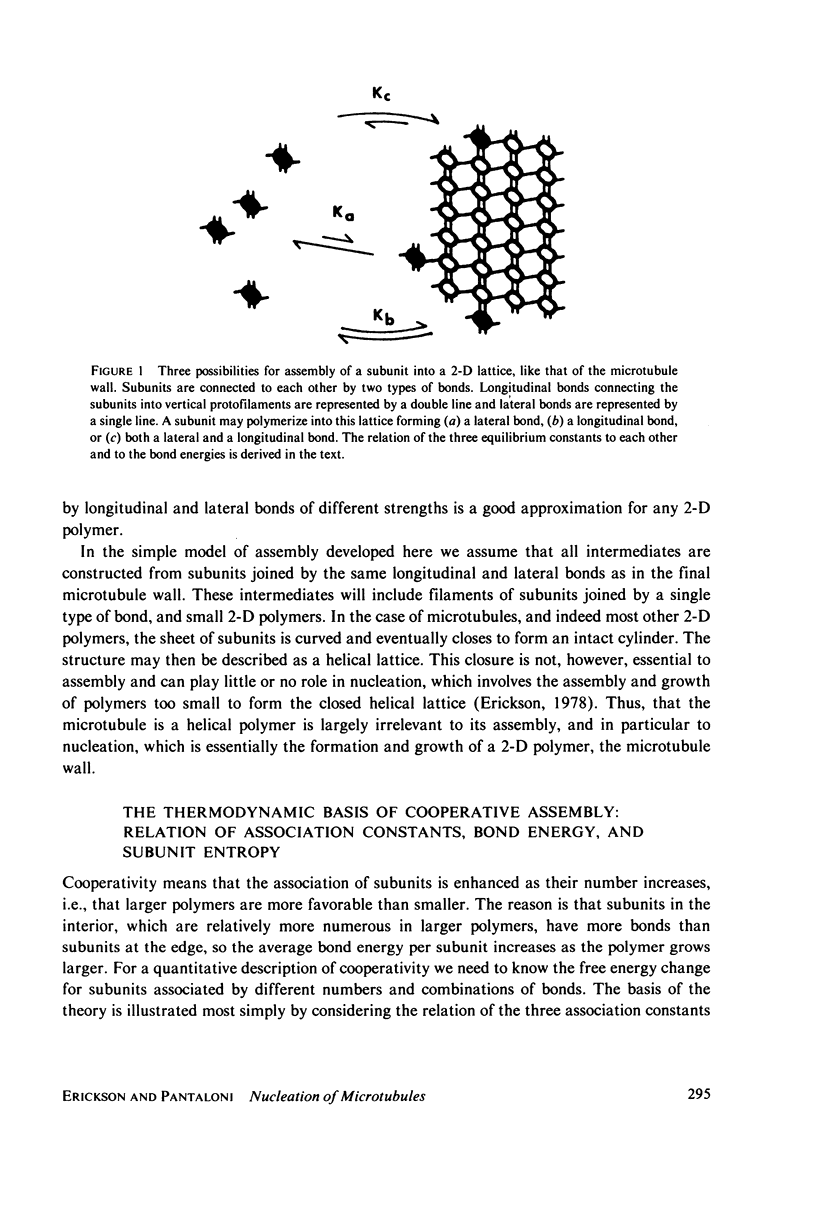
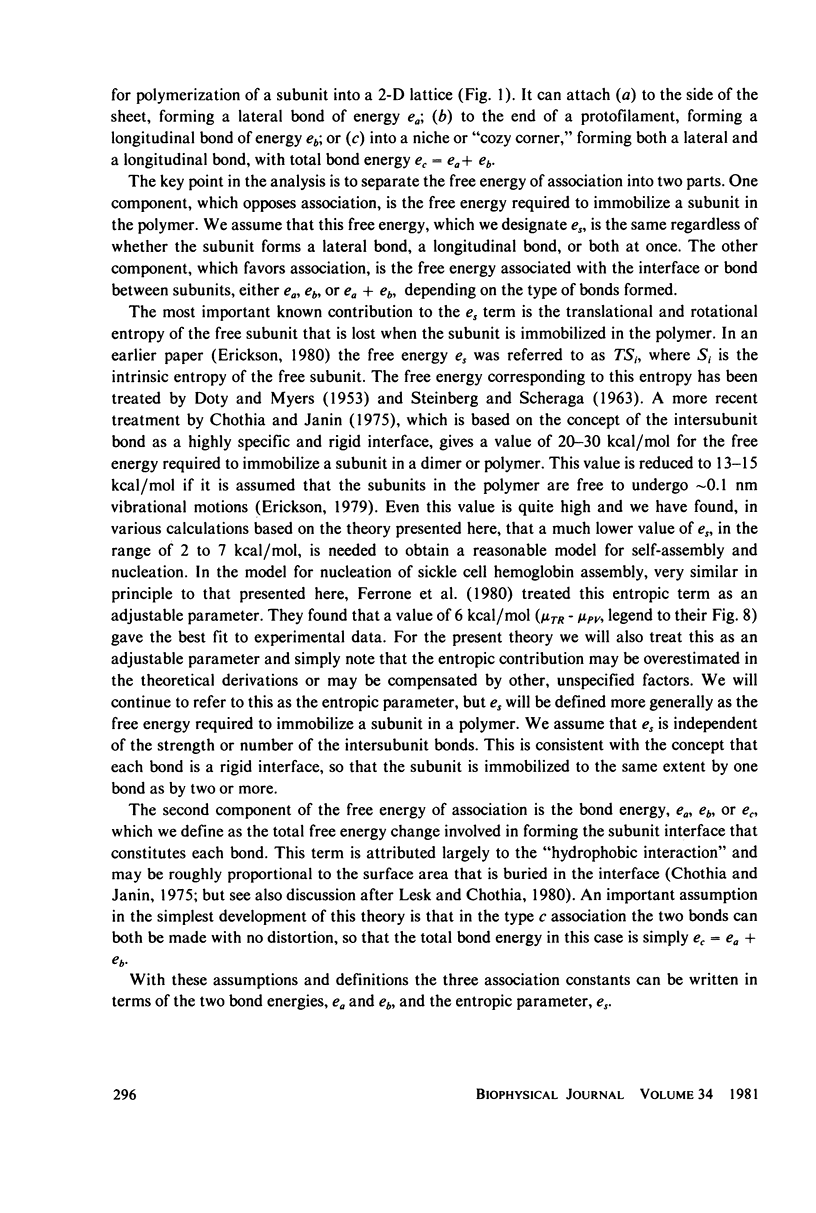
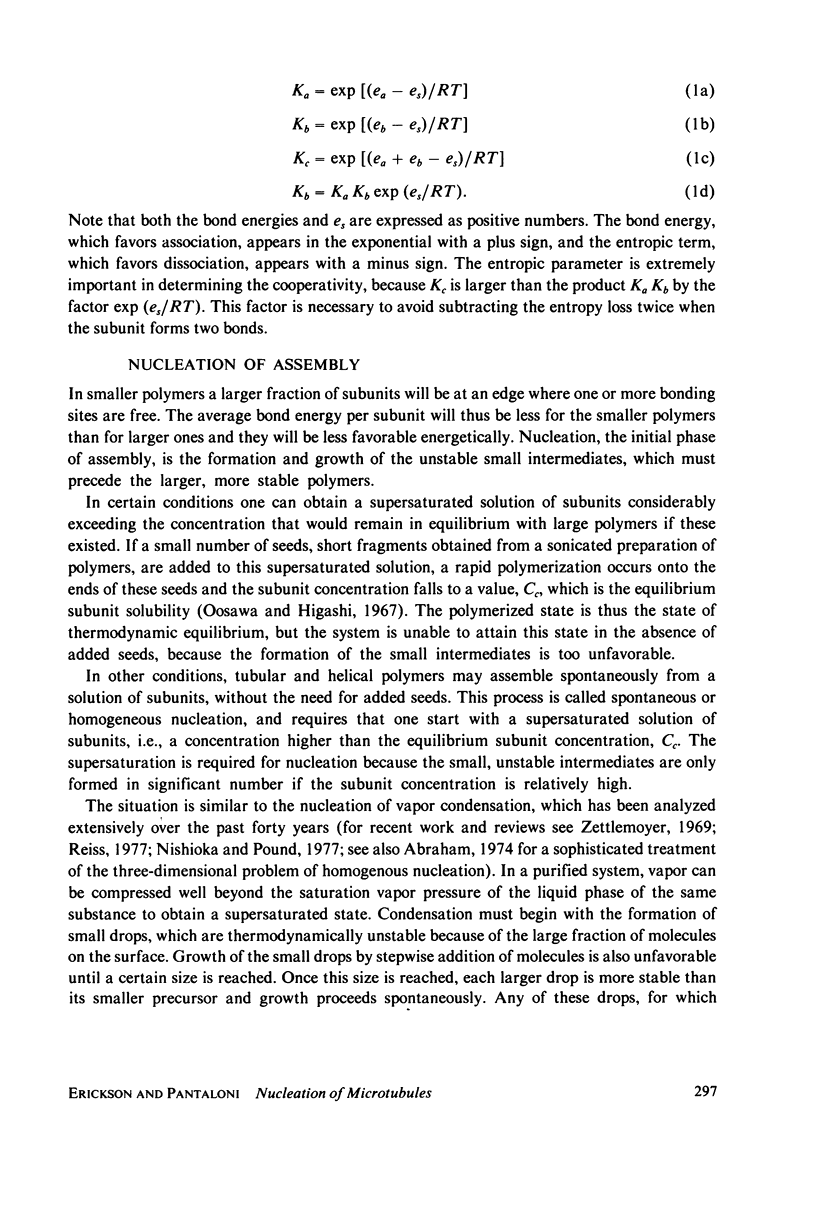
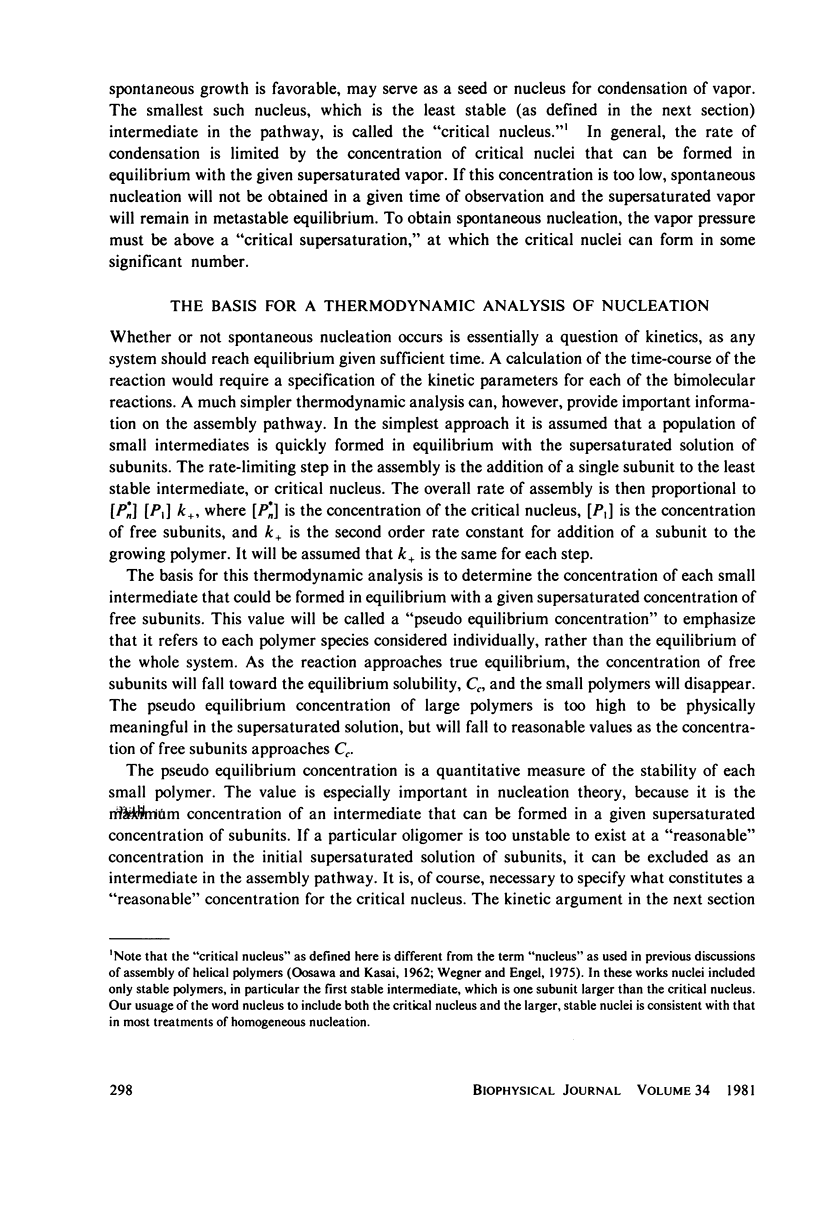
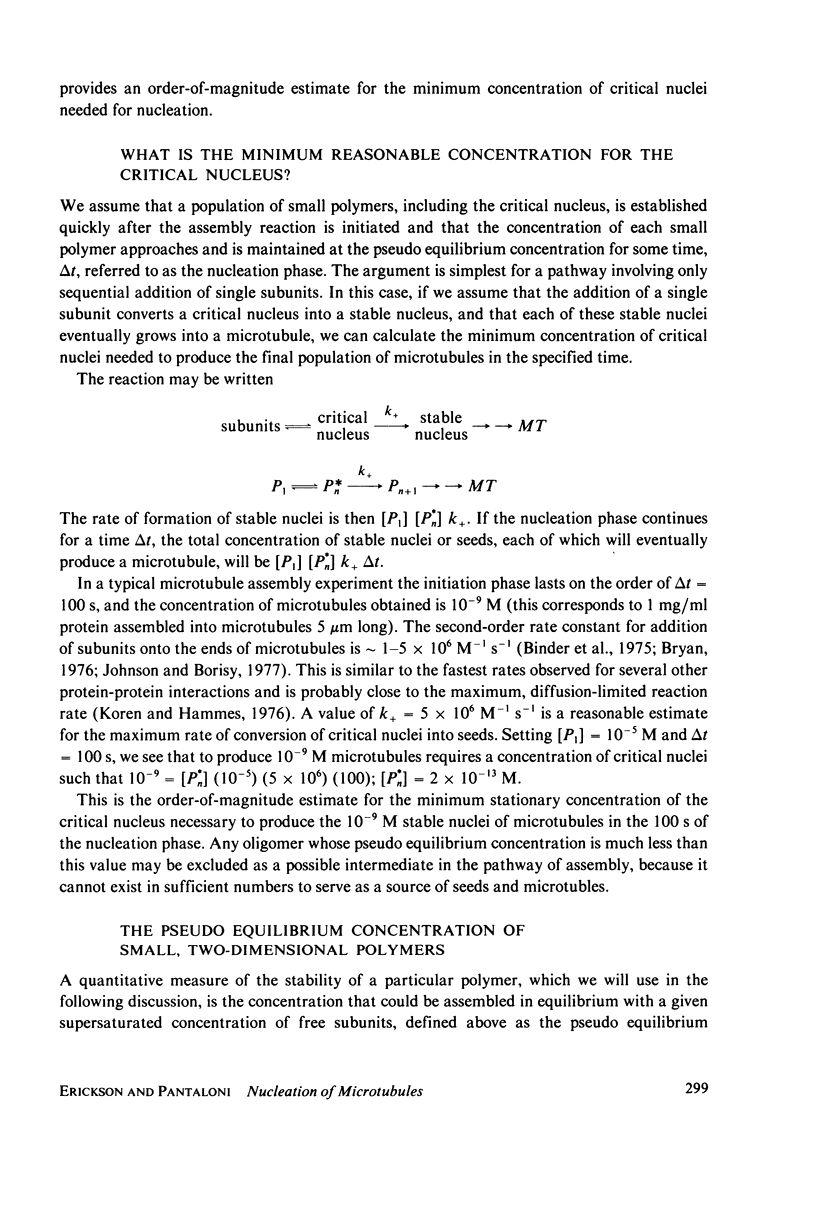
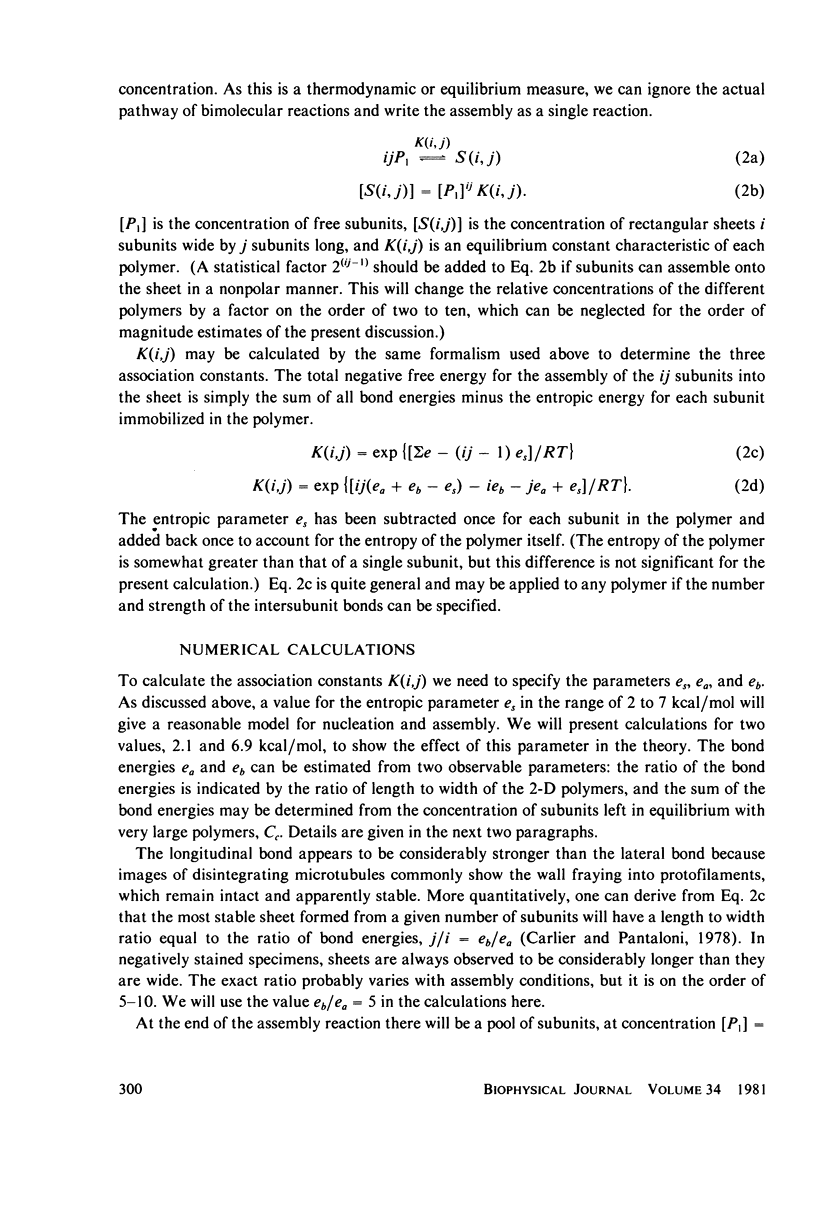
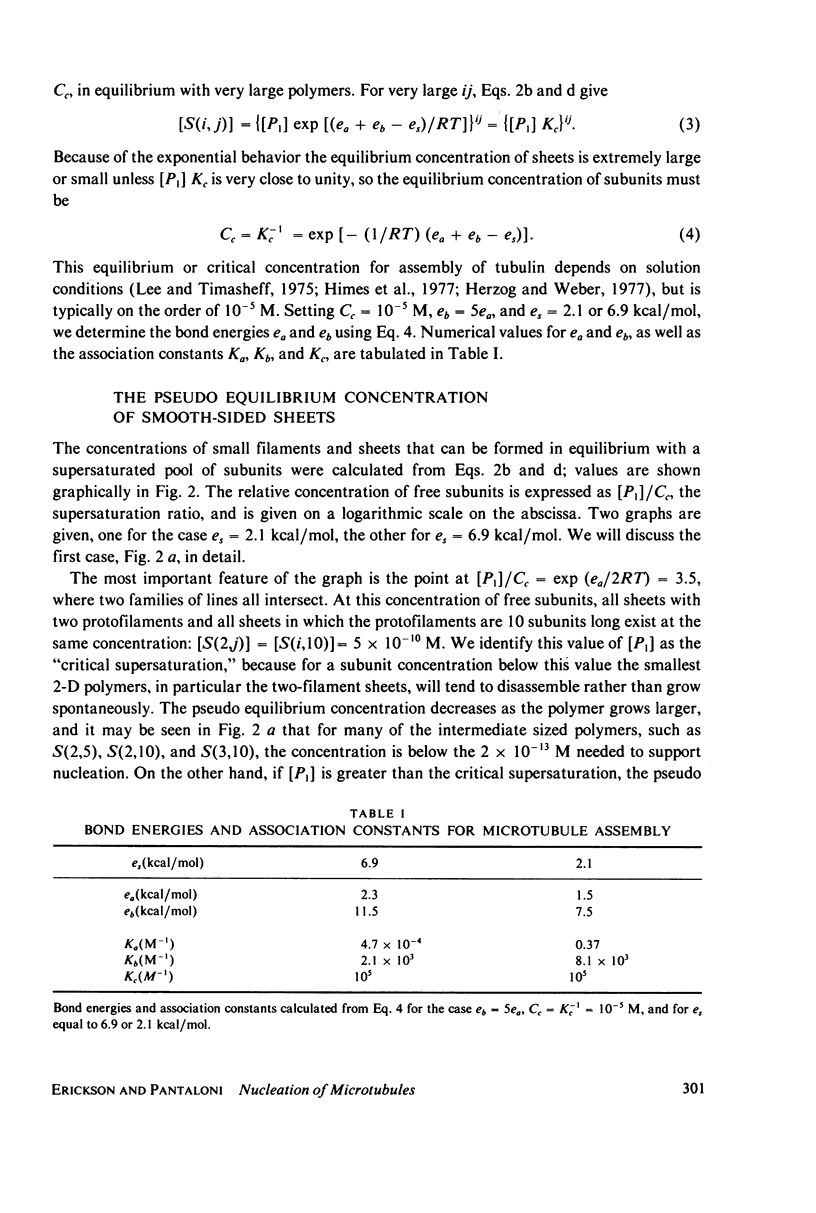
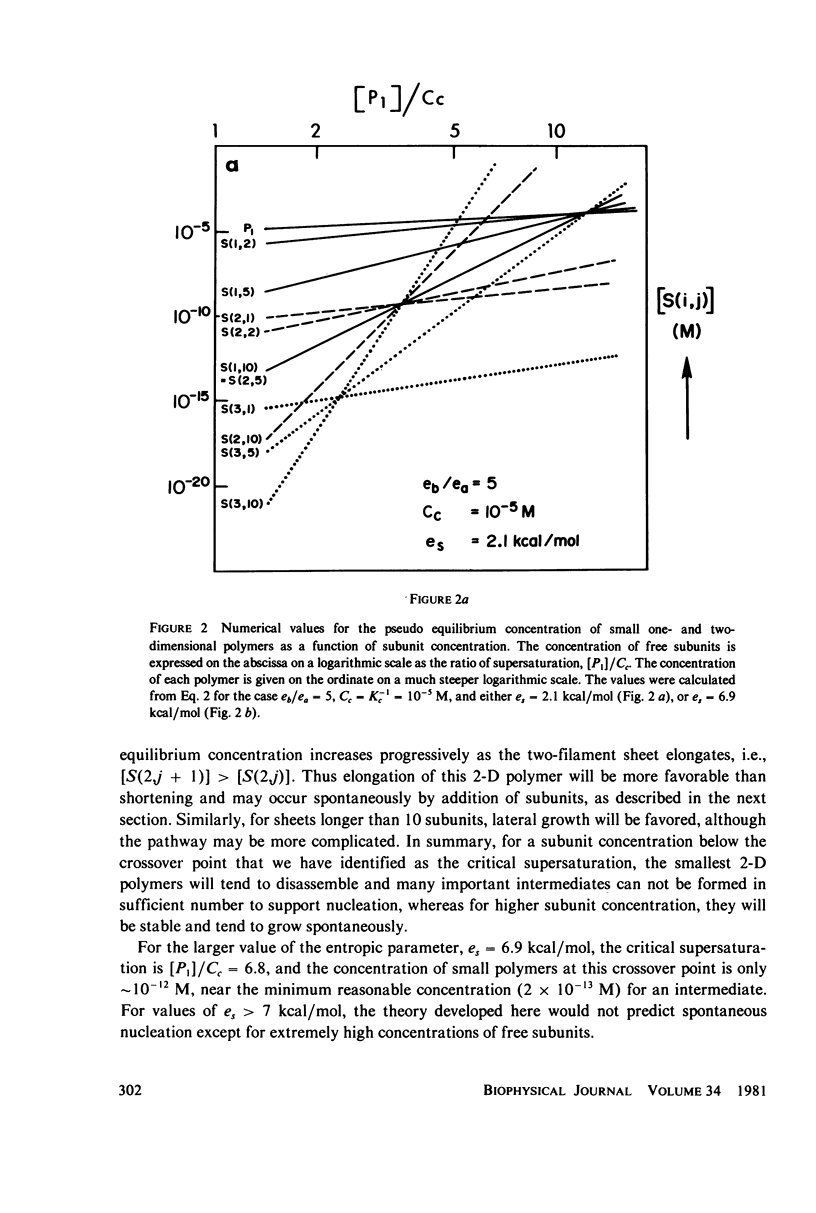
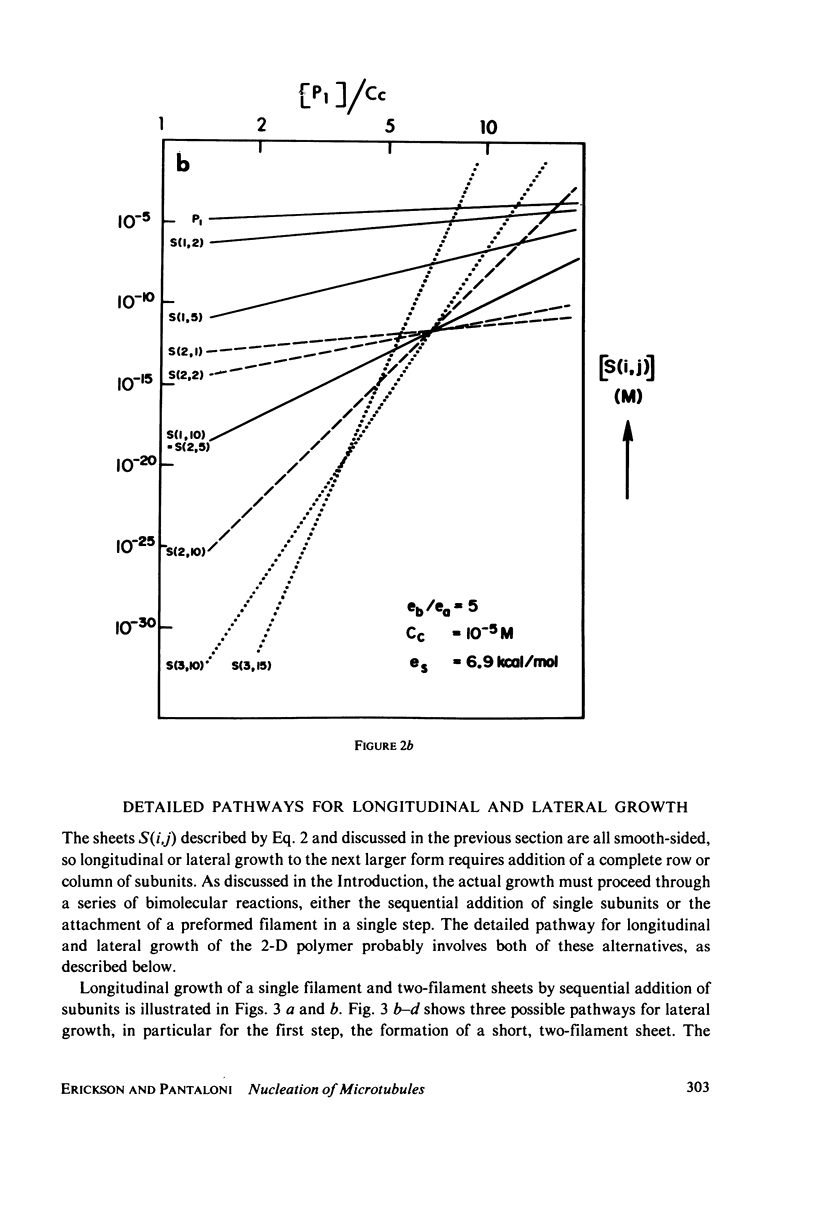
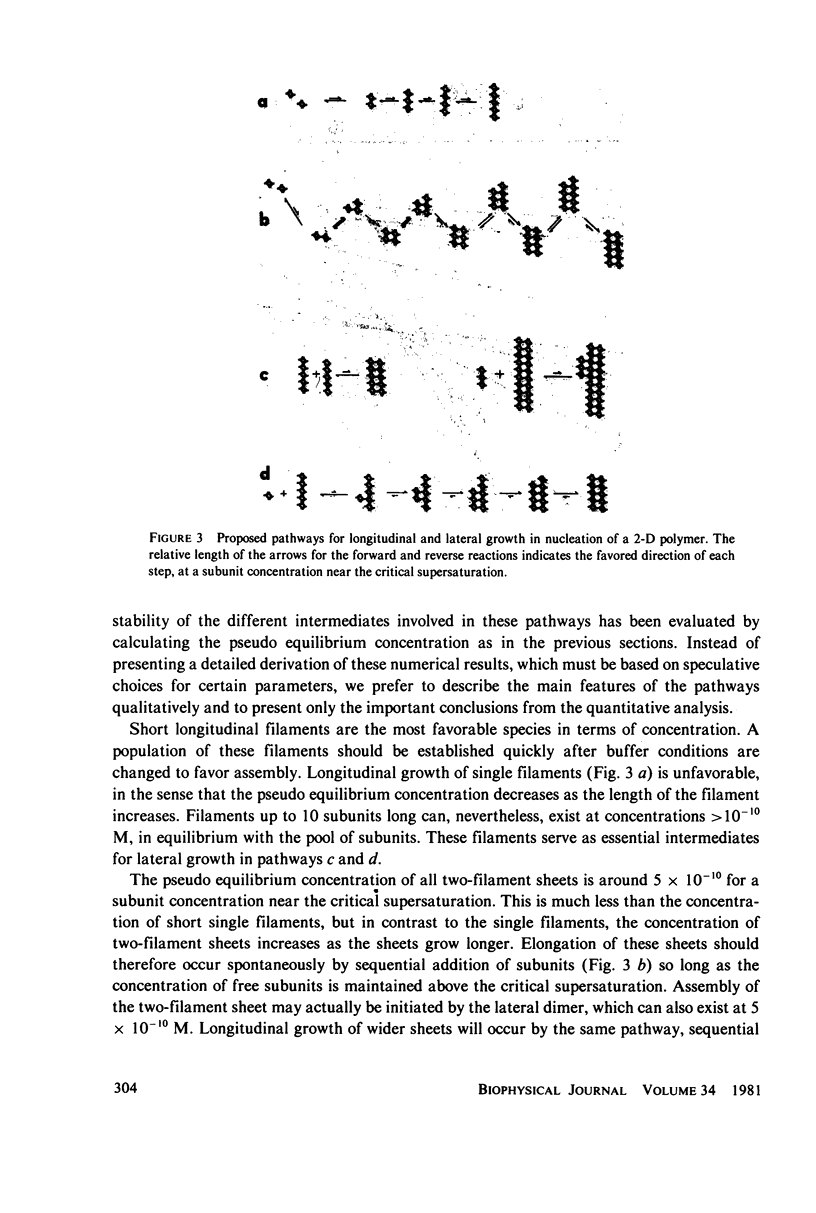
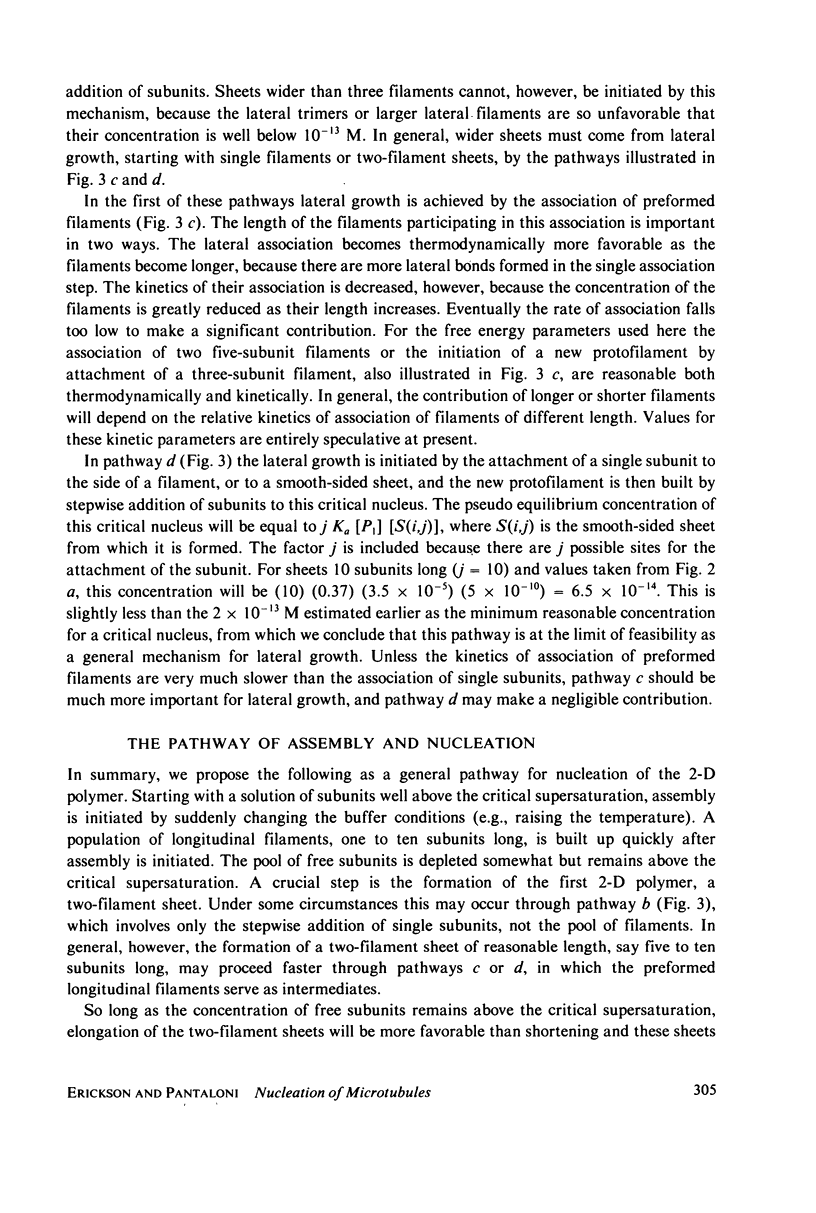
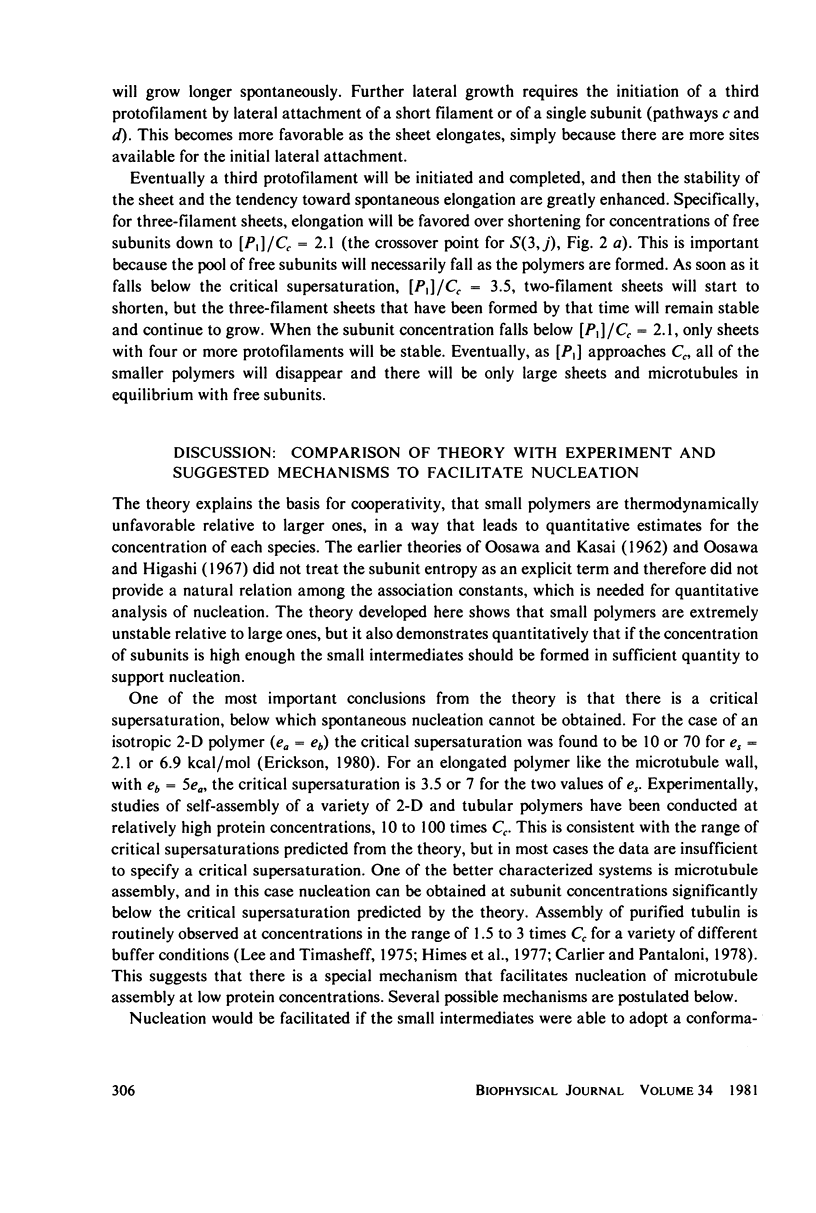
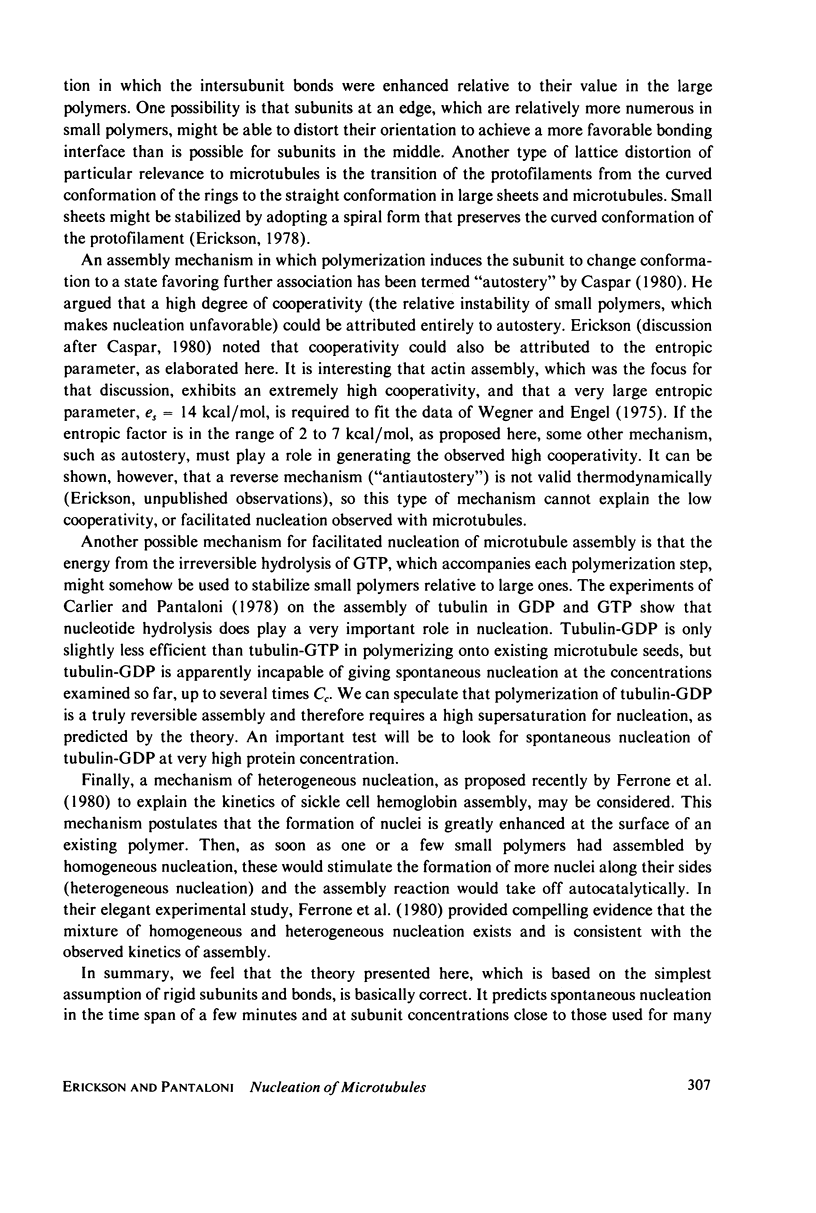
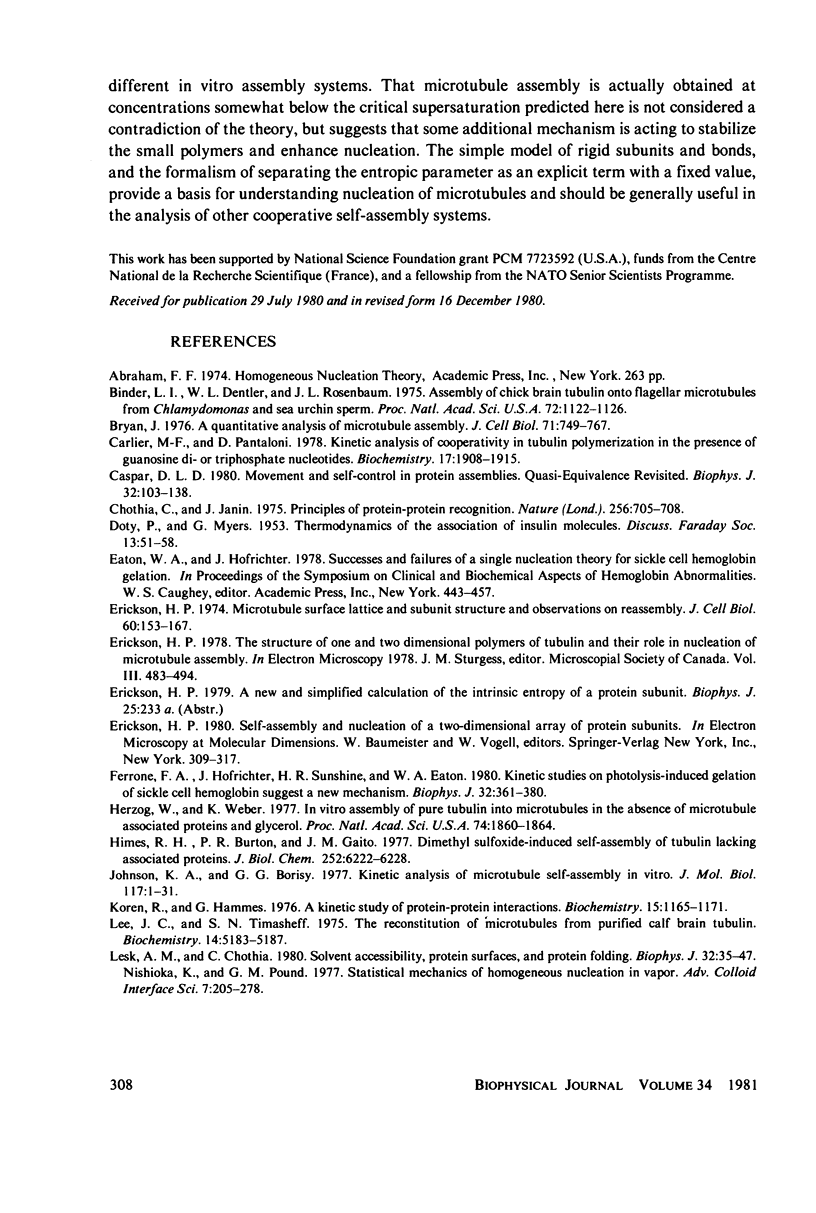
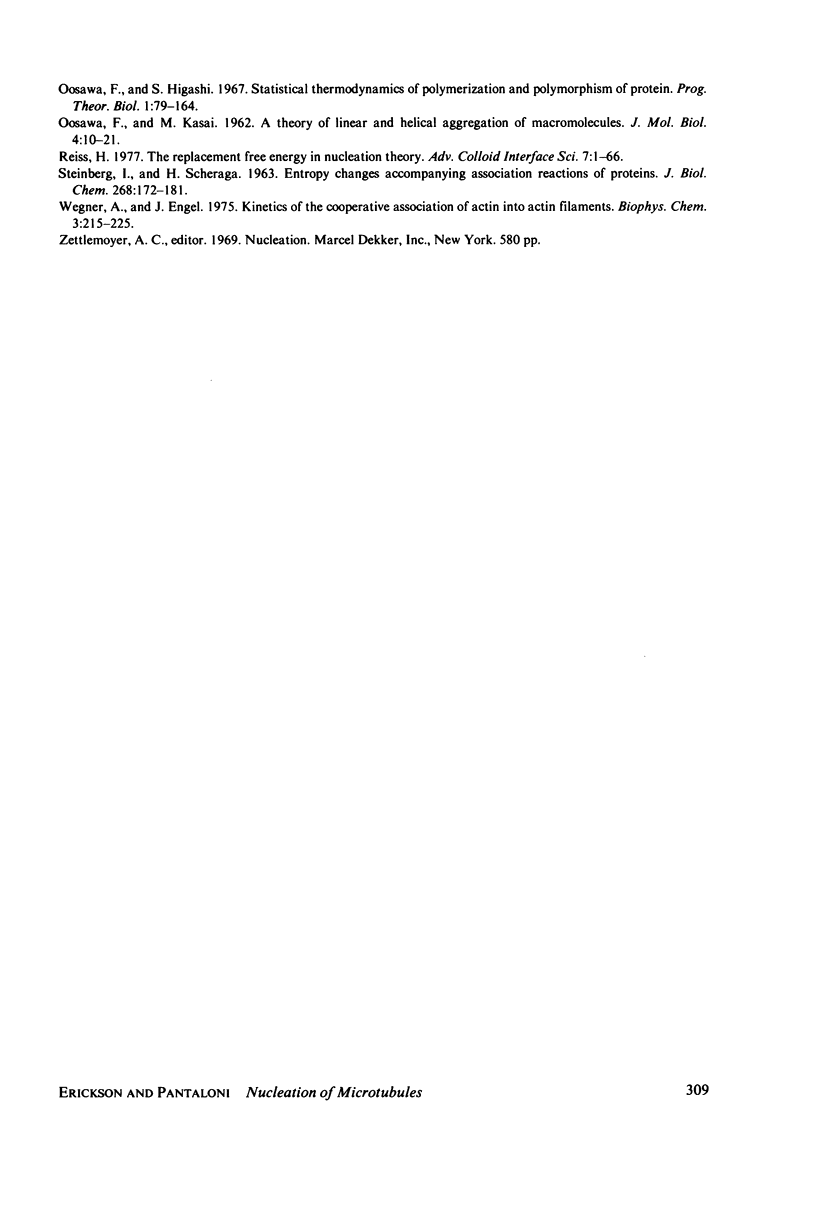
Selected References
These references are in PubMed. This may not be the complete list of references from this article.
- Binder L. I., Dentler W. L., Rosenbaum J. L. Assembly of chick brain tubulin onto flagellar microtubules from Chlamydomonas and sea urchin sperm. Proc Natl Acad Sci U S A. 1975 Mar;72(3):1122–1126. doi: 10.1073/pnas.72.3.1122. [DOI] [PMC free article] [PubMed] [Google Scholar]
- Bryan J. A quantitative analysis of microtubule elongation. J Cell Biol. 1976 Dec;71(3):749–767. doi: 10.1083/jcb.71.3.749. [DOI] [PMC free article] [PubMed] [Google Scholar]
- Carlier M. F., Pantaloni D. Kinetic analysis of cooperativity in tubulin polymerization in the presence of guanosine di- or triphosphate nucleotides. Biochemistry. 1978 May 16;17(10):1908–1915. doi: 10.1021/bi00603a017. [DOI] [PubMed] [Google Scholar]
- Caspar D. L. Movement and self-control in protein assemblies. Quasi-equivalence revisited. Biophys J. 1980 Oct;32(1):103–138. doi: 10.1016/S0006-3495(80)84929-0. [DOI] [PMC free article] [PubMed] [Google Scholar]
- Chothia C., Janin J. Principles of protein-protein recognition. Nature. 1975 Aug 28;256(5520):705–708. doi: 10.1038/256705a0. [DOI] [PubMed] [Google Scholar]
- Erickson H. P. Microtubule surface lattice and subunit structure and observations on reassembly. J Cell Biol. 1974 Jan;60(1):153–167. doi: 10.1083/jcb.60.1.153. [DOI] [PMC free article] [PubMed] [Google Scholar]
- Ferrone F. A., Hofrichter J., Sunshine H. R., Eaton W. A. Kinetic studies on photolysis-induced gelation of sickle cell hemoglobin suggest a new mechanism. Biophys J. 1980 Oct;32(1):361–380. doi: 10.1016/S0006-3495(80)84962-9. [DOI] [PMC free article] [PubMed] [Google Scholar]
- Herzog W., Weber K. In vitro assembly of pure tubulin into microtubules in the absence of microtubule-associated proteins and glycerol. Proc Natl Acad Sci U S A. 1977 May;74(5):1860–1864. doi: 10.1073/pnas.74.5.1860. [DOI] [PMC free article] [PubMed] [Google Scholar]
- Himes R. H., Burton P. R., Gaito J. M. Dimethyl sulfoxide-induced self-assembly of tubulin lacking associated proteins. J Biol Chem. 1977 Sep 10;252(17):6222–6228. [PubMed] [Google Scholar]
- Johnson K. A., Borisy G. G. Kinetic analysis of microtubule self-assembly in vitro. J Mol Biol. 1977 Nov 25;117(1):1–31. doi: 10.1016/0022-2836(77)90020-1. [DOI] [PubMed] [Google Scholar]
- Koren R., Hammes G. G. A kinetic study of protein-protein interactions. Biochemistry. 1976 Mar 9;15(5):1165–1171. doi: 10.1021/bi00650a032. [DOI] [PubMed] [Google Scholar]
- Lee J. C., Timasheff S. N. The reconstitution of microtubules from purified calf brain tubulin. Biochemistry. 1975 Nov 18;14(23):5183–5187. doi: 10.1021/bi00694a025. [DOI] [PubMed] [Google Scholar]
- Lesk A. M., Chothia C. Solvent accessibility, protein surfaces, and protein folding. Biophys J. 1980 Oct;32(1):35–47. doi: 10.1016/S0006-3495(80)84914-9. [DOI] [PMC free article] [PubMed] [Google Scholar]
- OOSAWA F., KASAI M. A theory of linear and helical aggregations of macromolecules. J Mol Biol. 1962 Jan;4:10–21. doi: 10.1016/s0022-2836(62)80112-0. [DOI] [PubMed] [Google Scholar]
- STEINBERG I. Z., SCHERAGA H. A. Entropy changes accompanying association reactions of proteins. J Biol Chem. 1963 Jan;238:172–181. [PubMed] [Google Scholar]
- Wegner A., Engel J. Kinetics of the cooperative association of actin to actin filaments. Biophys Chem. 1975 Jul;3(3):215–225. doi: 10.1016/0301-4622(75)80013-5. [DOI] [PubMed] [Google Scholar]