Abstract
We have modeled the time-course of Ca2+ binding to calmodulin, troponin, parvalbumin, and myosin in response to trains of transient increases in the free myoplasmic calcium ion concentration (pCa). A simple mathematical expression was used to describe each pCa transient, the shape and duration of which is qualitatively similar to those thought to occur in vivo. These calculations assumed that all individual metal binding sites are noninteracting and that Ca2+ bind competitively to the Ca2+-Mg2+ sites of troponin, parvalbumin, and myosin. All the on-and-off rate constants for both Ca2+ and Mg2+ were obtained either from the literature or from our own research. The percent saturation of the Ca2+-Mg2+ sites with Ca2+ was found to change very little in response to each pCa transient in the presence of 2.5 X 10(-3)M Mg2+. Our analysis suggests that the Ca2+ content of these sites is a measure of the intensity and frequency of recent muscle activity because large changes in the Ca2+ occupancy of these sites can occur with repeated stimulation. In contrast, large rapid changes in the amount of Ca2+ bound to the Ca2+-specific sites of troponin and calmodulin are induced by each pCa transient. Thus, only sites of the "Ca2+-specific" type can act as rapid Ca2+-regulatory sites in muscle. Fluctuation in the total amount of Ca2+ bound to these sites in response to various types of pCa transients further suggests that in vivo only about one-half to one-third of the total steady-state myofibrillar Ca2+-binding capacity exchanges Ca2+ during any single transient.
Full text
PDF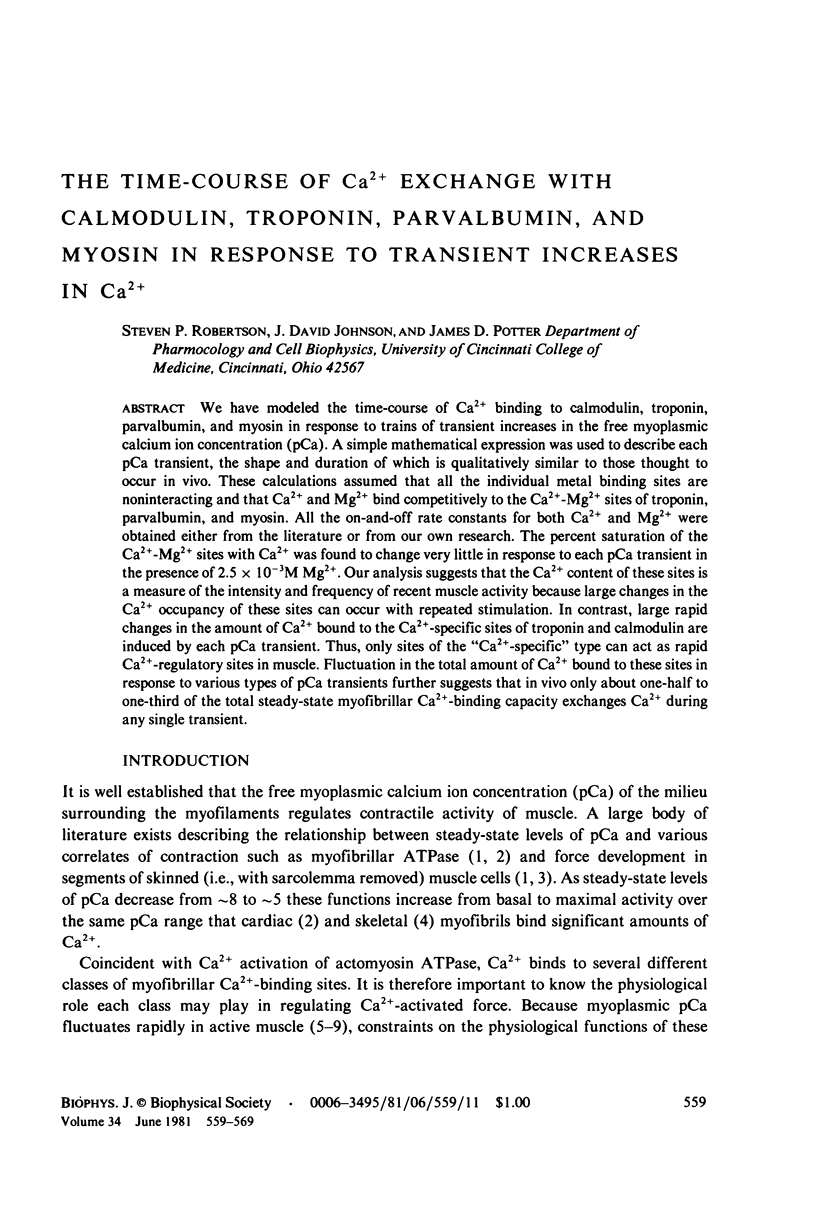
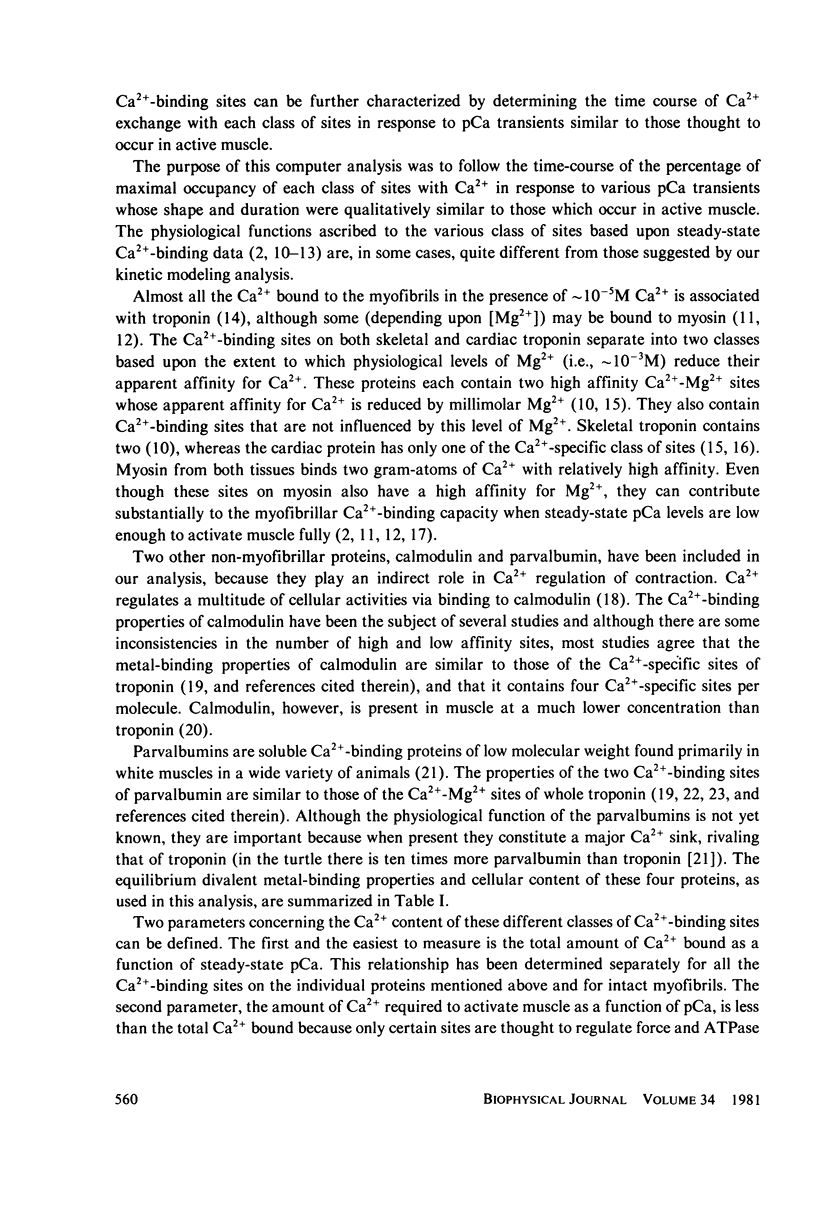
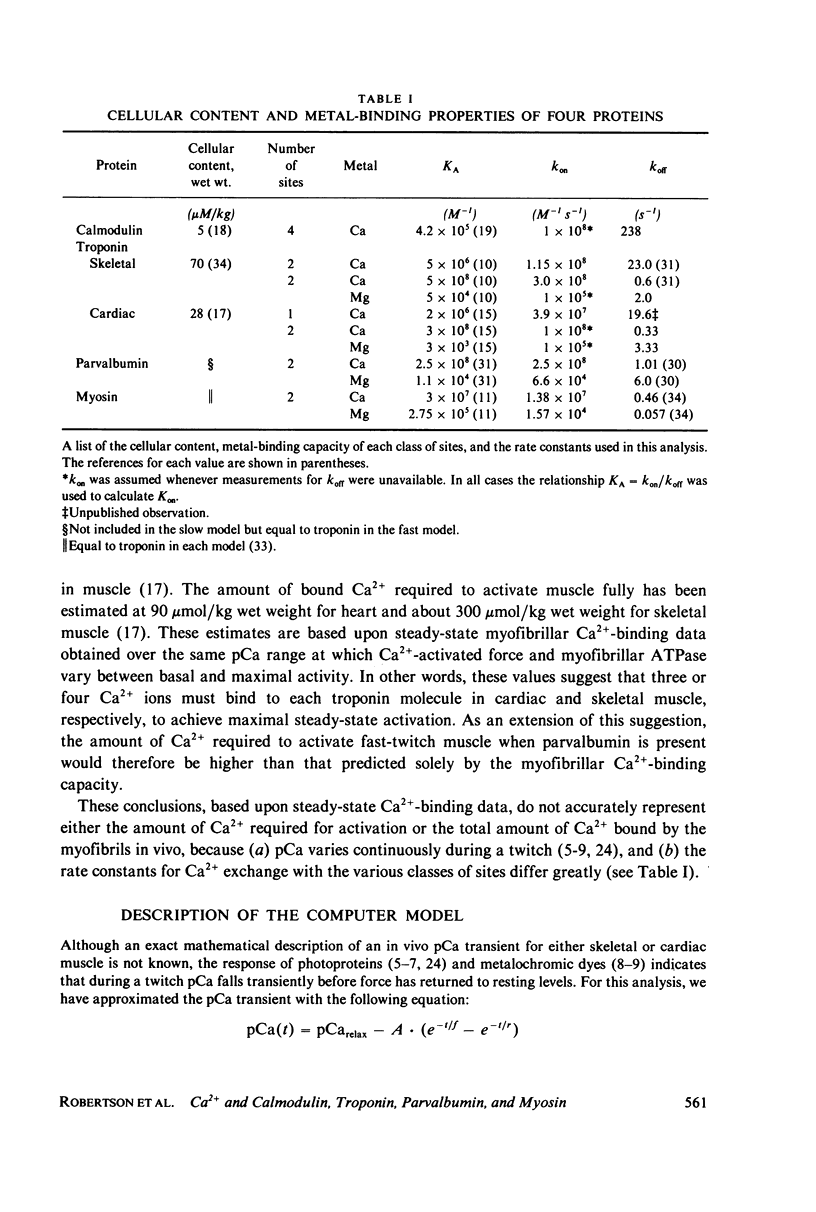
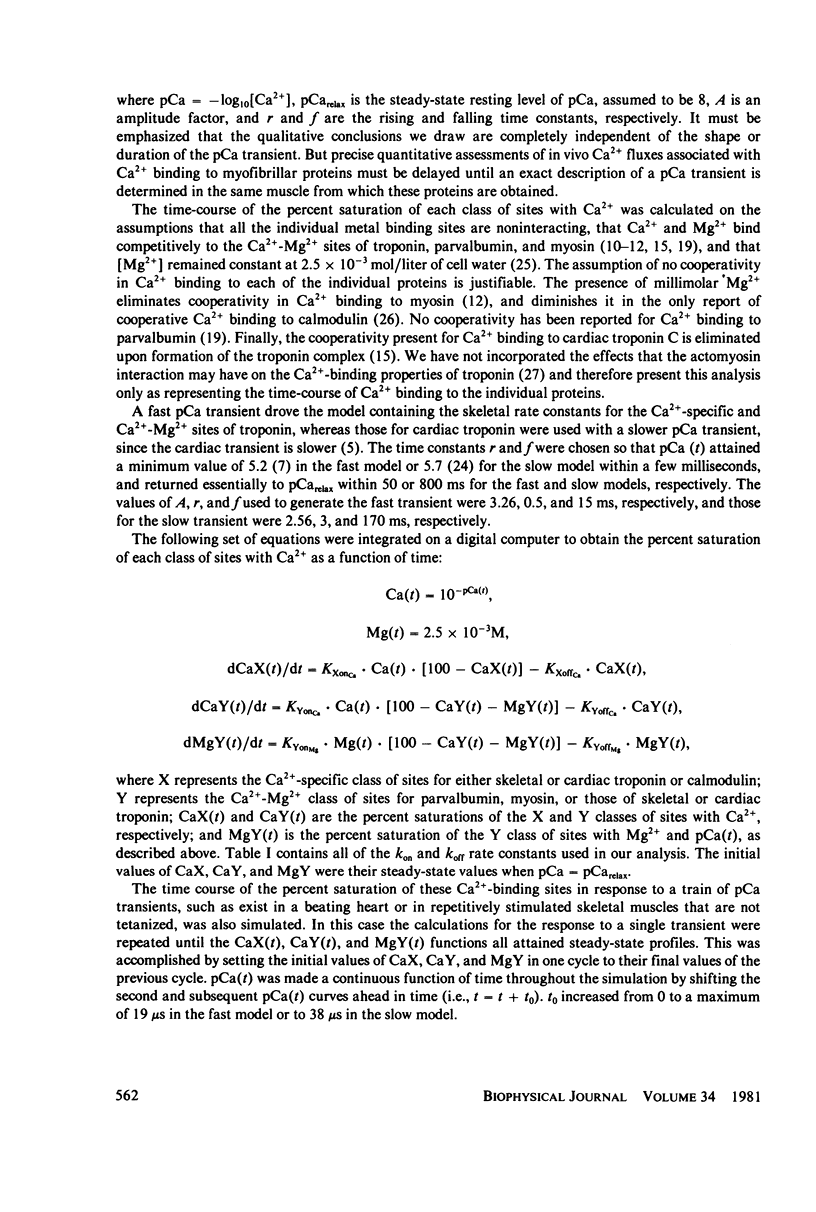
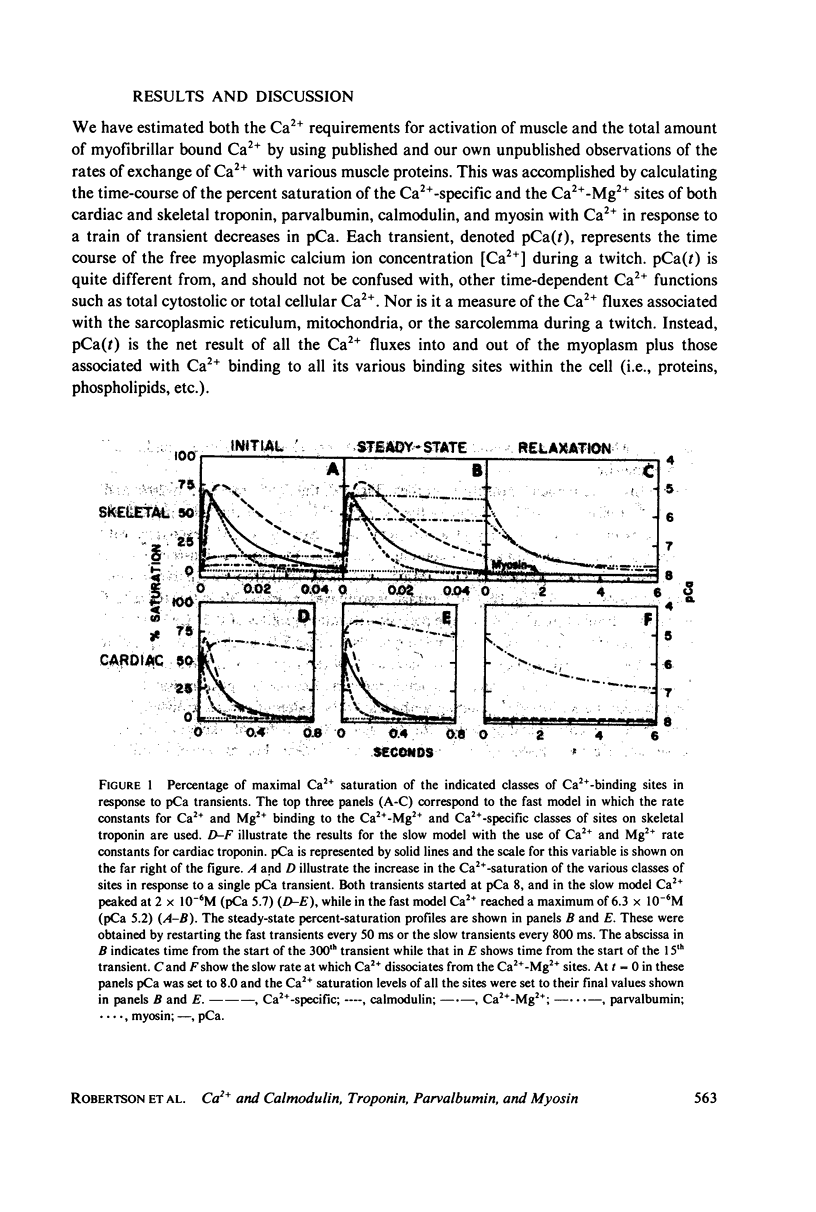
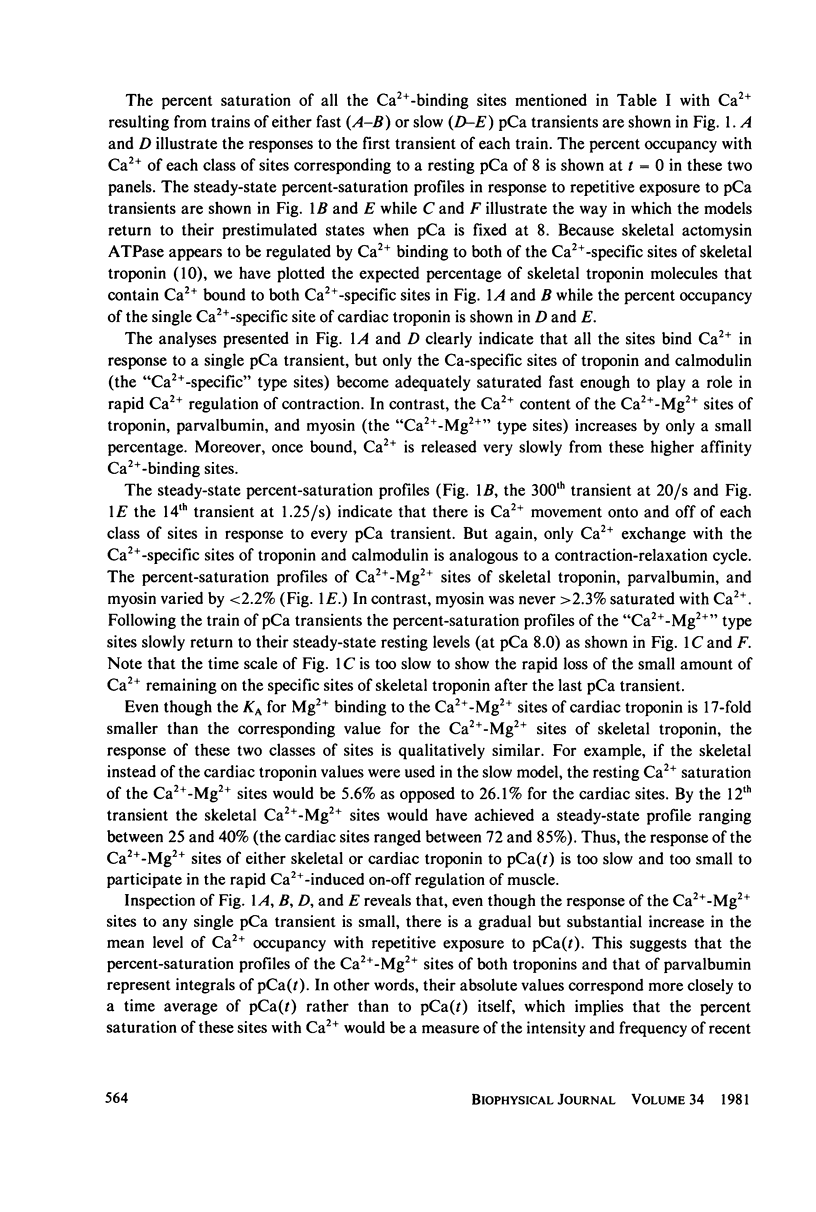
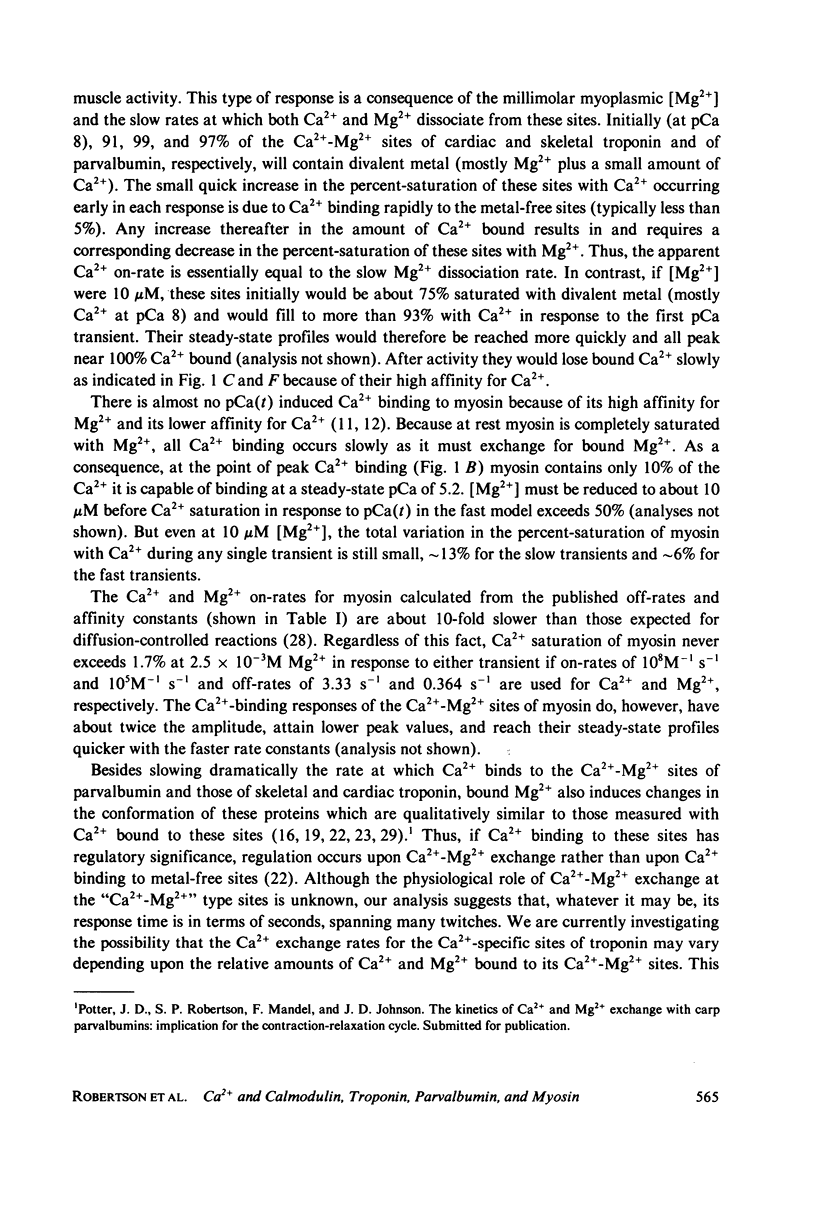
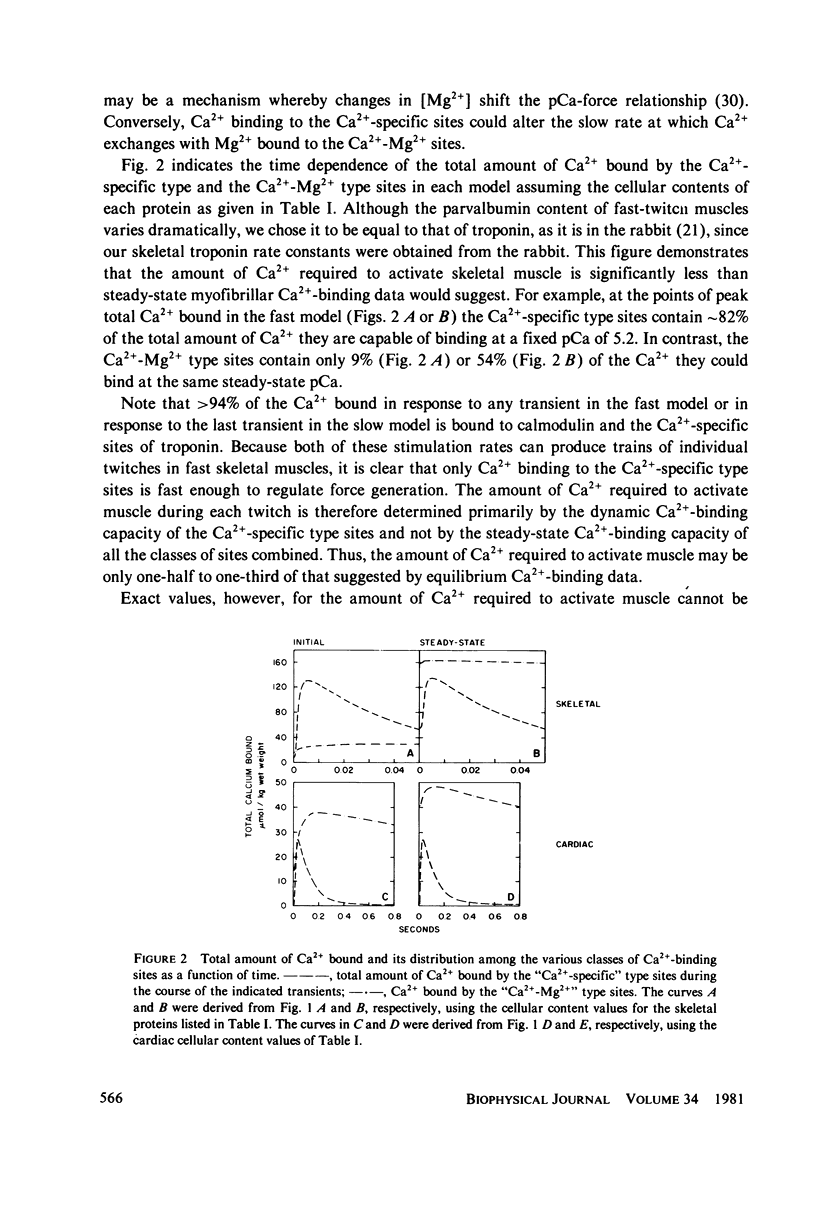
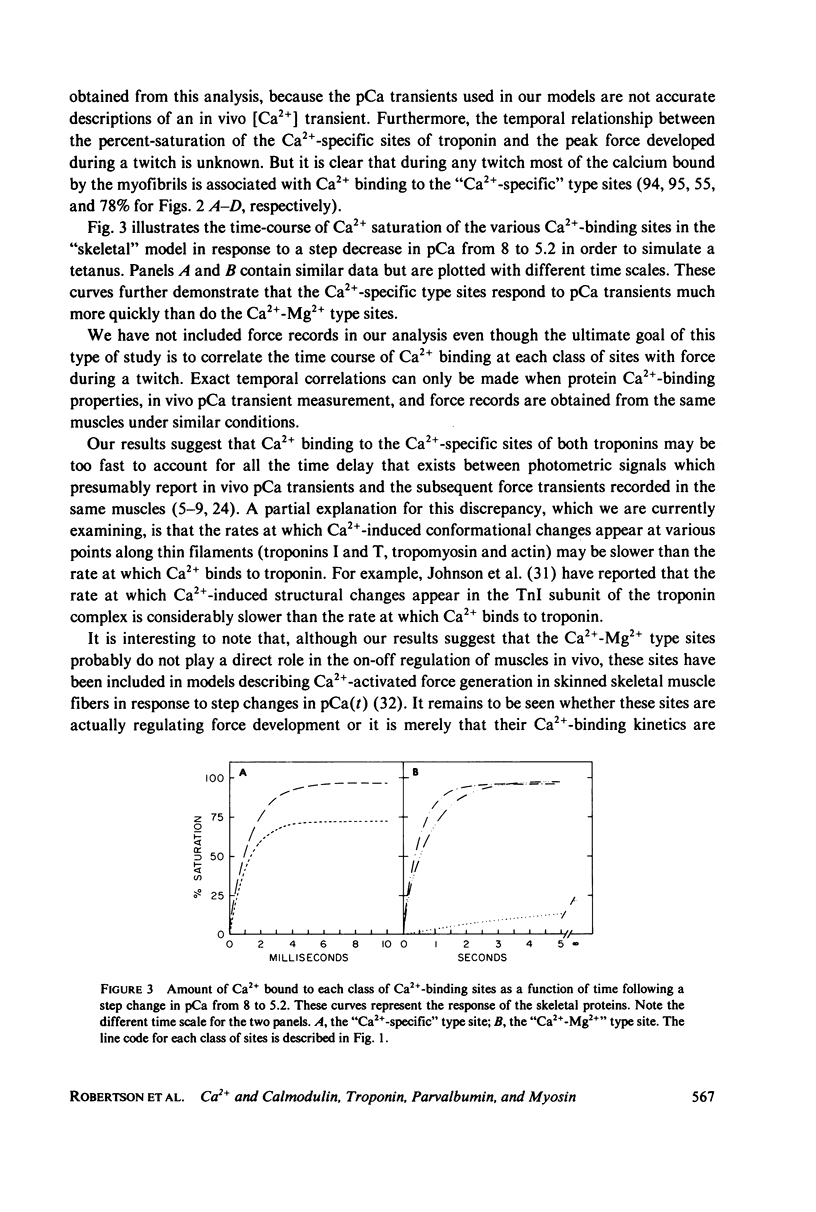
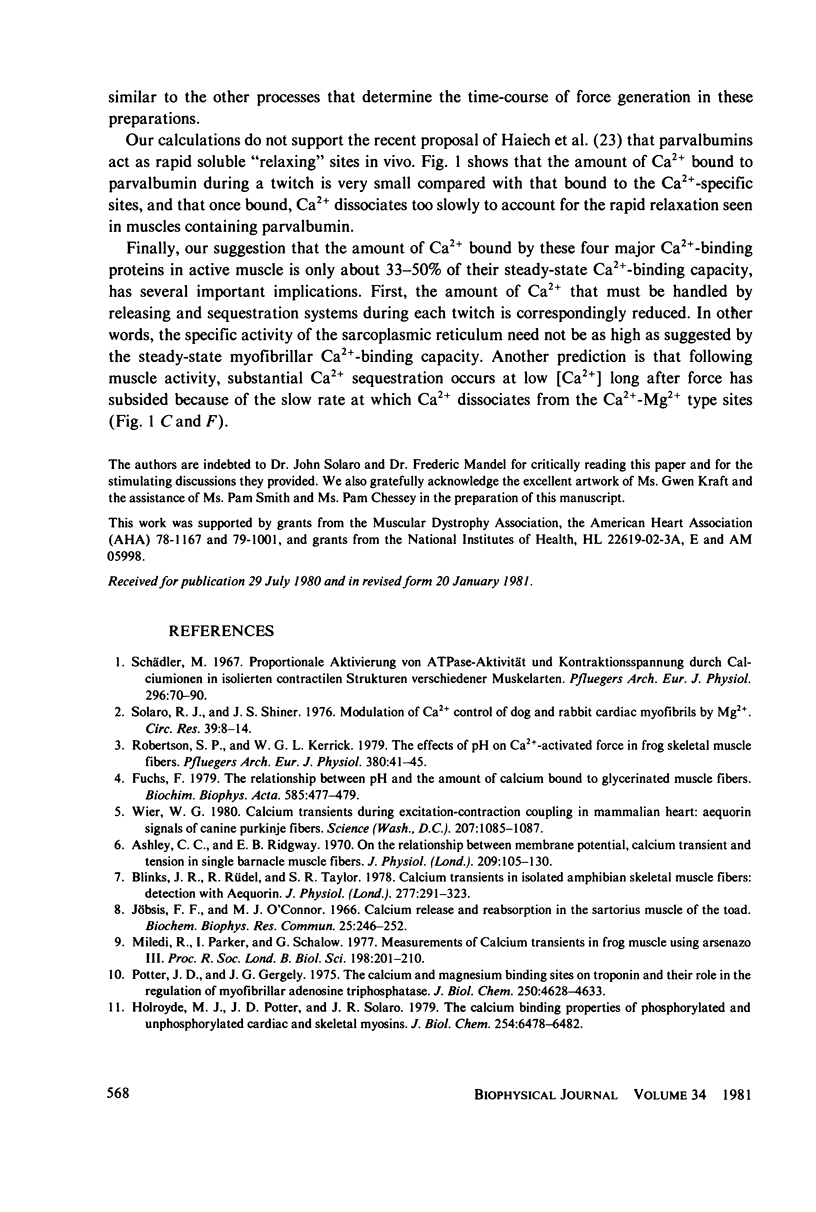
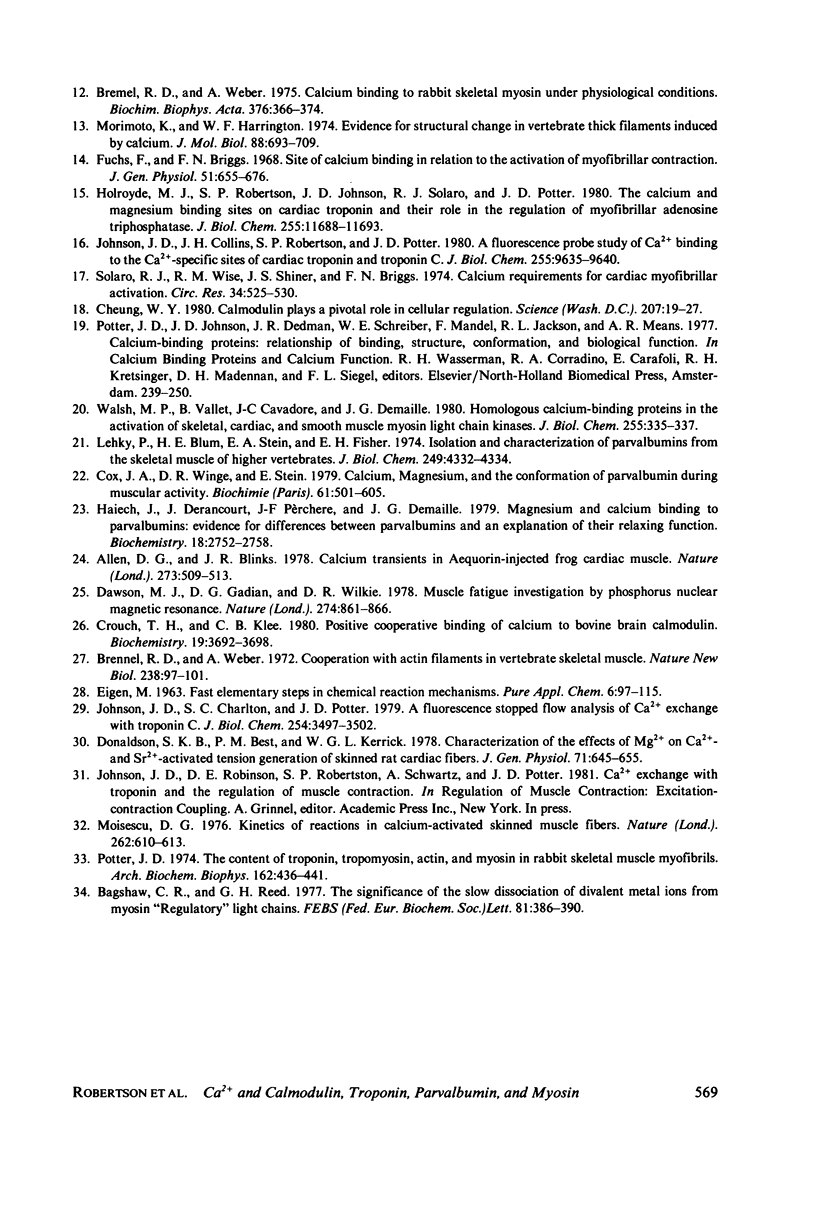
Selected References
These references are in PubMed. This may not be the complete list of references from this article.
- Allen D. G., Blinks J. R. Calcium transients in aequorin-injected frog cardiac muscle. Nature. 1978 Jun 15;273(5663):509–513. doi: 10.1038/273509a0. [DOI] [PubMed] [Google Scholar]
- Ashley C. C., Ridgway E. B. On the relationships between membrane potential, calcium transient and tension in single barnacle muscle fibres. J Physiol. 1970 Jul;209(1):105–130. doi: 10.1113/jphysiol.1970.sp009158. [DOI] [PMC free article] [PubMed] [Google Scholar]
- Bagshaw C. R., Reed G. H. The significance of the slow dissociation of divalent metal ions from myosin 'regulatory' light chains. FEBS Lett. 1977 Sep 15;81(2):386–390. doi: 10.1016/0014-5793(77)80560-7. [DOI] [PubMed] [Google Scholar]
- Blinks J. R., Rüdel R., Taylor S. R. Calcium transients in isolated amphibian skeletal muscle fibres: detection with aequorin. J Physiol. 1978 Apr;277:291–323. doi: 10.1113/jphysiol.1978.sp012273. [DOI] [PMC free article] [PubMed] [Google Scholar]
- Bremel R. D., Weber A. Calcium binding to rabbit skeletal myosin under physiological conditions. Biochim Biophys Acta. 1975 Feb 17;376(2):366–374. doi: 10.1016/0005-2728(75)90028-6. [DOI] [PubMed] [Google Scholar]
- Bremel R. D., Weber A. Cooperation within actin filament in vertebrate skeletal muscle. Nat New Biol. 1972 Jul 26;238(82):97–101. doi: 10.1038/newbio238097a0. [DOI] [PubMed] [Google Scholar]
- Cheung W. Y. Calmodulin plays a pivotal role in cellular regulation. Science. 1980 Jan 4;207(4426):19–27. doi: 10.1126/science.6243188. [DOI] [PubMed] [Google Scholar]
- Cox J. A., Winge D. R., Stein E. A. Calcium, magnesium and the conformation of parvalbumin during muscular activity. Biochimie. 1979;61(5-6):601–605. doi: 10.1016/s0300-9084(79)80157-1. [DOI] [PubMed] [Google Scholar]
- Crouch T. H., Klee C. B. Positive cooperative binding of calcium to bovine brain calmodulin. Biochemistry. 1980 Aug 5;19(16):3692–3698. doi: 10.1021/bi00557a009. [DOI] [PubMed] [Google Scholar]
- Dawson M. J., Gadian D. G., Wilkie D. R. Muscular fatigue investigated by phosphorus nuclear magnetic resonance. Nature. 1978 Aug 31;274(5674):861–866. doi: 10.1038/274861a0. [DOI] [PubMed] [Google Scholar]
- Donaldson S. K., Best P. M., Kerrick G. L. Characterization of the effects of Mg2+ on Ca2+- and Sr2+-activated tension generation of skinned rat cardiac fibers. J Gen Physiol. 1978 Jun;71(6):645–655. doi: 10.1085/jgp.71.6.645. [DOI] [PMC free article] [PubMed] [Google Scholar]
- Fuchs F., Briggs F. N. The site of calcium binding in relation to the activation of myofibrillar contraction. J Gen Physiol. 1968 May;51(5):655–676. doi: 10.1085/jgp.51.5.655. [DOI] [PMC free article] [PubMed] [Google Scholar]
- Fuchs F. The relationship between pH and the amount of calcium bound to glycerinated muscle fibers. Biochim Biophys Acta. 1979 Jul 4;585(3):477–479. doi: 10.1016/0304-4165(79)90092-8. [DOI] [PubMed] [Google Scholar]
- Haiech J., Derancourt J., Pechère J. F., Demaille J. G. Magnesium and calcium binding to parvalbumins: evidence for differences between parvalbumins and an explanation of their relaxing function. Biochemistry. 1979 Jun 26;18(13):2752–2758. doi: 10.1021/bi00580a010. [DOI] [PubMed] [Google Scholar]
- Holroyde M. J., Potter J. D., Solaro R. J. The calcium binding properties of phosphorylated and unphosphorylated cardiac and skeletal myosins. J Biol Chem. 1979 Jul 25;254(14):6478–6482. [PubMed] [Google Scholar]
- Holroyde M. J., Robertson S. P., Johnson J. D., Solaro R. J., Potter J. D. The calcium and magnesium binding sites on cardiac troponin and their role in the regulation of myofibrillar adenosine triphosphatase. J Biol Chem. 1980 Dec 25;255(24):11688–11693. [PubMed] [Google Scholar]
- Johnson J. D., Charlton S. C., Potter J. D. A fluorescence stopped flow analysis of Ca2+ exchange with troponin C. J Biol Chem. 1979 May 10;254(9):3497–3502. [PubMed] [Google Scholar]
- Johnson J. D., Collins J. H., Robertson S. P., Potter J. D. A fluorescent probe study of Ca2+ binding to the Ca2+-specific sites of cardiac troponin and troponin C. J Biol Chem. 1980 Oct 25;255(20):9635–9640. [PubMed] [Google Scholar]
- Jöbsis F. F., O'Connor M. J. Calcium release and reabsorption in the sartorius muscle of the toad. Biochem Biophys Res Commun. 1966 Oct 20;25(2):246–252. doi: 10.1016/0006-291x(66)90588-2. [DOI] [PubMed] [Google Scholar]
- Lehky P., Blum H. E., Stein E. A., Fischer E. H. Isolation and characterization of parvalbumins from the skeletal muscle of higher vertebrates. J Biol Chem. 1974 Jul 10;249(13):4332–4334. [PubMed] [Google Scholar]
- Miledi R., Parker I., Schalow G. Measurement of calcium transients in frog muscle by the use of arsenazo III. Proc R Soc Lond B Biol Sci. 1977 Aug 22;198(1131):201–210. doi: 10.1098/rspb.1977.0094. [DOI] [PubMed] [Google Scholar]
- Moisescu D. G. Kinetics of reaction in calcium-activated skinned muscle fibres. Nature. 1976 Aug 12;262(5569):610–613. doi: 10.1038/262610a0. [DOI] [PubMed] [Google Scholar]
- Morimoto K., Harrington W. F. Evidence for structural changes in vertebrate thick filaments induced by calcium. J Mol Biol. 1974 Sep 25;88(3):693–709. doi: 10.1016/0022-2836(74)90417-3. [DOI] [PubMed] [Google Scholar]
- Potter J. D., Gergely J. The calcium and magnesium binding sites on troponin and their role in the regulation of myofibrillar adenosine triphosphatase. J Biol Chem. 1975 Jun 25;250(12):4628–4633. [PubMed] [Google Scholar]
- Potter J. D. The content of troponin, tropomyosin, actin, and myosin in rabbit skeletal muscle myofibrils. Arch Biochem Biophys. 1974 Jun;162(2):436–441. doi: 10.1016/0003-9861(74)90202-1. [DOI] [PubMed] [Google Scholar]
- Robertson S. P., Kerrick W. G. The effects of pH on Ca2+-activated force in frog skeletal muscle fibers. Pflugers Arch. 1979 May 15;380(1):41–45. doi: 10.1007/BF00582610. [DOI] [PubMed] [Google Scholar]
- Schädler M. Proportional Aktivierung von ATPase-Aktivität und Kontraktionsspannung durch Calciumionen in isolierten contractilen Strukturen verschiedener Muskelarten. Pflugers Arch Gesamte Physiol Menschen Tiere. 1967;296(1):70–90. [PubMed] [Google Scholar]
- Solaro R. J., Shiner J. S. Modulation of Ca2+ control of dog and rabbit cardiac myofibrils by Mg2+. Comparison with rabbit skeletal myofibrils. Circ Res. 1976 Jul;39(1):8–14. doi: 10.1161/01.res.39.1.8. [DOI] [PubMed] [Google Scholar]
- Solaro R. J., Wise R. M., Shiner J. S., Briggs F. N. Calcium requirements for cardiac myofibrillar activation. Circ Res. 1974 Apr;34(4):525–530. doi: 10.1161/01.res.34.4.525. [DOI] [PubMed] [Google Scholar]
- Walsh M. P., Vallet B., Cavadore J. C., Demaille J. G. Homologous calcium-binding proteins in the activation of skeletal, cardiac, and smooth muscle myosin light chain kinases. J Biol Chem. 1980 Jan 25;255(2):335–337. [PubMed] [Google Scholar]
- Wier W. G. Calcium transients during excitation-contraction coupling in mammalian heart: aequorin signals of canine Purkinje fibers. Science. 1980 Mar 7;207(4435):1085–1087. doi: 10.1126/science.7355274. [DOI] [PubMed] [Google Scholar]