Abstract
Silverman et al. (1979. J. Am. Chem. Soc. 101:6734-6740) have reported measurements of the loss of 18O to solvent from the isotopically labeled CO2--HCO3-system and of the mixing of 18O and 13C labels within the system, as catalyzed by human carbonic anhydrase C in the pH range 6-8. This work is an extension of earlier work (Silverman and Tu. 1976. J. Am. Chem. Soc. 98:978-984) on the very similar bovine enzyme. The more recent work is analyzed by its authors in terms of the "hydroxide" model for the apparent pH-dependence of enzymatic activity, a model in which the pH-dependence is associated with the presumed ionization of an H2O ligand of the active-site metal ion to OH-. From a comparison of their data with a solution of the coupled differential equations that describe the kinetics of isotope exchange in terms of the model, Silverman et al. derived a pH-dependent rate of exchange for the water molecule which is formed at the active site of the enzyme during dehydration. By contrast, using the same data and a model in which active enzyme has a water molecule on the metal ion at the active site, and similar differential equations, we derive a value for the rate of exchange of water that is pH-independent. This model has the attraction that it explains the magnetic relaxation rate of solvent water protons in the Co2+-substituted enzyme, whereas the hydroxide mechanism cannot explain these data without the introduction of unfounded ad hoc assumptions; further, the presence of an OH- ligand of the metal has never been demonstrated. We also include an analysis of analogous data for the bovine enzyme. One result of our analysis is that the pKa for activity of the enzyme samples used is near 6.0, implying that the bulk of the data were taken when the enzyme was essentially all active. It is straightforward to account for the pH-dependence of the data near and below the pKa by using an empirically-derived value for the pKa. However, we have recently developed a model for the low pH (inactive) enzyme that has been successful in interpreting a wide range of data, and we show that this new view can explain the few points at low pH quite adequately. Additionally, we consider the recent kinetic results for the human C enzyme, obtained at chemical equilibrium by studies of the linewidths of nuclear magnetic resonances of 13C in labeled substrate (Simonsson et al. 1979. Eur. J. Biochem. 93:409-417) and show that these experiments and those of Silverman et al. are all consistent with kinetic data from nonequilibrium stopped-flow experiments, viewed in terms of our model, in the limit of low substrate concentration. Results at higher concentrations indicate that the Michaelis constants and equilibrium constants differ somewhat.
Full text
PDF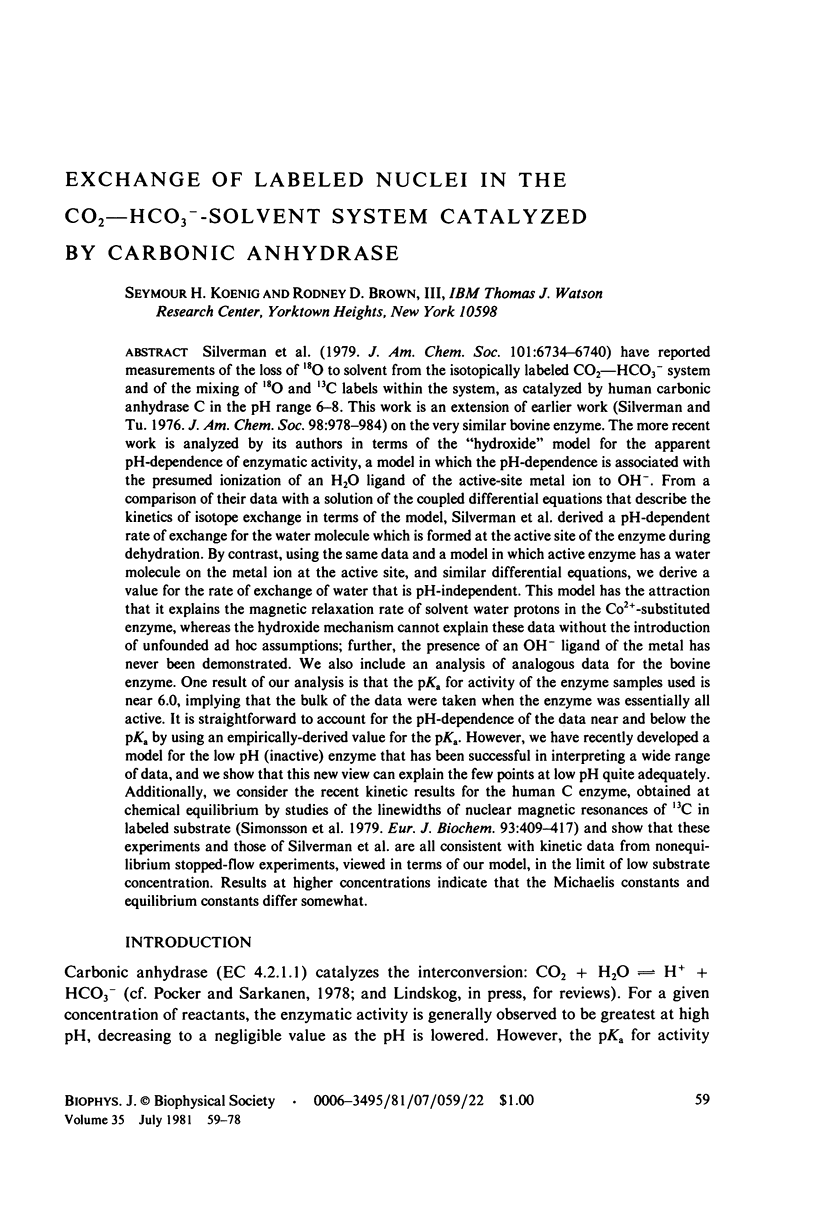
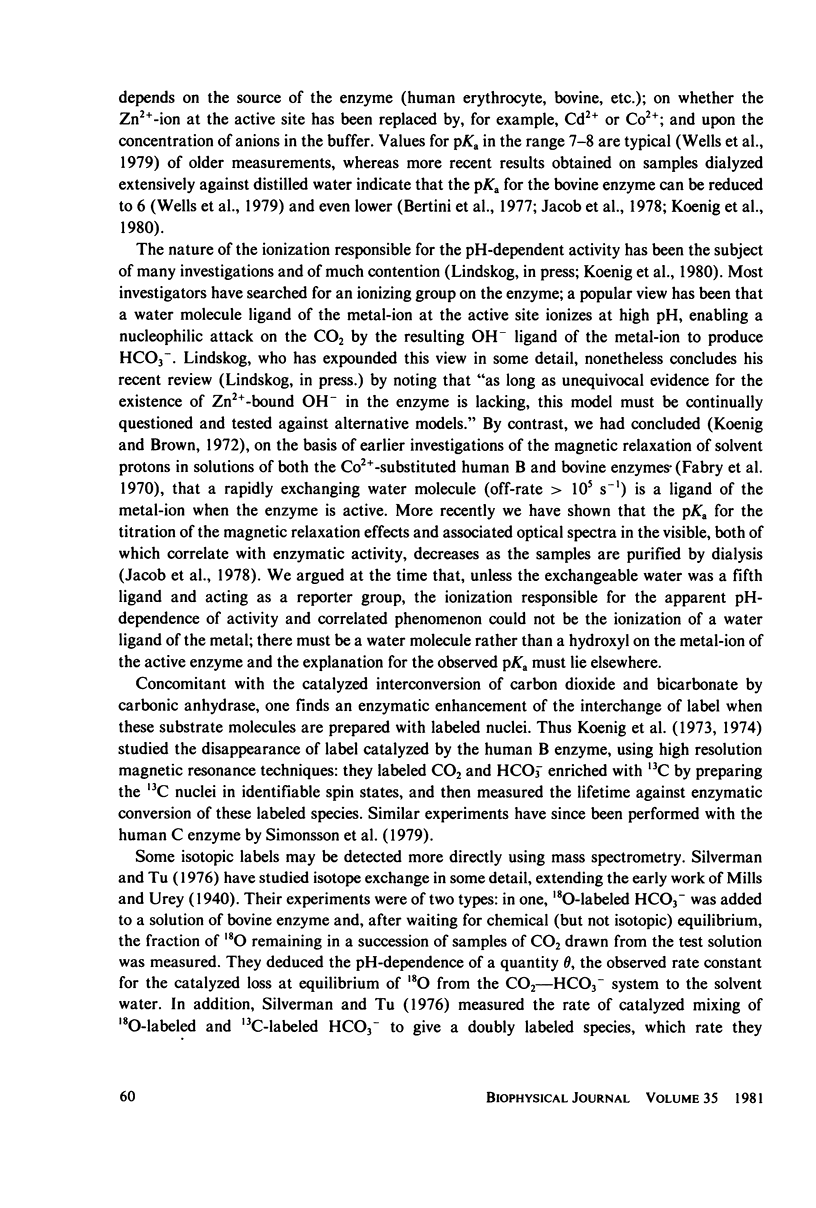
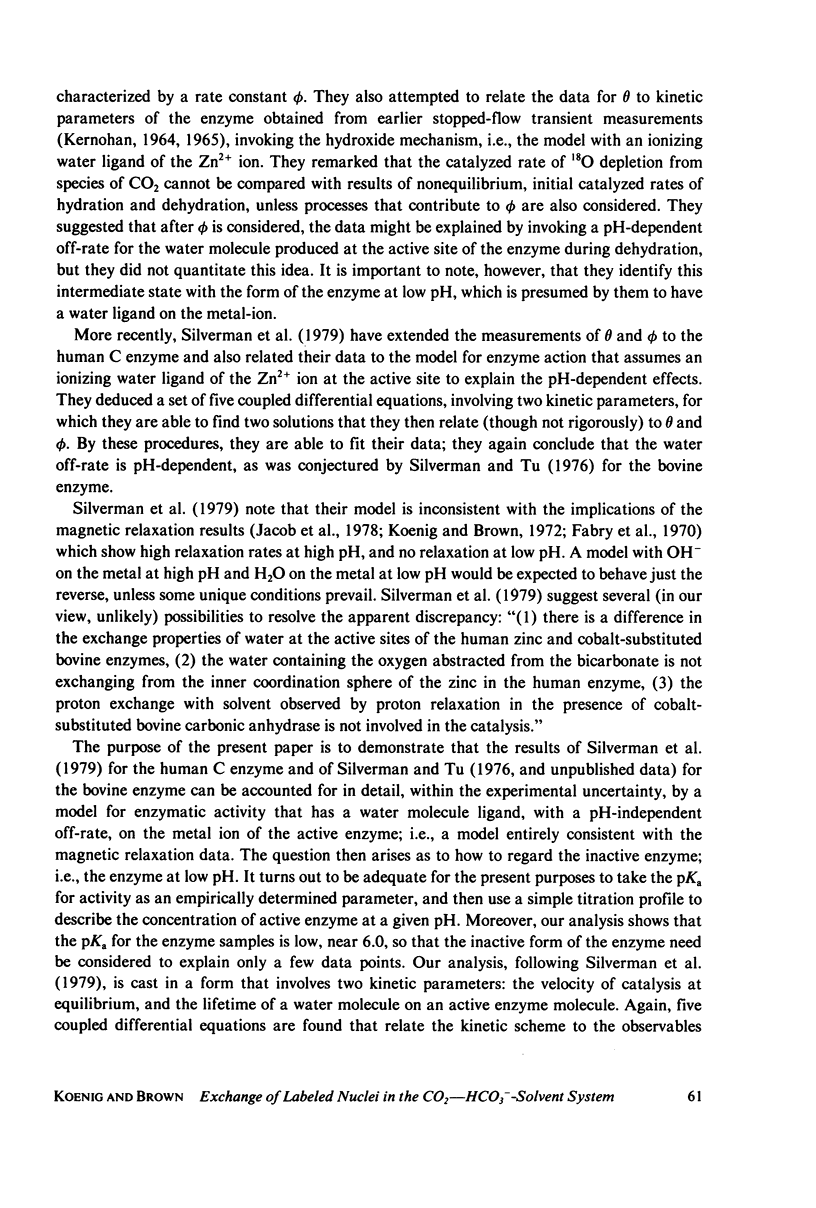
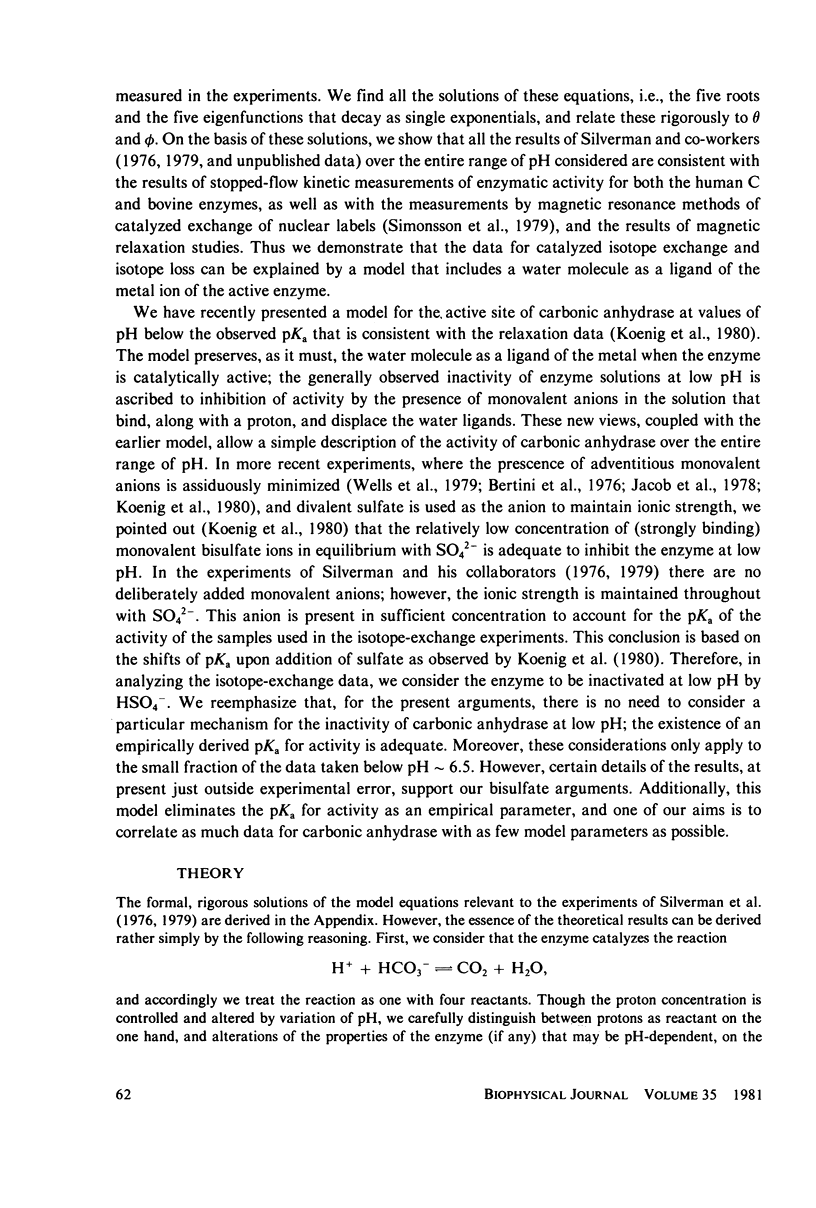
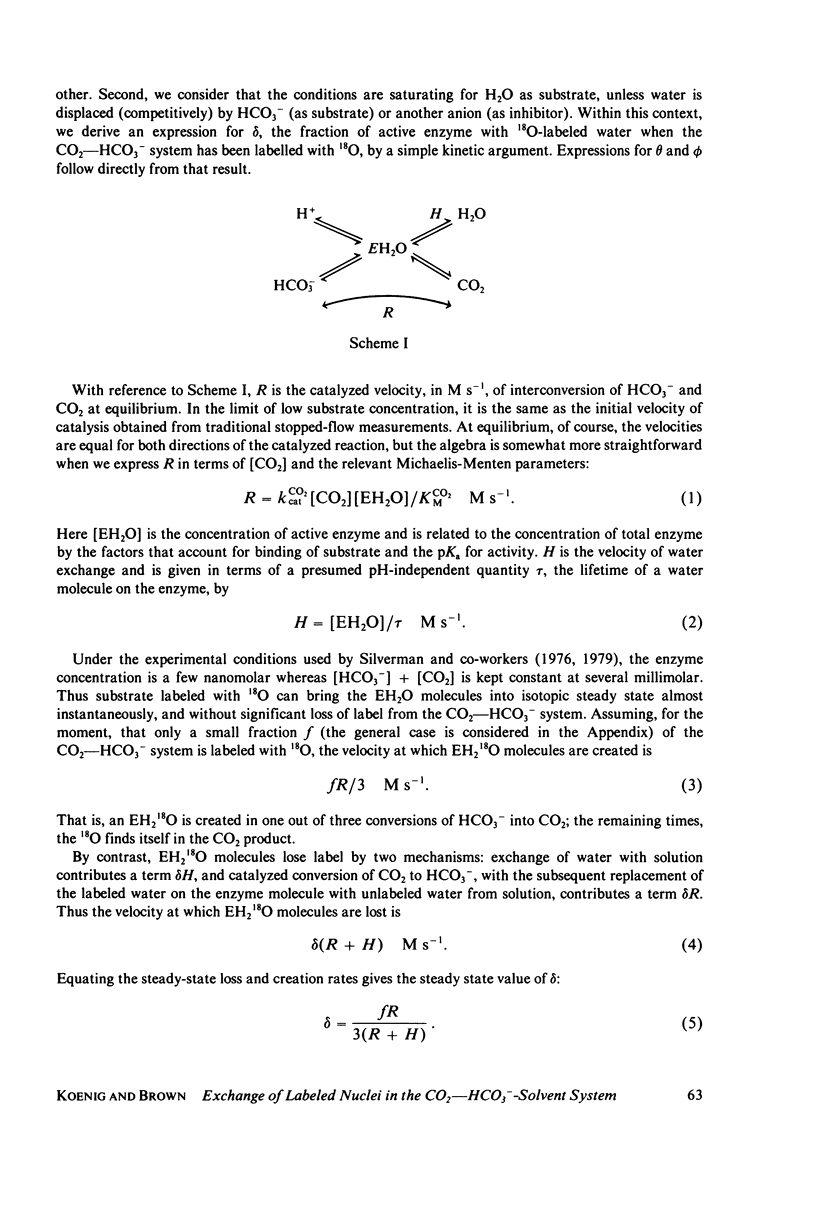
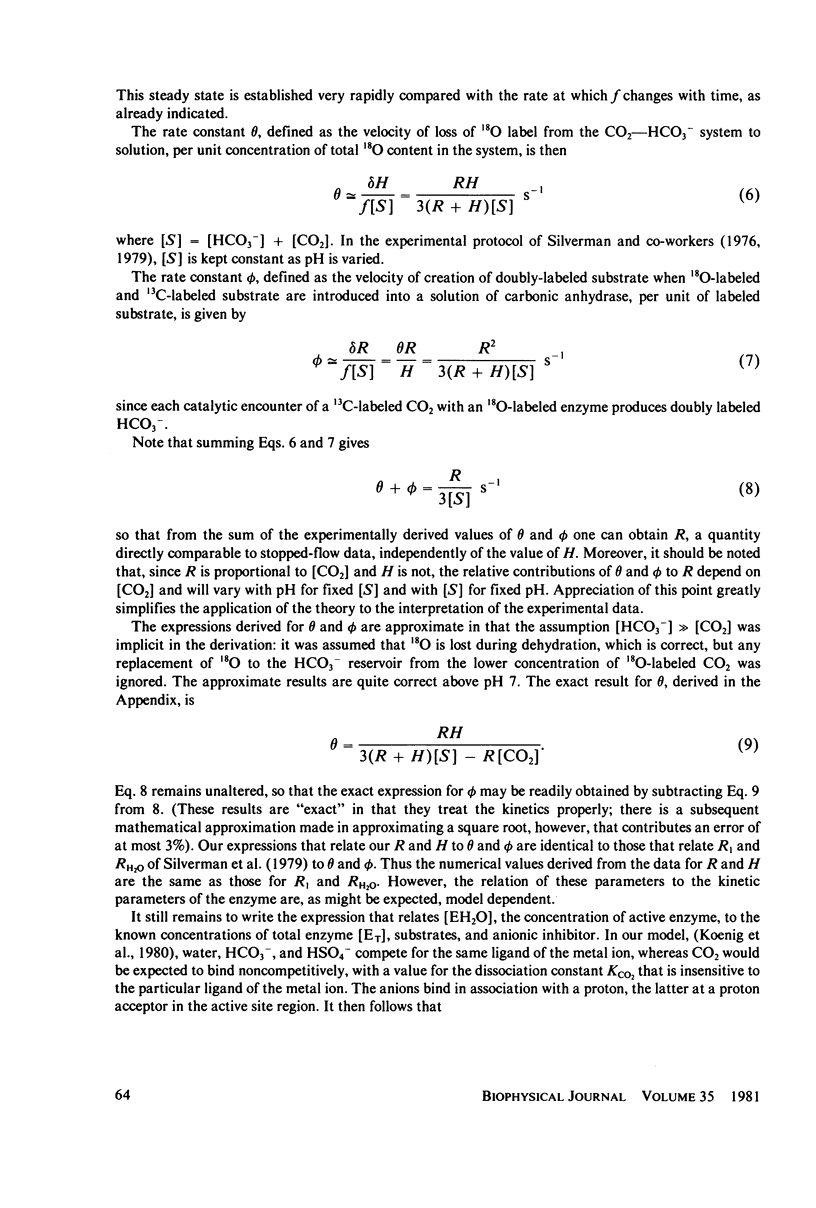
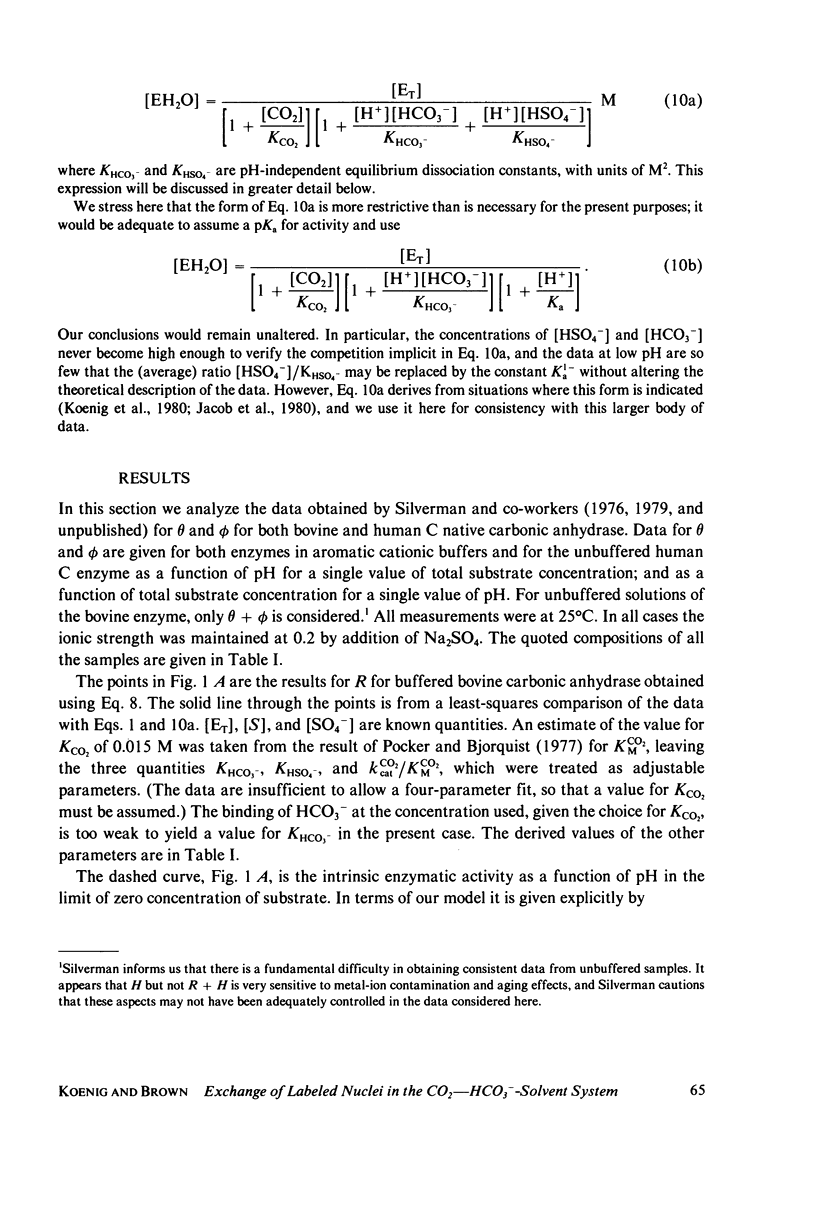
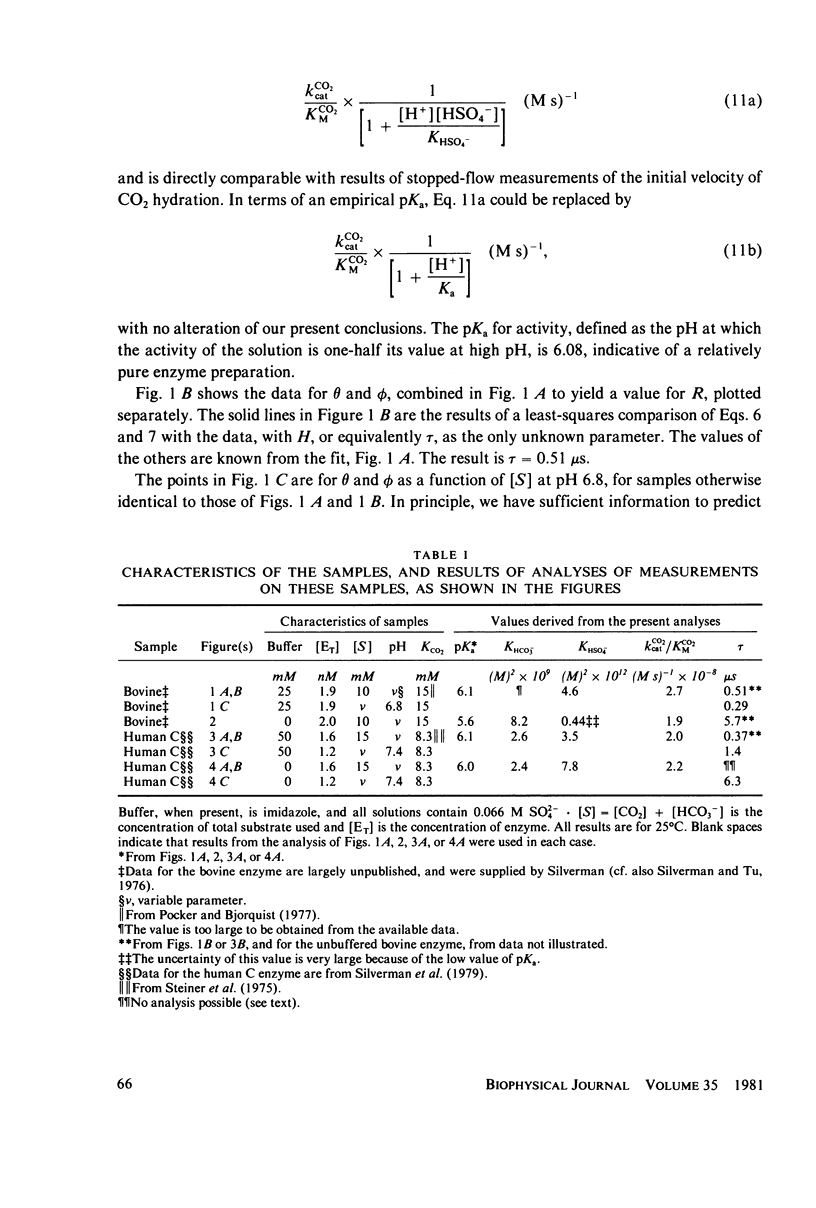
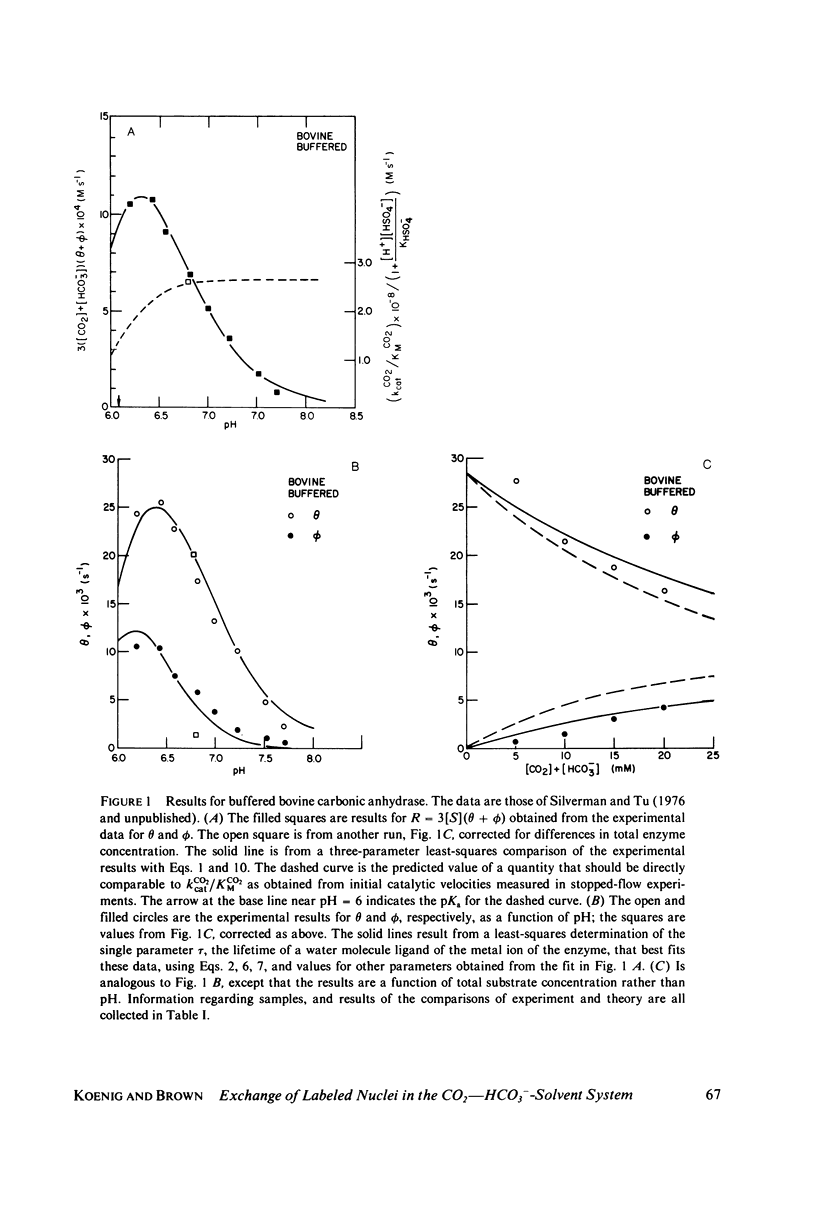
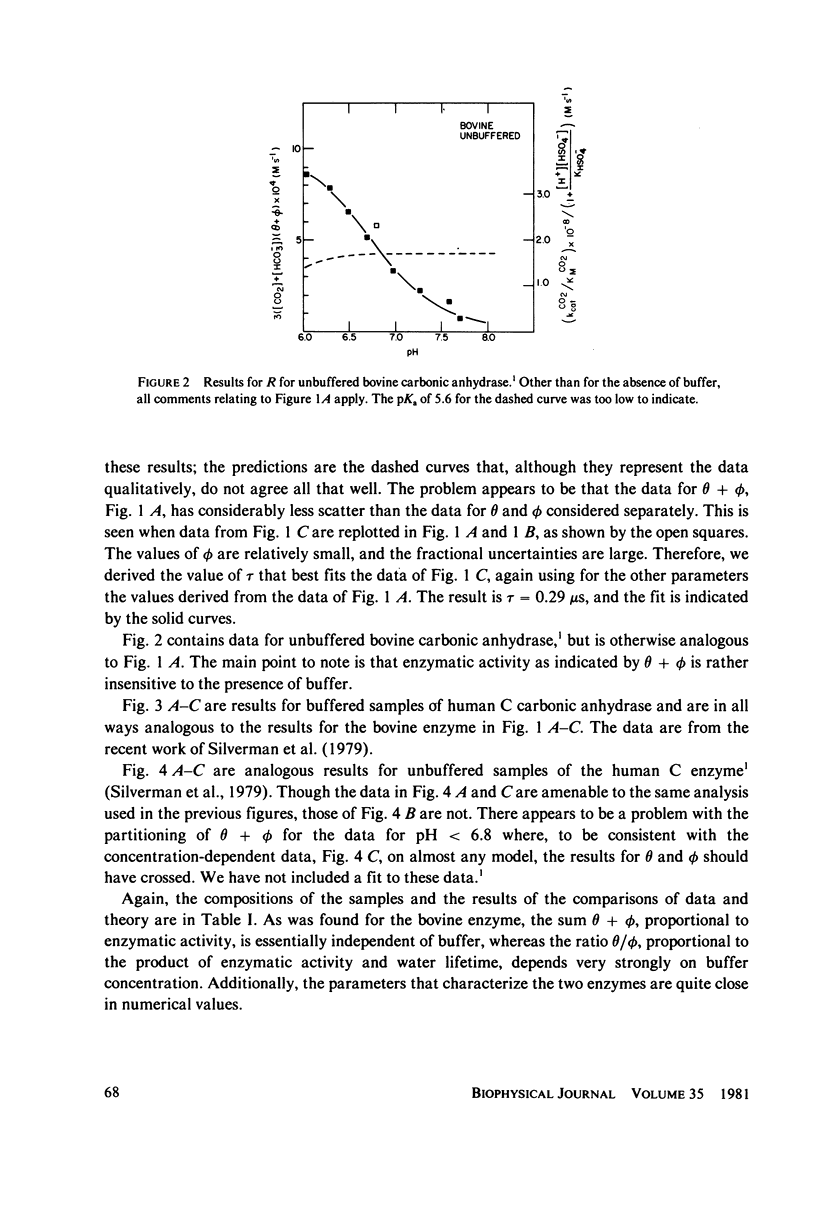
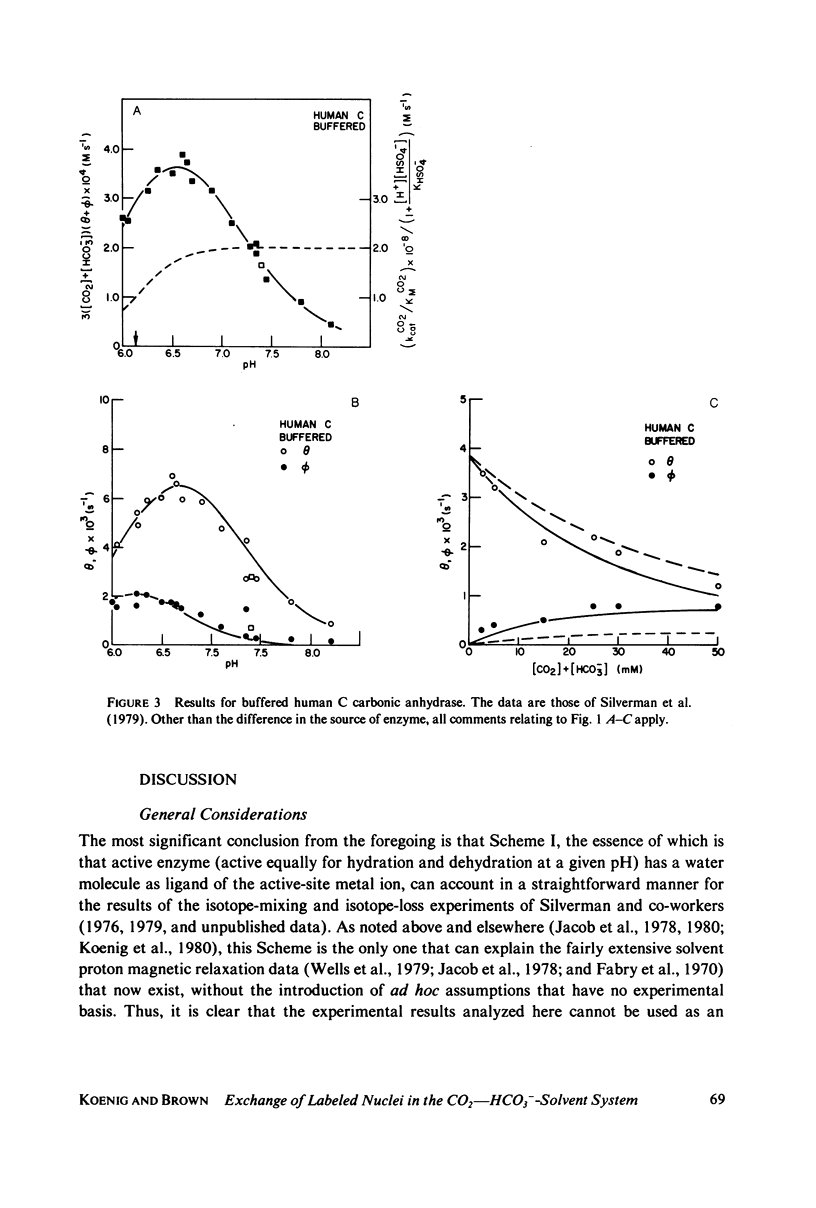
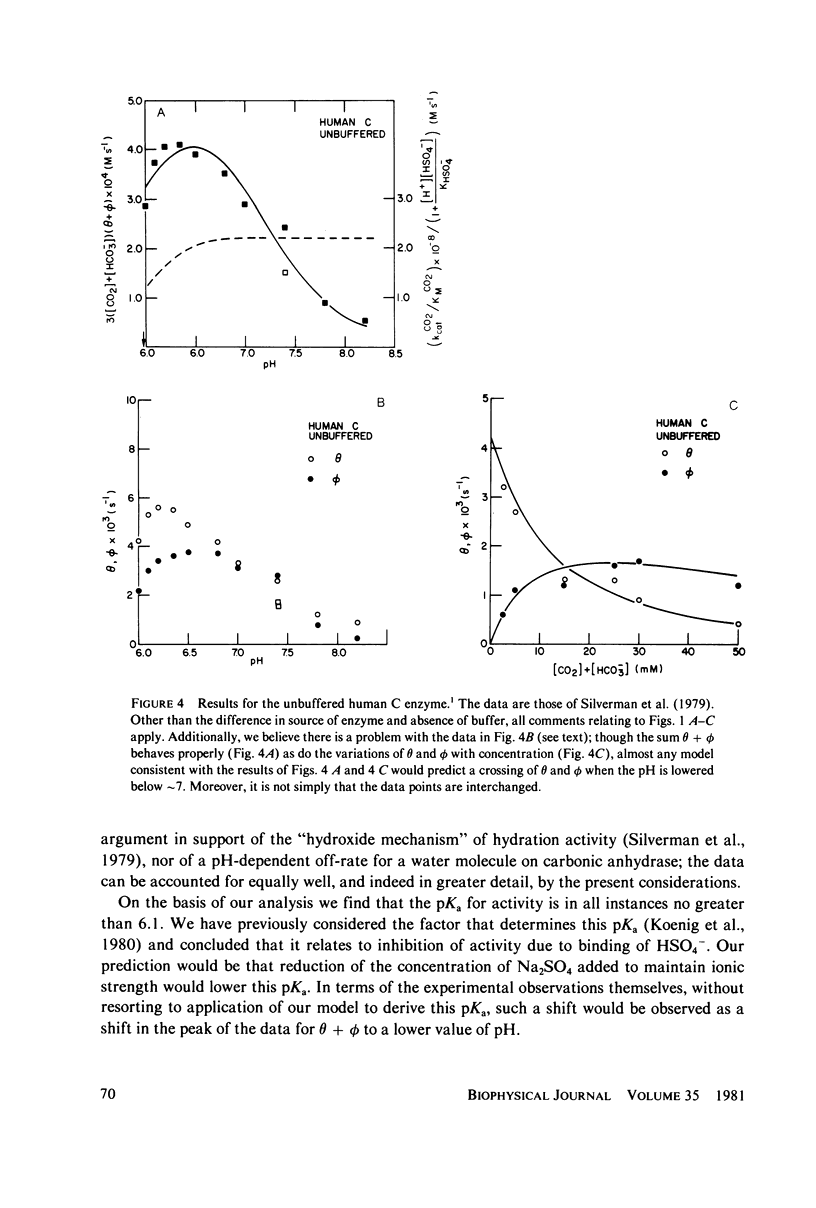
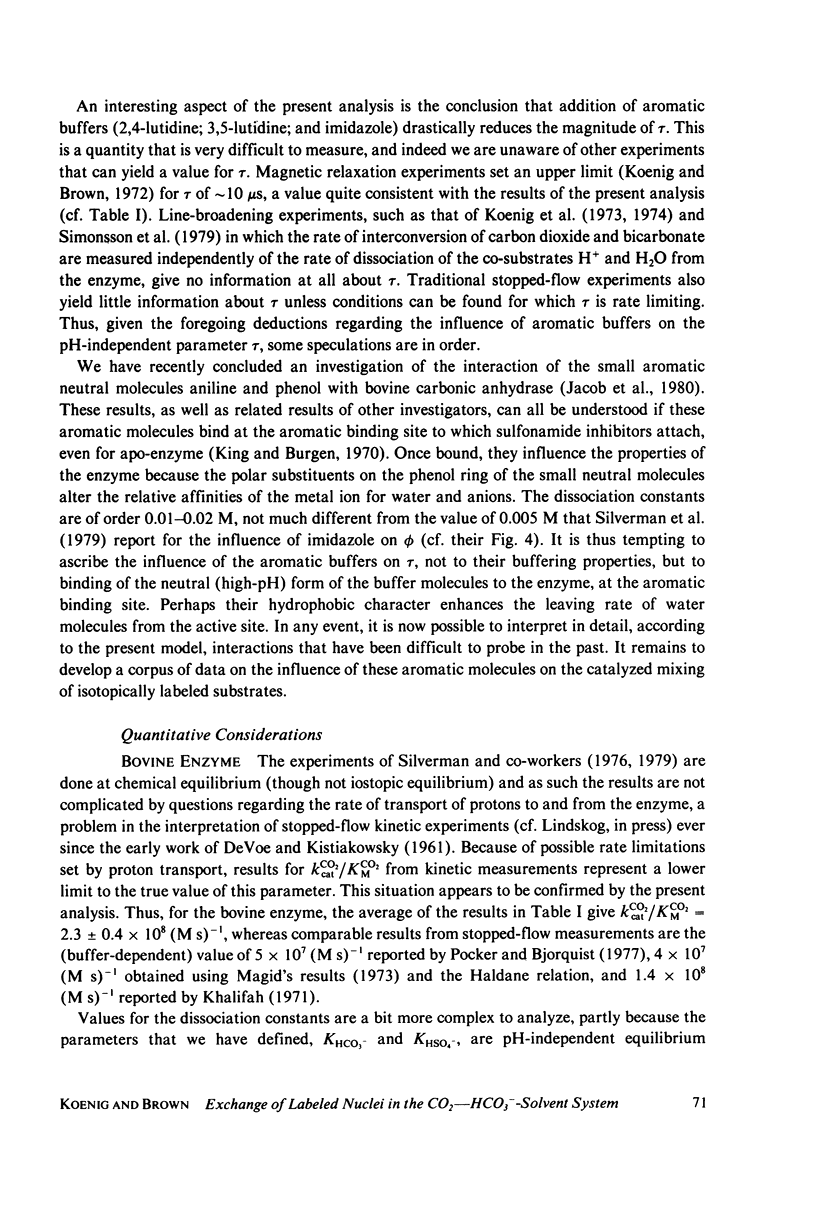
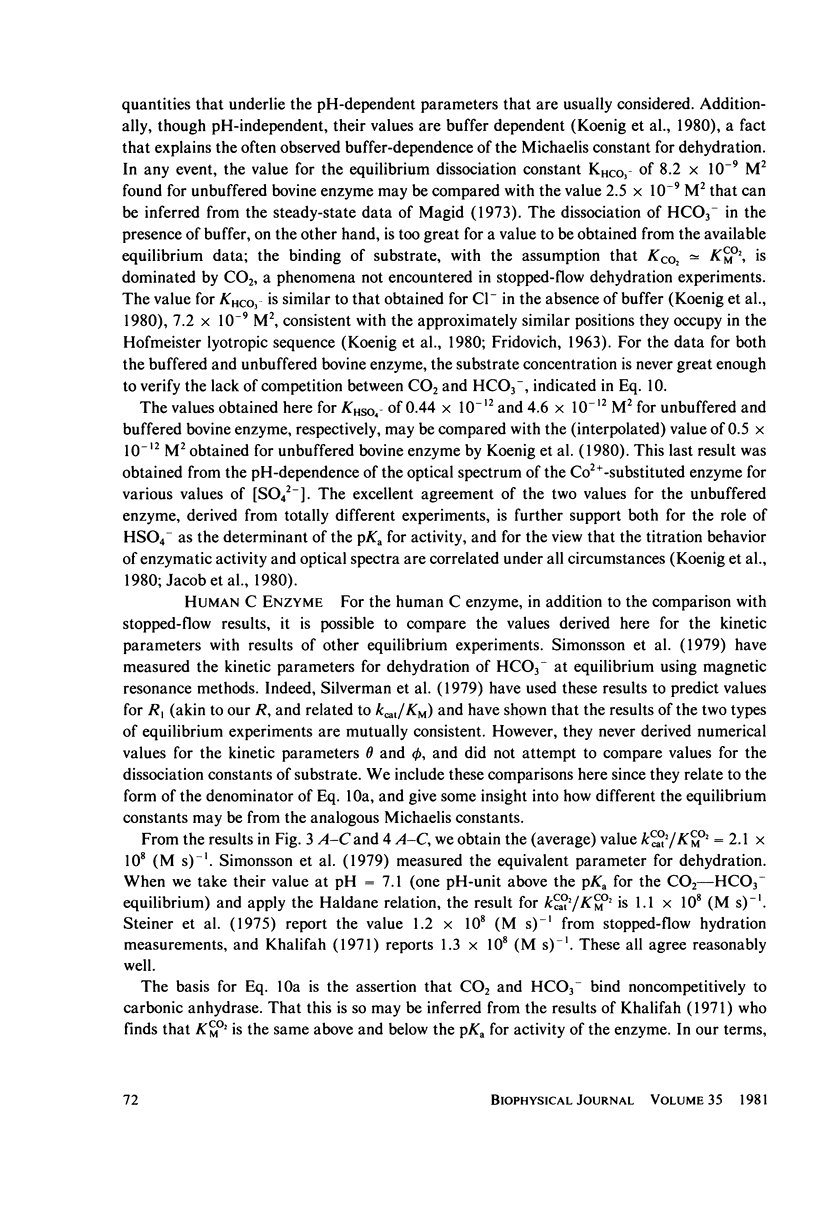
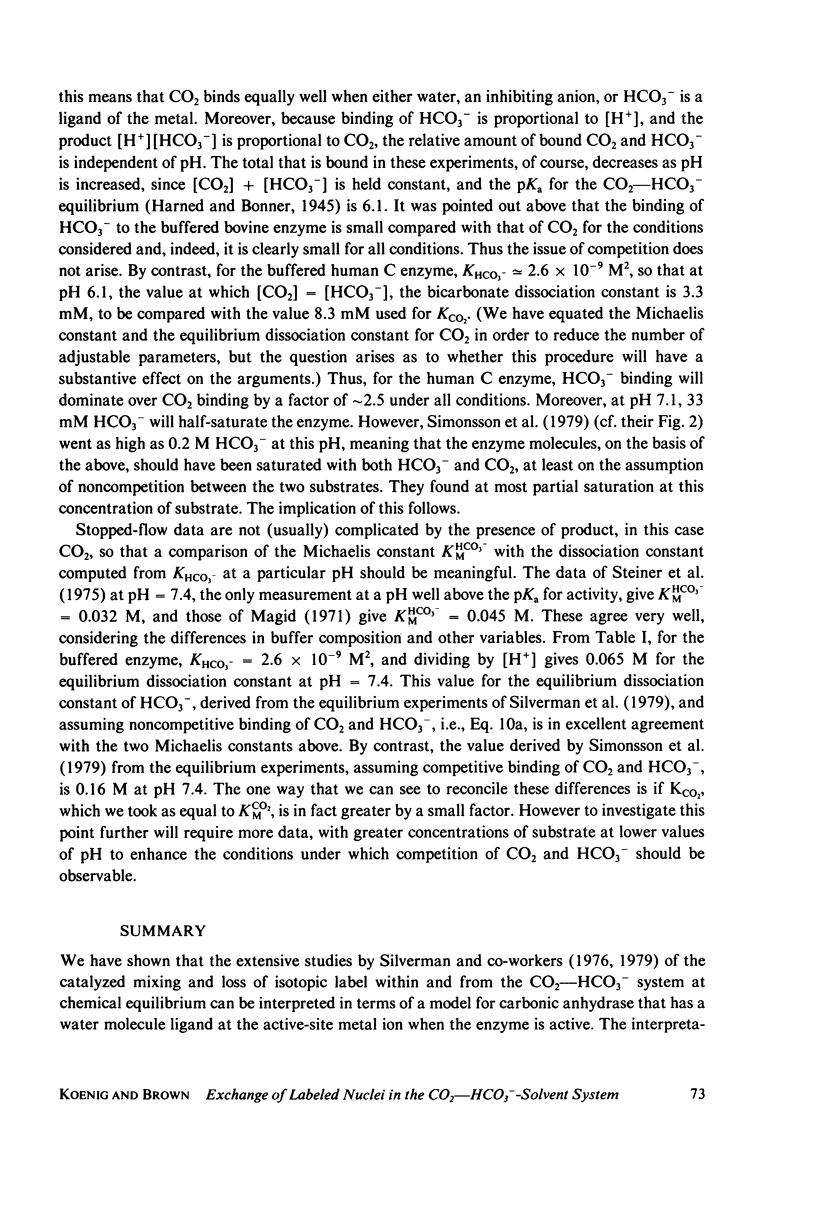
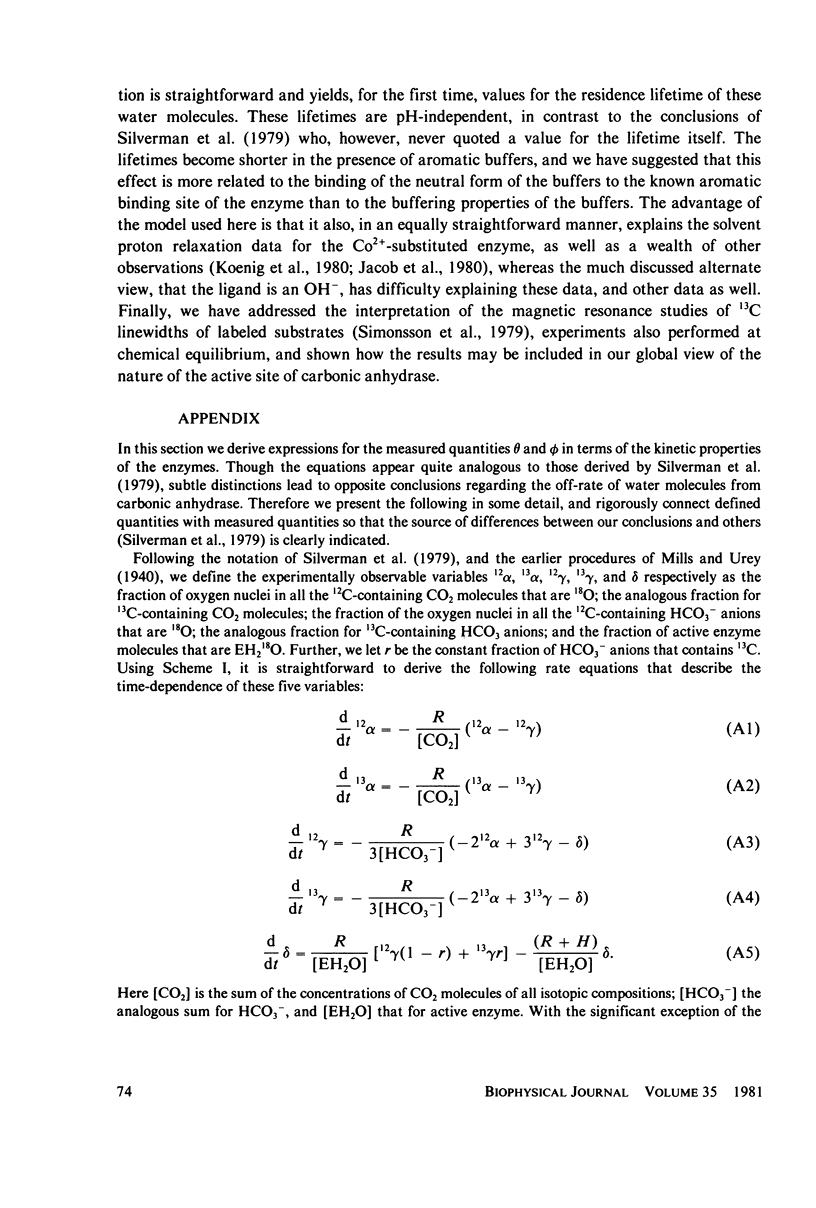
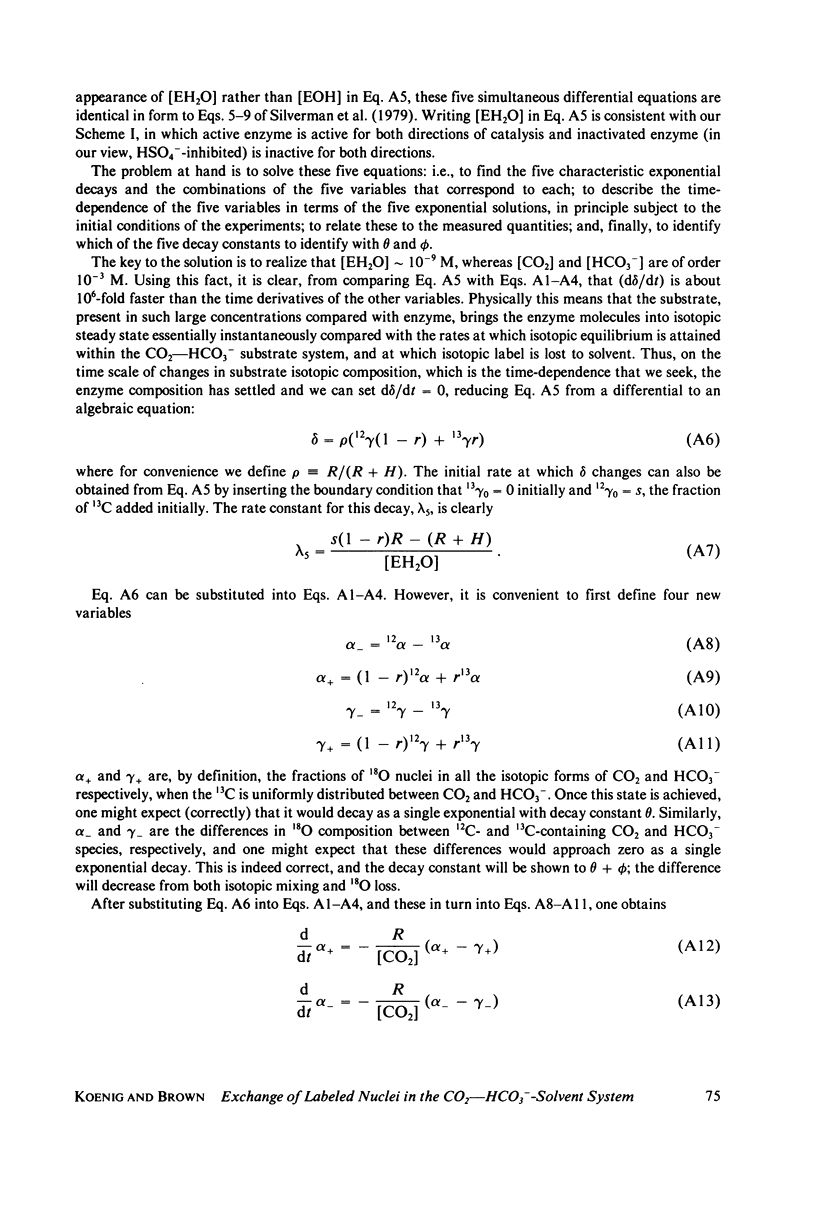
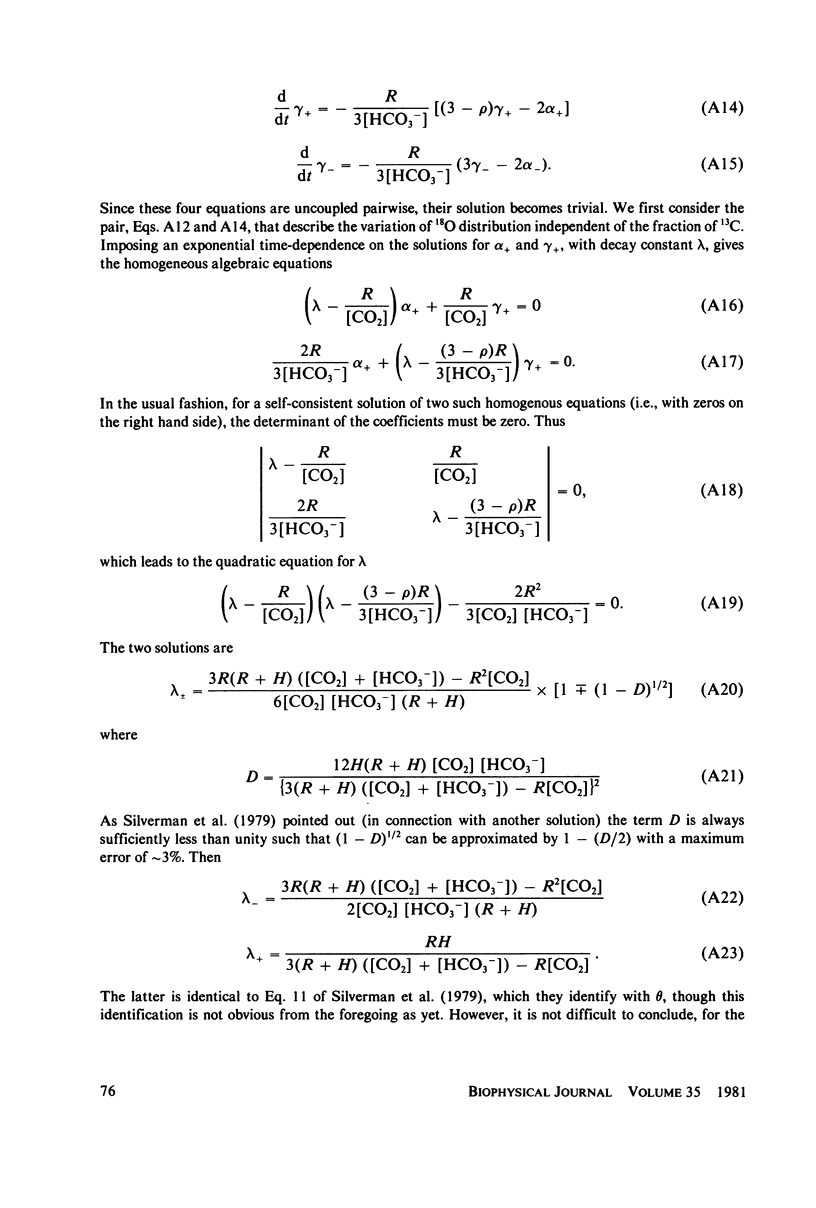
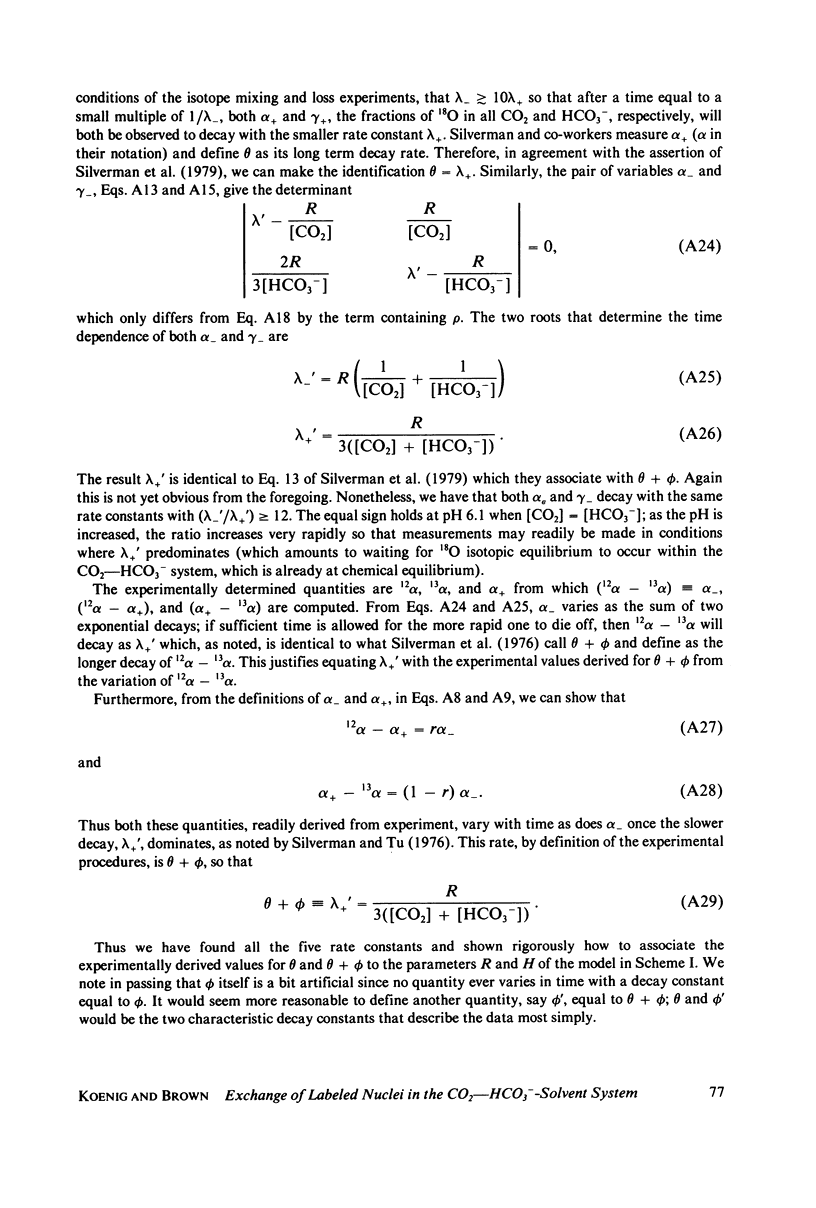
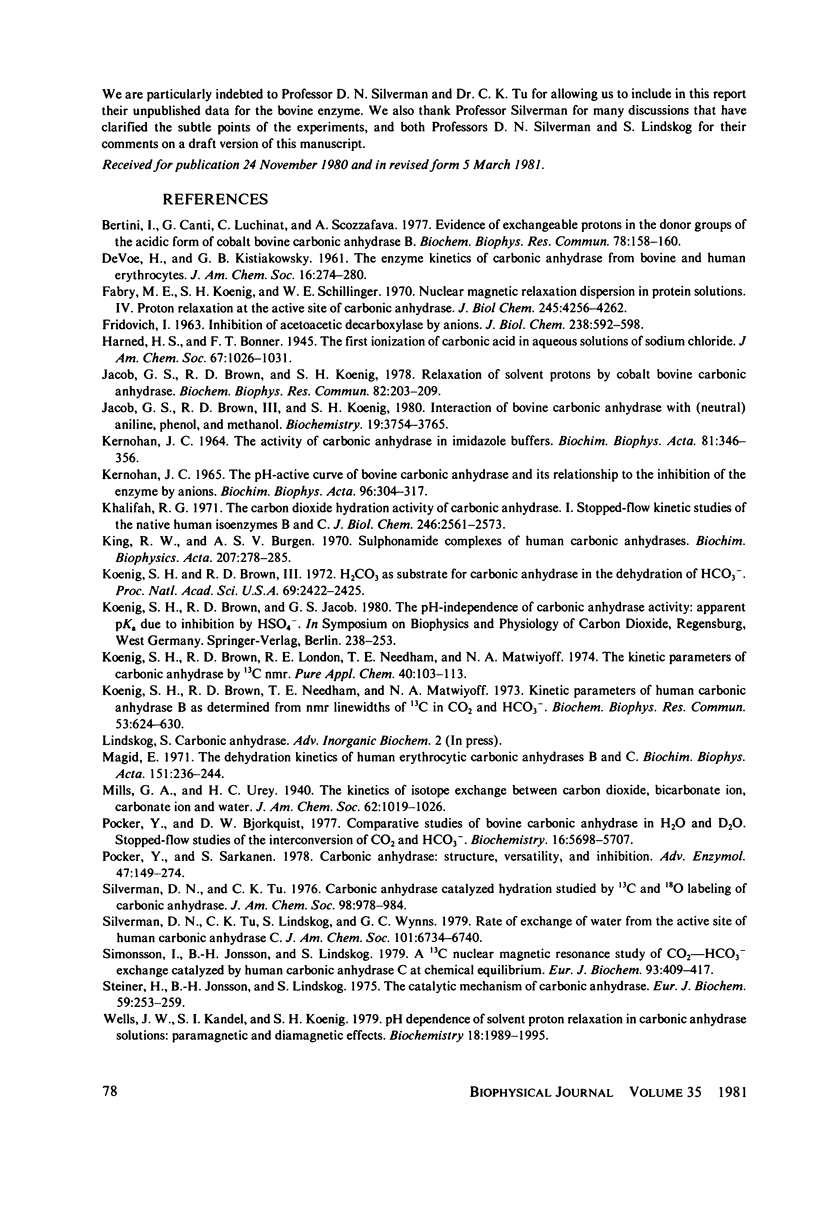
Selected References
These references are in PubMed. This may not be the complete list of references from this article.
- Bertini I., Canti G., Luchinat C., Scozzafava A. Evidence of exchangeable protons in the donor groups of the acidic form of cobalt bovine carbonic anhydrase B. Biochem Biophys Res Commun. 1977 Sep 9;78(1):158–160. doi: 10.1016/0006-291x(77)91234-7. [DOI] [PubMed] [Google Scholar]
- FRIDOVICH I. Inhibition of acetoacetic decarboxylase by anions. The Hofmeister lyotropic series. J Biol Chem. 1963 Feb;238:592–598. [PubMed] [Google Scholar]
- Fabry M. E., Koenig S. H., Schillinger W. E. Nuclear magnetic relaxation dispersion in protein solutions. IV. Proton relaxation at the active site of carbonic anhydrase. J Biol Chem. 1970 Sep 10;245(17):4256–4262. [PubMed] [Google Scholar]
- Jacob G. S., Brown R. D., 3rd, Koenig S. H. Interaction of bovine carbonic anhydrase with (neutral) aniline, phenol, and methanol. Biochemistry. 1980 Aug 5;19(16):3754–3765. doi: 10.1021/bi00557a017. [DOI] [PubMed] [Google Scholar]
- Jacob G. S., Brown R. D., 3rd, Koenig S. H. Relaxation of solvent protons by cobalt bovine carbonic anhydrase. Biochem Biophys Res Commun. 1978 May 15;82(1):203–209. doi: 10.1016/0006-291x(78)90596-x. [DOI] [PubMed] [Google Scholar]
- KERNOHAN J. C. THE PH-ACTIVITY CURVE OF BOVINE CARBONIC ANHYDRASE AND ITS RELATIONSHIP TO THE INHIBITION OF THE ENZYME BY ANIONS. Biochim Biophys Acta. 1965 Feb 22;96:304–317. [PubMed] [Google Scholar]
- Khalifah R. G. The carbon dioxide hydration activity of carbonic anhydrase. I. Stop-flow kinetic studies on the native human isoenzymes B and C. J Biol Chem. 1971 Apr 25;246(8):2561–2573. [PubMed] [Google Scholar]
- King R. W., Burgen A. S. Sulphonamide complexes of human carbonic anhydrases. Ultraviolet difference spectroscopy. Biochim Biophys Acta. 1970 May 26;207(2):278–285. doi: 10.1016/0005-2795(70)90020-6. [DOI] [PubMed] [Google Scholar]
- Koenig S. H., Brown R. D., 3rd H 2 CO 3 as substrate for carbonic anhydrase in the dehydration of HCO 3 . Proc Natl Acad Sci U S A. 1972 Sep;69(9):2422–2425. doi: 10.1073/pnas.69.9.2422. [DOI] [PMC free article] [PubMed] [Google Scholar]
- Koenig S. H., Brown R. D. Kinetic parameters of human carbonic anhydrase B as determined from NMR linewidths of 13 C in CO 2 and HCO 3 - . Biochem Biophys Res Commun. 1973 Jul 17;53(2):624–630. doi: 10.1016/0006-291x(73)90707-9. [DOI] [PubMed] [Google Scholar]
- Magid E. The dehydration kinetics of human erythrocytic carbonic anhydrases B and C. Biochim Biophys Acta. 1968 Jan 8;151(1):236–244. doi: 10.1016/0005-2744(68)90178-2. [DOI] [PubMed] [Google Scholar]
- Pocker Y., Bjorkquist D. W. Comparative studies of bovine carbonic anhydrase in H2O and D2O. Stopped-flow studies of the kinetics of interconversion of CO2 and HCO3. Biochemistry. 1977 Dec 27;16(26):5698–5707. doi: 10.1021/bi00645a008. [DOI] [PubMed] [Google Scholar]
- Pocker Y., Sarkanen S. Carbonic anhydrase: structure catalytic versatility, and inhibition. Adv Enzymol Relat Areas Mol Biol. 1978;47:149–274. doi: 10.1002/9780470122921.ch3. [DOI] [PubMed] [Google Scholar]
- Silverman D. N., Tu C. K. Carbonic anhydrase catalyzed hydration studied by 13C and 18O labeling of carbon dioxide. J Am Chem Soc. 1976 Feb 18;98(4):978–984. doi: 10.1021/ja00420a019. [DOI] [PubMed] [Google Scholar]
- Simonsson I., Jonsson B. H., Lindskog S. A 13C nuclear-magnetic-resonance study of CO2-HCO3-exchange catalyzed by human carbonic anhydrase C at chemical equilibrium. Eur J Biochem. 1979 Jan 15;93(2):409–417. doi: 10.1111/j.1432-1033.1979.tb12837.x. [DOI] [PubMed] [Google Scholar]
- Steiner H., Jonsson B. H., Lindskog S. The catalytic mechanism of carbonic anhydrase. Hydrogen-isotope effects on the kinetic parameters of the human C isoenzyme. Eur J Biochem. 1975 Nov 1;59(1):253–259. doi: 10.1111/j.1432-1033.1975.tb02449.x. [DOI] [PubMed] [Google Scholar]
- Wells J. W., Kandel S. I., Koenig S. H. pH dependence of solvent proton relaxation in carbonic anhydrase solutions: paramagnetic and diamagnetic effects. Biochemistry. 1979 May 15;18(10):1989–1995. doi: 10.1021/bi00577a022. [DOI] [PubMed] [Google Scholar]