Abstract
The relations among membrane structure, mechanical properties, and cell shape have been investigated. The fluid mosaic membrane models used contains several components that move freely in the membrane plane. These components interact with each other and determine properties of the membrane such as curvature and elasticity. A free energy equation is postulated for such a multicomponent membrane and the condition of free energy minimum is used to obtain differential equations relating the distribution of membrane components and the local membrane curvature. The force that moves membrane components along the membrane in a variable curvature field is calculated. A change in the intramembrane interactions can bring about phase separation or particle clustering. This, in turn, may strongly affect the local curvature. The numerical solution of the set of equations for the two dimensional case allows determination of the cell shape and the component distribution along the membrane. The model has been applied to describe certain erythrocytes shape transformations.
Full text
PDF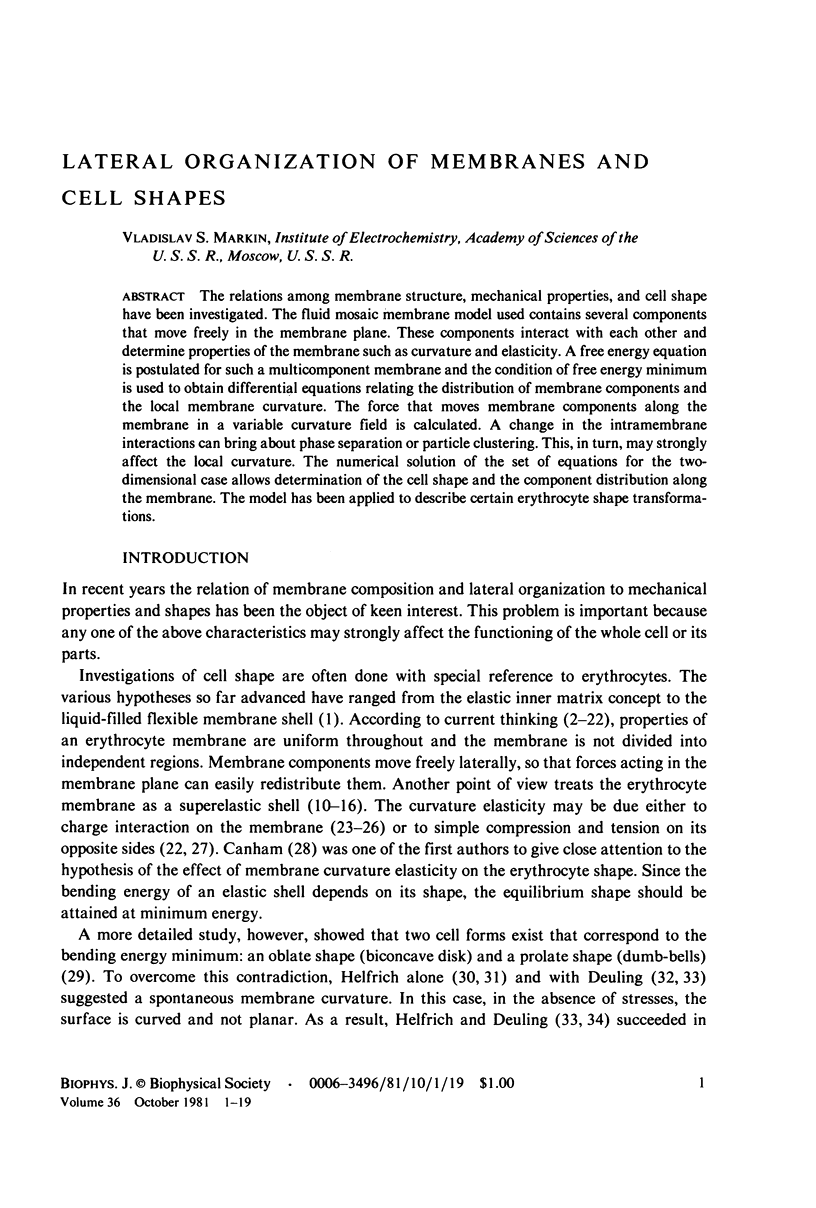
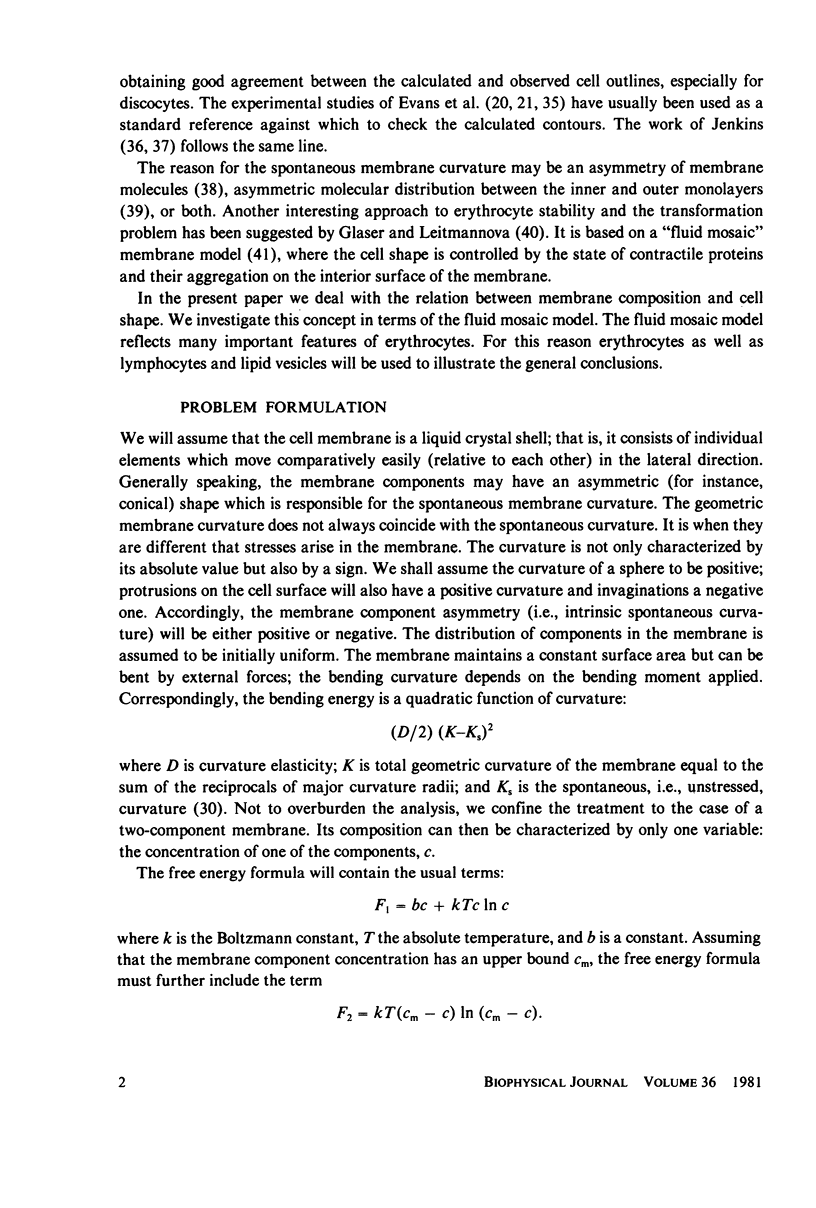
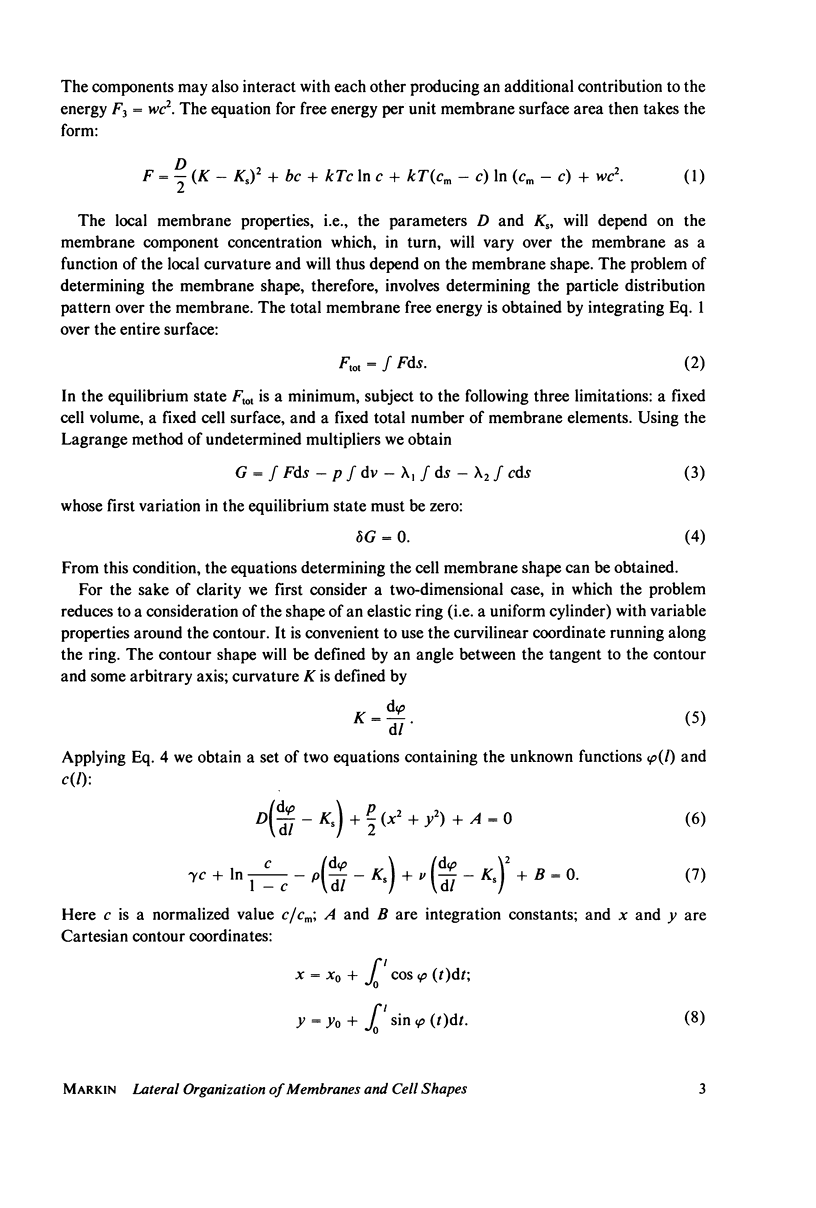
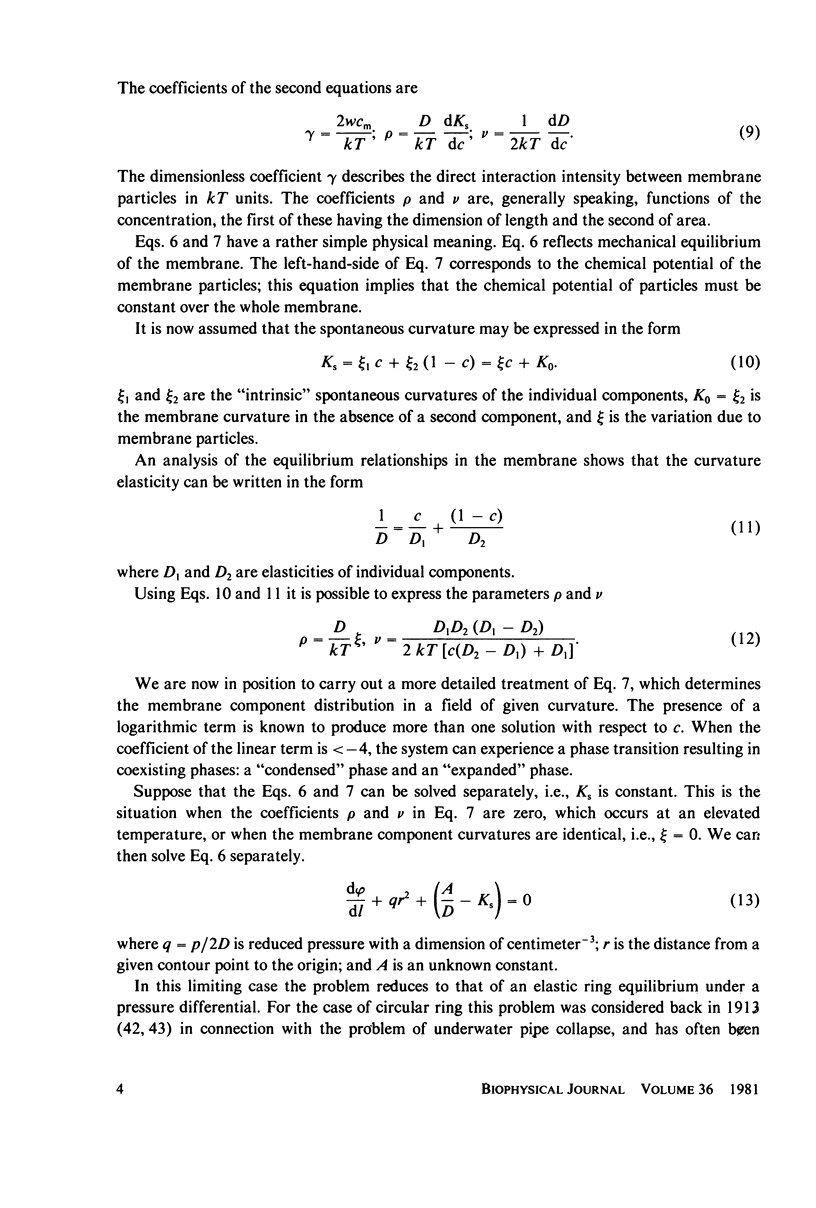
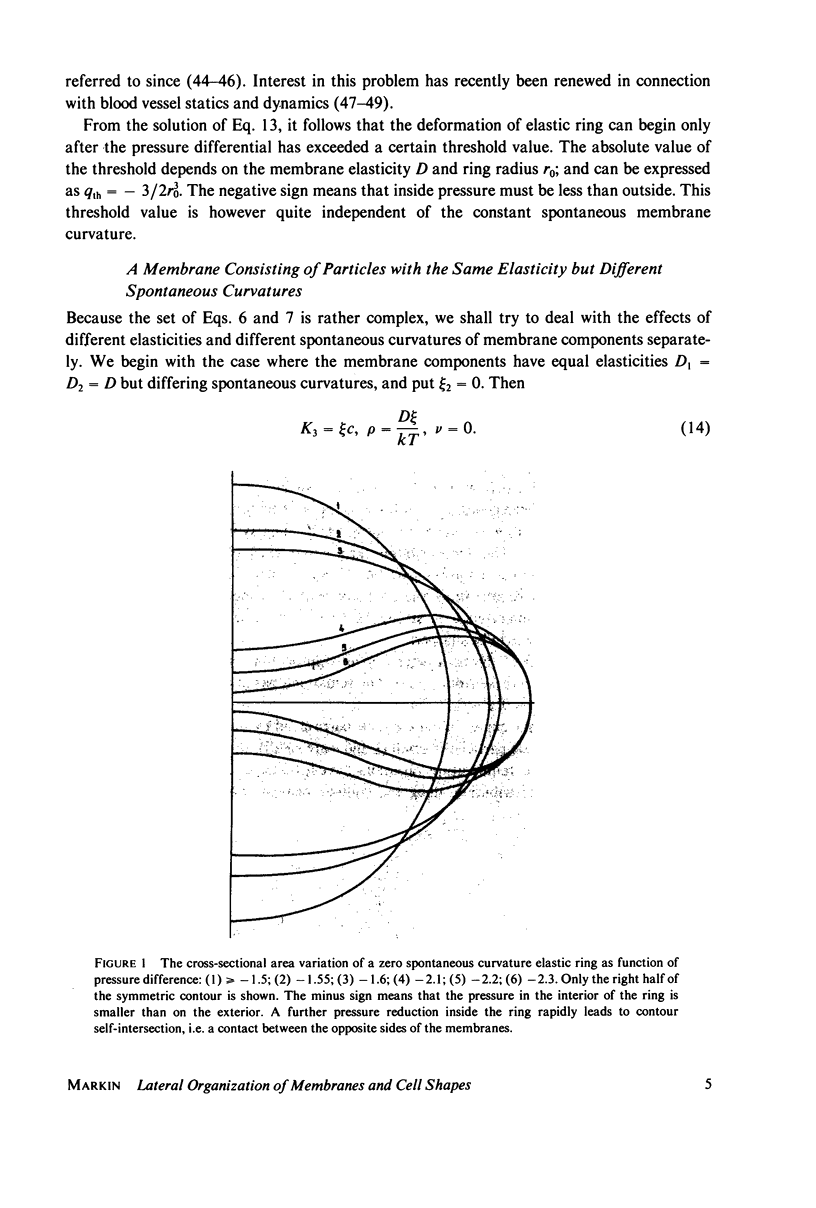
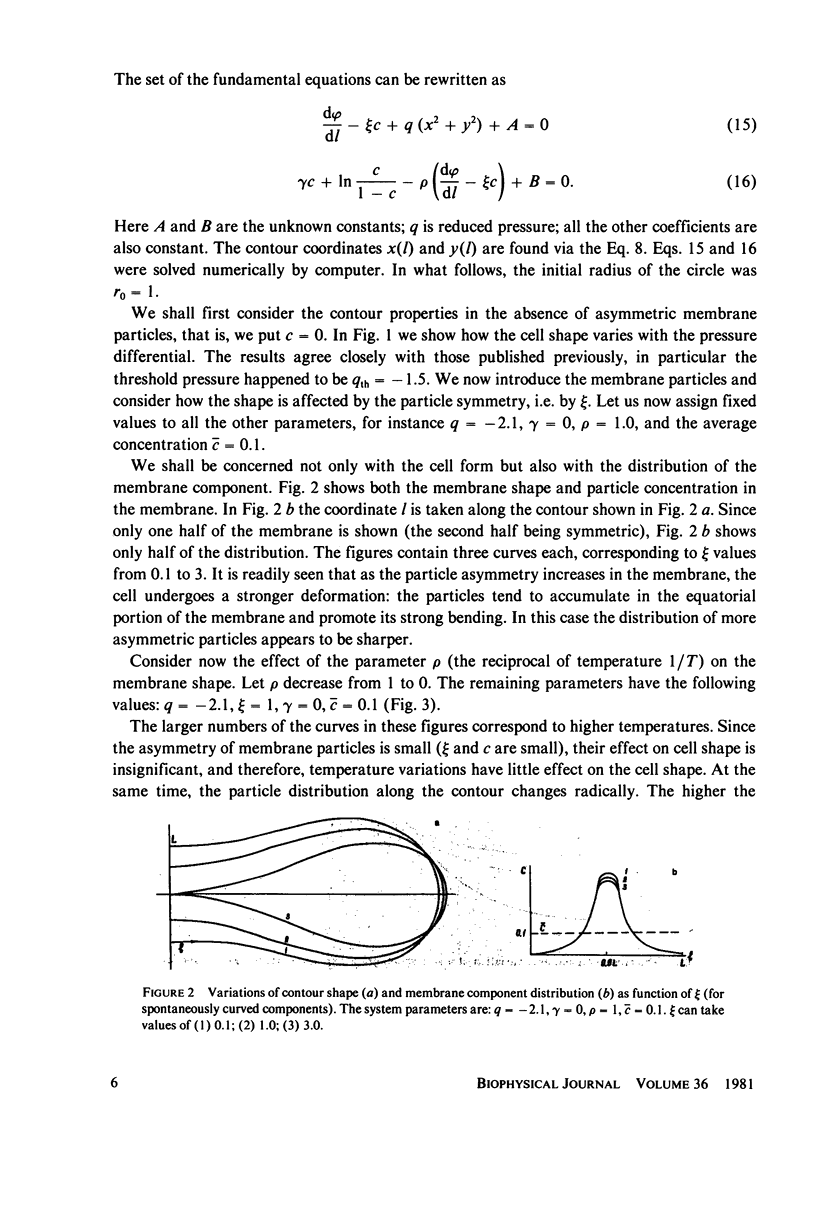
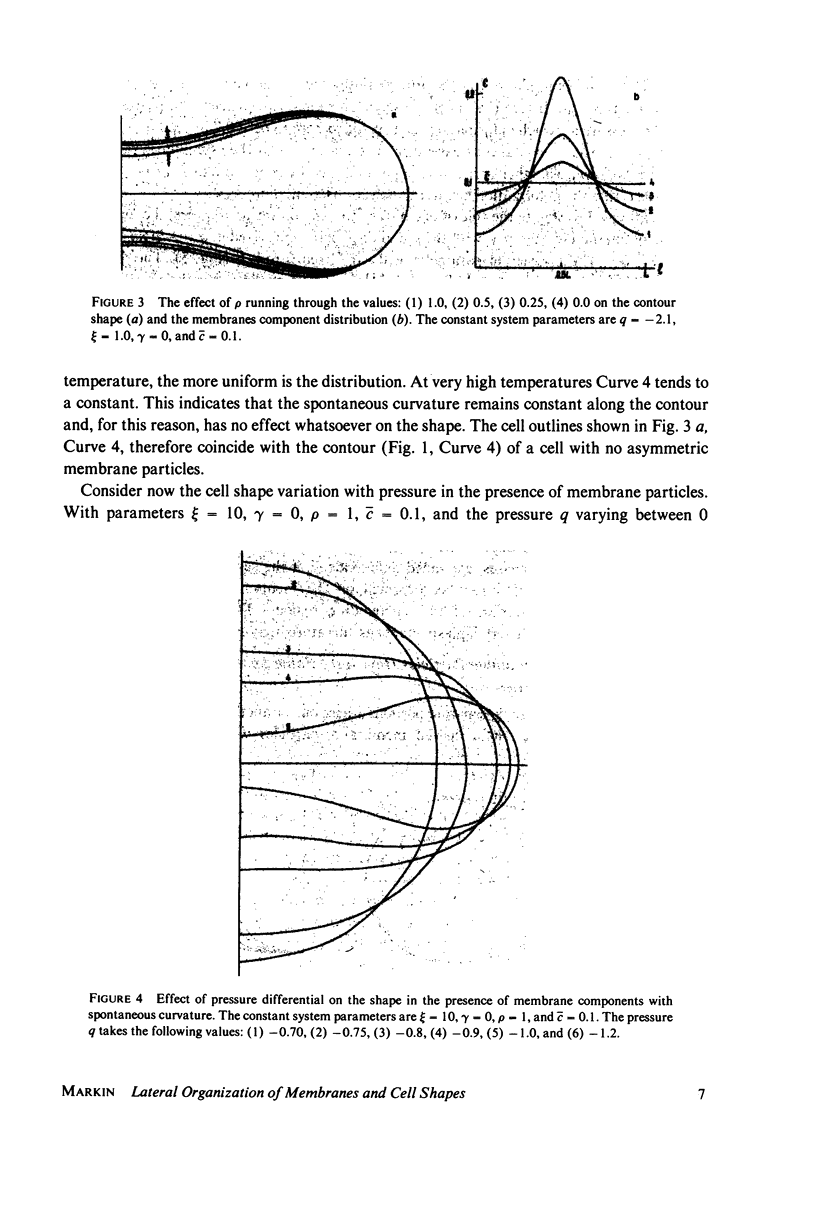
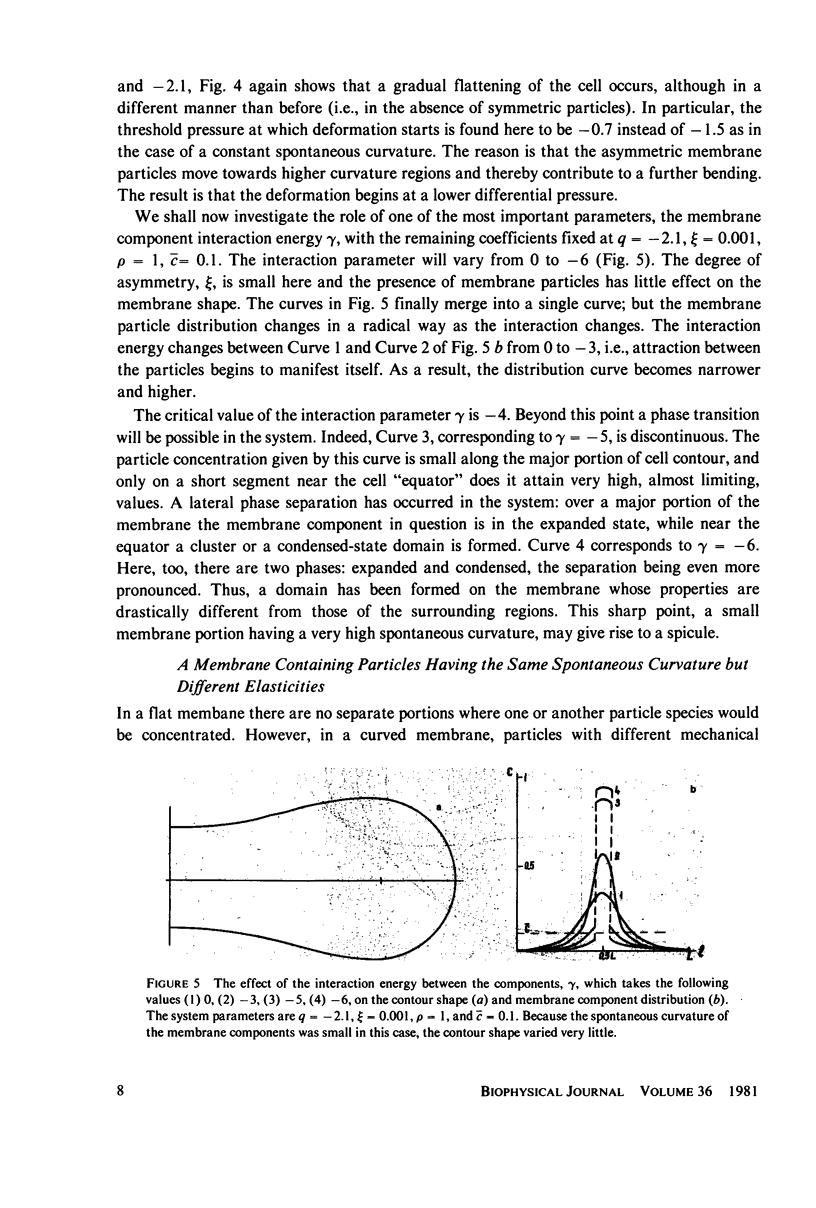
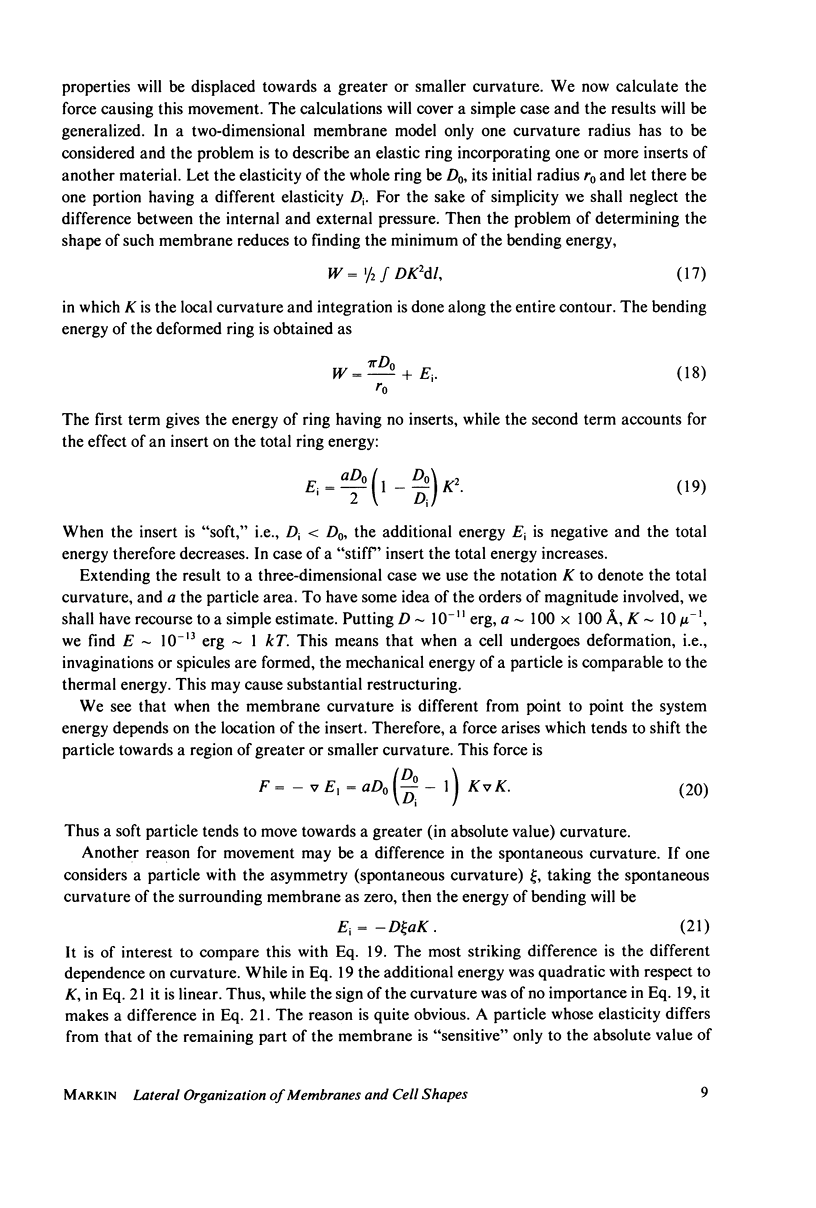
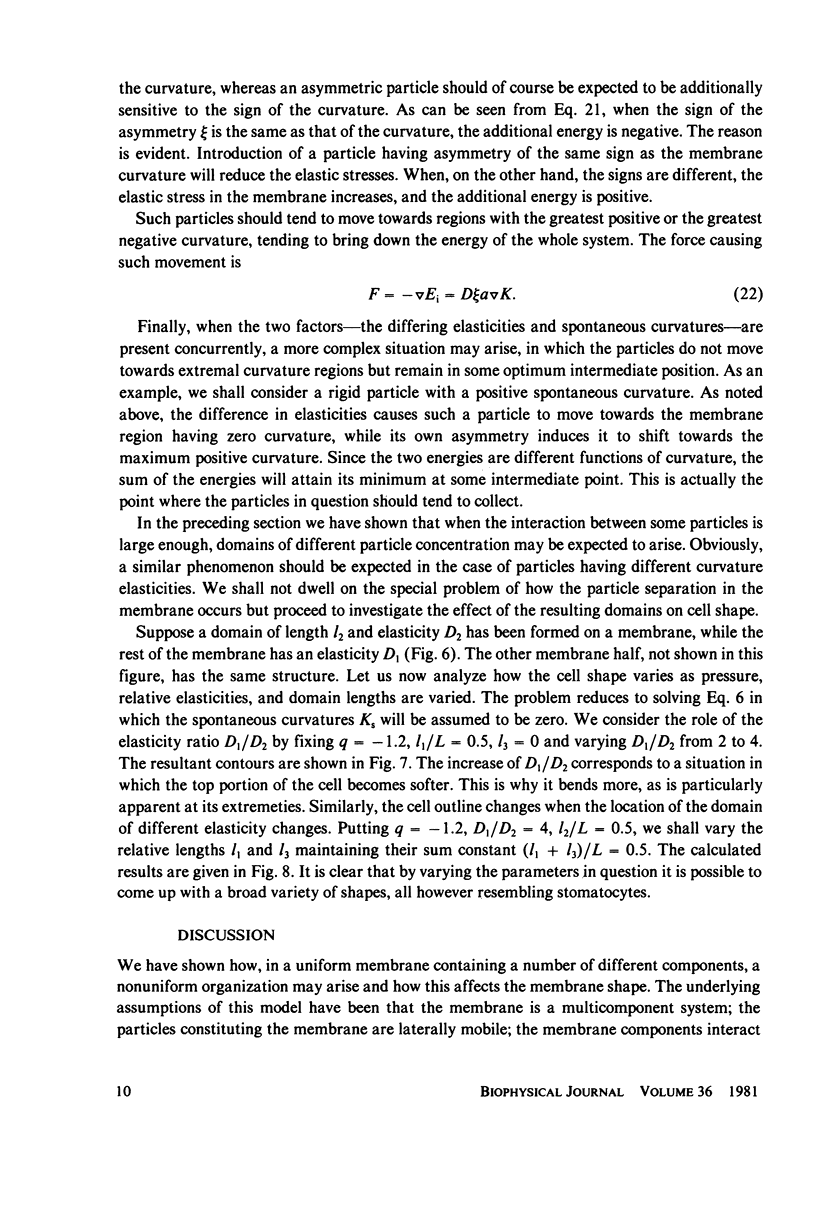
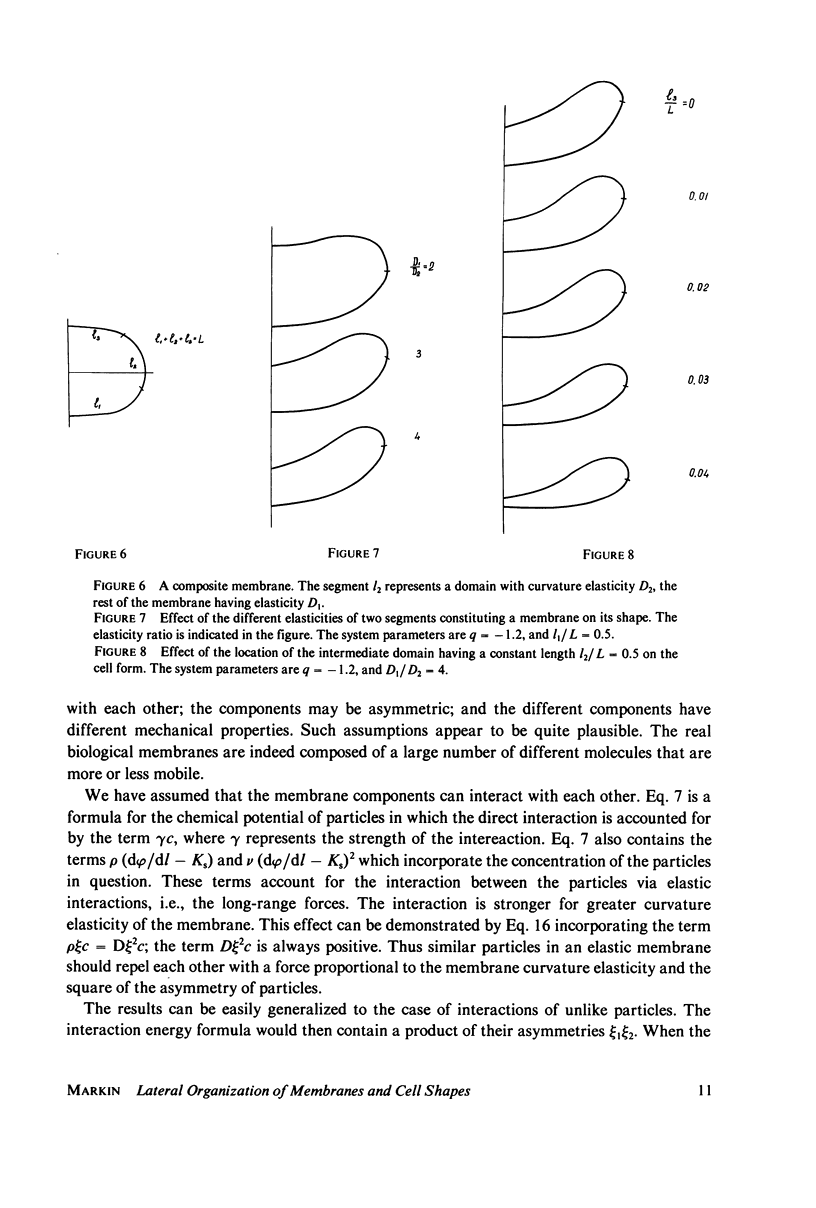
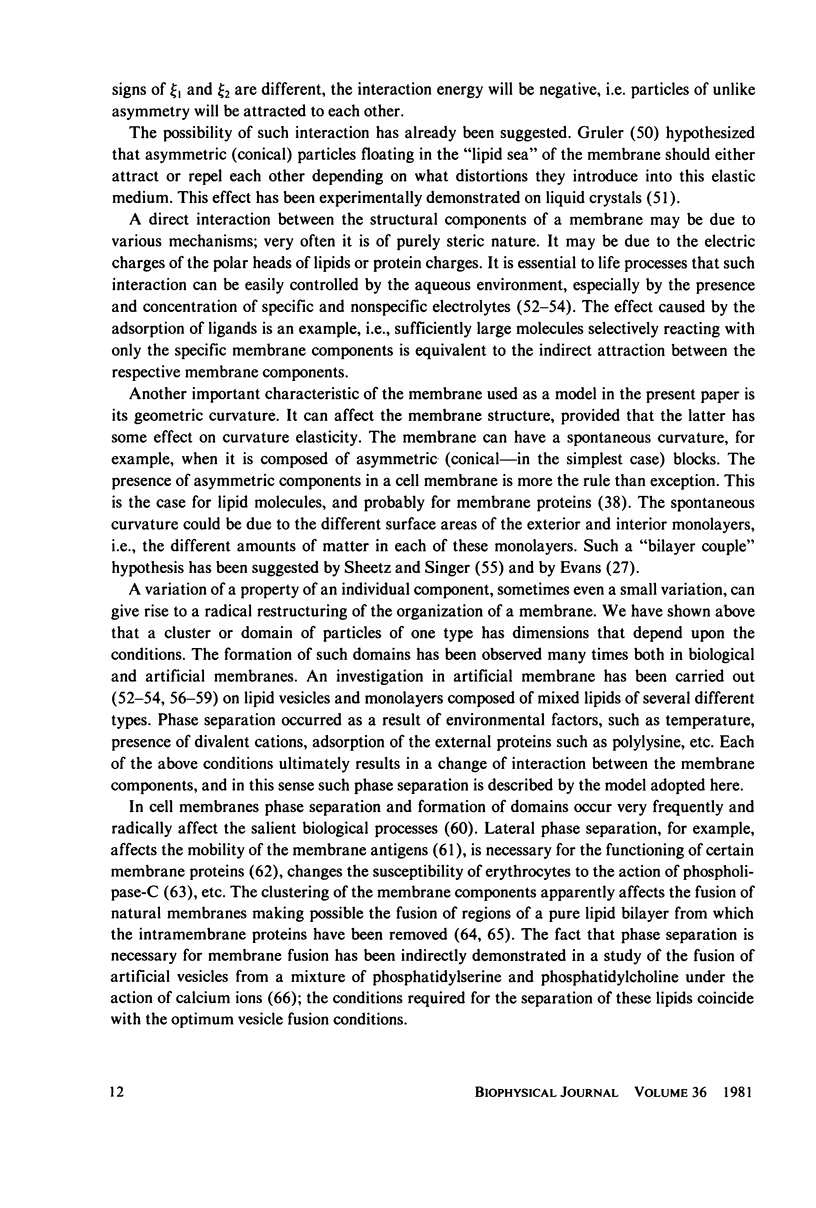
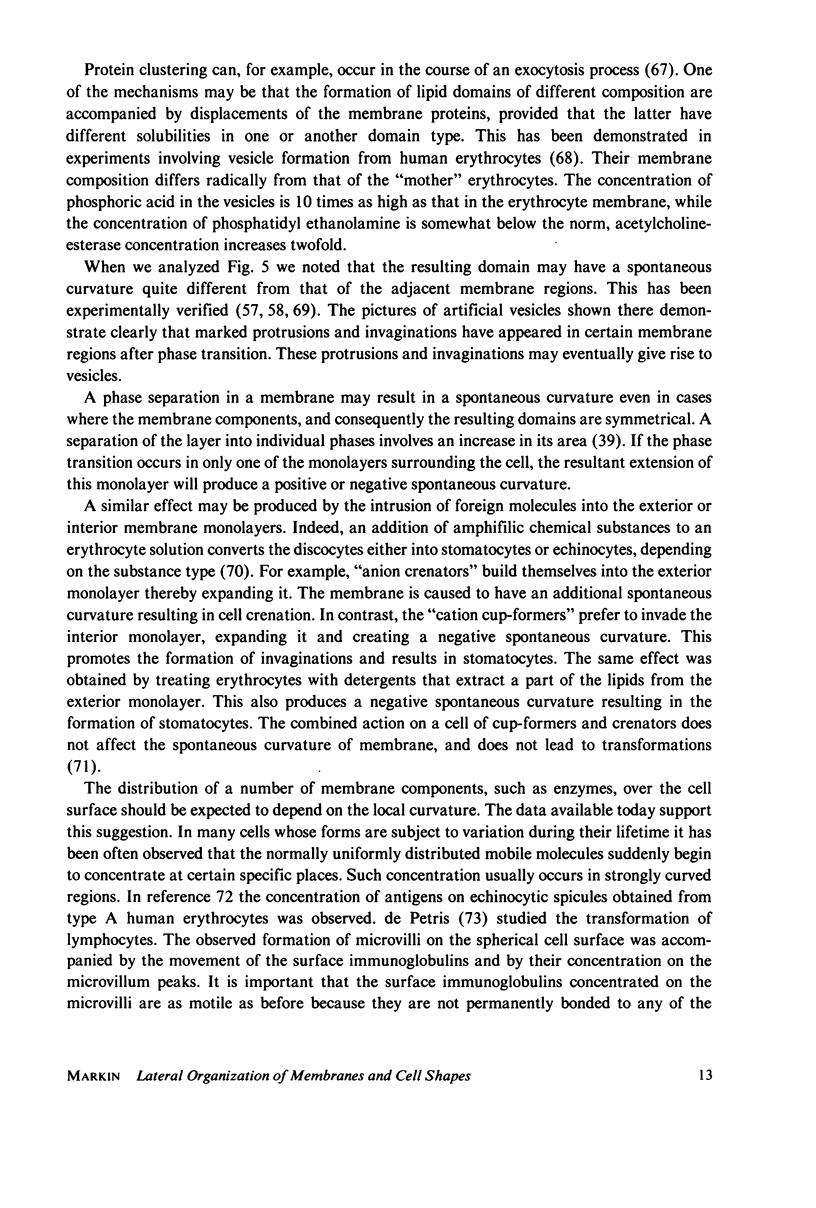
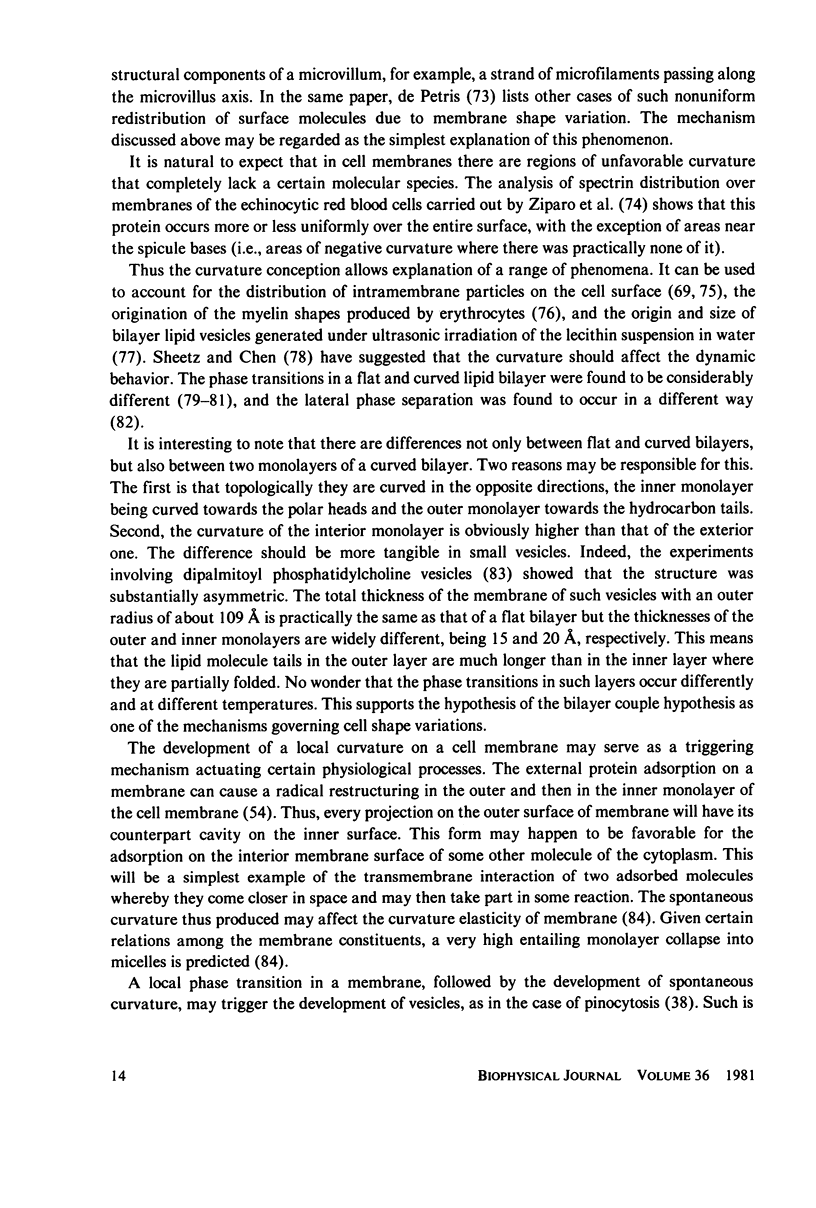
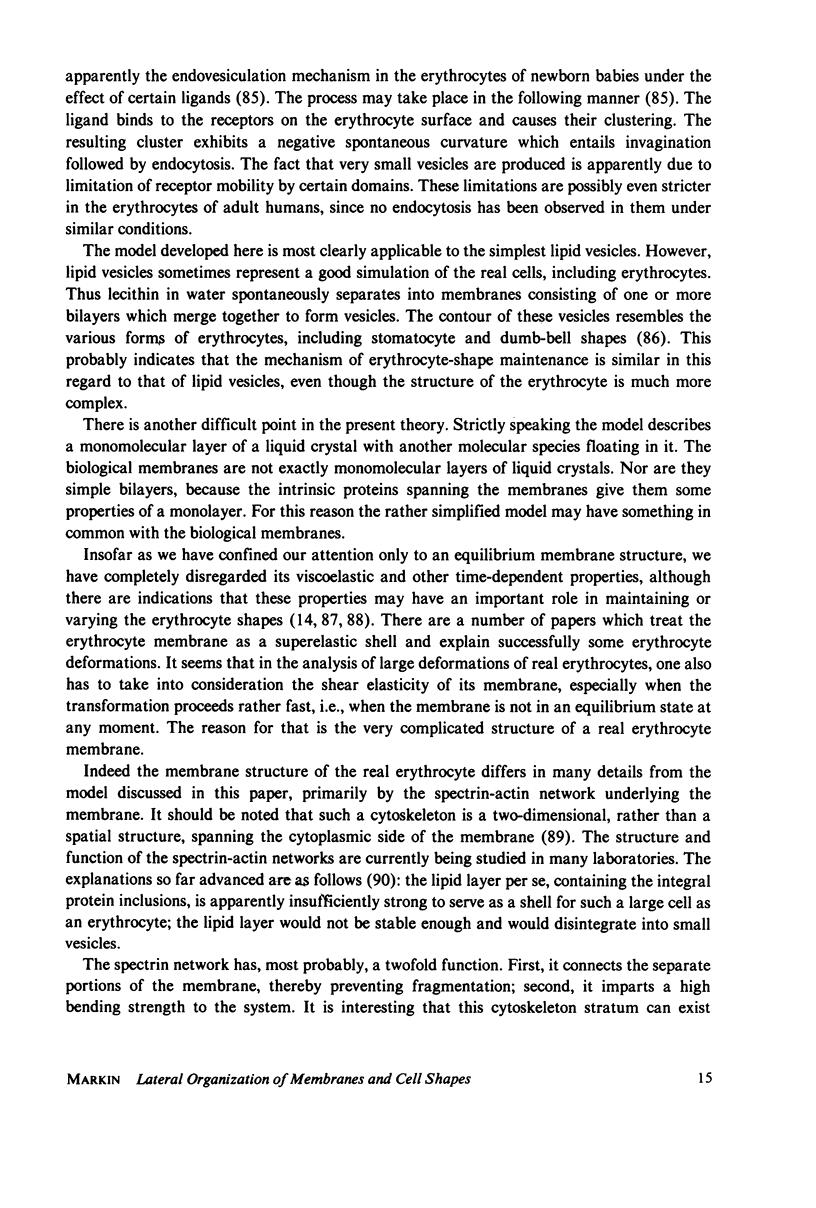
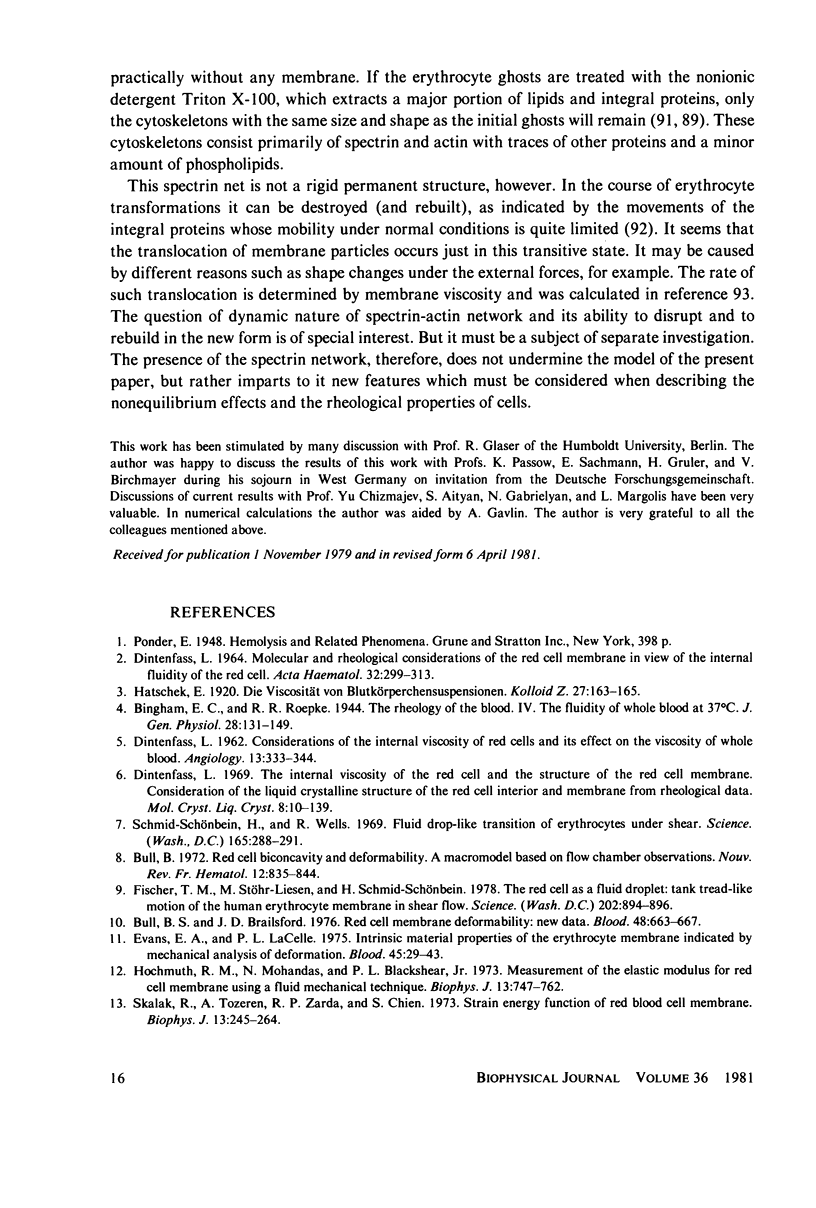
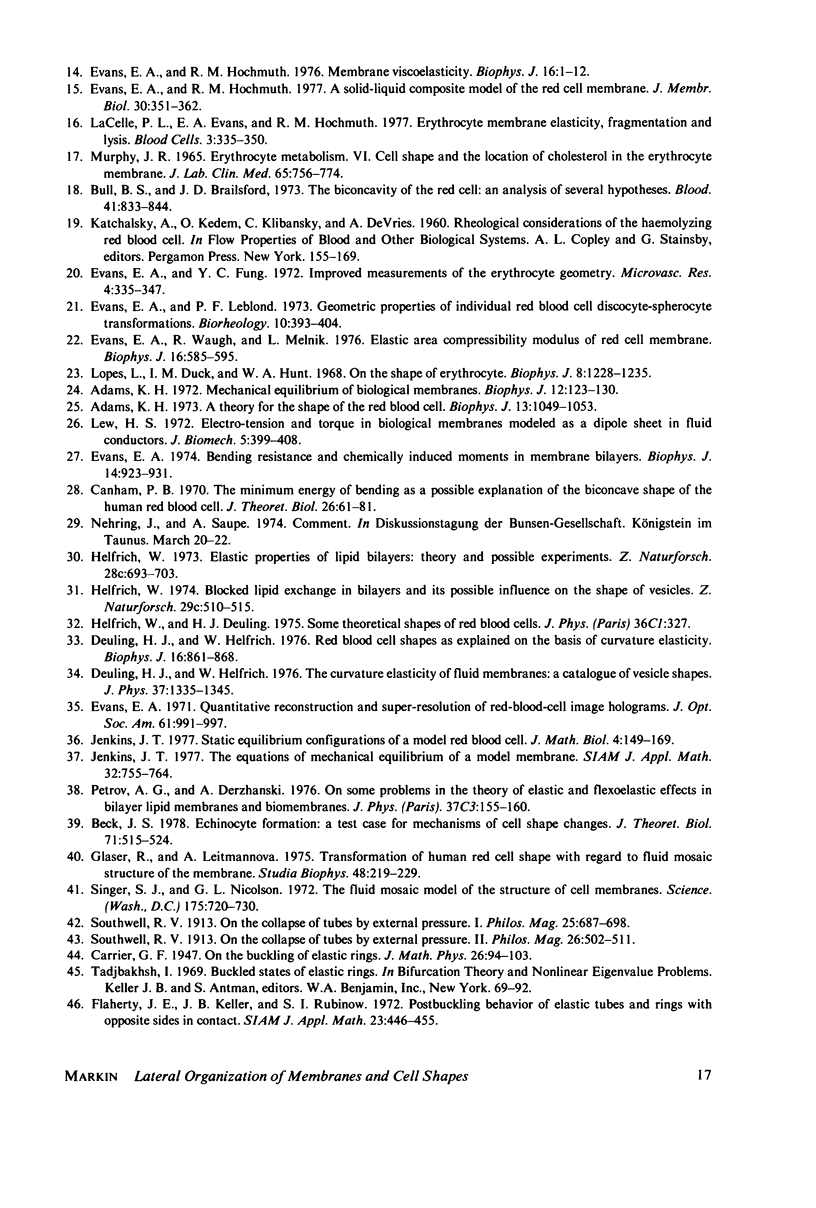
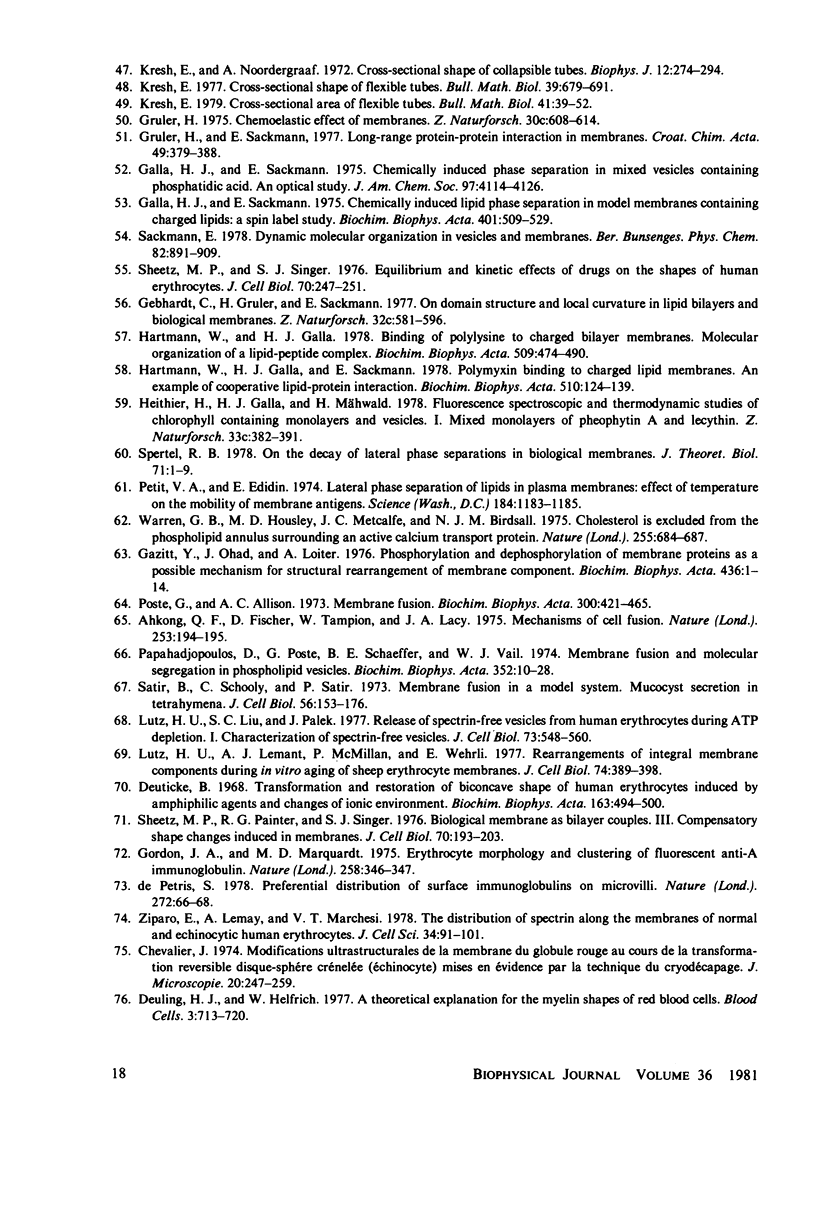
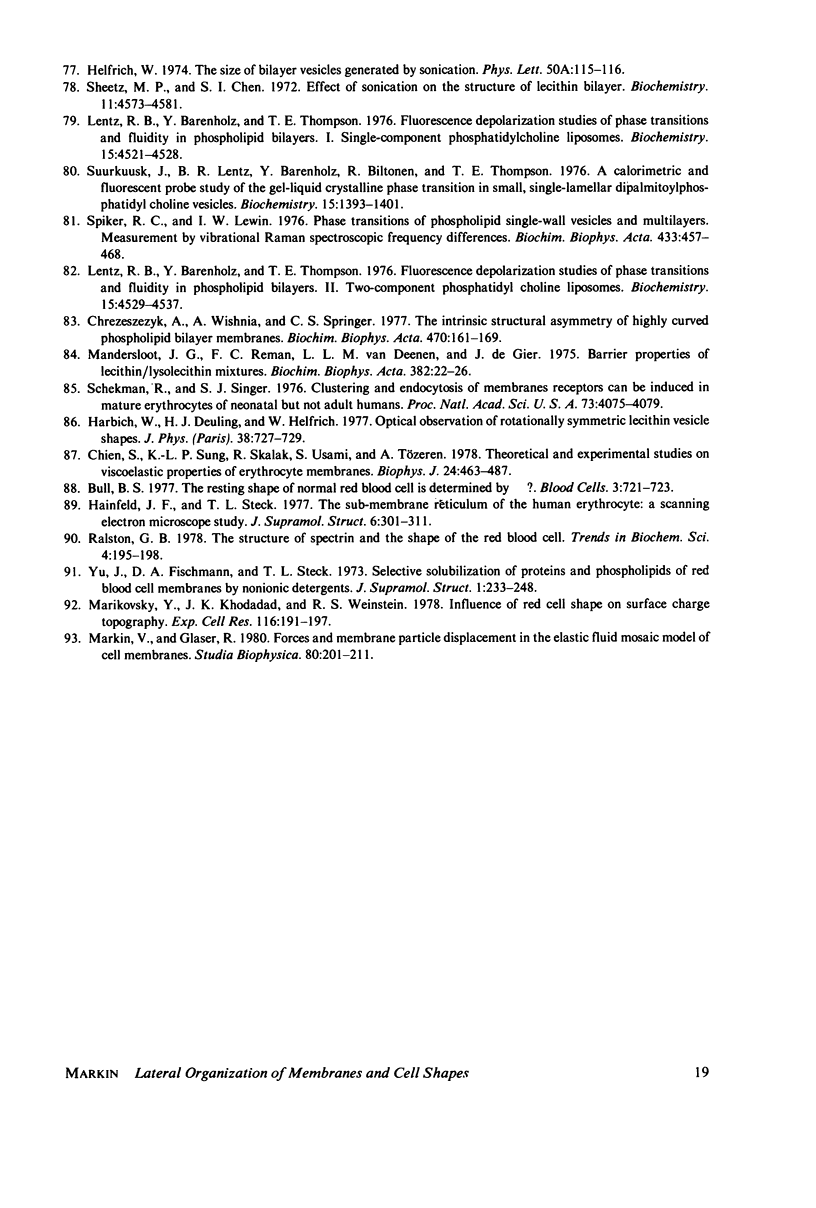
Selected References
These references are in PubMed. This may not be the complete list of references from this article.
- Adams K. H. A theory for the shape of the red blood cell. Biophys J. 1973 Oct;13(10):1049–1053. doi: 10.1016/S0006-3495(73)86044-8. [DOI] [PMC free article] [PubMed] [Google Scholar]
- Adams K. H. Mechanical equilibrium of biological membranes. Biophys J. 1972 Jan;12(1):123–130. doi: 10.1016/S0006-3495(72)86075-2. [DOI] [PMC free article] [PubMed] [Google Scholar]
- Ahkong Q. F., Fisher D., Tampion W., Lucy J. A. Mechanisms of cell fusion. Nature. 1975 Jan 17;253(5488):194–195. doi: 10.1038/253194a0. [DOI] [PubMed] [Google Scholar]
- Beck J. S. Echinocyte formation: a test case for mechanisms of cell shape changes. J Theor Biol. 1978 Apr 20;71(4):515–524. doi: 10.1016/0022-5193(78)90322-3. [DOI] [PubMed] [Google Scholar]
- Bingham E. C., Roepke R. R. THE RHEOLOGY OF THE BLOOD : IV. THE FLUIDITY OF WHOLE BLOOD AT 37 degrees C. J Gen Physiol. 1944 Nov 20;28(2):131–149. doi: 10.1085/jgp.28.2.131. [DOI] [PMC free article] [PubMed] [Google Scholar]
- Bull B. S., Brailsford J. D. Red cell membrane deformability: new data. Blood. 1976 Nov;48(5):663–667. [PubMed] [Google Scholar]
- Bull B. S., Brailsford J. D. The biconcavity of the red cell: an analysis of several hypotheses. Blood. 1973 Jun;41(6):833–844. [PubMed] [Google Scholar]
- Bull B. Red cell biconcavity and deformability. A macromodel based on flow chamber observations. Nouv Rev Fr Hematol. 1972 Nov-Dec;12(6):835–844. [PubMed] [Google Scholar]
- Canham P. B. The minimum energy of bending as a possible explanation of the biconcave shape of the human red blood cell. J Theor Biol. 1970 Jan;26(1):61–81. doi: 10.1016/s0022-5193(70)80032-7. [DOI] [PubMed] [Google Scholar]
- Chien S., Sung K. L., Skalak R., Usami S., Tözeren A. Theoretical and experimental studies on viscoelastic properties of erythrocyte membrane. Biophys J. 1978 Nov;24(2):463–487. doi: 10.1016/S0006-3495(78)85395-8. [DOI] [PMC free article] [PubMed] [Google Scholar]
- Chrzeszczyk A., Wishnia A., Springer C. S., Jr The intrinsic structural asymmetry of highly curved phospholipid bilayer membranes. Biochim Biophys Acta. 1977 Oct 17;470(2):161–169. doi: 10.1016/0005-2736(77)90097-9. [DOI] [PubMed] [Google Scholar]
- DINTENFASS L. Considerations of the internal viscosity of red cells and its effect on the viscosity of whole blood. Angiology. 1962 Aug;13:333–344. doi: 10.1177/000331976201300801. [DOI] [PubMed] [Google Scholar]
- DINTENFASS L. MOLECULAR AND RHEOLOGICAL CONSIDERATIONS OF THE RED CELL MEMBRANE IN VIEW OF THE INTERNAL FLUIDITY OF THE RED CELL. Acta Haematol. 1964 Nov;32:299–313. doi: 10.1159/000209575. [DOI] [PubMed] [Google Scholar]
- Deuling H. J., Helfrich W. Red blood cell shapes as explained on the basis of curvature elasticity. Biophys J. 1976 Aug;16(8):861–868. doi: 10.1016/S0006-3495(76)85736-0. [DOI] [PMC free article] [PubMed] [Google Scholar]
- Deuticke B. Transformation and restoration of biconcave shape of human erythrocytes induced by amphiphilic agents and changes of ionic environment. Biochim Biophys Acta. 1968 Dec 10;163(4):494–500. doi: 10.1016/0005-2736(68)90078-3. [DOI] [PubMed] [Google Scholar]
- Evans E. A. Bending resistance and chemically induced moments in membrane bilayers. Biophys J. 1974 Dec;14(12):923–931. doi: 10.1016/S0006-3495(74)85959-X. [DOI] [PMC free article] [PubMed] [Google Scholar]
- Evans E. A., Hochmuth R. M. A solid-liquid composite model of the red cell membrane. J Membr Biol. 1977 Jan 28;30(4):351–362. doi: 10.1007/BF01869676. [DOI] [PubMed] [Google Scholar]
- Evans E. A., Hochmuth R. M. Membrane viscoelasticity. Biophys J. 1976 Jan;16(1):1–11. doi: 10.1016/S0006-3495(76)85658-5. [DOI] [PMC free article] [PubMed] [Google Scholar]
- Evans E. A., La Celle P. L. Intrinsic material properties of the erythrocyte membrane indicated by mechanical analysis of deformation. Blood. 1975 Jan;45(1):29–43. [PubMed] [Google Scholar]
- Evans E. A., Leblond P. F. Geometric properties of individual red blood cell discocyte-spherocyte transformations. Biorheology. 1973 Sep;10(3):393–404. doi: 10.3233/bir-1973-10313. [DOI] [PubMed] [Google Scholar]
- Evans E. A. Quantitative reconstruction and superresolution of red-blood-cell image holograms. J Opt Soc Am. 1971 Aug;61(8):991–997. doi: 10.1364/josa.61.000991. [DOI] [PubMed] [Google Scholar]
- Evans E. A., Waugh R., Melnik L. Elastic area compressibility modulus of red cell membrane. Biophys J. 1976 Jun;16(6):585–595. doi: 10.1016/S0006-3495(76)85713-X. [DOI] [PMC free article] [PubMed] [Google Scholar]
- Evans E., Fung Y. C. Improved measurements of the erythrocyte geometry. Microvasc Res. 1972 Oct;4(4):335–347. doi: 10.1016/0026-2862(72)90069-6. [DOI] [PubMed] [Google Scholar]
- Fischer T. M., Stöhr-Lissen M., Schmid-Schönbein H. The red cell as a fluid droplet: tank tread-like motion of the human erythrocyte membrane in shear flow. Science. 1978 Nov 24;202(4370):894–896. doi: 10.1126/science.715448. [DOI] [PubMed] [Google Scholar]
- Galla H. J., Sackmann E. Chemically induced lipid phase separation in model membranes containing charged lipids: a spin label study. Biochim Biophys Acta. 1975 Sep 2;401(3):509–529. doi: 10.1016/0005-2736(75)90249-7. [DOI] [PubMed] [Google Scholar]
- Galla H. J., Sackmann E. Chemically induced phase separation in mixed vesicles containing phosphatidic acid. An optical study. J Am Chem Soc. 1975 Jul 9;97(14):4114–4120. doi: 10.1021/ja00847a040. [DOI] [PubMed] [Google Scholar]
- Gazitt Y., Ohad I., Loyter A. Phosphorylation and dephosphorylation of membrane proteins as a possible mechanism for structural rearrangement of membrane components. Biochim Biophys Acta. 1976 Jun 4;436(1):1–14. doi: 10.1016/0005-2736(76)90214-5. [DOI] [PubMed] [Google Scholar]
- Gebhardt C., Gruler H., Sackmann E. On domain structure and local curvature in lipid bilayers and biological membranes. Z Naturforsch C. 1977 Jul-Aug;32(7-8):581–596. doi: 10.1515/znc-1977-7-817. [DOI] [PubMed] [Google Scholar]
- Gordon J. A., Marquardt M. D. Erythrocyte morphology and clustering of fluorescent anti-A immunoglobulin. Nature. 1975 Nov 27;258(5533):346–347. doi: 10.1038/258346a0. [DOI] [PubMed] [Google Scholar]
- Gruler H. Chemoelastic effect of membranes. Z Naturforsch C. 1975 Sep-Oct;30(5):608–614. doi: 10.1515/znc-1975-9-1009. [DOI] [PubMed] [Google Scholar]
- Hainfeld J. F., Steck T. L. The sub-membrane reticulum of the human erythrocyte: a scanning electron microscope study. J Supramol Struct. 1977;6(3):301–311. doi: 10.1002/jss.400060303. [DOI] [PubMed] [Google Scholar]
- Hartmann W., Galla H. J. Binding of polylysine to charged bilayer membranes: molecular organization of a lipid.peptide complex. Biochim Biophys Acta. 1978 Jun 2;509(3):474–490. doi: 10.1016/0005-2736(78)90241-9. [DOI] [PubMed] [Google Scholar]
- Hartmann W., Galla H. J., Sackmann E. Polymyxin binding to charged lipid membranes. An example of cooperative lipid-protein interaction. Biochim Biophys Acta. 1978 Jun 16;510(1):124–139. doi: 10.1016/0005-2736(78)90135-9. [DOI] [PubMed] [Google Scholar]
- Helfrich W. Blocked lipid exchange in bilayers and its possible influence on the shape of vesicles. Z Naturforsch C. 1974 Sep-Oct;29C(9-10):510–515. doi: 10.1515/znc-1974-9-1010. [DOI] [PubMed] [Google Scholar]
- Helfrich W. Elastic properties of lipid bilayers: theory and possible experiments. Z Naturforsch C. 1973 Nov-Dec;28(11):693–703. doi: 10.1515/znc-1973-11-1209. [DOI] [PubMed] [Google Scholar]
- Hochmuth R. M., Mohandas N., Blackshear P. L., Jr Measurement of the elastic modulus for red cell membrane using a fluid mechanical technique. Biophys J. 1973 Aug;13(8):747–762. doi: 10.1016/S0006-3495(73)86021-7. [DOI] [PMC free article] [PubMed] [Google Scholar]
- Jenkins J. T. Static equilibrium configurations of a model red blood cell. J Math Biol. 1977 May 23;4(2):149–169. doi: 10.1007/BF00275981. [DOI] [PubMed] [Google Scholar]
- Kresch E. Cross-sectional area of flexible tubes. Bull Math Biol. 1979;41(1):39–52. doi: 10.1007/BF02547923. [DOI] [PubMed] [Google Scholar]
- Kresch E. Cross-sectional shape of flexible tubes. Bull Math Biol. 1977;39(6):679–691. doi: 10.1007/BF02461777. [DOI] [PubMed] [Google Scholar]
- Kresch E., Noordergraaf A. Cross-sectional shape of collapsible tubes. Biophys J. 1972 Mar;12(3):274–294. doi: 10.1016/S0006-3495(72)86086-7. [DOI] [PMC free article] [PubMed] [Google Scholar]
- Lentz B. R., Barenholz Y., Thompson T. E. Fluorescence depolarization studies of phase transitions and fluidity in phospholipid bilayers. 1. Single component phosphatidylcholine liposomes. Biochemistry. 1976 Oct 5;15(20):4521–4528. doi: 10.1021/bi00665a029. [DOI] [PubMed] [Google Scholar]
- Lentz B. R., Barenholz Y., Thompson T. E. Fluorescence depolarization studies of phase transitions and fluidity in phospholipid bilayers. 2 Two-component phosphatidylcholine liposomes. Biochemistry. 1976 Oct 5;15(20):4529–4537. doi: 10.1021/bi00665a030. [DOI] [PubMed] [Google Scholar]
- Lew H. S. Electro-tension and torque in biological membranes modeled as a dipole sheet in fluid conductors. J Biomech. 1972 Jul;5(4):399–408. doi: 10.1016/0021-9290(72)90067-x. [DOI] [PubMed] [Google Scholar]
- Lopez L., Duck I. M., Hunt W. A. On the shape of the erythrocyte. Biophys J. 1968 Nov;8(11):1228–1235. doi: 10.1016/S0006-3495(68)86552-X. [DOI] [PMC free article] [PubMed] [Google Scholar]
- Lutz H. U., Liu S. C., Palek J. Release of spectrin-free vesicles from human erythrocytes during ATP depletion. I. Characterization of spectrin-free vesicles. J Cell Biol. 1977 Jun;73(3):548–560. doi: 10.1083/jcb.73.3.548. [DOI] [PMC free article] [PubMed] [Google Scholar]
- Lutz H. U., Lomant A. J., McMillan P., Wehrli E. Rearrangements of integral membrane components during in vitro aging of sheep erythrocyte membranes. J Cell Biol. 1977 Aug;74(2):389–398. doi: 10.1083/jcb.74.2.389. [DOI] [PMC free article] [PubMed] [Google Scholar]
- MURPHY J. R. ERYTHROCYTE METABOLISM. VI. CELL SHAPE AND THE LOCATION OF CHOLESTEROL IN THE ERYTHROCYTE MEMBRANE. J Lab Clin Med. 1965 May;65:756–774. [PubMed] [Google Scholar]
- Mandersloot J. G., Reman F. C., Van Deenen L. L., De Gier J. Barrier properties of lecithin/lysolecithin mixtures. Biochim Biophys Acta. 1975 Feb 28;382(1):22–26. doi: 10.1016/0005-2736(75)90368-5. [DOI] [PubMed] [Google Scholar]
- Marikovsky Y., Khodadad J. K., Weinstein R. S. Influence of red cell shape on surface charge topography. Exp Cell Res. 1978 Oct 1;116(1):191–197. doi: 10.1016/0014-4827(78)90075-7. [DOI] [PubMed] [Google Scholar]
- Papahadjopoulos D., Poste G., Schaeffer B. E., Vail W. J. Membrane fusion and molecular segregation in phospholipid vesicles. Biochim Biophys Acta. 1974 May 30;352(1):10–28. doi: 10.1016/0005-2736(74)90175-8. [DOI] [PubMed] [Google Scholar]
- Petit V. A., Edidin M. Lateral phase separation of lipids in plasma membranes: effect of temperature on the mobility of membrane antigens. Science. 1974 Jun 14;184(4142):1183–1185. doi: 10.1126/science.184.4142.1183. [DOI] [PubMed] [Google Scholar]
- Poste G., Allison A. C. Membrane fusion. Biochim Biophys Acta. 1973 Dec 28;300(4):421–465. doi: 10.1016/0304-4157(73)90015-4. [DOI] [PubMed] [Google Scholar]
- Satir B., Schooley C., Satir P. Membrane fusion in a model system. Mucocyst secretion in Tetrahymena. J Cell Biol. 1973 Jan;56(1):153–176. doi: 10.1083/jcb.56.1.153. [DOI] [PMC free article] [PubMed] [Google Scholar]
- Schekman R., Singer S. J. Clustering and endocytosis of membrane receptors can be induced in mature erythrocytes of neonatal but not adult humans. Proc Natl Acad Sci U S A. 1976 Nov;73(11):4075–4079. doi: 10.1073/pnas.73.11.4075. [DOI] [PMC free article] [PubMed] [Google Scholar]
- Schmid-Schöenbein H., Wells R. Fluid drop-like transition of erythrocytes under shear. Science. 1969 Jul 18;165(3890):288–291. doi: 10.1126/science.165.3890.288. [DOI] [PubMed] [Google Scholar]
- Sheetz M. P., Chan S. I. Effect of sonication on the structure of lecithin bilayers. Biochemistry. 1972 Nov 21;11(24):4573–4581. doi: 10.1021/bi00774a024. [DOI] [PubMed] [Google Scholar]
- Sheetz M. P., Painter R. G., Singer S. J. Biological membranes as bilayer couples. III. Compensatory shape changes induced in membranes. J Cell Biol. 1976 Jul;70(1):193–203. doi: 10.1083/jcb.70.1.193. [DOI] [PMC free article] [PubMed] [Google Scholar]
- Sheetz M. P., Singer S. J. Equilibrium and kinetic effects of drugs on the shapes of human erythrocytes. J Cell Biol. 1976 Jul;70(1):247–251. doi: 10.1083/jcb.70.1.247. [DOI] [PMC free article] [PubMed] [Google Scholar]
- Singer S. J., Nicolson G. L. The fluid mosaic model of the structure of cell membranes. Science. 1972 Feb 18;175(4023):720–731. doi: 10.1126/science.175.4023.720. [DOI] [PubMed] [Google Scholar]
- Skalak R., Tozeren A., Zarda R. P., Chien S. Strain energy function of red blood cell membranes. Biophys J. 1973 Mar;13(3):245–264. doi: 10.1016/S0006-3495(73)85983-1. [DOI] [PMC free article] [PubMed] [Google Scholar]
- Spertell R. B. On the decay of lateral phase separations in biological membranes. J Theor Biol. 1978 Mar 7;71(1):1–9. doi: 10.1016/0022-5193(78)90209-6. [DOI] [PubMed] [Google Scholar]
- Spiker R. C., Levin I. W. Phase transitions of phospholipid single-wall vesicles and multilayers. Measurement by vibrational Raman spectroscopic frequency differences. Biochim Biophys Acta. 1976 May 21;433(3):457–468. doi: 10.1016/0005-2736(76)90273-x. [DOI] [PubMed] [Google Scholar]
- Suurkuusk J., Lentz B. R., Barenholz Y., Biltonen R. L., Thompson T. E. A calorimetric and fluorescent probe study of the gel-liquid crystalline phase transition in small, single-lamellar dipalmitoylphosphatidylcholine vesicles. Biochemistry. 1976 Apr 6;15(7):1393–1401. doi: 10.1021/bi00652a007. [DOI] [PubMed] [Google Scholar]
- Warren G. B., Houslay M. D., Metcalfe J. C., Birdsall N. J. Cholesterol is excluded from the phospholipid annulus surrounding an active calcium transport protein. Nature. 1975 Jun 26;255(5511):684–687. doi: 10.1038/255684a0. [DOI] [PubMed] [Google Scholar]
- Yu J., Fischman D. A., Steck T. L. Selective solubilization of proteins and phospholipids from red blood cell membranes by nonionic detergents. J Supramol Struct. 1973;1(3):233–248. doi: 10.1002/jss.400010308. [DOI] [PubMed] [Google Scholar]
- Ziparo E., Lemay A., Marchesi V. T. The distribution of spectrin along the membranes of normal and echinocytic human erythrocytes. J Cell Sci. 1978 Dec;34:91–101. doi: 10.1242/jcs.34.1.91. [DOI] [PubMed] [Google Scholar]
- de Petris S. Preferential distribution of surface immunoglobulins on microvilli. Nature. 1978 Mar 2;272(5648):66–68. doi: 10.1038/272066a0. [DOI] [PubMed] [Google Scholar]