Abstract
The mechanism for transport of divalent cations across phospholipid bilayers by the ionophore A23187 was investigated. The intrinsic fluorescence of the ionophore was used in equilibrium and rapid-mixing experiments as an indicator of ionophore environment and complexation with divalent cations. The neutral (protonated) form of the ionophore binds strongly to the membrane, with a high quantum yield relative to that in the aqueous phase. The negatively charged form of the ionophore binds somewhat less strongly, with a lower quantum yield, and does not move across the membrane. Complexation of the negatively charged form with divalent cations was measured by the decrease in fluorescence. An apparent rate constant (kapp) for transport of the ionophore across the membrane was determined from the rate of fluorescence changes observed in stopped-flow rapid kinetic experiments. The variation of kapp was studied as a function of pH, temperature, ionophore concentration, membrane lipid composition, and divalent cation concentration and type. Analysis and comparison with equilibrium constants for protonation and complexation show that A23187 and its metal:ionophore complexes bind near the membrane-water interface in the lipid polar-head region. The interfacial reactions occur rapidly, compared with the transmembrane reactions, and are thus in equilibrium during transport. The transport cycle can be described as follows: a 1:1 complex is formed between the membrane bound A23187-(Am-) and the aqueous divalent cation with dissociation constant K1 approximately 4.6 x 10(-4) M. This is in equilibrium with a 1:2 (metal:ionophore) complex (K2 approximately 3.0 x 10(-4) [ionophore/lipid]) that is responsible for transporting the divalent cations across the membrane. The rate constant for translocation of the 1:2 complex is 0.1-0.3 s-1. Dissociation of the complex of the trans side and protonation occur rapidly. The rate constant for translocation of H+ . A23187- is 28 s-1. A theory is presented that is capable of reproducing the kinetic data at any calcium concentration. The cation specificity for ionophore complex transport (kapp), determined at low ionophore concentration for a series of divalent cations, was found to be proportional to the equilibrium constant for 1:1 complexation. The order of ion specificity for these processes was found to be Ca2+ greater than Mg2+ greater Sr2+ greater than Ba2+. Interactions with Na+ were not observed. Maximal values of kapp were observed for vesicles prepared from pure dimyristoyl phosphatidylcholine. Inclusion of phosphatidyl ethanolamine, phosphatidic acid, or dipalmatoyl phosphatidylcholine resulted in lower values of kapp. Calcium transport by A23187 is compared with that of X537A, and it is shown that the former is 67-fold faster. The difference in rates is due to differences in the ability of each ionophore to form a 1:2 complex from a 1:1 complex.
Full text
PDF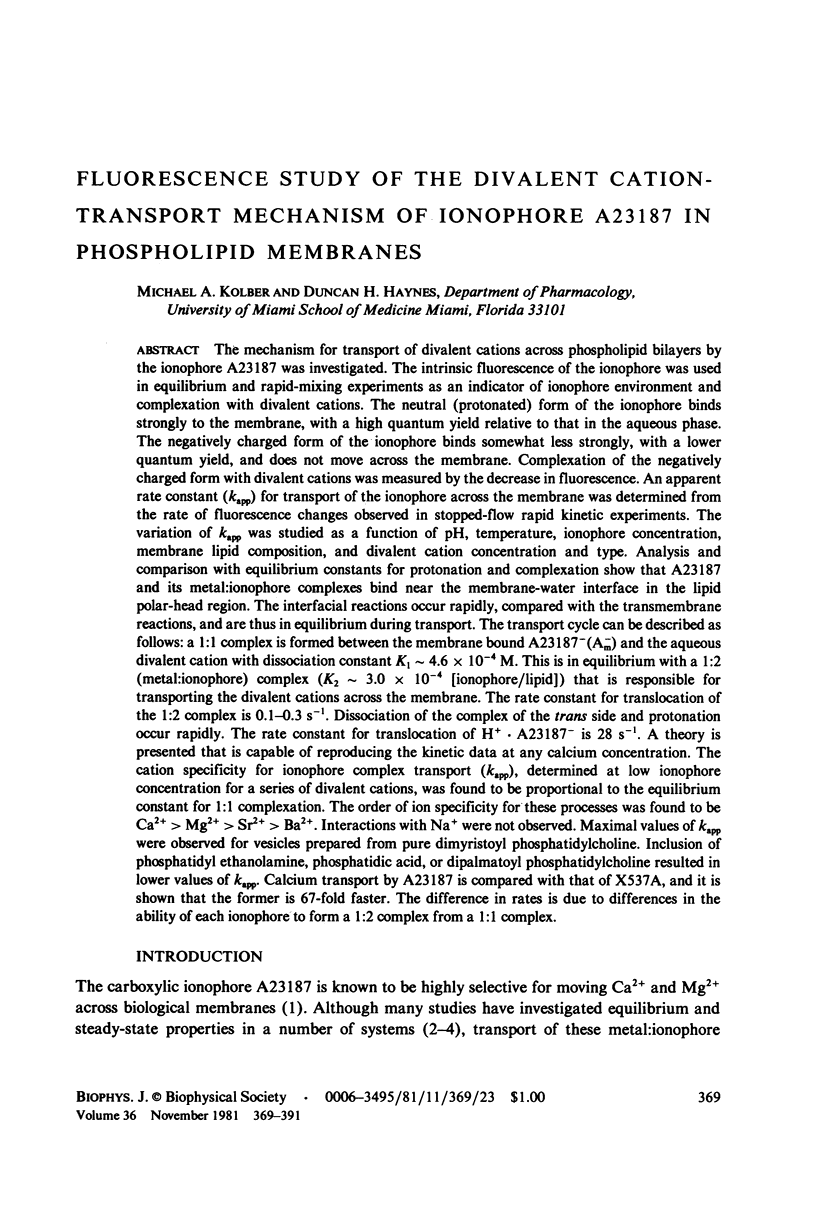
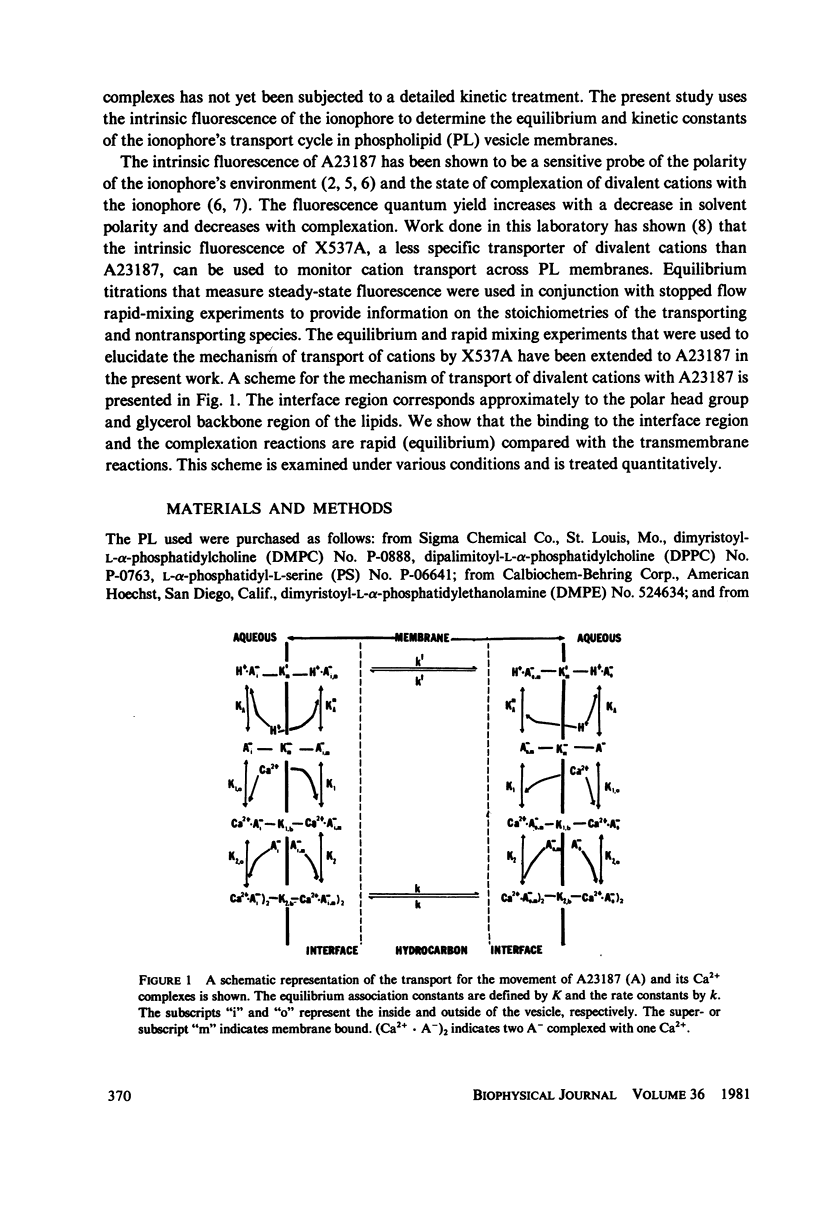
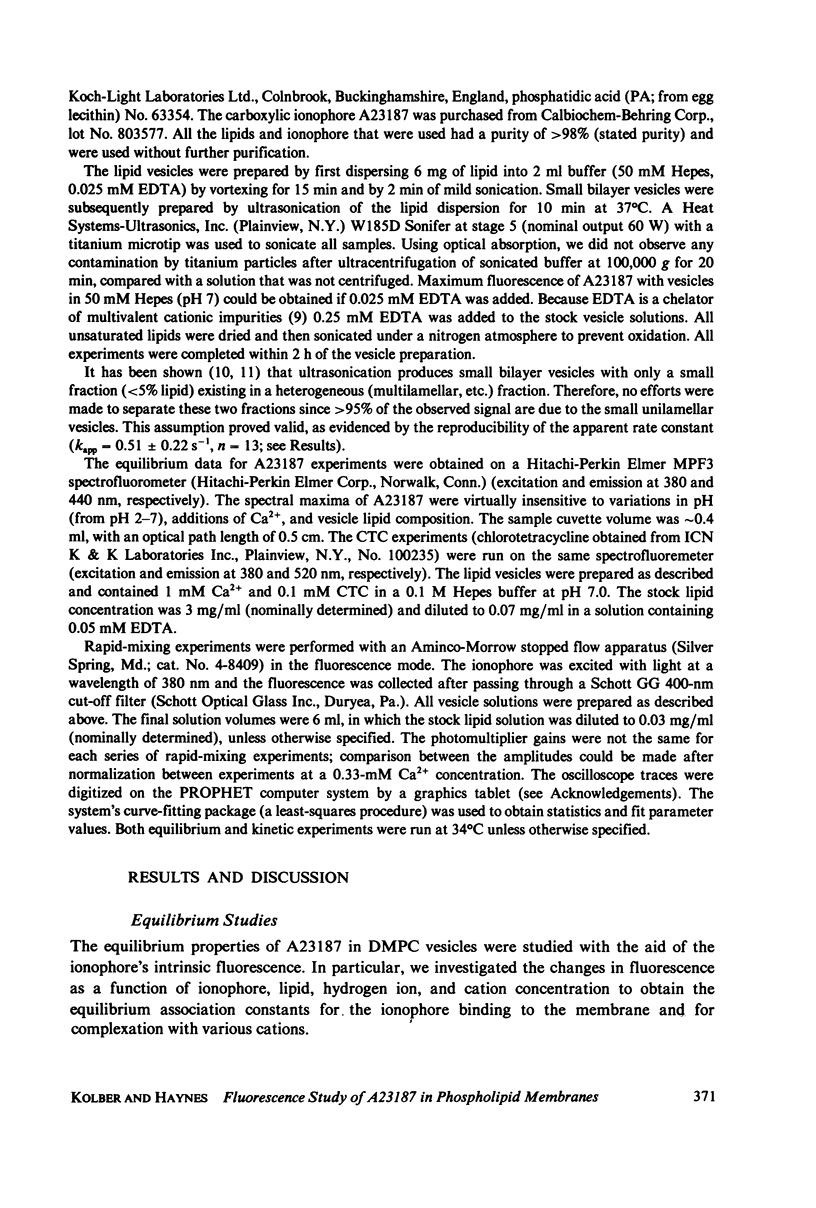
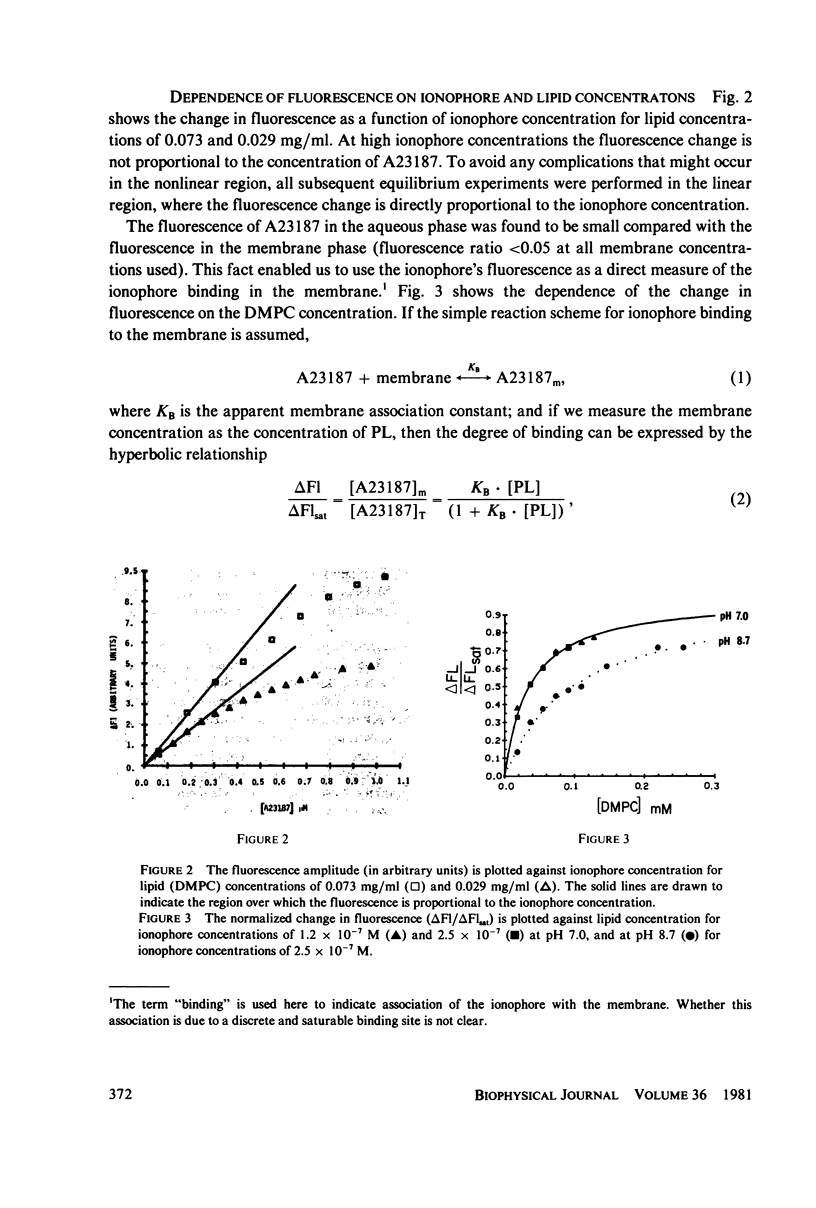
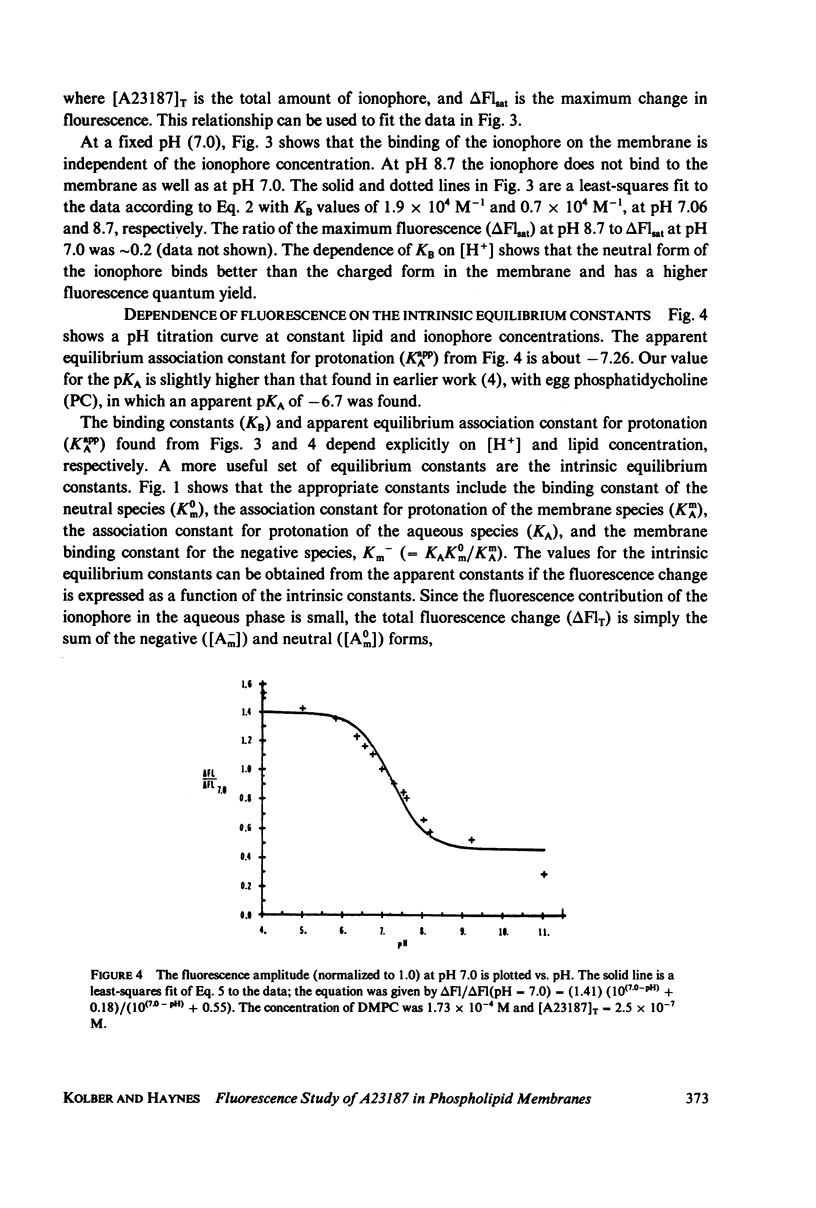
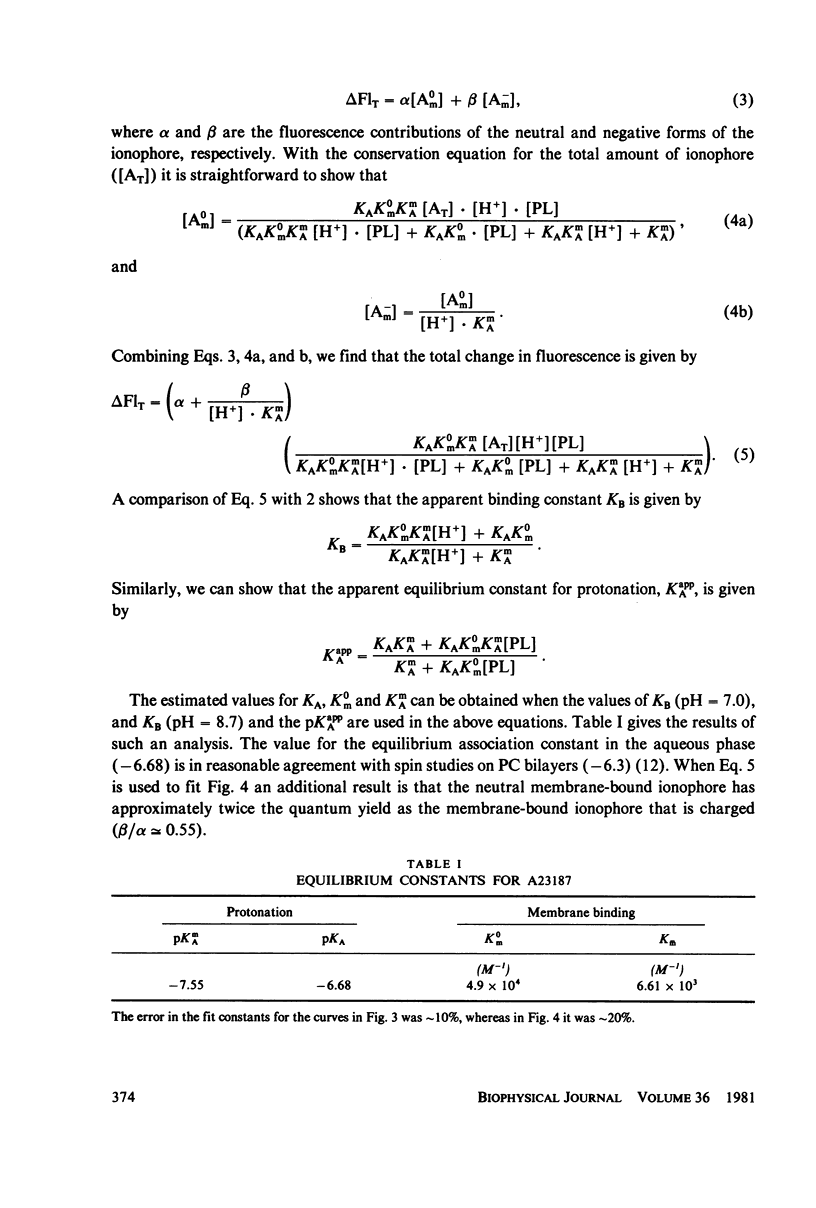
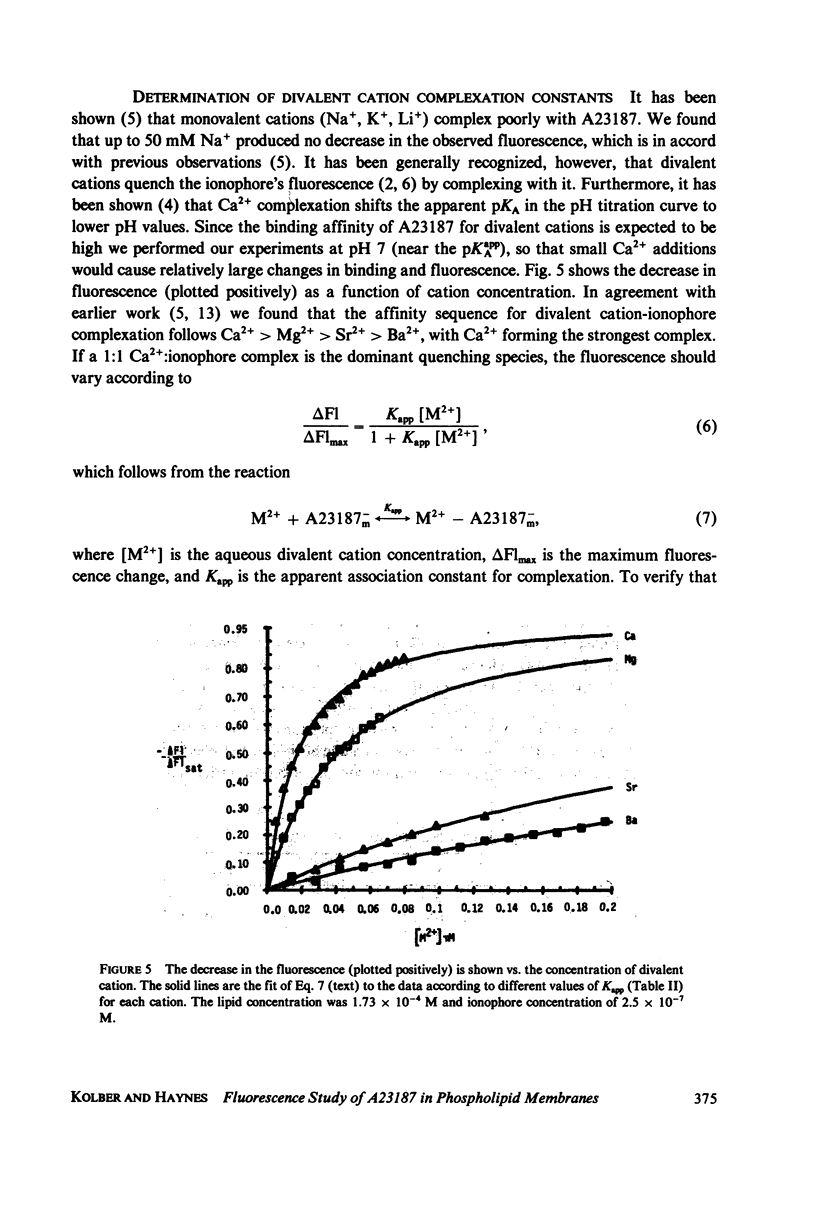
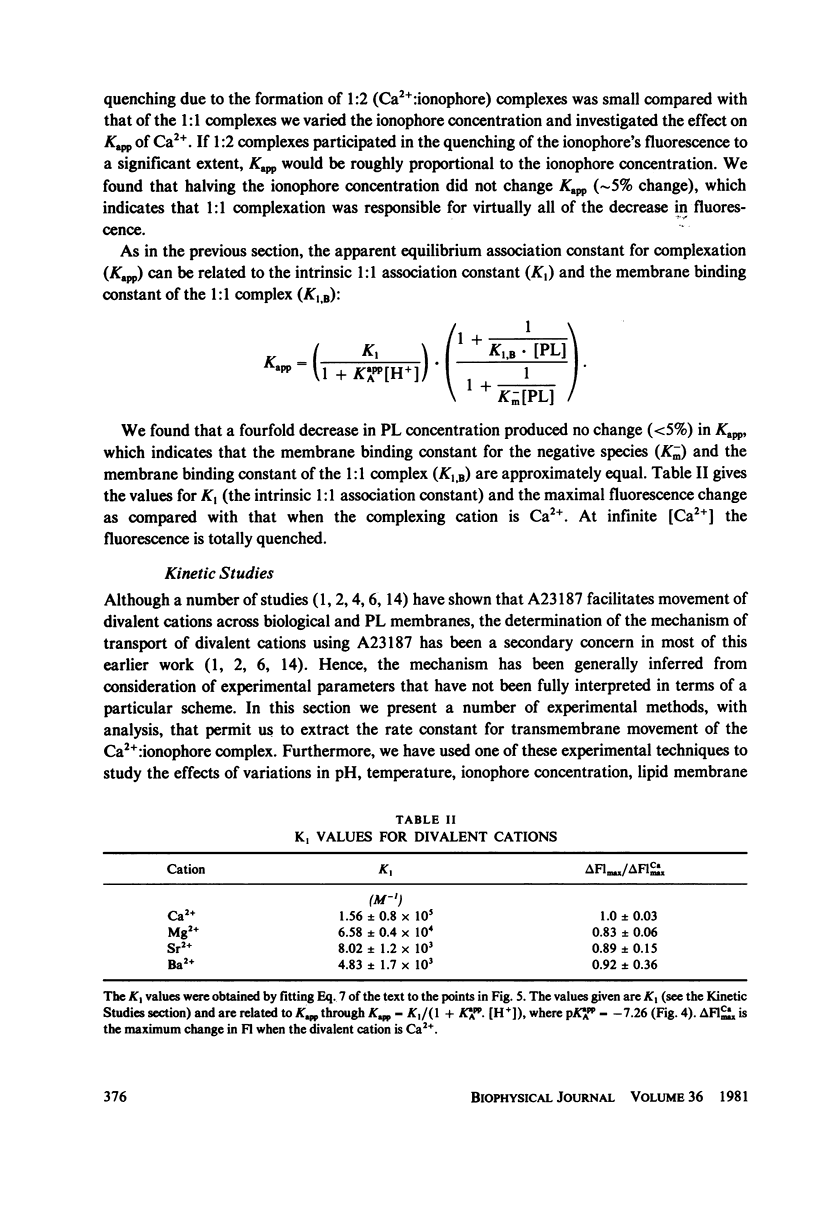
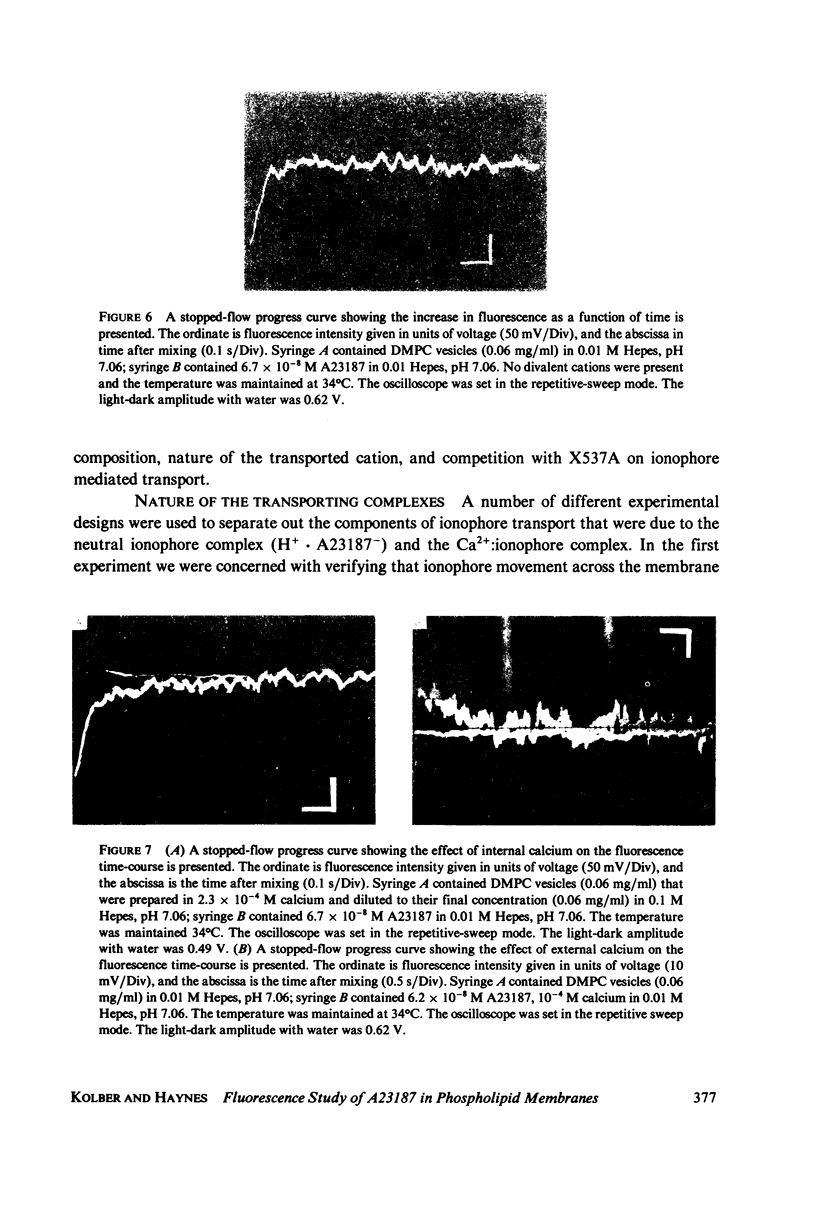
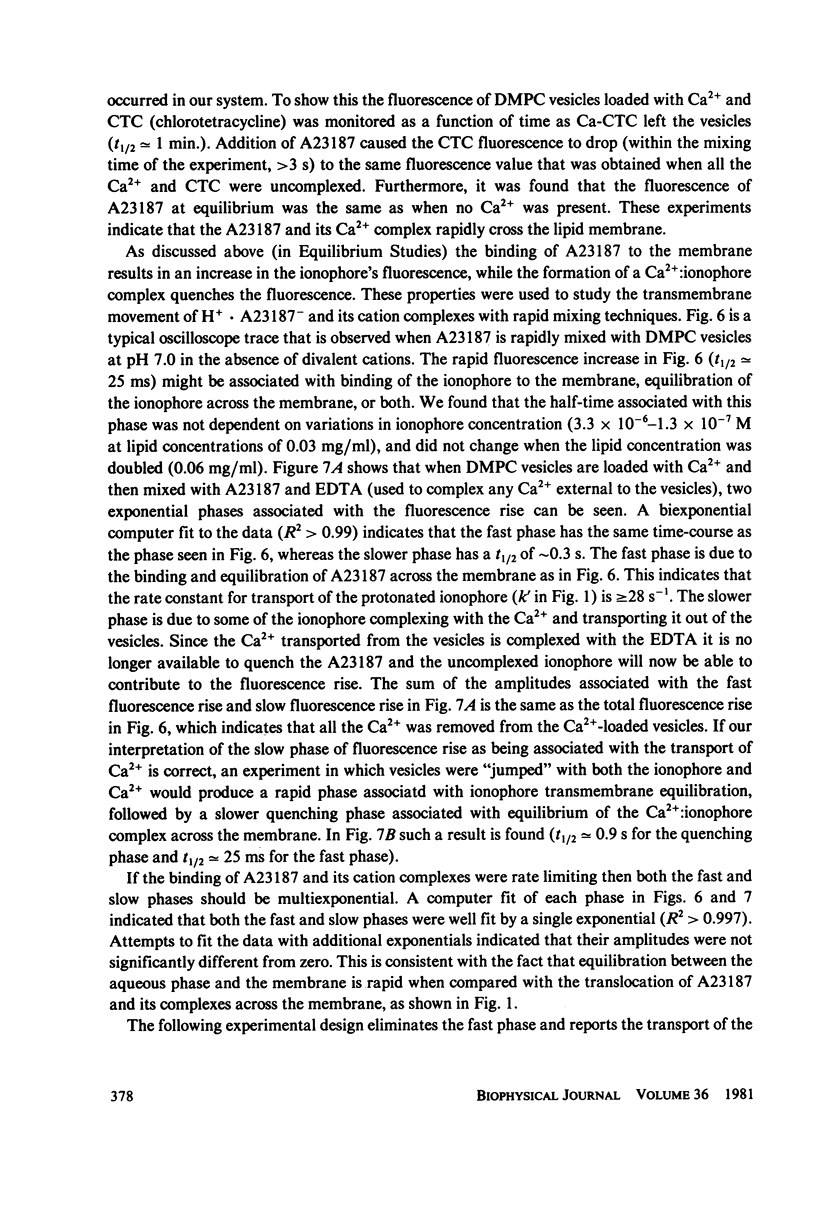
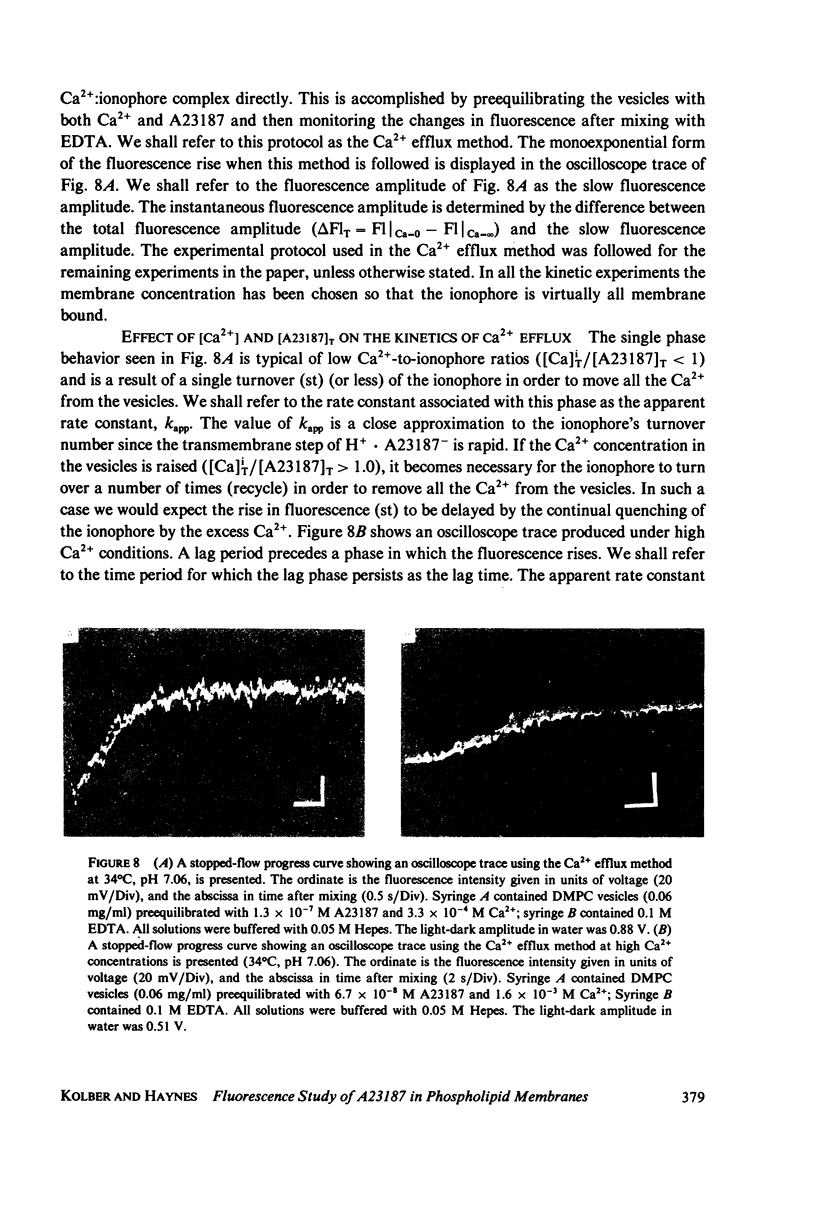
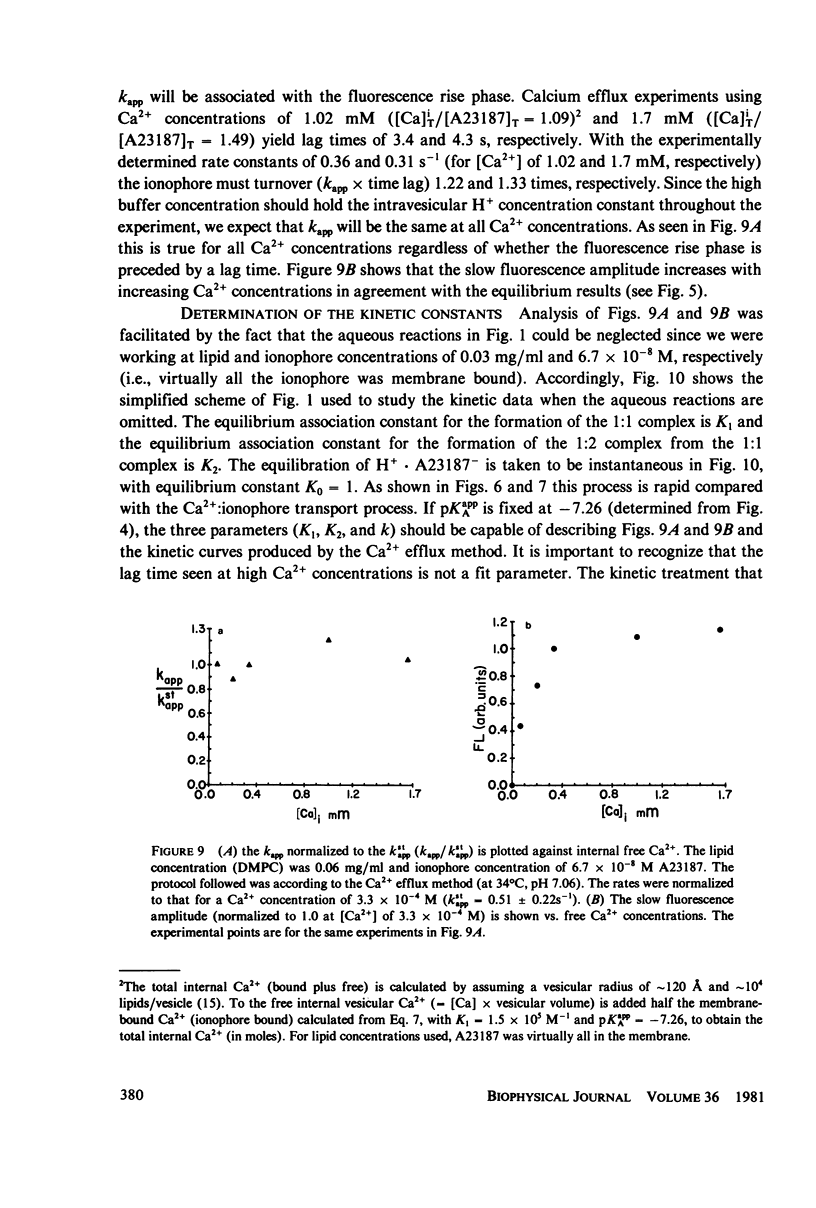
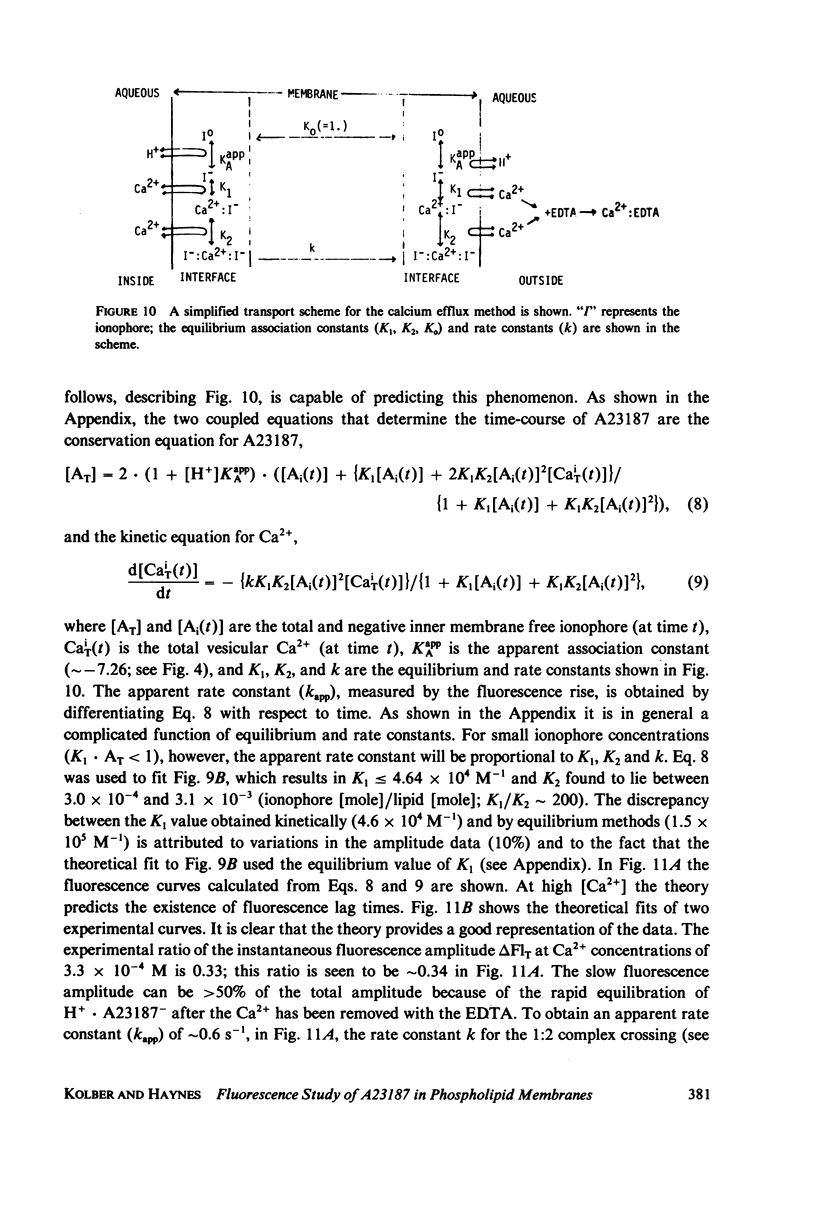
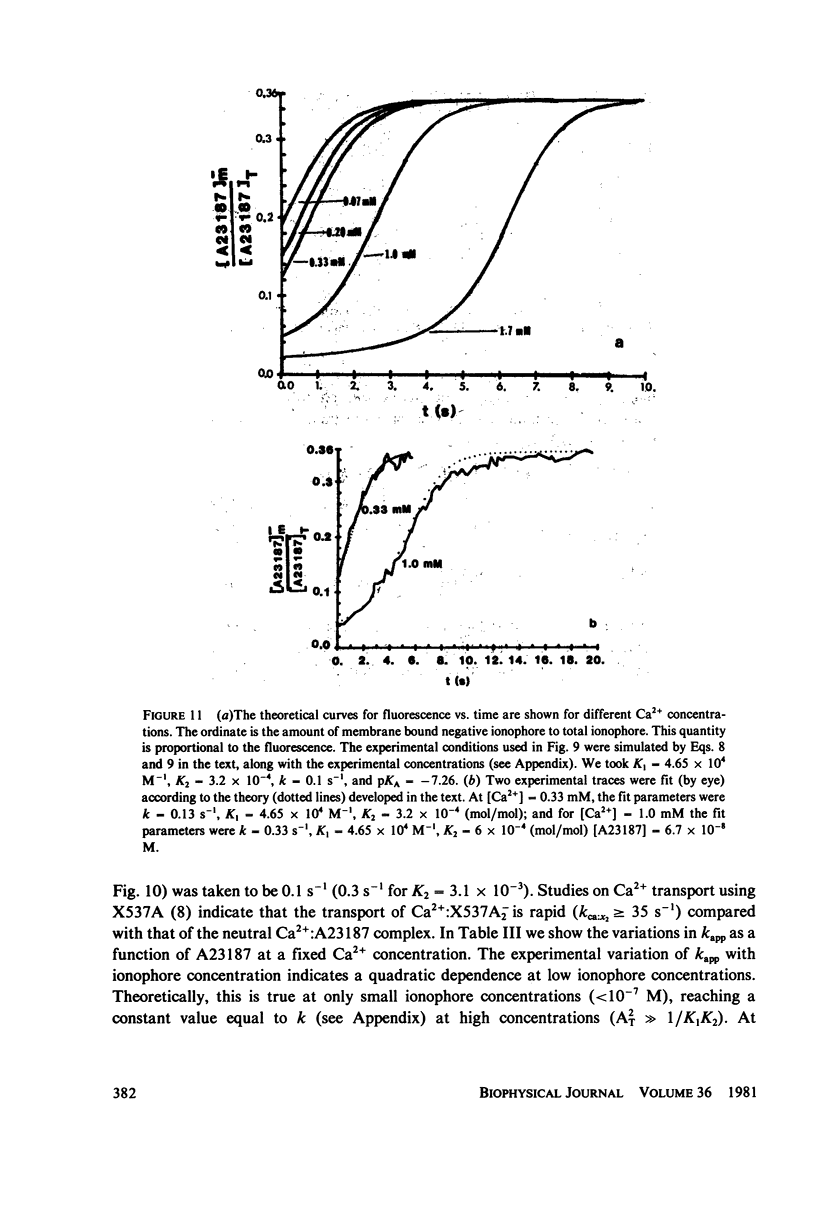
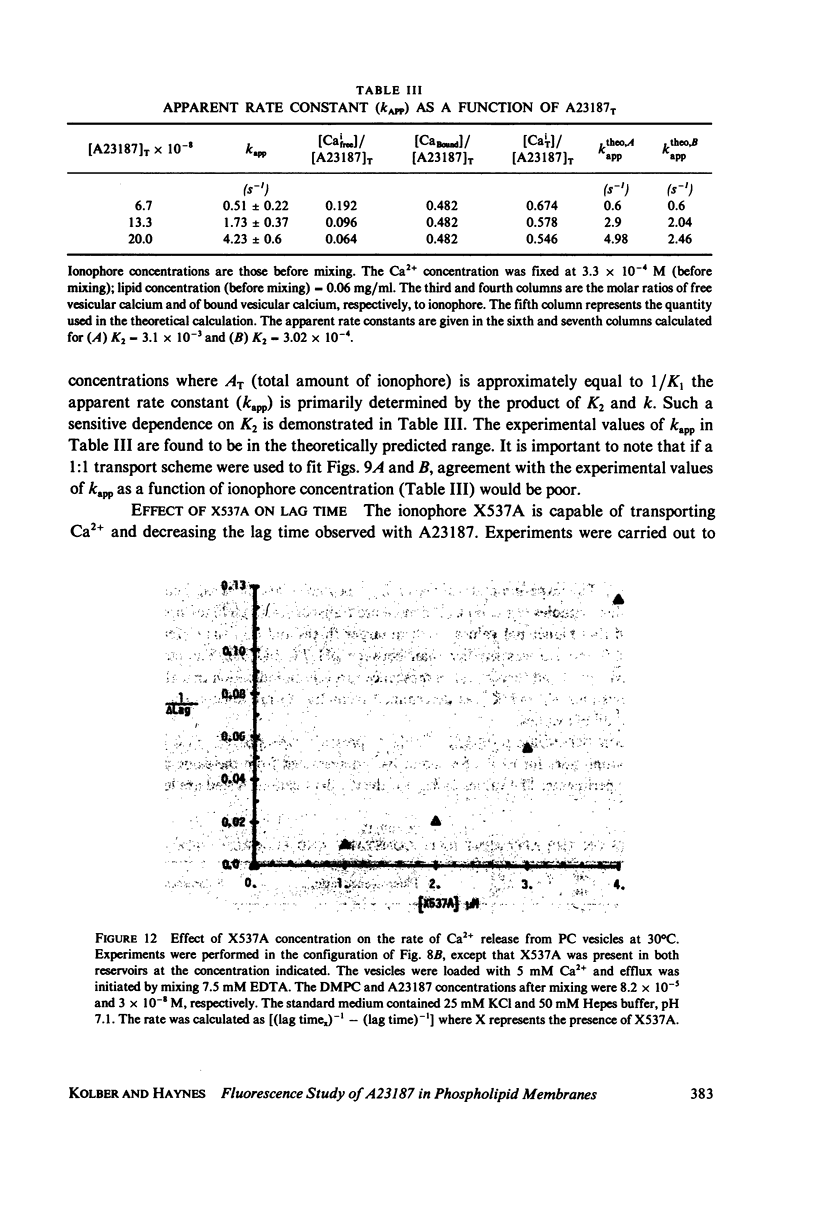
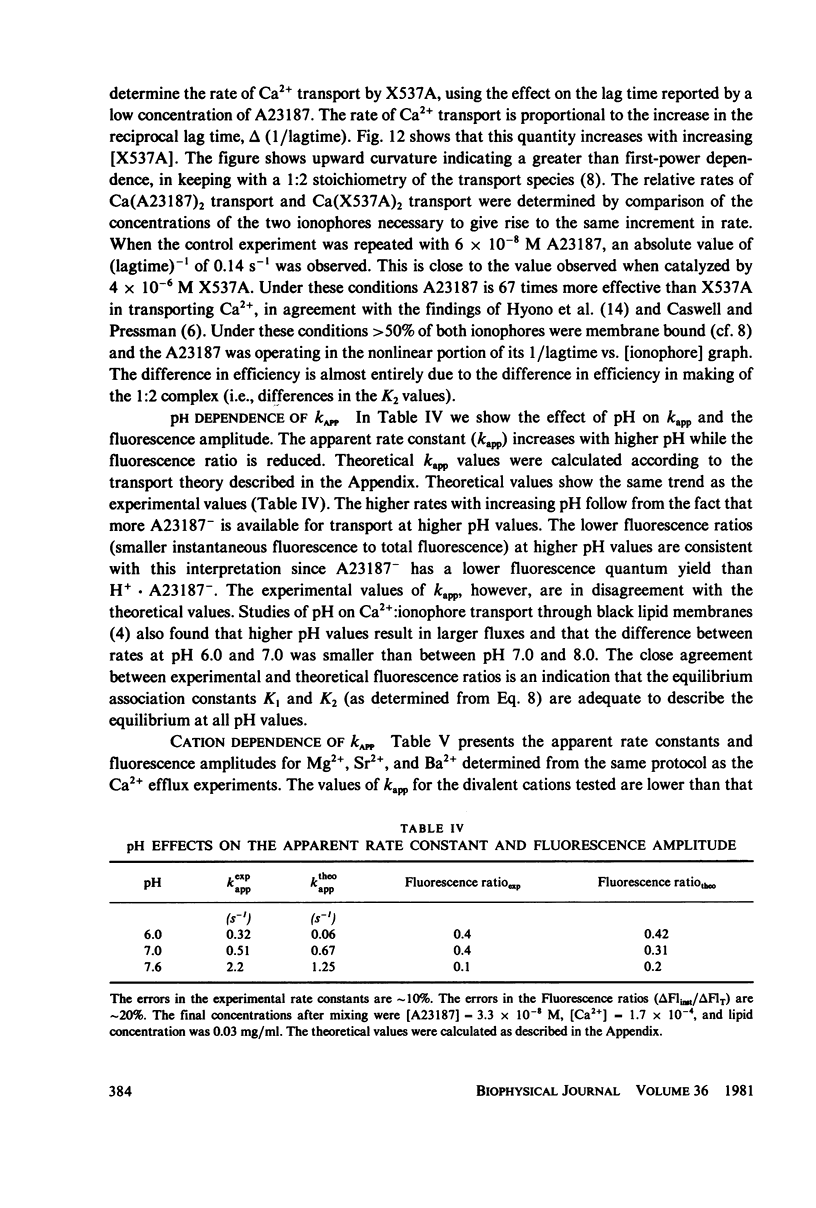
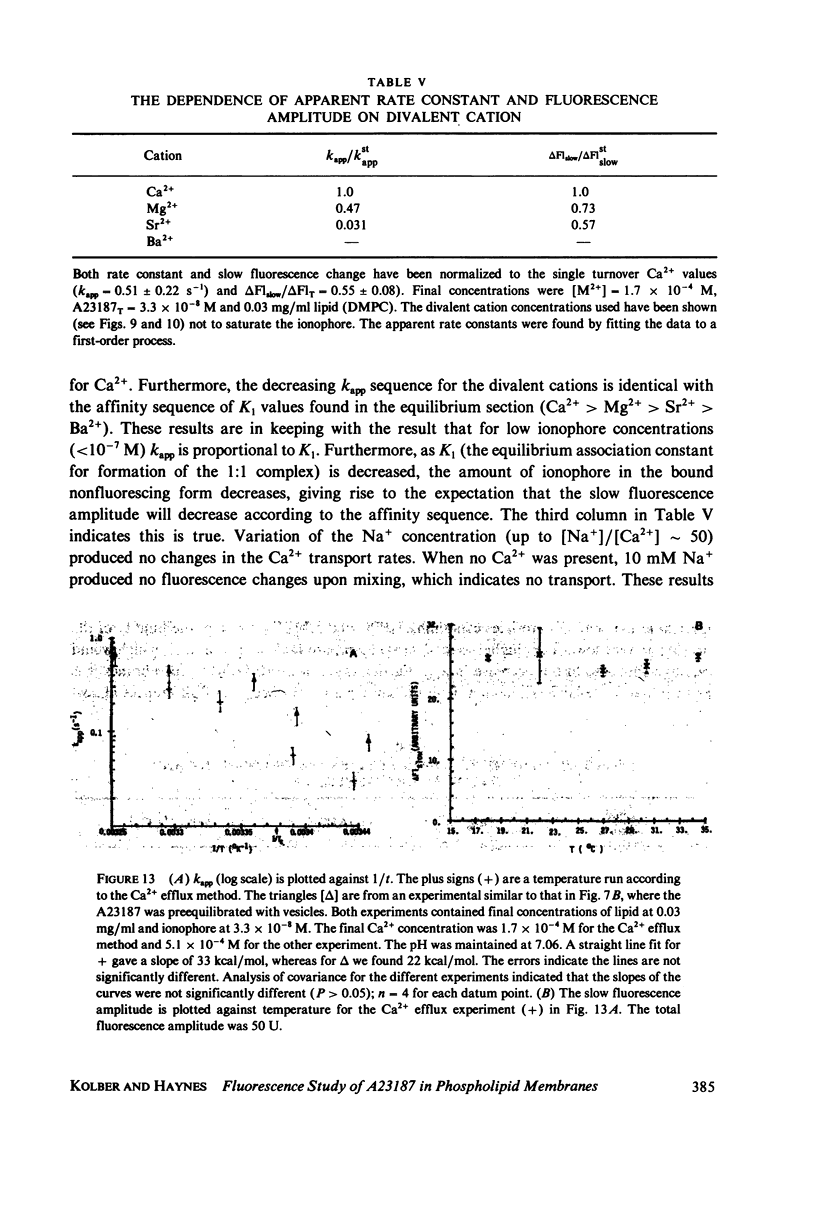
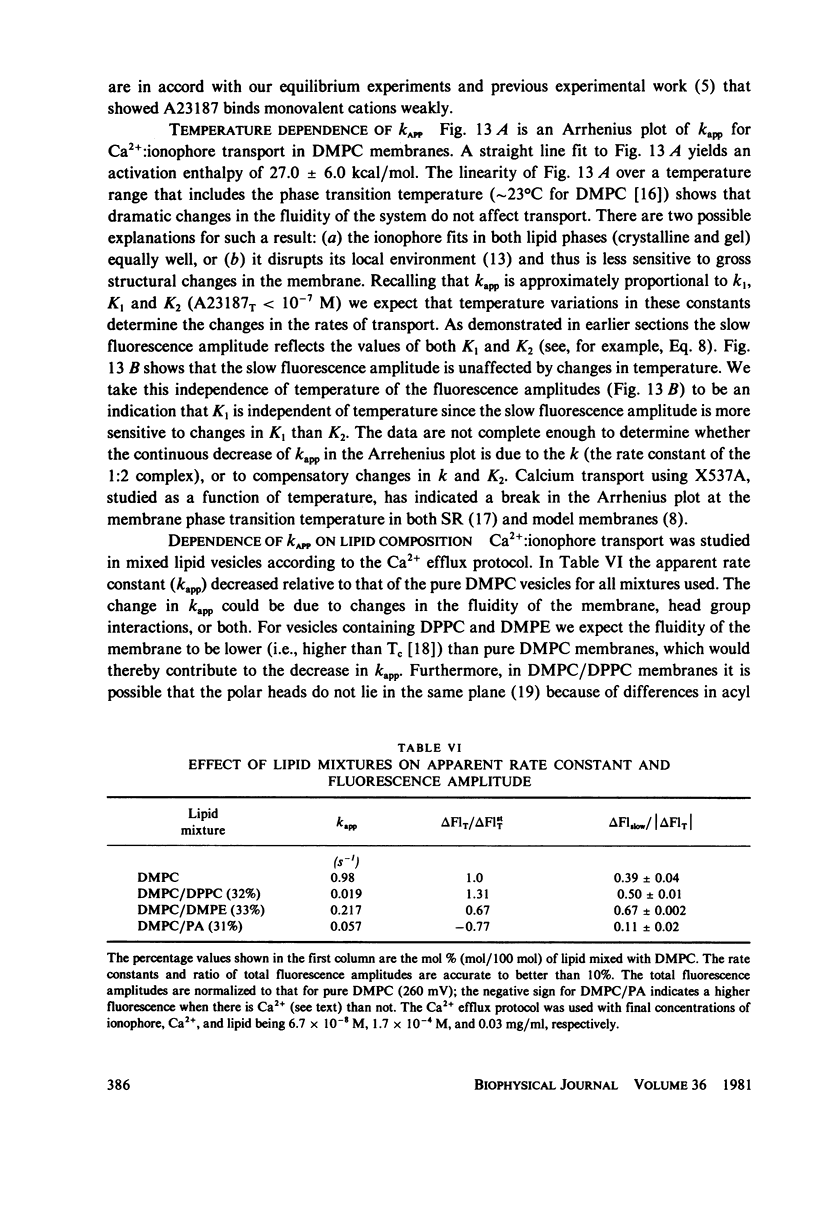
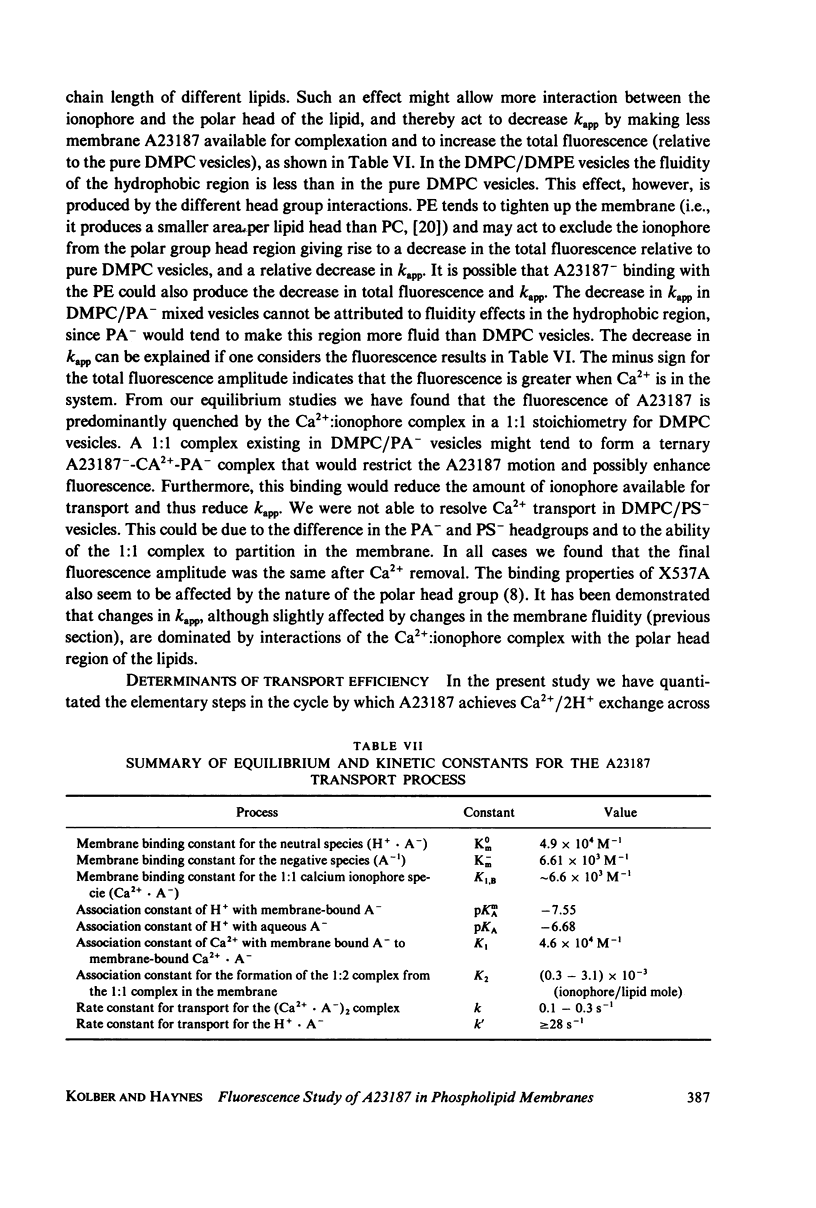
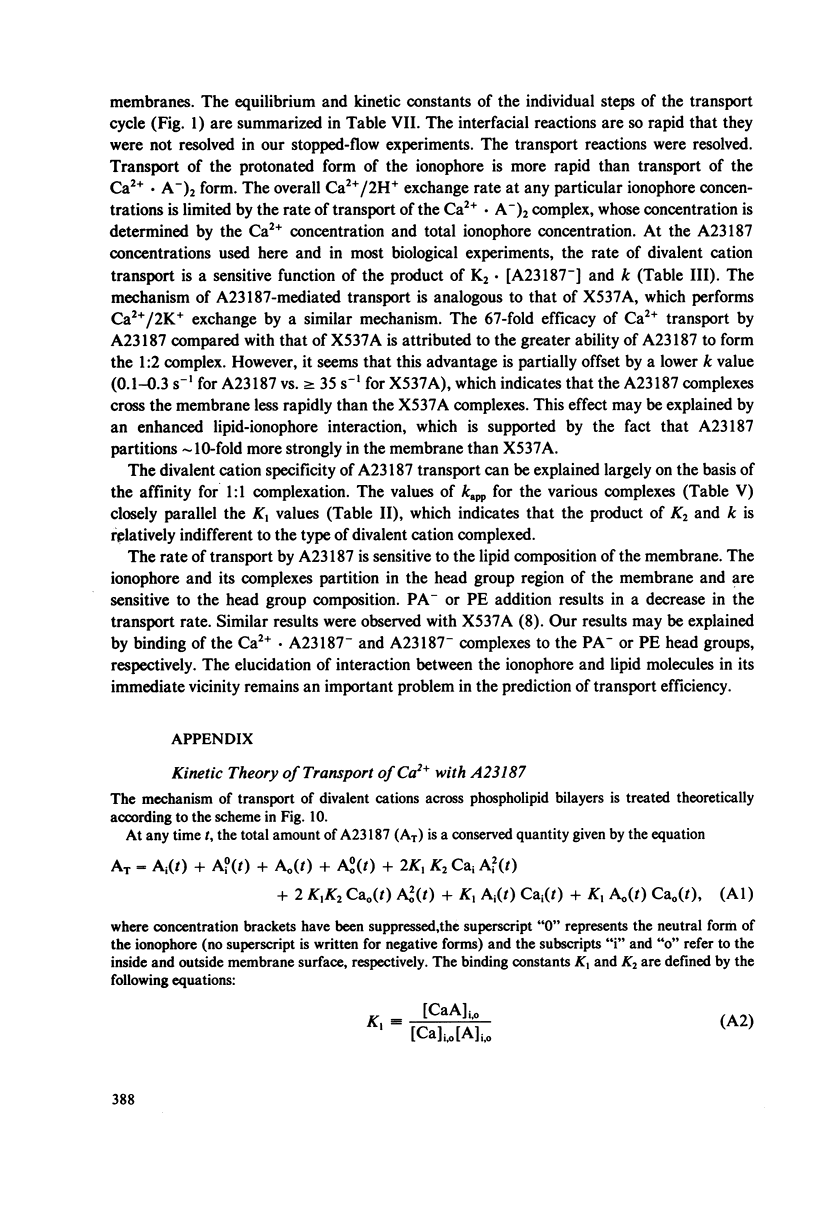
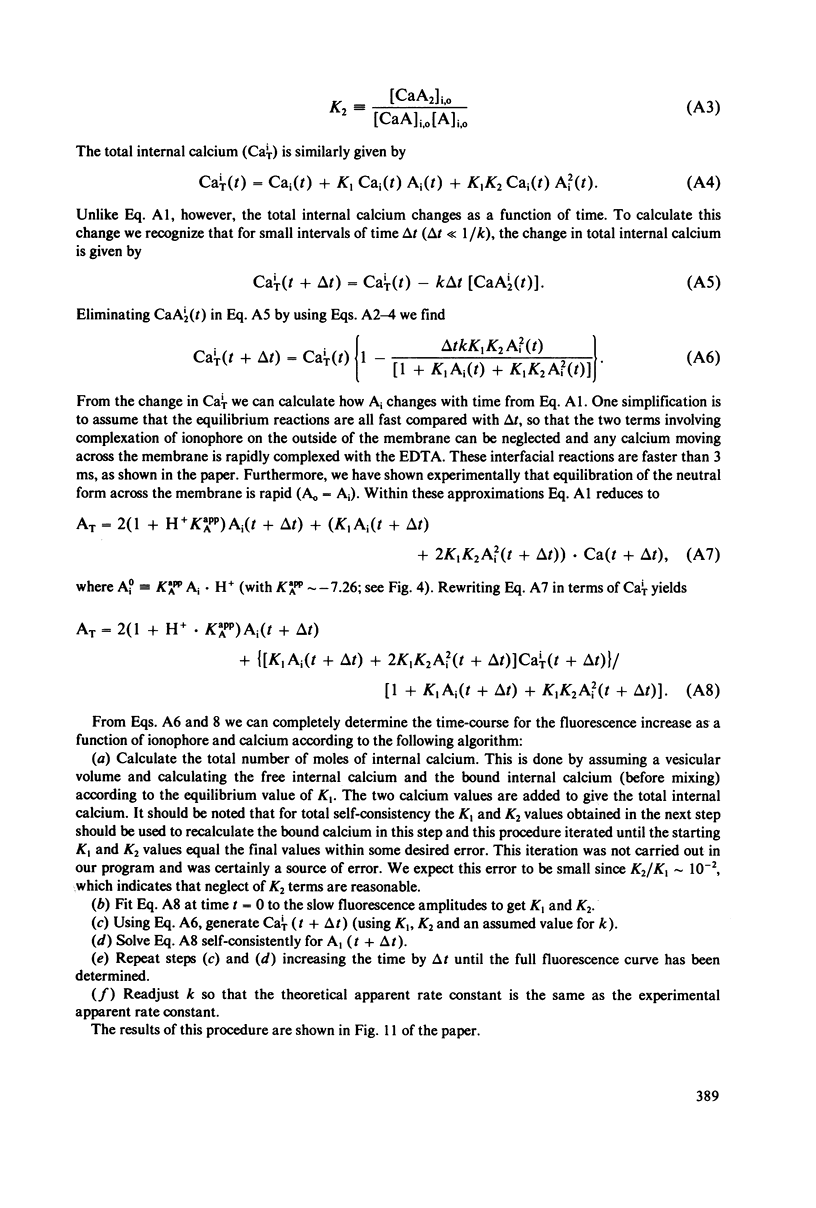
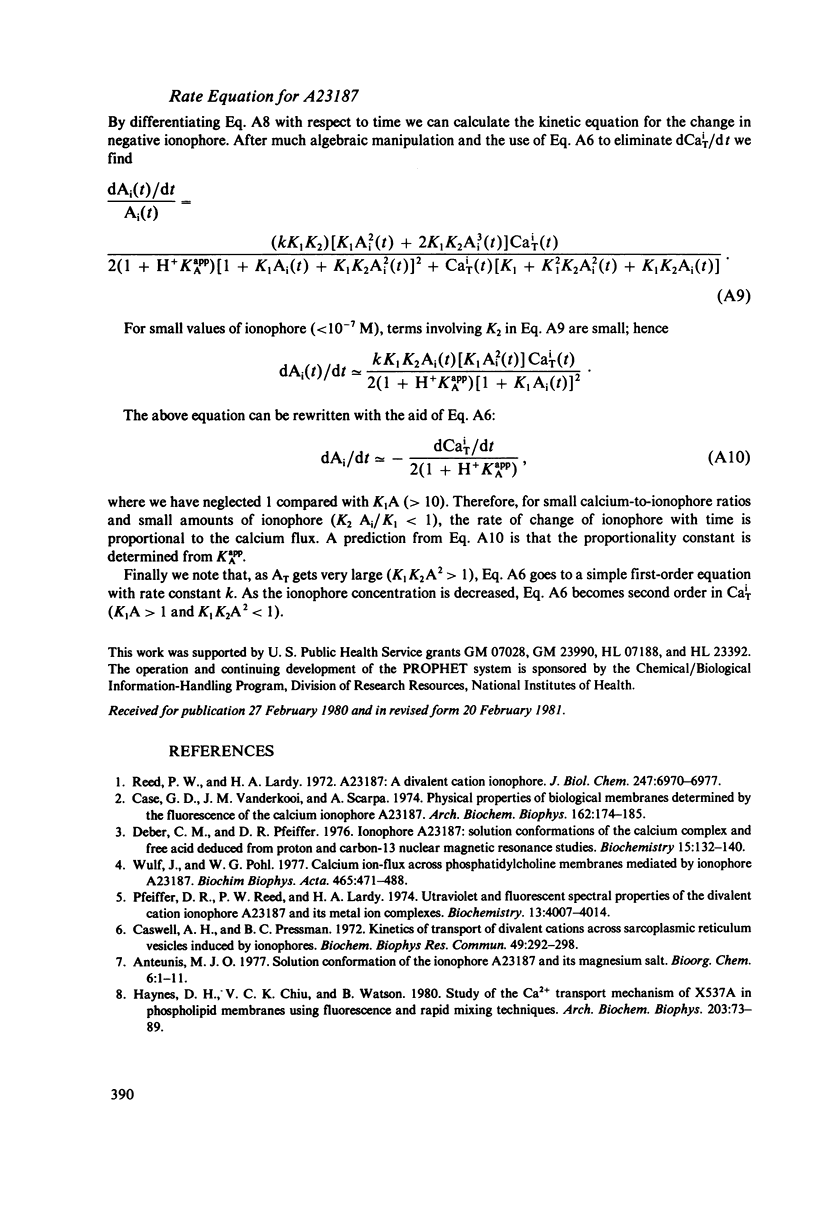
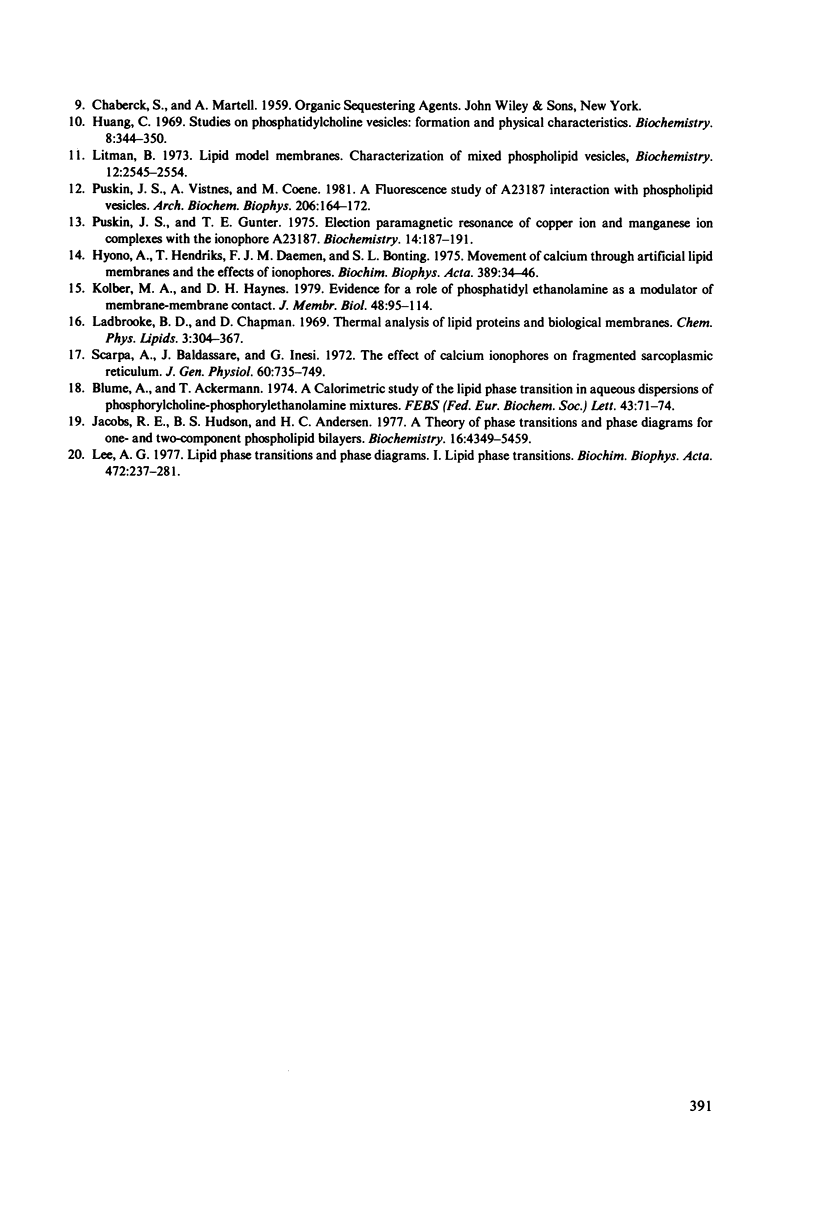
Images in this article
Selected References
These references are in PubMed. This may not be the complete list of references from this article.
- Blume A., Ackermann T. A calorimetric study of the lipid phase transitions in aqueous dispersions of phosphorylcholine--phosphorylethanolamine mixtures. FEBS Lett. 1974 Jul 1;43(1):71–74. doi: 10.1016/0014-5793(74)81108-7. [DOI] [PubMed] [Google Scholar]
- Case G. D., Vanderkooi J. M., Scarpa A. Physical properties of biological membranes determined by the fluorescence of the calcium ionophore A23187. Arch Biochem Biophys. 1974 May;162(1):174–185. doi: 10.1016/0003-9861(74)90116-7. [DOI] [PubMed] [Google Scholar]
- Caswell A. H., Pressman B. C. Kinetics of transport of divalent cations across sarcoplasmic reticulum vesicles induced by ionophores. Biochem Biophys Res Commun. 1972 Oct 6;49(1):292–298. doi: 10.1016/0006-291x(72)90043-5. [DOI] [PubMed] [Google Scholar]
- Deber C. M., Pfieffer D. R. Ionophore A23187. Solution conformations of the calcium complex and free acid deduced from proton and carbon-13 nuclear magnetic resonance studies. Biochemistry. 1976 Jan 13;15(1):132–141. doi: 10.1021/bi00646a020. [DOI] [PubMed] [Google Scholar]
- Haynes D. H., Chiu V. C., Watson B. Study of the Ca2+ transport mechanism of X537A in phospholipid membranes using fluorescence and rapid kinetic techniques. Arch Biochem Biophys. 1980 Aug;203(1):73–89. doi: 10.1016/0003-9861(80)90155-1. [DOI] [PubMed] [Google Scholar]
- Huang C. Studies on phosphatidylcholine vesicles. Formation and physical characteristics. Biochemistry. 1969 Jan;8(1):344–352. doi: 10.1021/bi00829a048. [DOI] [PubMed] [Google Scholar]
- Hyono A., Hendriks T., Daemen F. J., Bonting S. L. Movement of calcium through artificial lipid membranes and the effects of ionophores. Biochim Biophys Acta. 1975 Apr 21;389(1):34–46. doi: 10.1016/0005-2736(75)90383-1. [DOI] [PubMed] [Google Scholar]
- Jacobs R. E., Hudson B. S., Andersen H. C. A theory of phase transitions and phase diagrams for one- and two-component phospholipid bilayers. Biochemistry. 1977 Oct 4;16(20):4349–4359. doi: 10.1021/bi00639a004. [DOI] [PubMed] [Google Scholar]
- Kolber M. A., Haynes D. H. Evidence for a role of phosphatidyl ethanolamine as a modulator of membrane-membrane contact. J Membr Biol. 1979 Jun 29;48(1):95–114. doi: 10.1007/BF01869258. [DOI] [PubMed] [Google Scholar]
- Ladbrooke B. D., Chapman D. Thermal analysis of lipids, proteins and biological membranes. A review and summary of some recent studies. Chem Phys Lipids. 1969 Dec;3(4):304–356. doi: 10.1016/0009-3084(69)90040-1. [DOI] [PubMed] [Google Scholar]
- Lee A. G. Lipid phase transitions and phase diagrams. I. Lipid phase transitions. Biochim Biophys Acta. 1977 Aug 9;472(2):237–281. doi: 10.1016/0304-4157(77)90018-1. [DOI] [PubMed] [Google Scholar]
- Litman B. J. Lipid model membranes. Characterization of mixed phospholipid vesicles. Biochemistry. 1973 Jun 19;12(13):2545–2554. doi: 10.1021/bi00737a028. [DOI] [PubMed] [Google Scholar]
- Pfeiffer D. R., Reed P. W., Lardy H. A. Ultraviolet and fluorescent spectral properties of the divalent cation ionophore A23187 and its metal ion complexes. Biochemistry. 1974 Sep 10;13(19):4007–4014. doi: 10.1021/bi00716a029. [DOI] [PubMed] [Google Scholar]
- Puskin J. S., Gunter T. E. Electron paramagnetic resonance of copper ion and manganese ion complexes with the ionophore A23187. Biochemistry. 1975 Jan 14;14(1):187–191. doi: 10.1021/bi00672a031. [DOI] [PubMed] [Google Scholar]
- Puskin J. S., Vistnes A. I., Coene M. T. A fluorescence study of A23187 interaction with phospholipid vesicles. Arch Biochem Biophys. 1981 Jan;206(1):164–172. doi: 10.1016/0003-9861(81)90077-1. [DOI] [PubMed] [Google Scholar]
- Reed P. W., Lardy H. A. A23187: a divalent cation ionophore. J Biol Chem. 1972 Nov 10;247(21):6970–6977. [PubMed] [Google Scholar]
- Scarpa A., Baldassare J., Inesi G. The effect of calcium ionophores on fragmented sarcoplasmic reticulum. J Gen Physiol. 1972 Dec;60(6):735–749. doi: 10.1085/jgp.60.6.735. [DOI] [PMC free article] [PubMed] [Google Scholar]
- Wulf J., Pohl W. G. Calcium ion-flux across phosphatidylcholine membranes mediated by ionophore A23187. Biochim Biophys Acta. 1977 Mar 17;465(3):471–485. doi: 10.1016/0005-2736(77)90266-8. [DOI] [PubMed] [Google Scholar]