Abstract
The interaction of a class of oxonol dyes with sonicated phospholipid vesicles was followed optically. The spectra of vesicle-associated dyes resemble those found for the dyes in organic solvents, indicating that the oxonols occupy a hydrophobic region of the membrane. At equilibrium the affinity of the oxonols for the vesicles depends on the structure of the dye, the physical and chemical composition of the vesicles, and the ionic strength of the medium. The oxonols occupy soybean lipid vesicles to a level of 147.9 ± 17.1 nmol/mg lipid with a dye membrane dissociation constant of 3.33 ± 0.54 μM. The interaction of the oxonols with soybean lipid vesicles is biphasic. The fast phase has a second order rate constant of 9.04 ± 0.36 × 106M-1 s-1 and the number of “fast” binding sites, 68 ± 8 nmol/mg lipid, was determined from the ratio of the second order rate constants obtained with lipid and with dye in excess. The dissociation of oxonols from soybean lipid vesicles is also biphasic, and the fast process has a rate constant of 17 ± 2 s-1, yielding a dissociation constant for the fast sites (k-1/k2) of 1.88 ± 0.15 μM. The slow phases of oxonol association with, and release from, soybean lipid vesicles are not second order and have half times of between 0.2 and 5 min, depending on the physical and chemical composition of the membrane lipids. The amplitudes of the slow phases are sensitive to the composition of the aqueous media on each side of the vesicle membranes, which suggests that the slow processes represent the permeation of the membrane by the oxonols. The importance of the properties of the oxonol dyes in the interpretation of their behavior in natural membranes is discussed.
Full text
PDF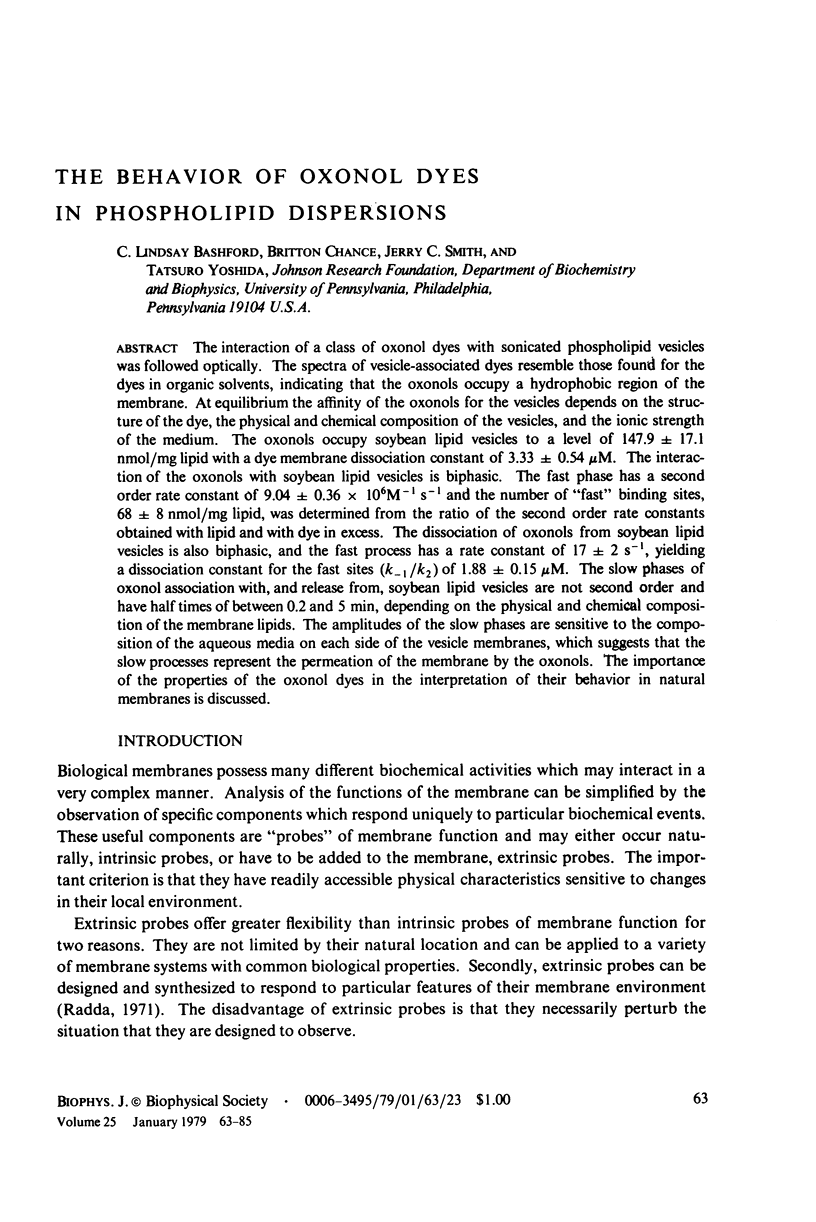
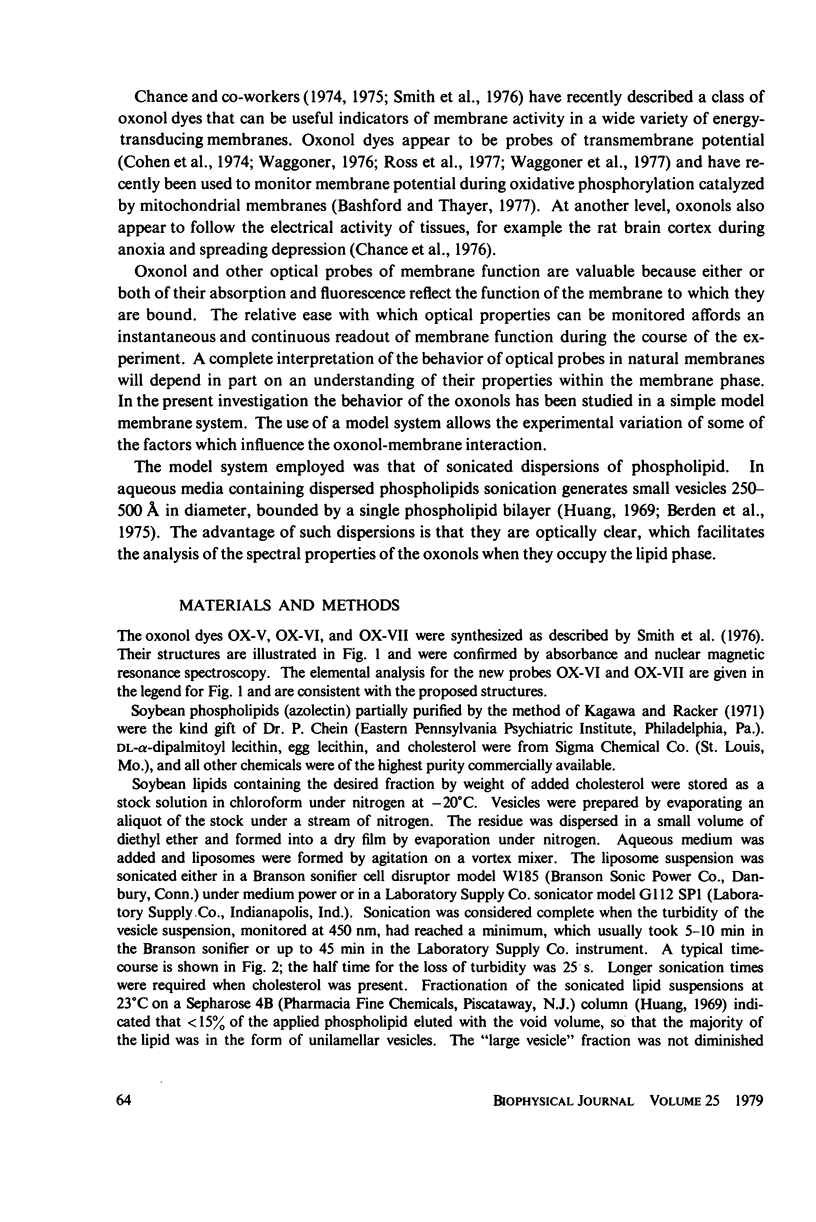
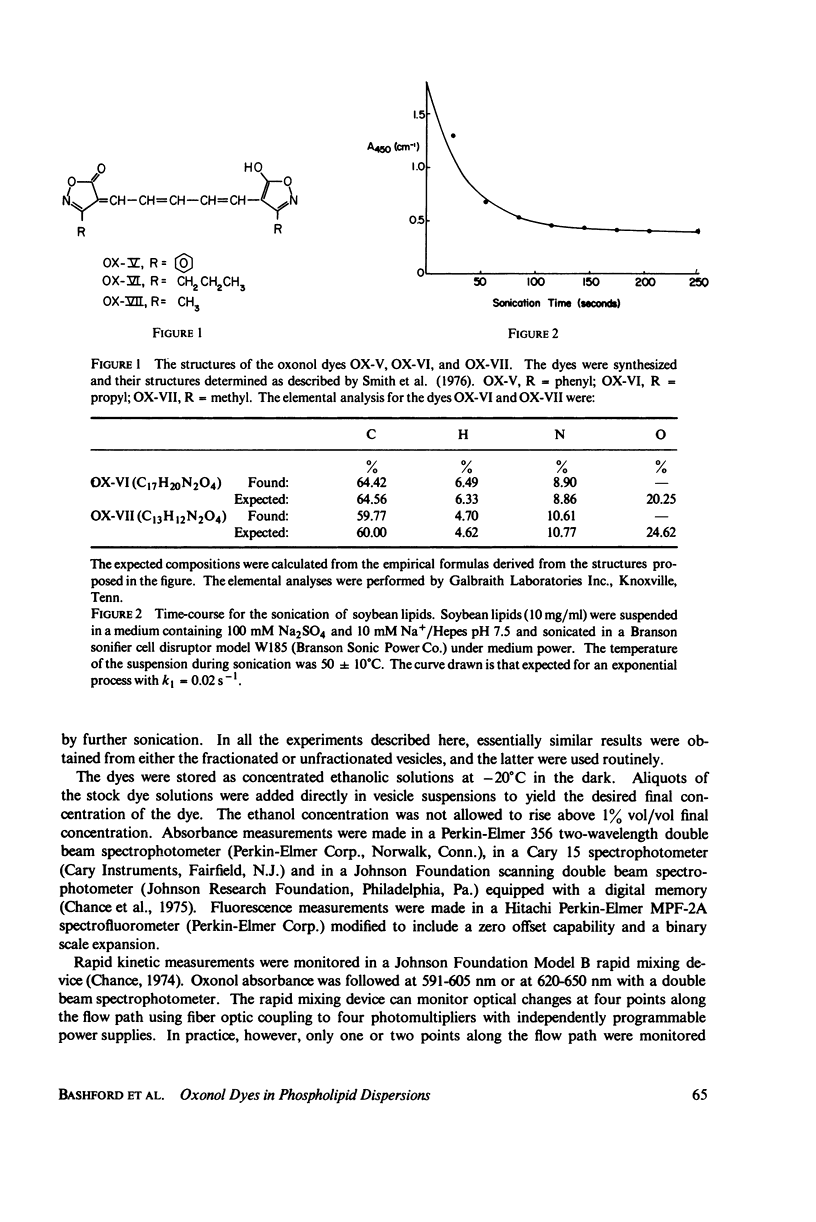
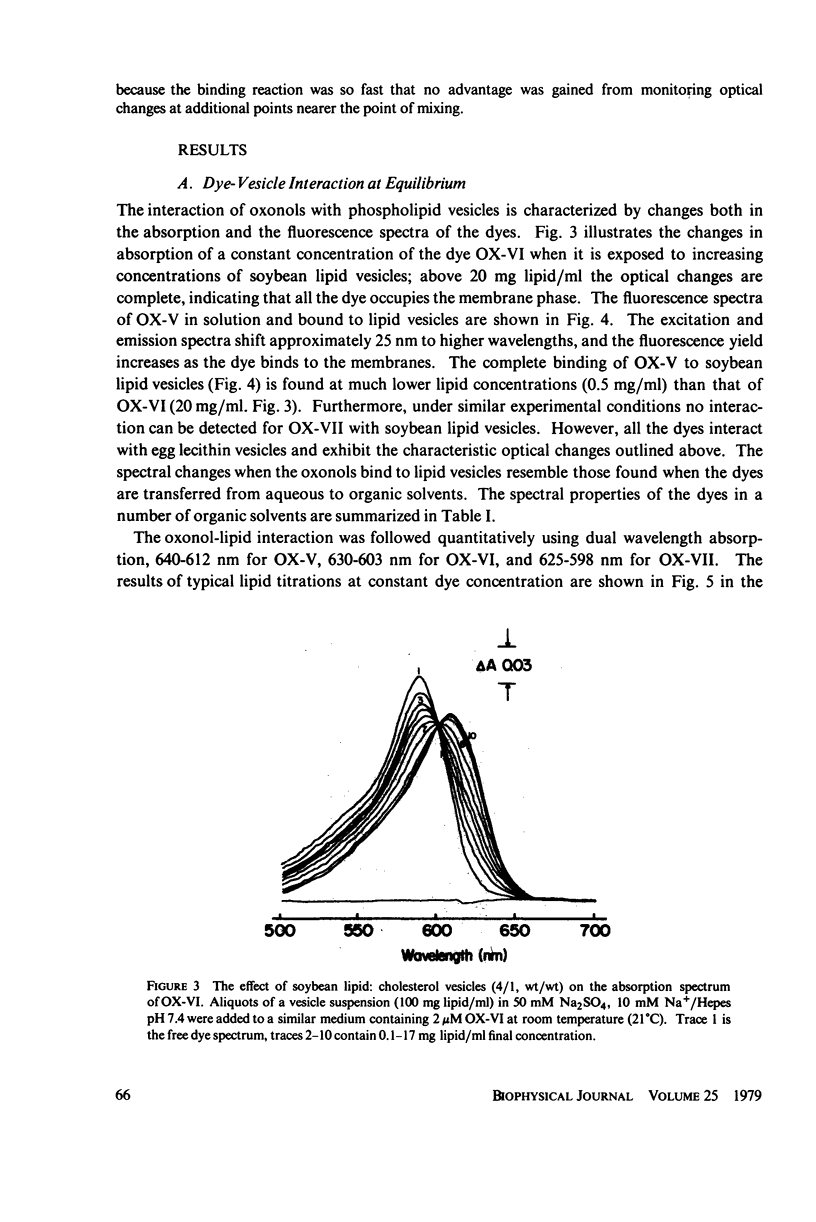
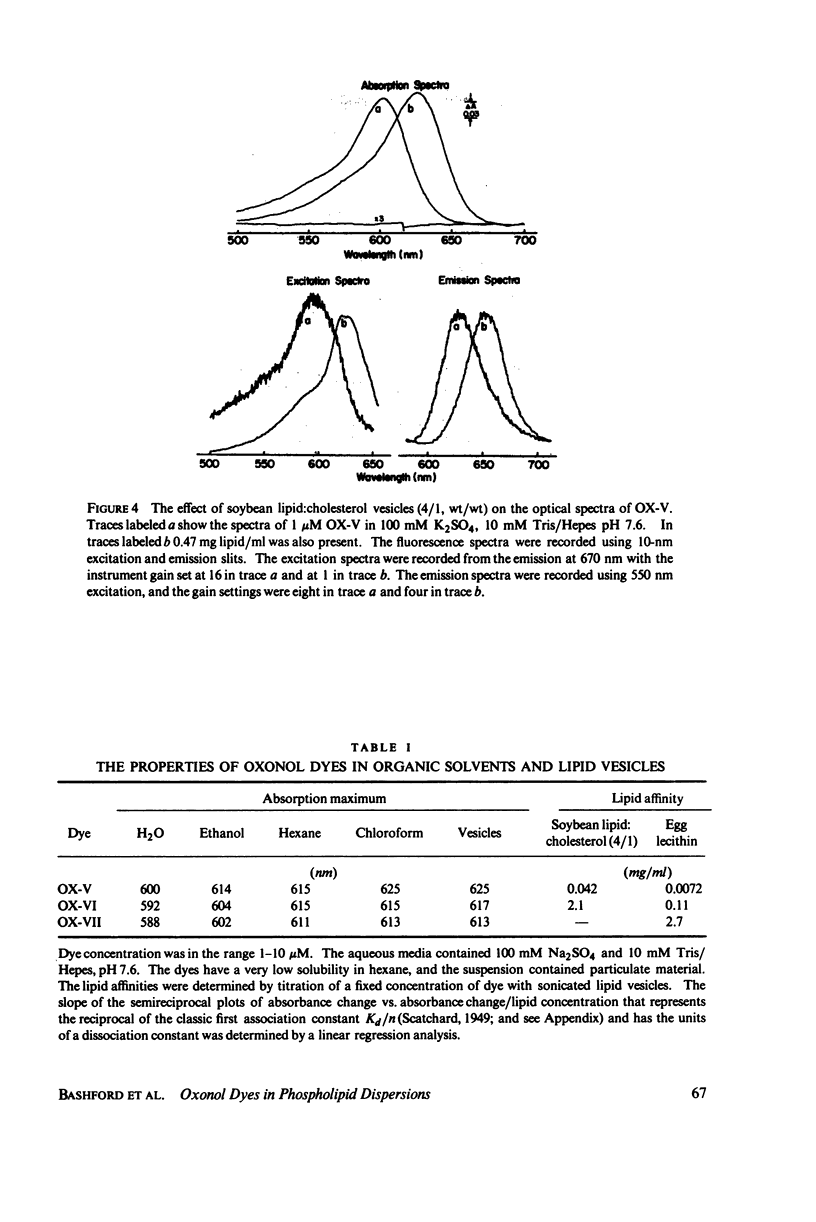
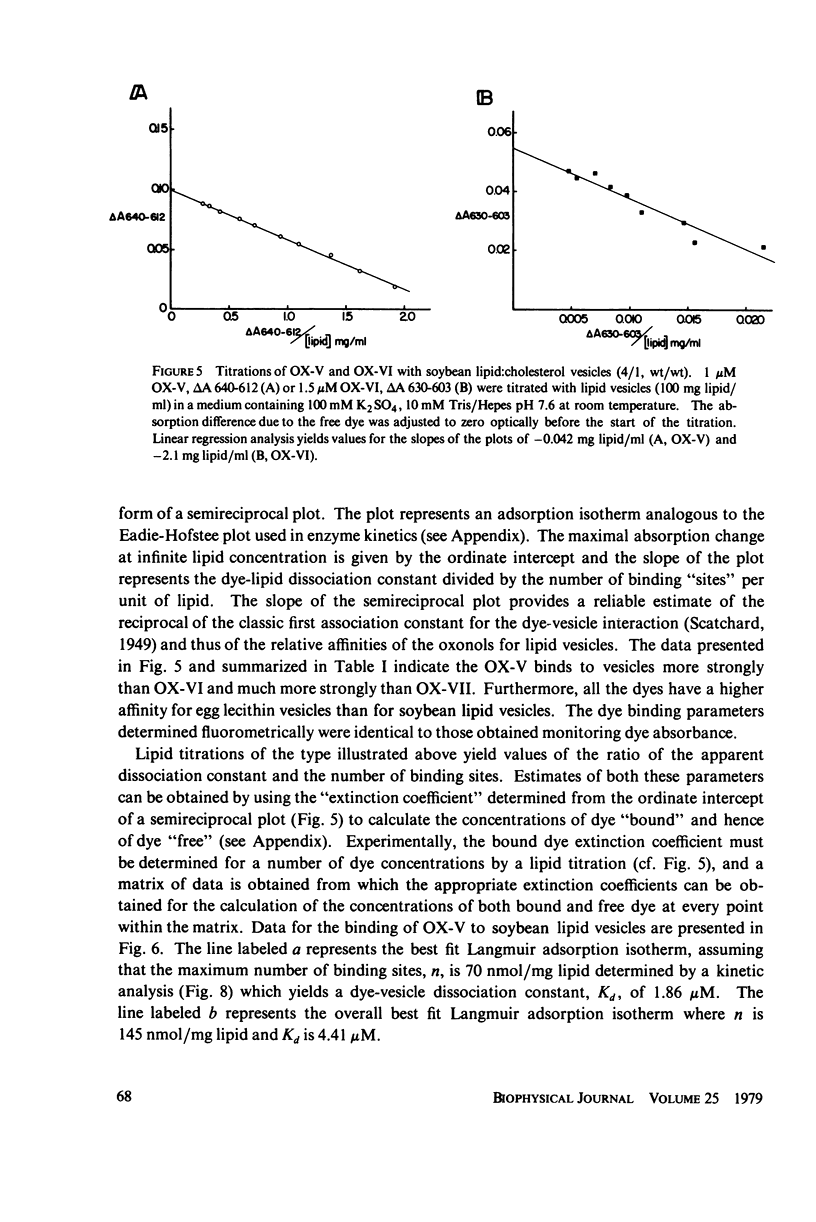
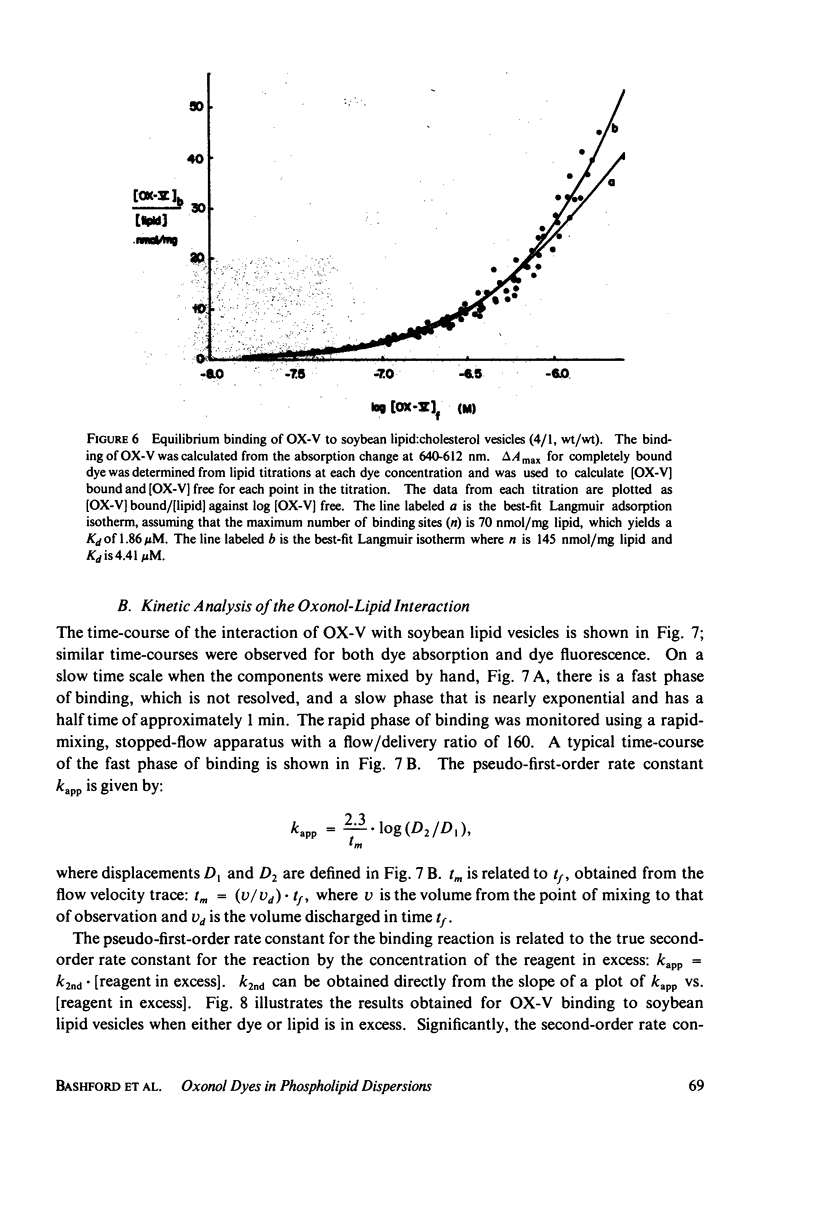
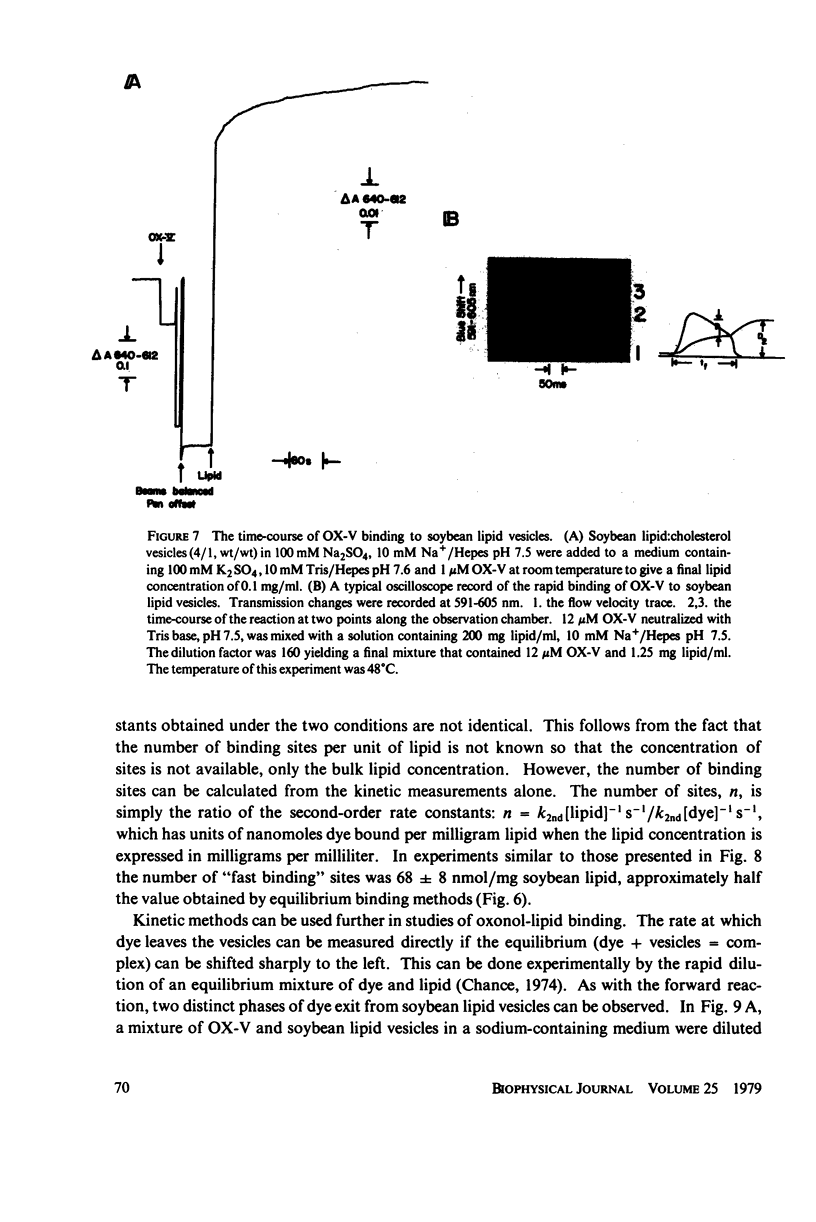
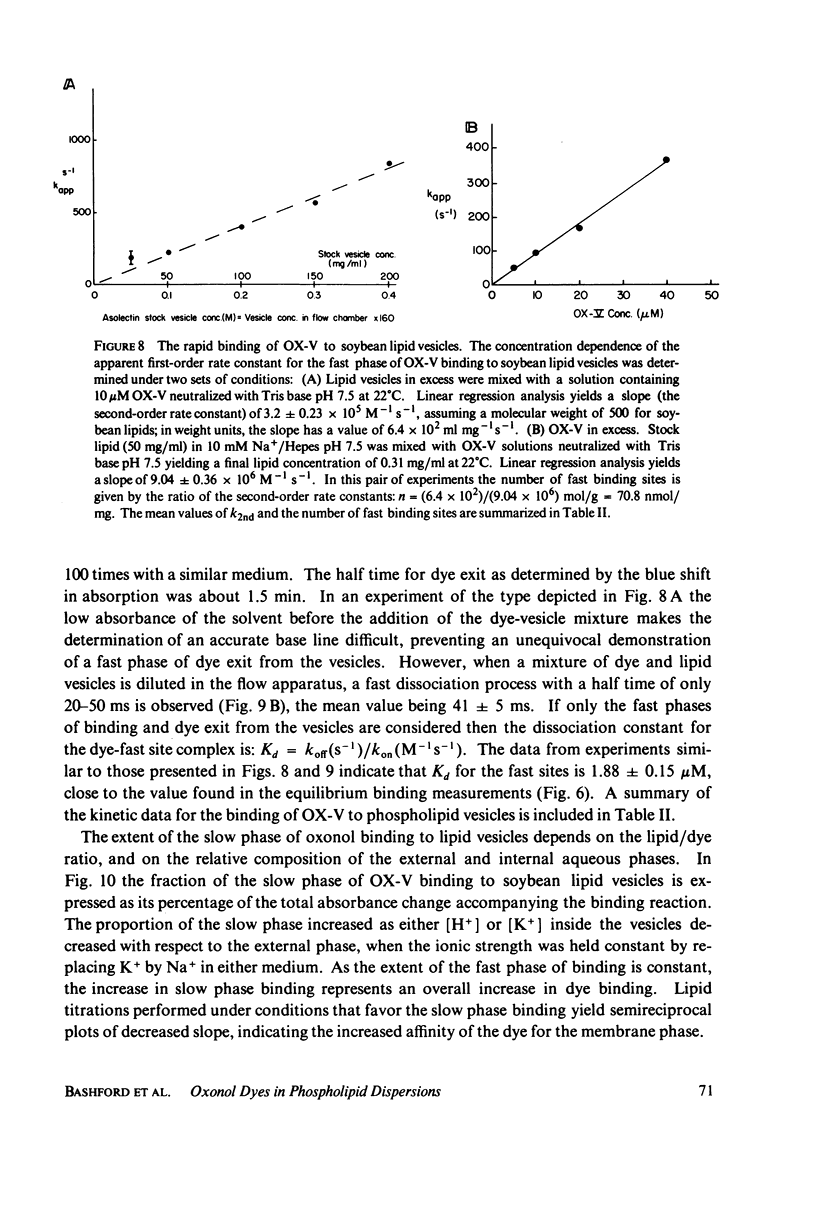
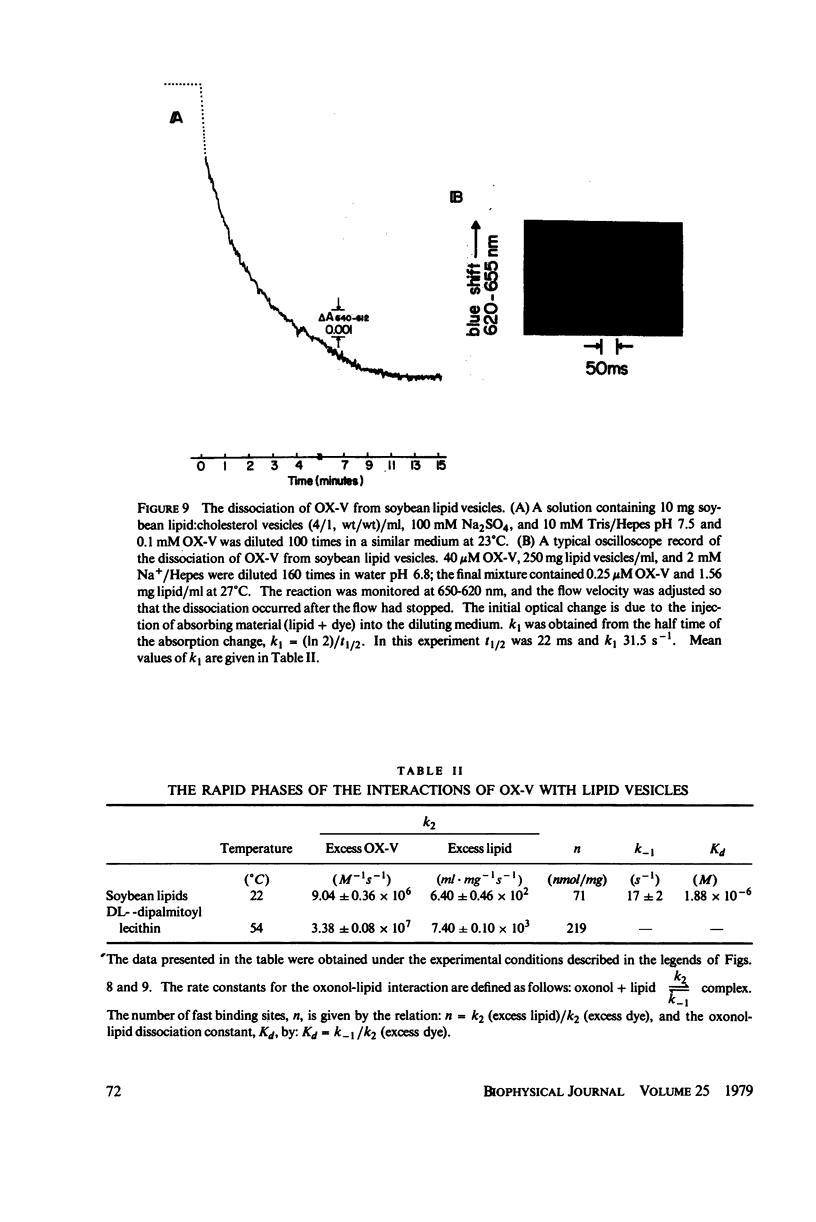
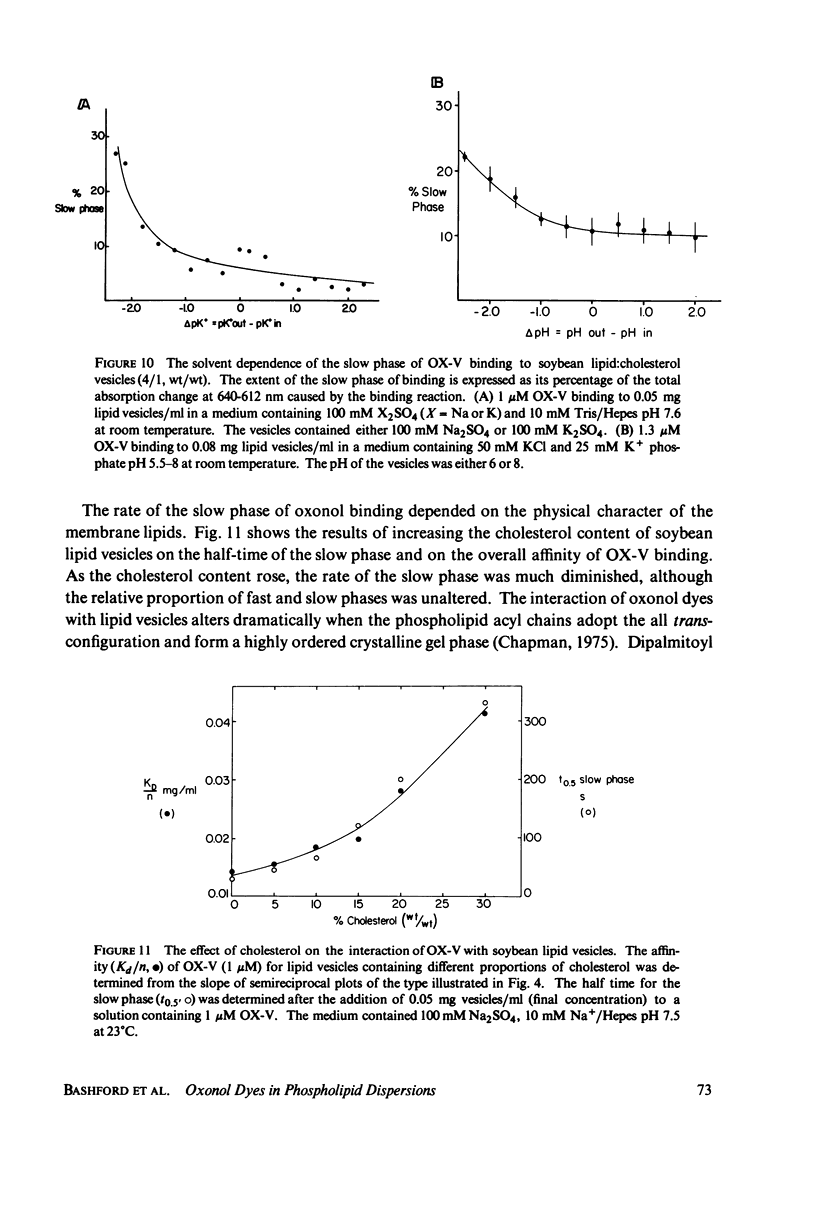
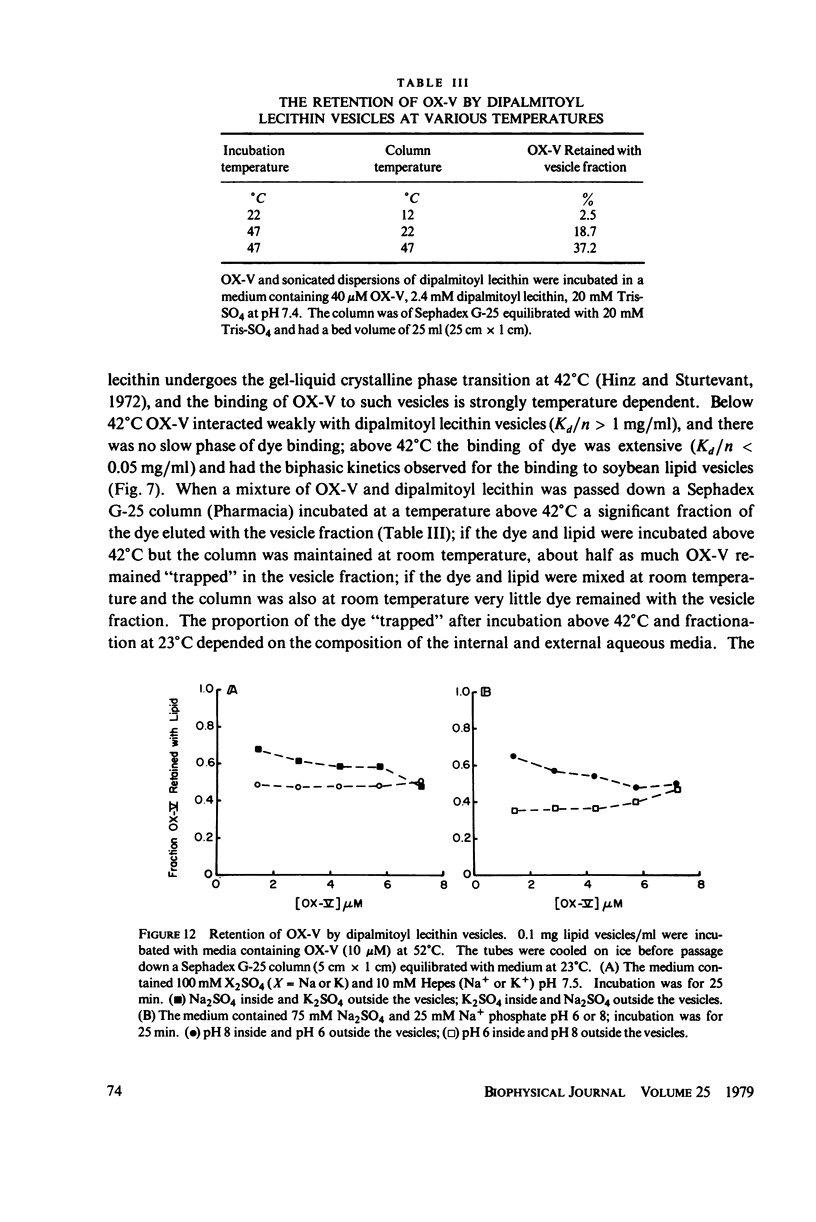
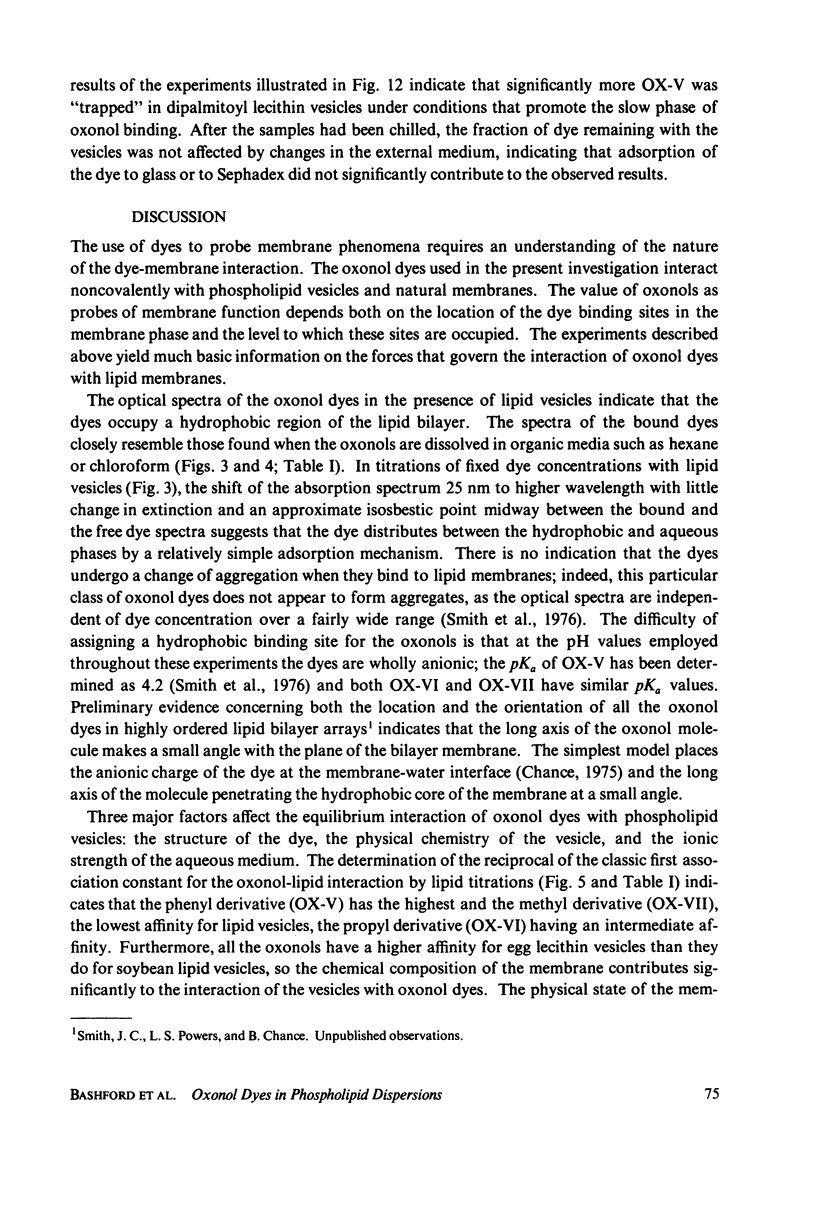
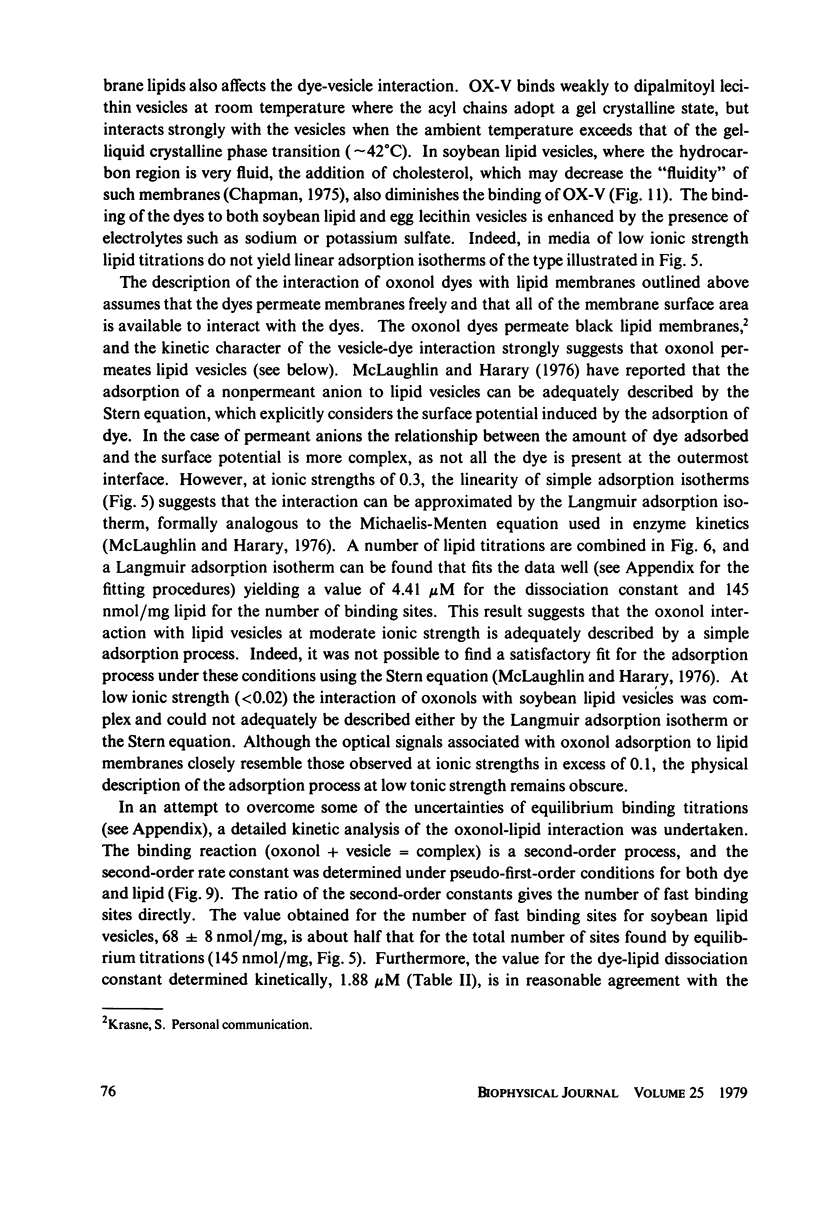
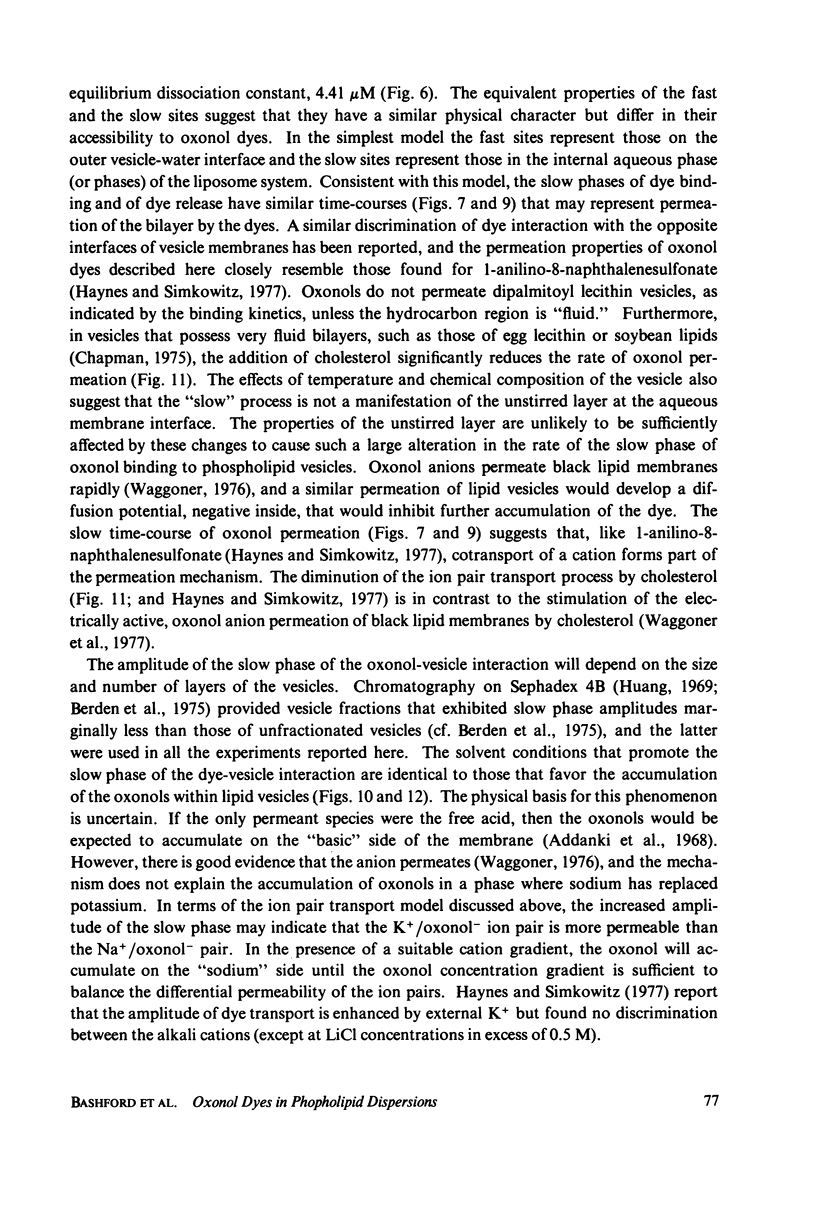
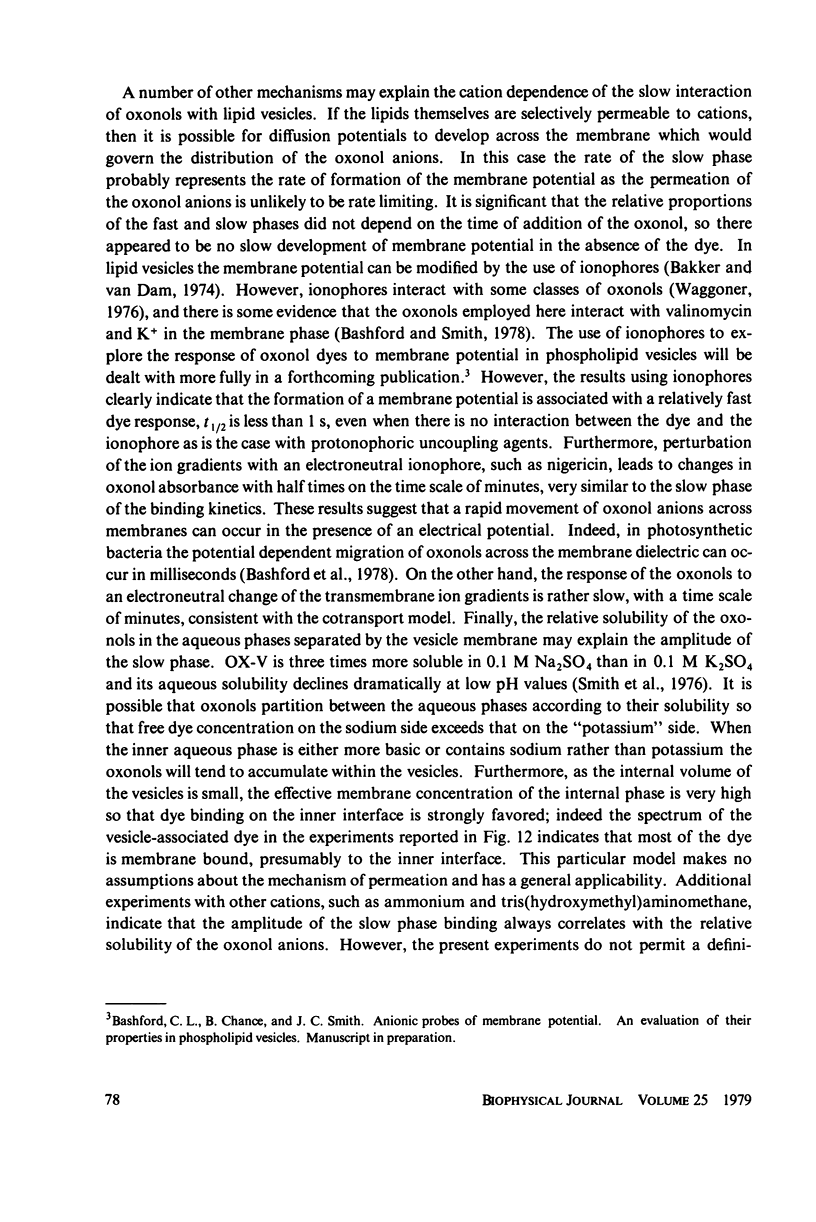
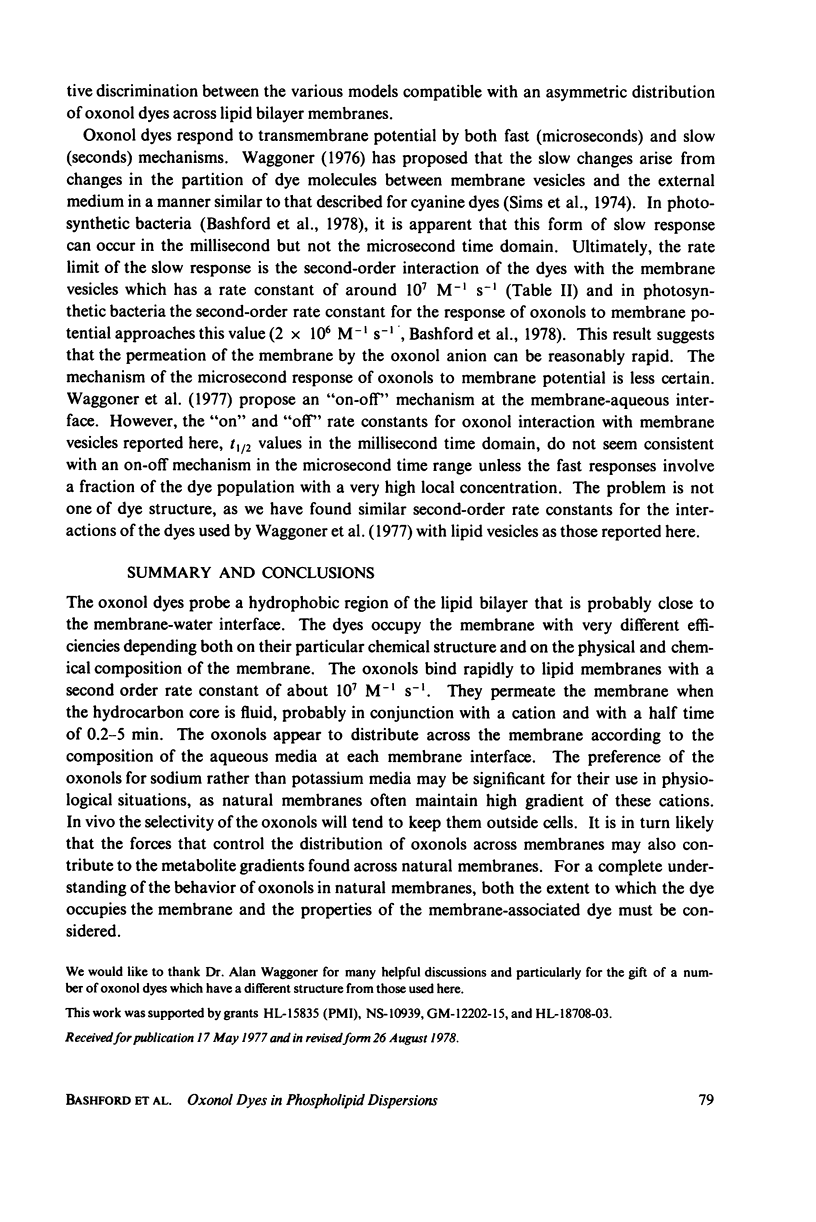
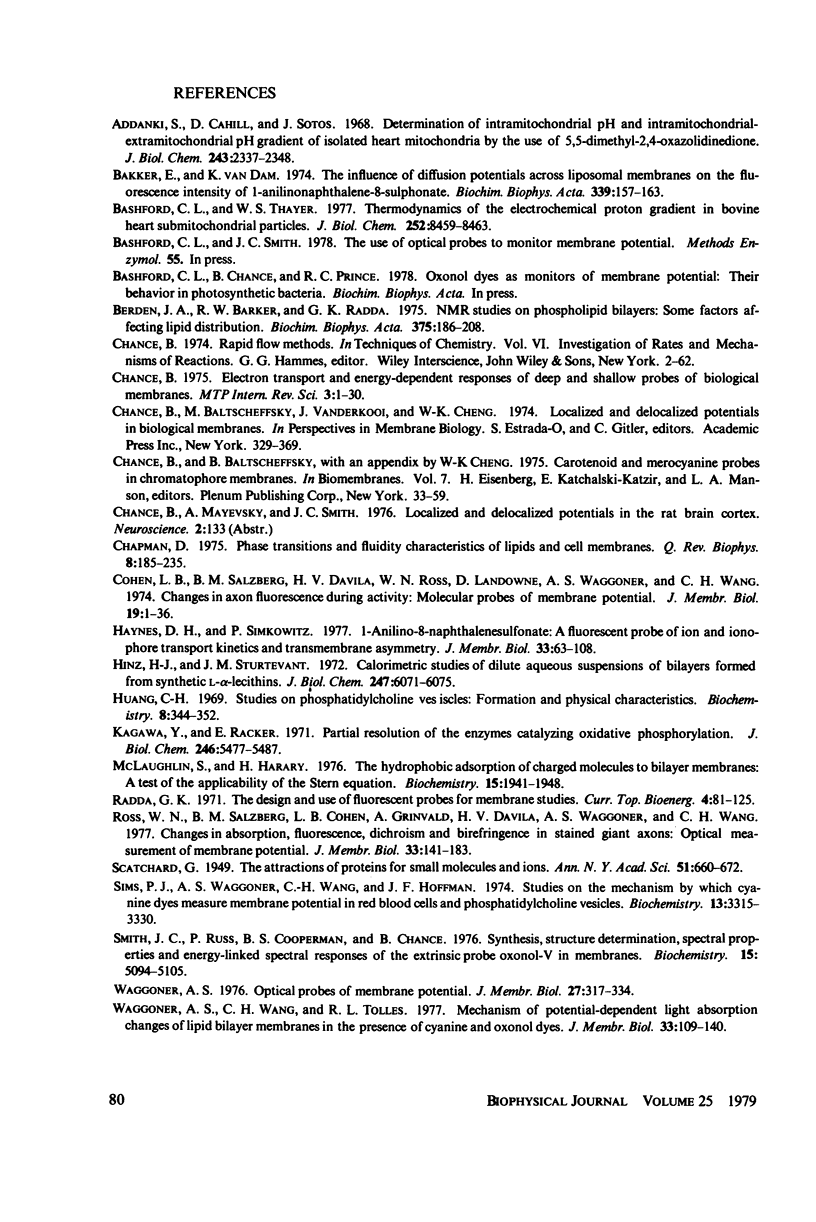
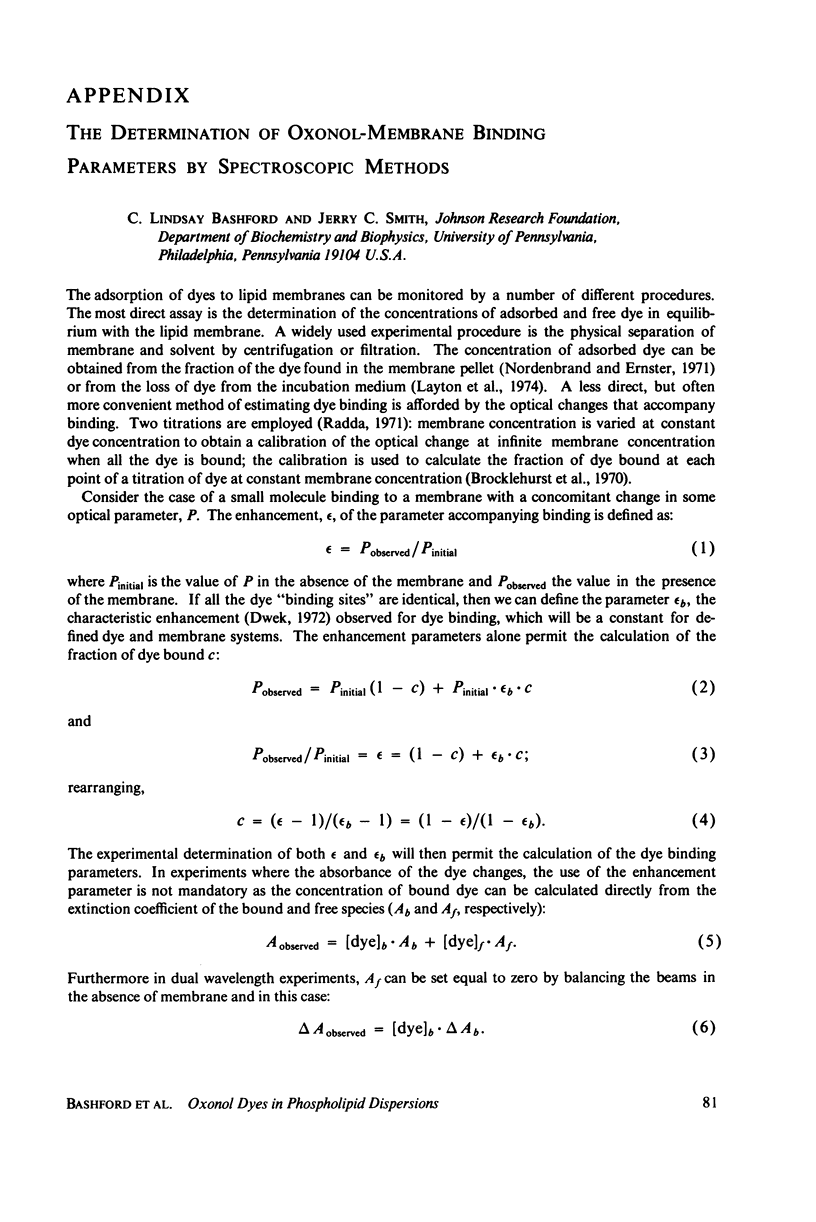
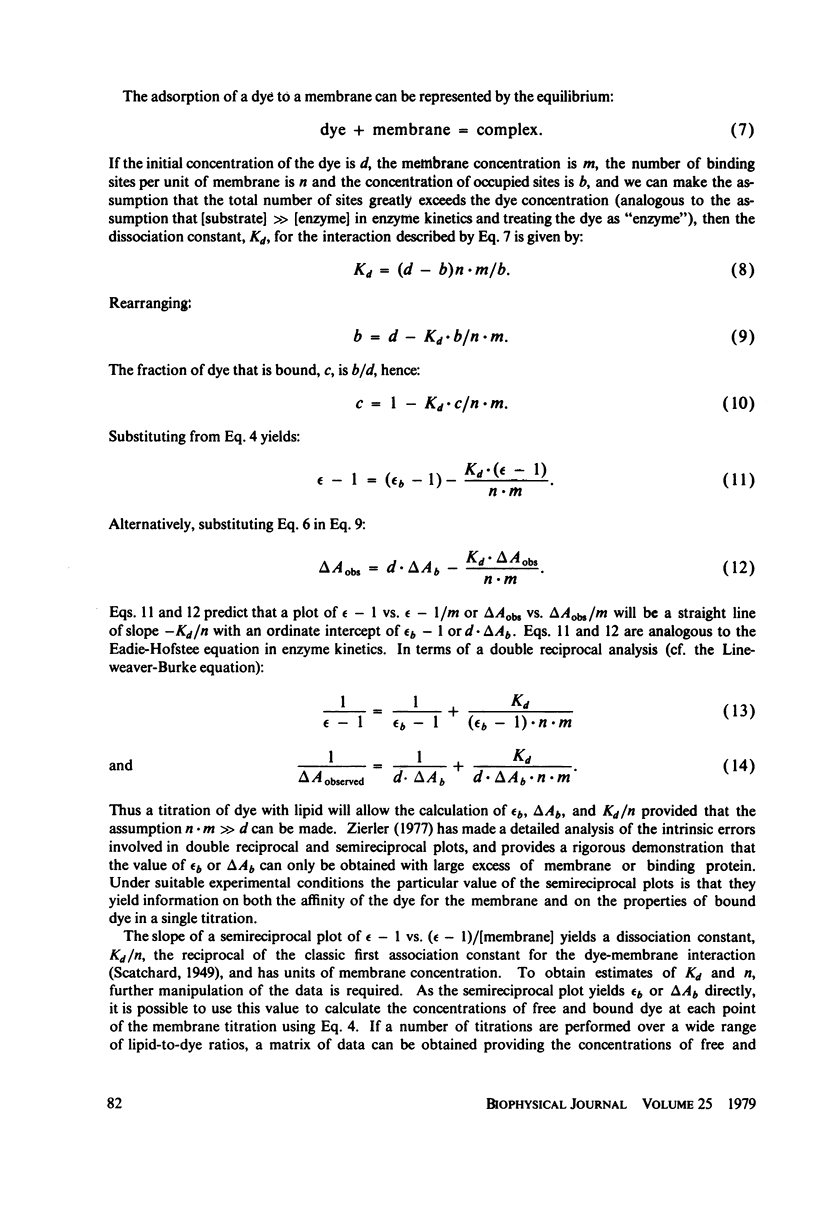
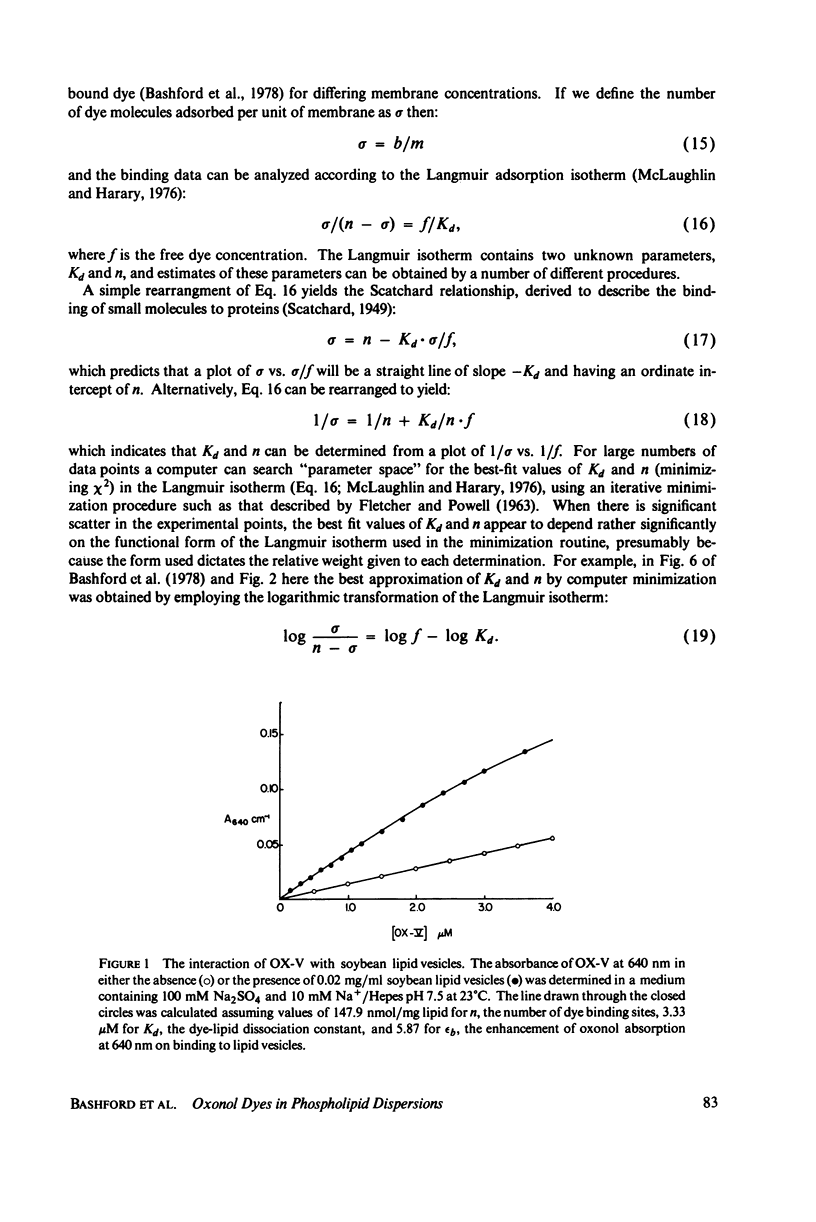
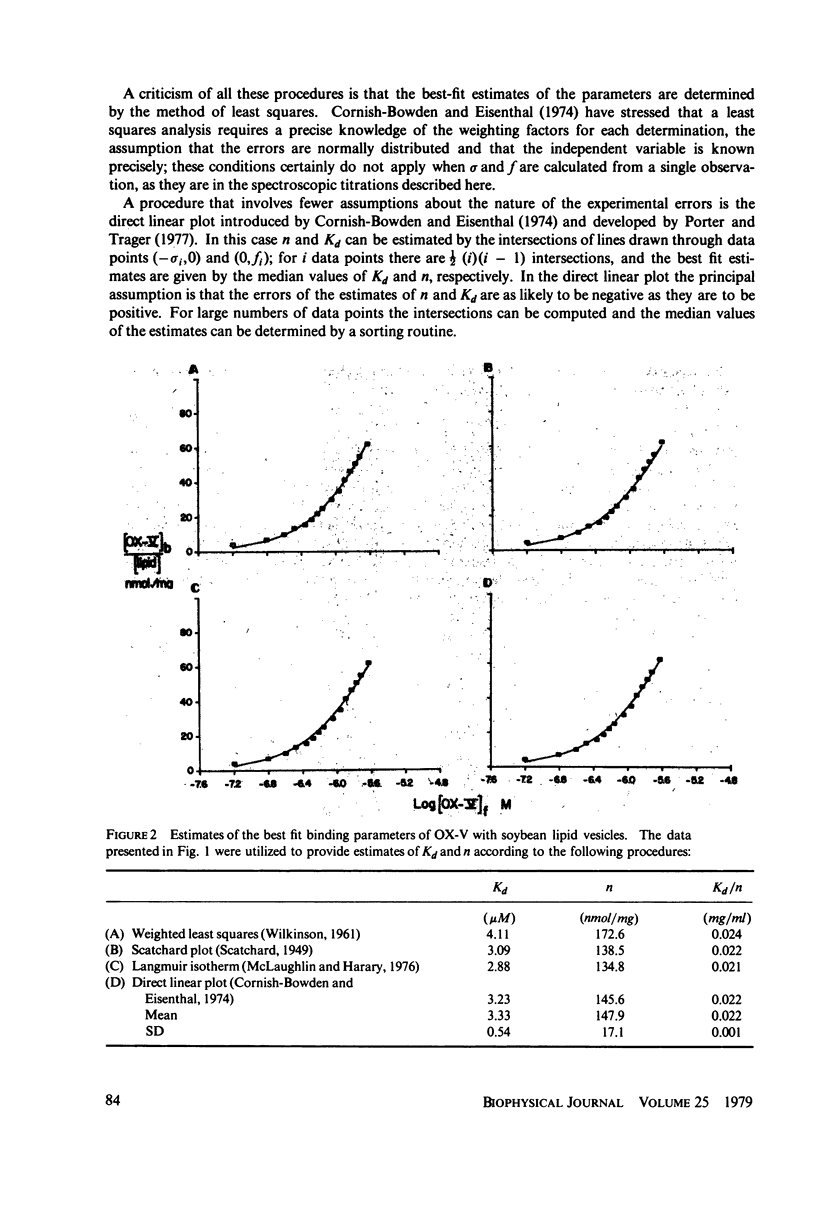
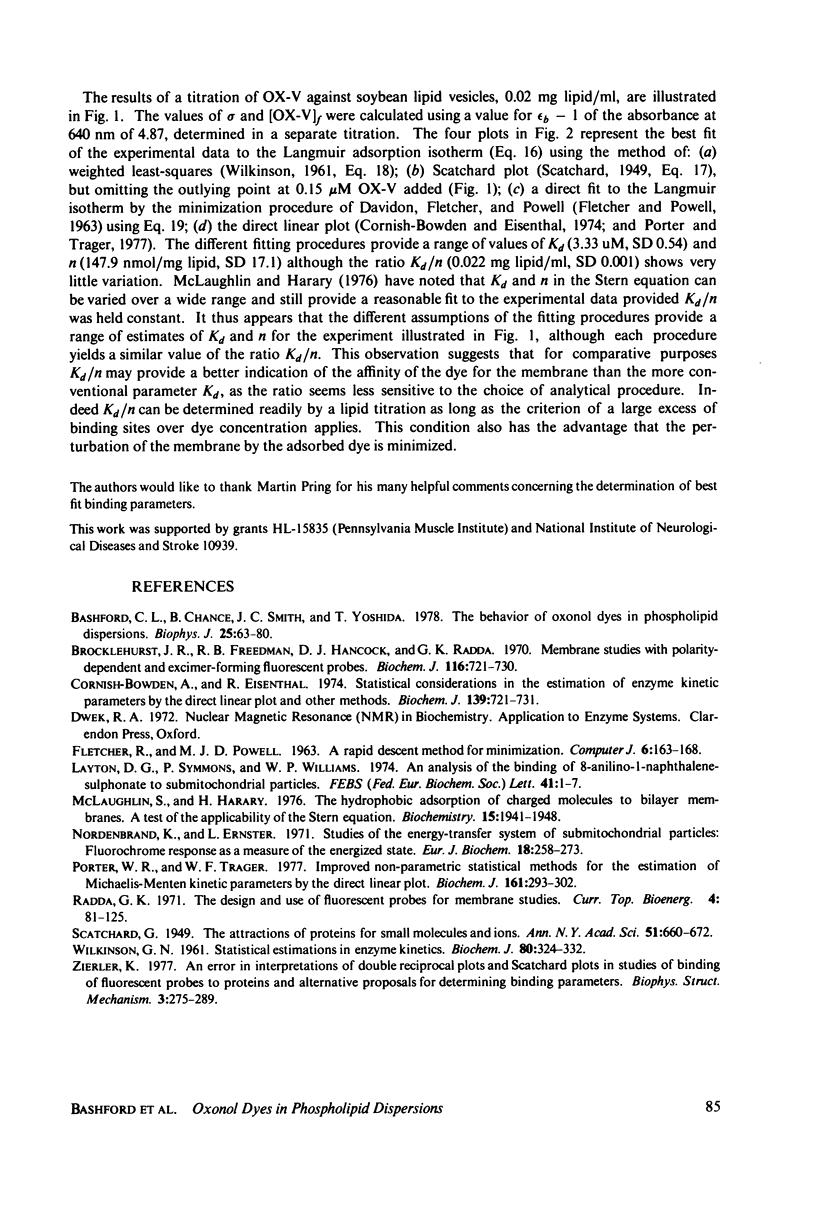
Selected References
These references are in PubMed. This may not be the complete list of references from this article.
- Addanki A., Cahill F. D., Sotos J. F. Determination of intramitochondrial pH and intramitochondrial-extramitochondrial pH gradient of isolated heart mitochondria by the use of 5,5-dimethyl-2,4-oxazolidinedione. I. Changes during respiration and adenosine triphosphate-dependent transport of Ca++, Mg++, and Zn++. J Biol Chem. 1968 May 10;243(9):2337–2348. [PubMed] [Google Scholar]
- Bakker E. P., van Dam K. The influence of diffusion potentials across liposomal membranes on the fluorescence intensity of 1-anilinonaphthalene-8-sulphonate. Biochim Biophys Acta. 1974 Mar 15;339(2):157–163. doi: 10.1016/0005-2736(74)90314-9. [DOI] [PubMed] [Google Scholar]
- Bashford C. L., Thayer W. S. Thermodynamics of the electrochemical proton gradient in bovine heart submitochondrial particles. J Biol Chem. 1977 Dec 10;252(23):8459–8463. [PubMed] [Google Scholar]
- Berden J. A., Barker R. W., Radda G. K. NMR studies on phospholipid bilayers. Some factors affecting lipid distribution. Biochim Biophys Acta. 1975 Jan 28;375(2):186–208. doi: 10.1016/0005-2736(75)90188-1. [DOI] [PubMed] [Google Scholar]
- Brocklehurst J. R., Freedman R. B., Hancock D. J., Radda G. K. Membrane studies with polarity-dependent and excimer-forming fluorescent probes. Biochem J. 1970 Feb;116(4):721–731. doi: 10.1042/bj1160721. [DOI] [PMC free article] [PubMed] [Google Scholar]
- Chapman D. Phase transitions and fluidity characteristics of lipids and cell membranes. Q Rev Biophys. 1975 May;8(2):185–235. doi: 10.1017/s0033583500001797. [DOI] [PubMed] [Google Scholar]
- Cohen L. B., Salzberg B. M., Davila H. V., Ross W. N., Landowne D., Waggoner A. S., Wang C. H. Changes in axon fluorescence during activity: molecular probes of membrane potential. J Membr Biol. 1974;19(1):1–36. doi: 10.1007/BF01869968. [DOI] [PubMed] [Google Scholar]
- Cornish-Bowden A., Eisenthal R. Statistical considerations in the estimation of enzyme kinetic parameters by the direct linear plot andother methods. Biochem J. 1974 Jun;139(3):721–730. doi: 10.1042/bj1390721. [DOI] [PMC free article] [PubMed] [Google Scholar]
- Haynes D. H., Simkowitz P. 1-Anilino-8-naphthalenesulfonate: a fluorescent probe of ion and ionophore transport kinetics and trans-membrane asymmetry. J Membr Biol. 1977 May 6;33(1-2):63–108. doi: 10.1007/BF01869512. [DOI] [PubMed] [Google Scholar]
- Hinz H. J., Sturtevant J. M. Calorimetric studies of dilute aqueous suspensions of bilayers formed from synthetic L- -lecithins. J Biol Chem. 1972 Oct 10;247(19):6071–6075. [PubMed] [Google Scholar]
- Huang C. Studies on phosphatidylcholine vesicles. Formation and physical characteristics. Biochemistry. 1969 Jan;8(1):344–352. doi: 10.1021/bi00829a048. [DOI] [PubMed] [Google Scholar]
- McLaughlin S., Harary H. The hydrophobic adsorption of charged molecules to bilayer membranes: a test of the applicability of the stern equation. Biochemistry. 1976 May 4;15(9):1941–1948. doi: 10.1021/bi00654a023. [DOI] [PubMed] [Google Scholar]
- McLaughlin S., Harary H. The hydrophobic adsorption of charged molecules to bilayer membranes: a test of the applicability of the stern equation. Biochemistry. 1976 May 4;15(9):1941–1948. doi: 10.1021/bi00654a023. [DOI] [PubMed] [Google Scholar]
- Nordenbrand K., Ernster L. Studies of the energy-transfer system of submitochondrial particles. Fluorochrome response as a measure of the energized state. Eur J Biochem. 1971 Jan;18(2):258–273. doi: 10.1111/j.1432-1033.1971.tb01239.x. [DOI] [PubMed] [Google Scholar]
- Porter W. R., Trager W. F. Improved non-parametric statistical methods for the estimation of Michaelis-Menten kinetic parameters by the direct linear plot. Biochem J. 1977 Feb 1;161(2):293–302. doi: 10.1042/bj1610293. [DOI] [PMC free article] [PubMed] [Google Scholar]
- Ross W. N., Salzberg B. M., Cohen L. B., Grinvald A., Davila H. V., Waggoner A. S., Wang C. H. Changes in absorption, fluorescence, dichroism, and Birefringence in stained giant axons: : optical measurement of membrane potential. J Membr Biol. 1977 May 6;33(1-2):141–183. doi: 10.1007/BF01869514. [DOI] [PubMed] [Google Scholar]
- Sims P. J., Waggoner A. S., Wang C. H., Hoffman J. F. Studies on the mechanism by which cyanine dyes measure membrane potential in red blood cells and phosphatidylcholine vesicles. Biochemistry. 1974 Jul 30;13(16):3315–3330. doi: 10.1021/bi00713a022. [DOI] [PubMed] [Google Scholar]
- Smith J. C., Russ P., Cooperman B. S., Chance B. Synthesis, structure determination, spectral properties, and energy-linked spectral responses of the extrinsic probe oxonol V in membranes. Biochemistry. 1976 Nov 16;15(23):5094–5105. doi: 10.1021/bi00668a023. [DOI] [PubMed] [Google Scholar]
- WILKINSON G. N. Statistical estimations in enzyme kinetics. Biochem J. 1961 Aug;80:324–332. doi: 10.1042/bj0800324. [DOI] [PMC free article] [PubMed] [Google Scholar]
- Waggoner A. S., Wang C. H., Tolles R. L. Mechanism of potential-dependent light absorption changes of lipid bilayer membranes in the presence of cyanine and oxonol dyes. J Membr Biol. 1977 May 6;33(1-2):109–140. doi: 10.1007/BF01869513. [DOI] [PubMed] [Google Scholar]
- Waggoner A. Optical probes of membrane potential. J Membr Biol. 1976 Jun 30;27(4):317–334. doi: 10.1007/BF01869143. [DOI] [PubMed] [Google Scholar]
- Zierler K. An error in interpretation of double-reciprocal plots and Scatchard plots in the studies of binding of fluorescent probes to proteins, and alternative proposals for determining binding parameters. Biophys Struct Mech. 1977 Sep 28;3(3-4):275–289. doi: 10.1007/BF00535701. [DOI] [PubMed] [Google Scholar]