Abstract
If the subfragment-2 (S2) portion of the myosin cross-bridge to actin does not lie parallel to the myofilament axes then when a muscle fiber contracts, there will be a radial component to the cross-bridge force. When the subfragment-1 (S1) portion of the cross-bridge attaches to actin with its long axis projecting through the filament axis, the magnitude of the radial force depends upon the azimuthal location of the actin site, but when the attachment of the S1 to actin is slewed, as in the reconstruction of Moore et al. (J. Mol. Biol., 1970, 50:279-294), then for a single cross-bridge the radial component of the cross-bridge force is not quite so sensitive to actin site location and is approximately 0.1 the axial component. In both cases, the ratio of the radial to axial force decreases with decreasing filament separation. If the radial-axial force ratio for each cross-bridge is approximately 0.1, then at full overlap in a frog skeletal muscle fiber the radial component of the cross-bridge force accompanying full activation will exert a compressive pressure of approximately 5 X 10(-3) atm. This would have little effect upon an intact muscle fiber where the volume constraints are likely osmotic, but it might produce a 1-2% change in filament spacing in a "skinned" muscle fiber from which the sarcolemma had been removed. These computations assume that the S2 link between the S1 head and the myosin filament does not support a bending moment of shear. If it does, then the radial component of the cross-bridge will be either greater or less, depending on the specific cross-bridge geometry.
Full text
PDF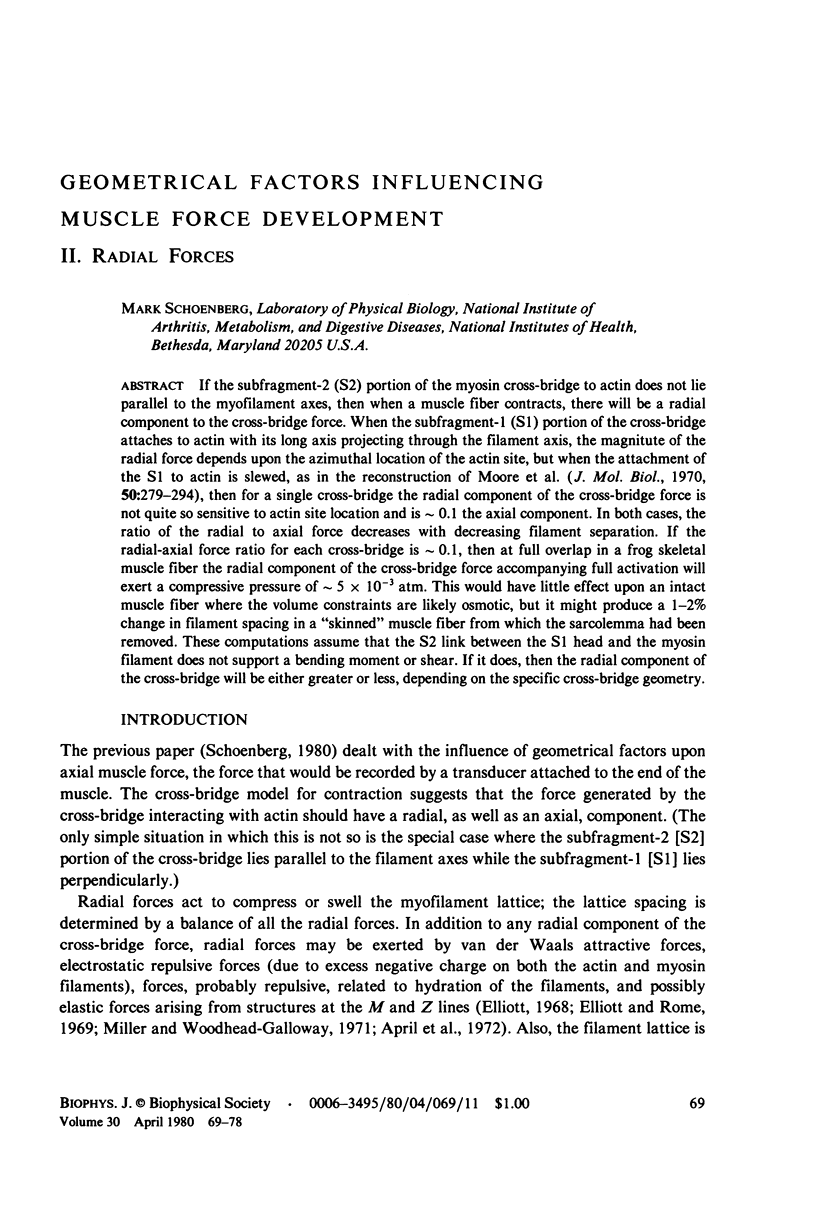
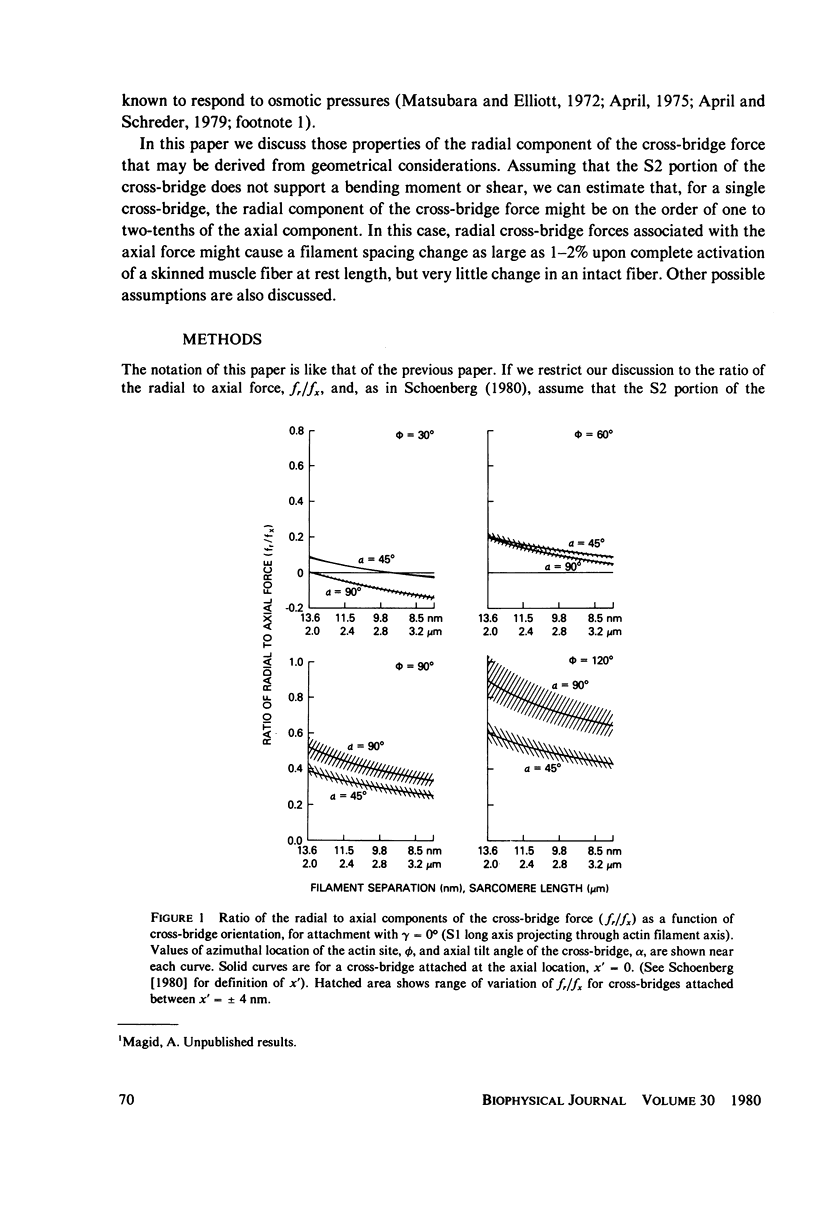
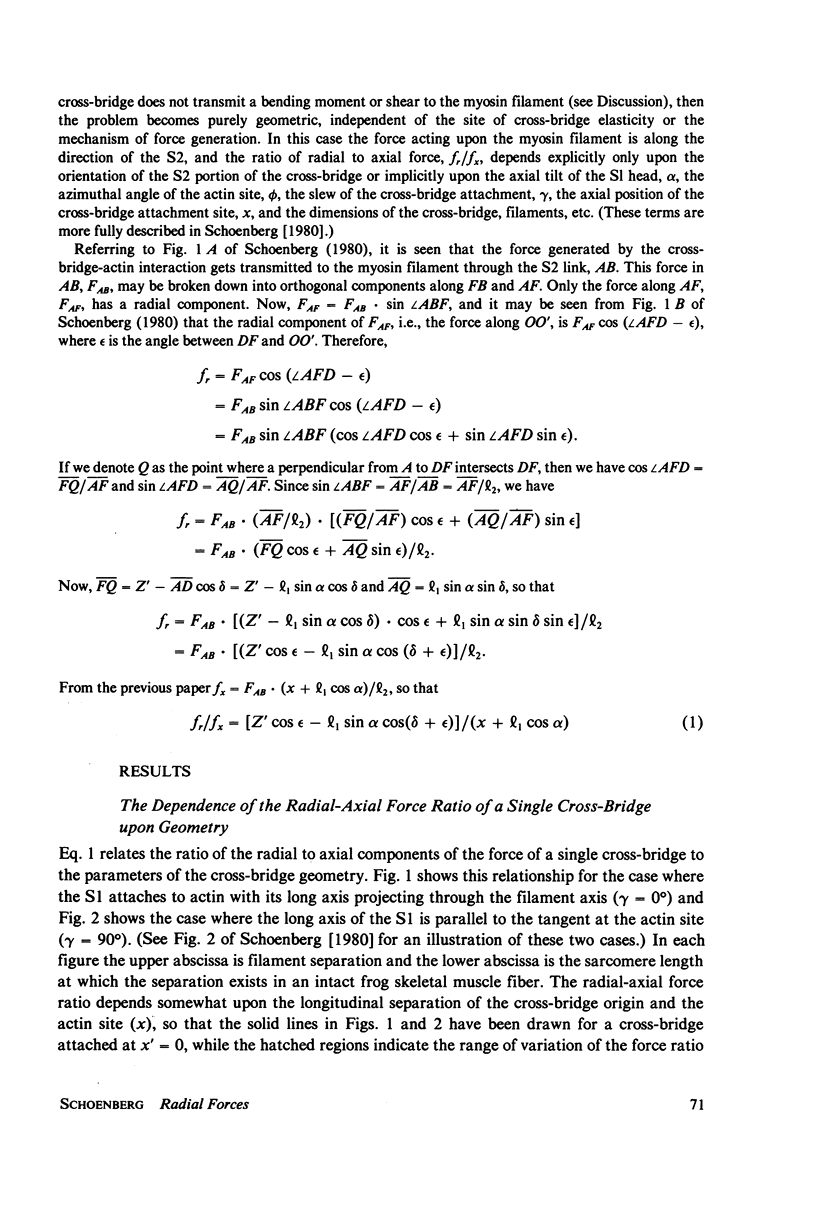
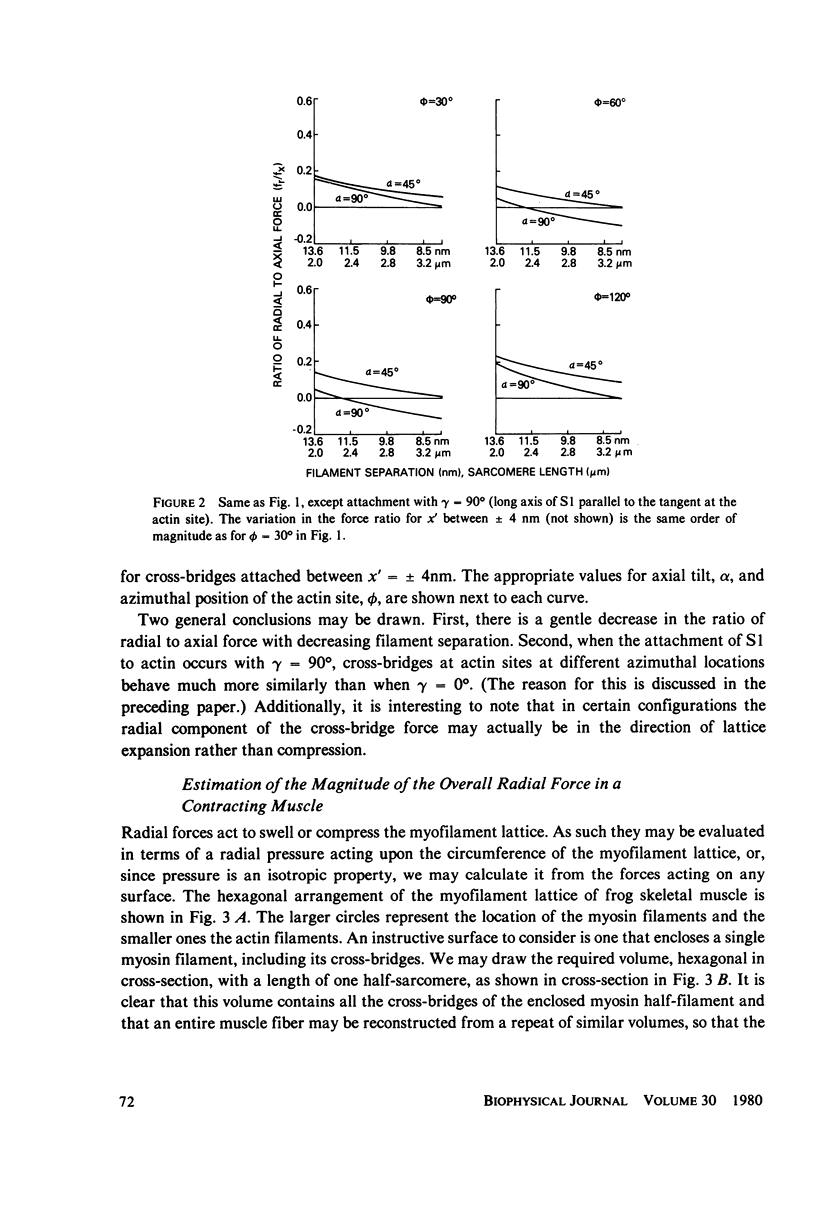
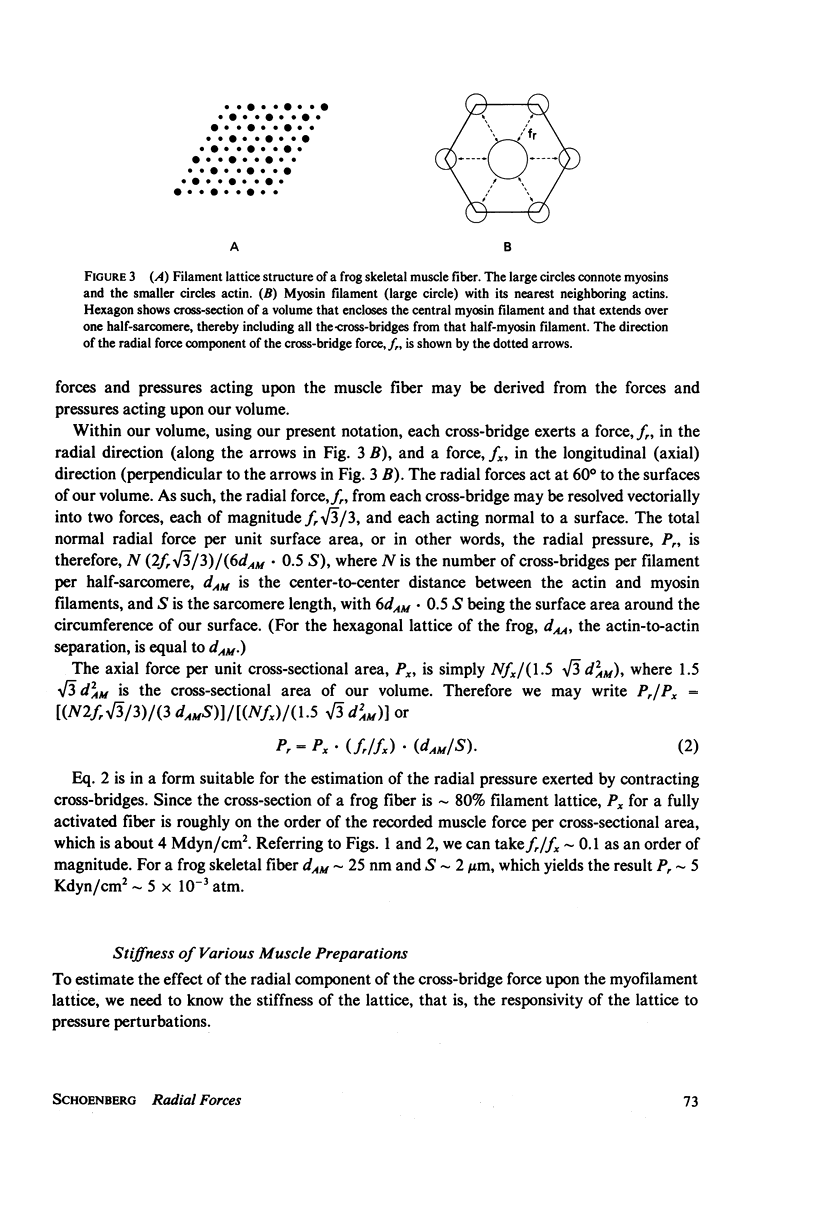
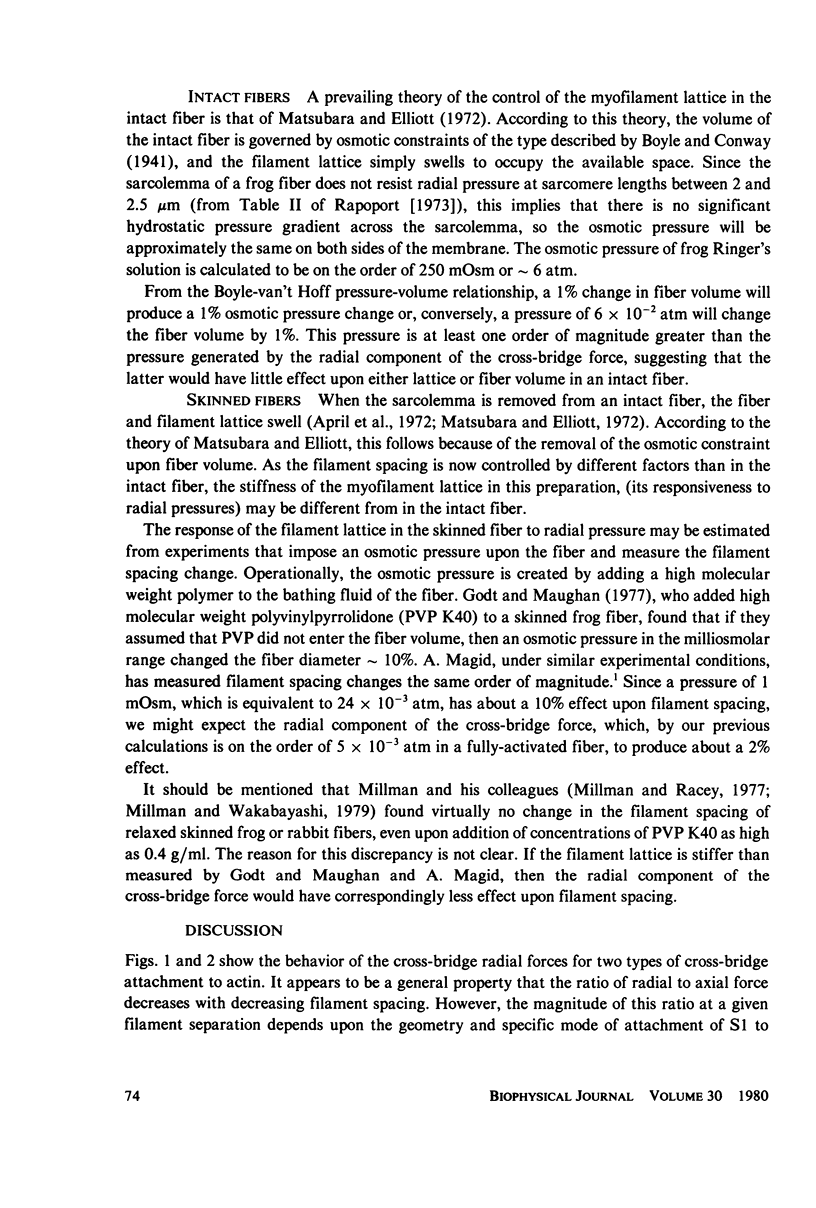
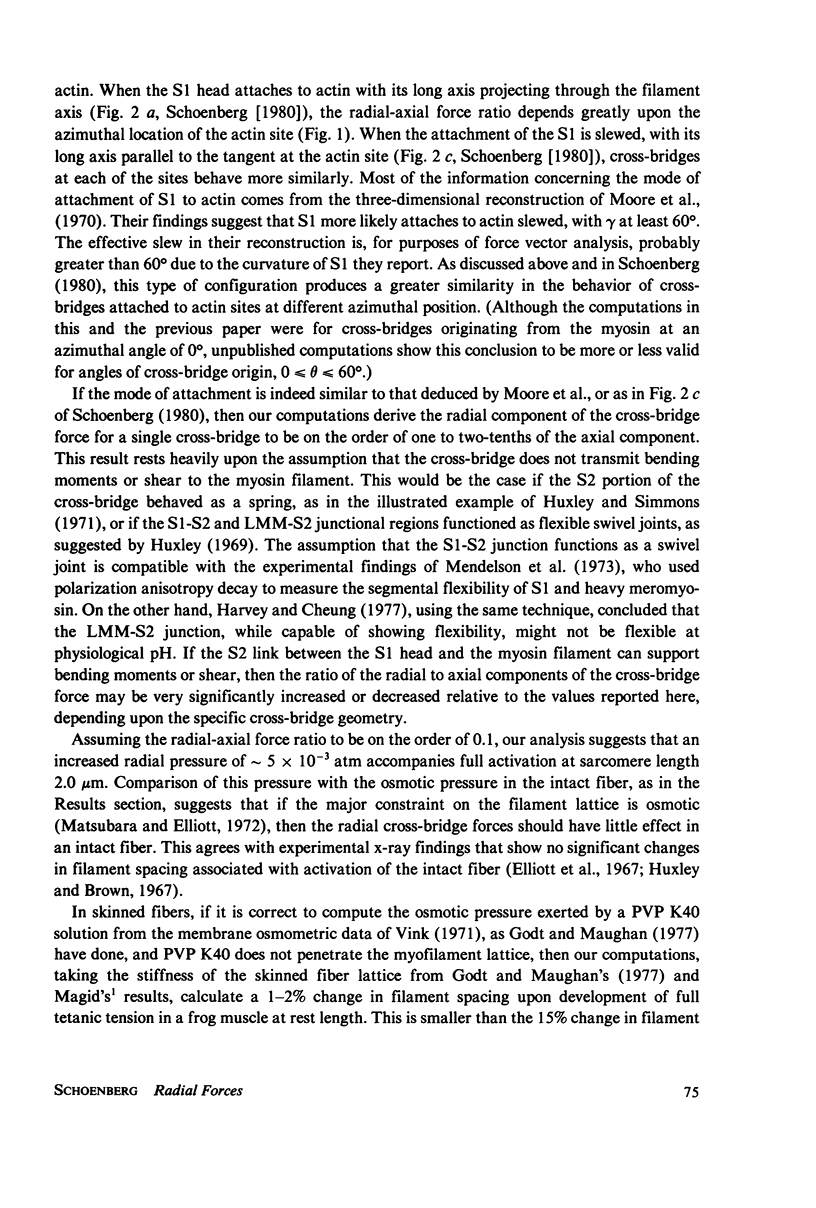
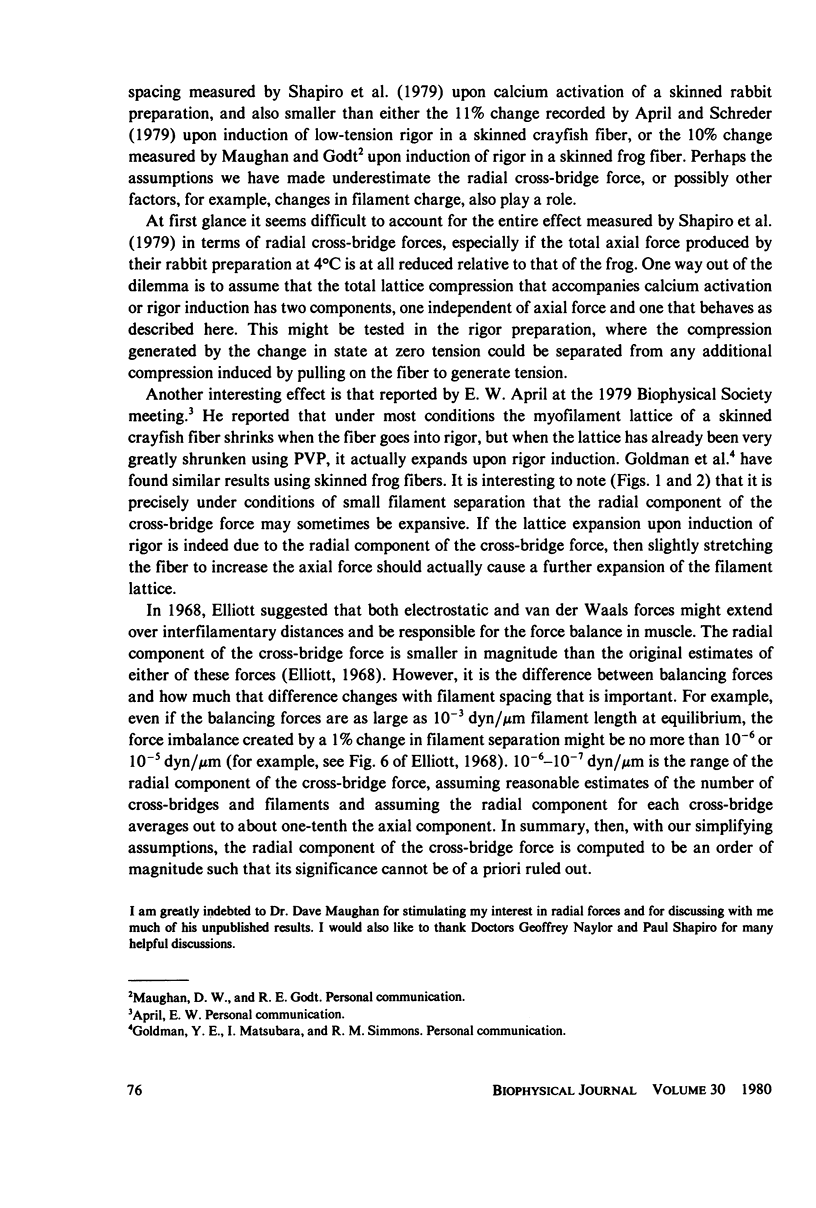
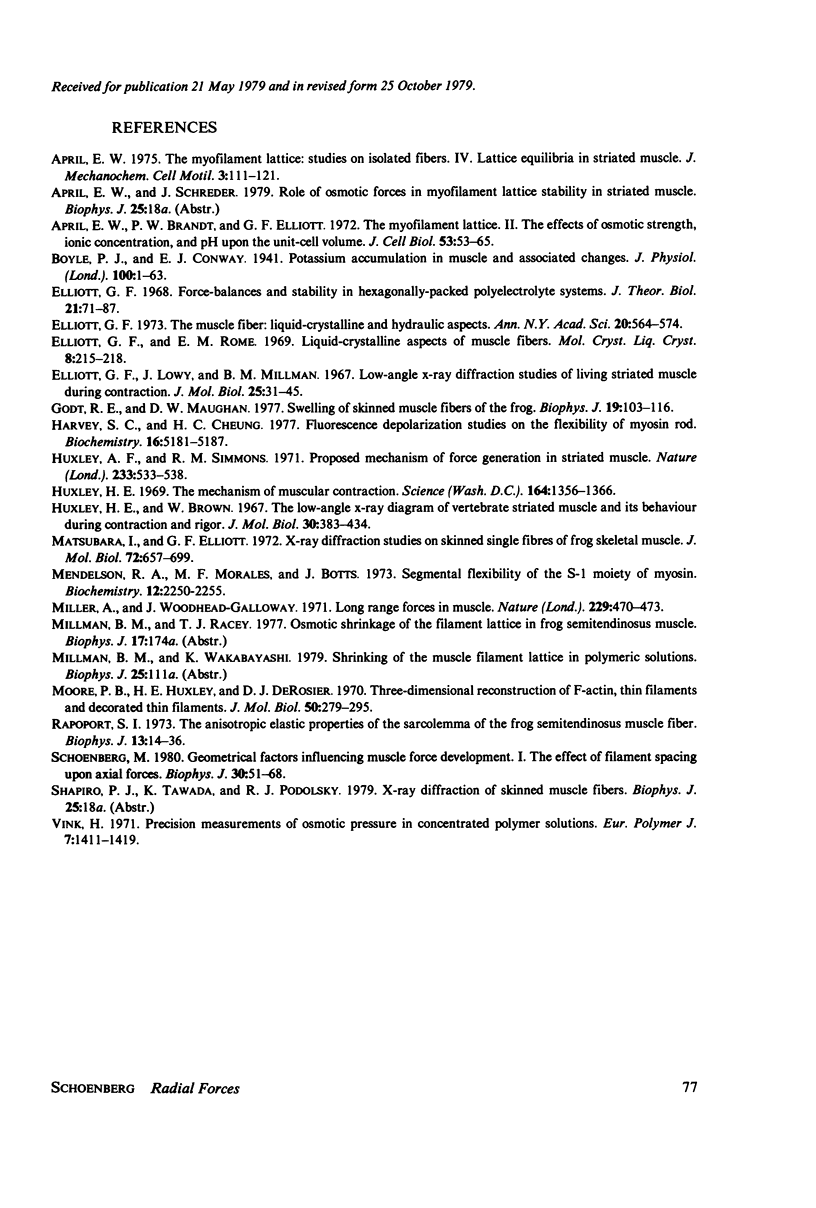
Selected References
These references are in PubMed. This may not be the complete list of references from this article.
- April E. W., Brandt P. W., Elliott G. F. The myofilament lattice: studies on isolated fibers. II. The effects of osmotic strength, ionic concentration, and pH upon the unit-cell volume. J Cell Biol. 1972 Apr;53(1):53–65. doi: 10.1083/jcb.53.1.53. [DOI] [PMC free article] [PubMed] [Google Scholar]
- April E. W. The myofilament lattice: studies on isolated fibers. IV. Lattice equilibria in striated muscle. J Mechanochem Cell Motil. 1975;3(2):111–121. [PubMed] [Google Scholar]
- Boyle P. J., Conway E. J. Potassium accumulation in muscle and associated changes. J Physiol. 1941 Aug 11;100(1):1–63. doi: 10.1113/jphysiol.1941.sp003922. [DOI] [PMC free article] [PubMed] [Google Scholar]
- Elliott G. F. Force-balances and stability in hexagonally-packed polyelectrolyte systems. J Theor Biol. 1968 Oct;21(1):71–87. doi: 10.1016/0022-5193(68)90060-x. [DOI] [PubMed] [Google Scholar]
- Elliott G. F., Lowy J., Millman B. M. Low-angle x-ray diffraction studies of living striated muscle during contraction. J Mol Biol. 1967 Apr 14;25(1):31–45. doi: 10.1016/0022-2836(67)90277-x. [DOI] [PubMed] [Google Scholar]
- Elliott G. F. The muscle fiber: liquid-crystalline and hydraulic aspects. Ann N Y Acad Sci. 1973 Mar 30;204:564–574. doi: 10.1111/j.1749-6632.1973.tb30804.x. [DOI] [PubMed] [Google Scholar]
- Godt R. E., Maughan D. W. Swelling of skinned muscle fibers of the frog. Experimental observations. Biophys J. 1977 Aug;19(2):103–116. doi: 10.1016/S0006-3495(77)85573-2. [DOI] [PMC free article] [PubMed] [Google Scholar]
- Harvey S. C., Cheung H. C. Fluorescence depolarization studies on the flexibility of myosin rod. Biochemistry. 1977 Nov 29;16(24):5181–5187. doi: 10.1021/bi00643a004. [DOI] [PubMed] [Google Scholar]
- Huxley A. F., Simmons R. M. Proposed mechanism of force generation in striated muscle. Nature. 1971 Oct 22;233(5321):533–538. doi: 10.1038/233533a0. [DOI] [PubMed] [Google Scholar]
- Huxley H. E., Brown W. The low-angle x-ray diagram of vertebrate striated muscle and its behaviour during contraction and rigor. J Mol Biol. 1967 Dec 14;30(2):383–434. doi: 10.1016/s0022-2836(67)80046-9. [DOI] [PubMed] [Google Scholar]
- Matsubara I., Elliott G. F. X-ray diffraction studies on skinned single fibres of frog skeletal muscle. J Mol Biol. 1972 Dec 30;72(3):657–669. doi: 10.1016/0022-2836(72)90183-0. [DOI] [PubMed] [Google Scholar]
- Mendelson R. A., Morales M. F., Botts J. Segmental flexibility of the S-1 moiety of myosin. Biochemistry. 1973 Jun 5;12(12):2250–2255. doi: 10.1021/bi00736a011. [DOI] [PubMed] [Google Scholar]
- Miller A., Woodhead-Galloway J. Long range forces in muscle. Nature. 1971 Feb 12;229(5285):470–473. doi: 10.1038/229470a0. [DOI] [PubMed] [Google Scholar]
- Moore P. B., Huxley H. E., DeRosier D. J. Three-dimensional reconstruction of F-actin, thin filaments and decorated thin filaments. J Mol Biol. 1970 Jun 14;50(2):279–295. doi: 10.1016/0022-2836(70)90192-0. [DOI] [PubMed] [Google Scholar]
- Rapoport S. I. The anisotropic elastic properties of the sarcolemma of the frog semitendinosus muscle fiber. Biophys J. 1973 Jan;13(1):14–36. doi: 10.1016/S0006-3495(73)85967-3. [DOI] [PMC free article] [PubMed] [Google Scholar]