Abstract
The Nernst-Planck continuum equation for a channel that can be occupied by at most two ions is solved for two different physical cases. The first case is for the assumption that the water and ion cannot get around each other anywhere in the channel, so that if there are two ions in the channel the distance between them is fixed by the number of water molecules between them. The second case is for the assumption that there are regions at he ends of the channel where the ions and water can get around each other. For these two cases, the validity of the simple two-site reaction-rate approximation when there is a continuously varying central energy barrier was evaluated by comparing it with the exact Nernst-Planck solution. For the first continuum case, the kinetics for the continuum and reaction-rate models are nearly identical. For the second case, the agreement depends on the strength of the ion-ion interaction energy. For a low interaction energy (large channel diameter) a high ion concentrations, there is a large difference in the flux as a function of voltage for the two models-with the continuum flux becoming more than four times larger at 250 mV. Simple analytical expressions are derived for the two-ion continuum channel for the case where the ends are in equilibrium with the bulk solution and for the case where ion mobility becomes zero when there are two ions in the channel. The implications of these results for biological channels are discussed.
Full text
PDF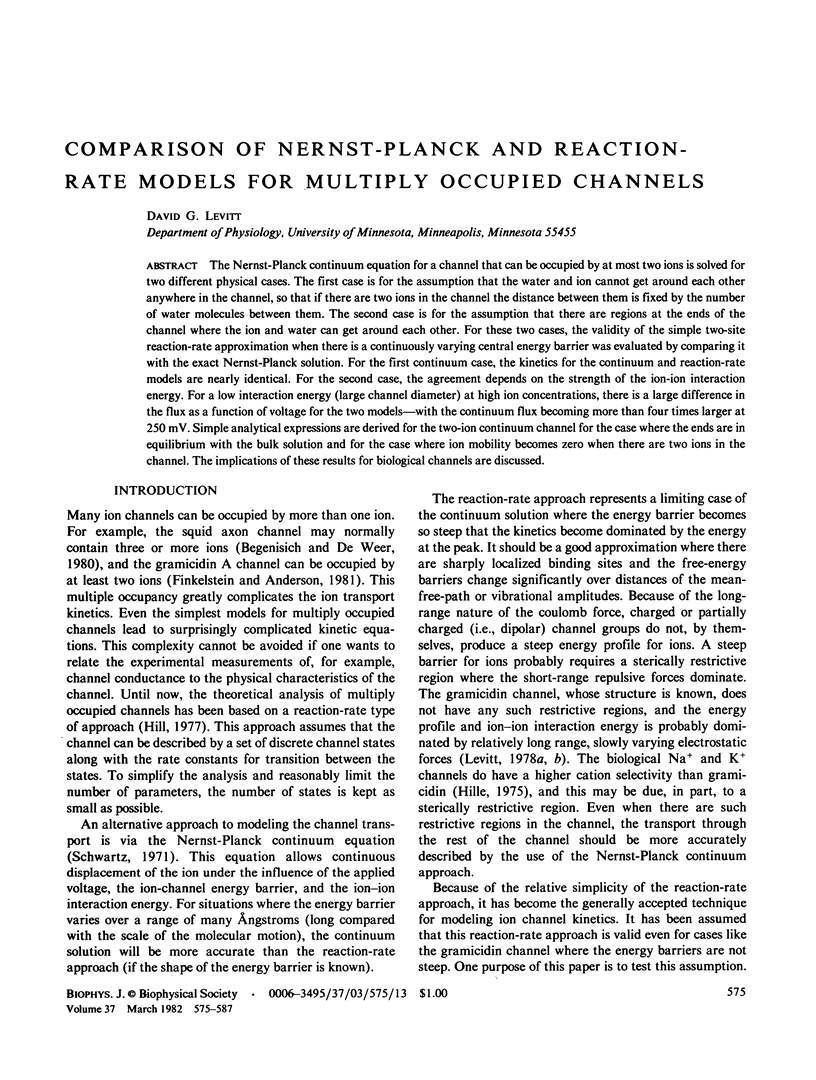
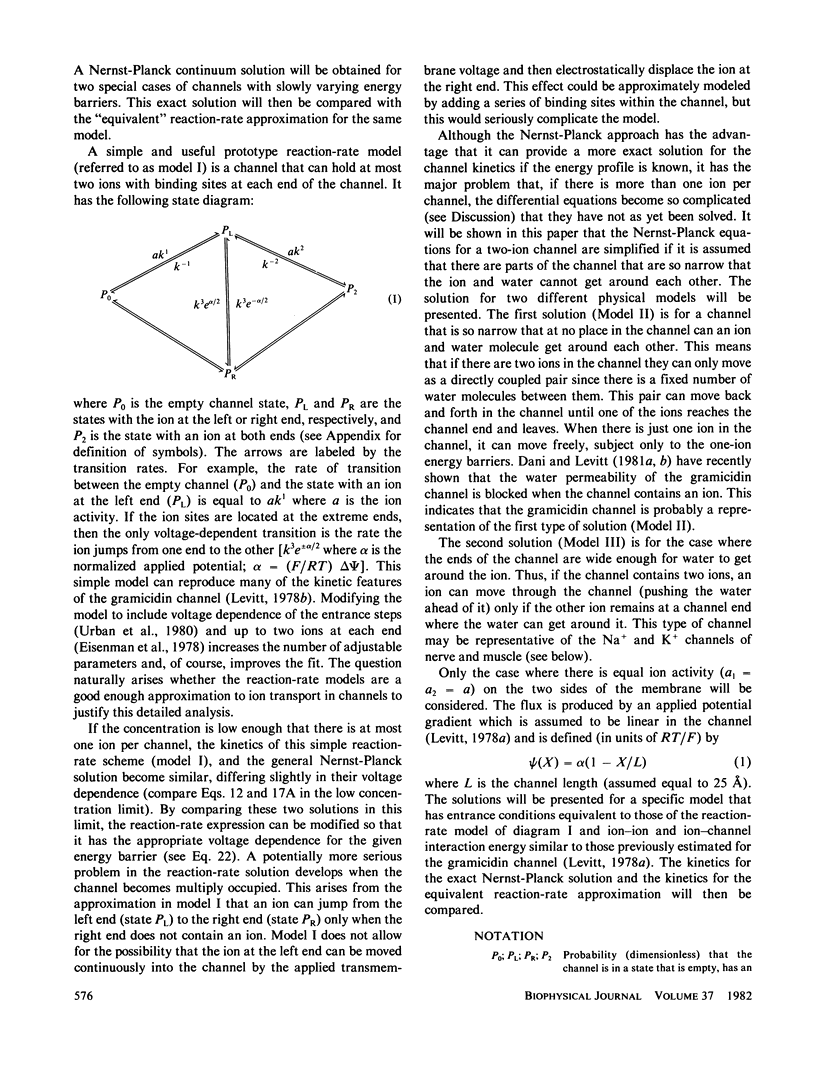
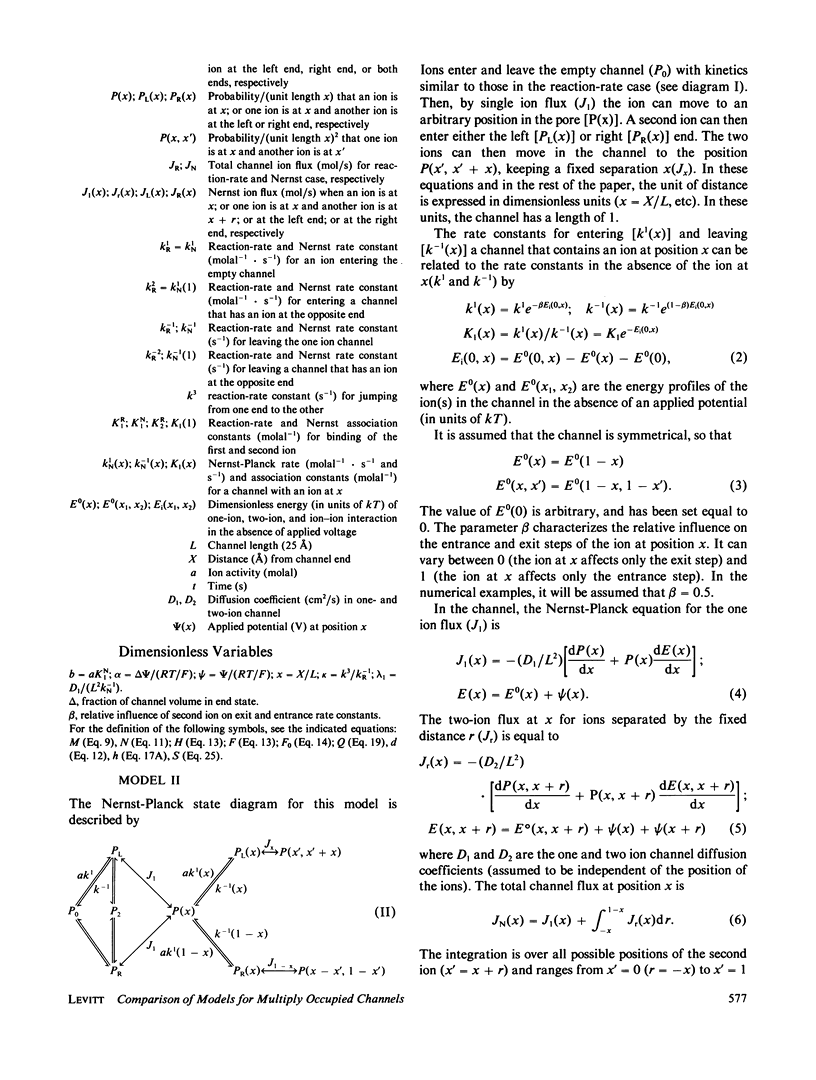
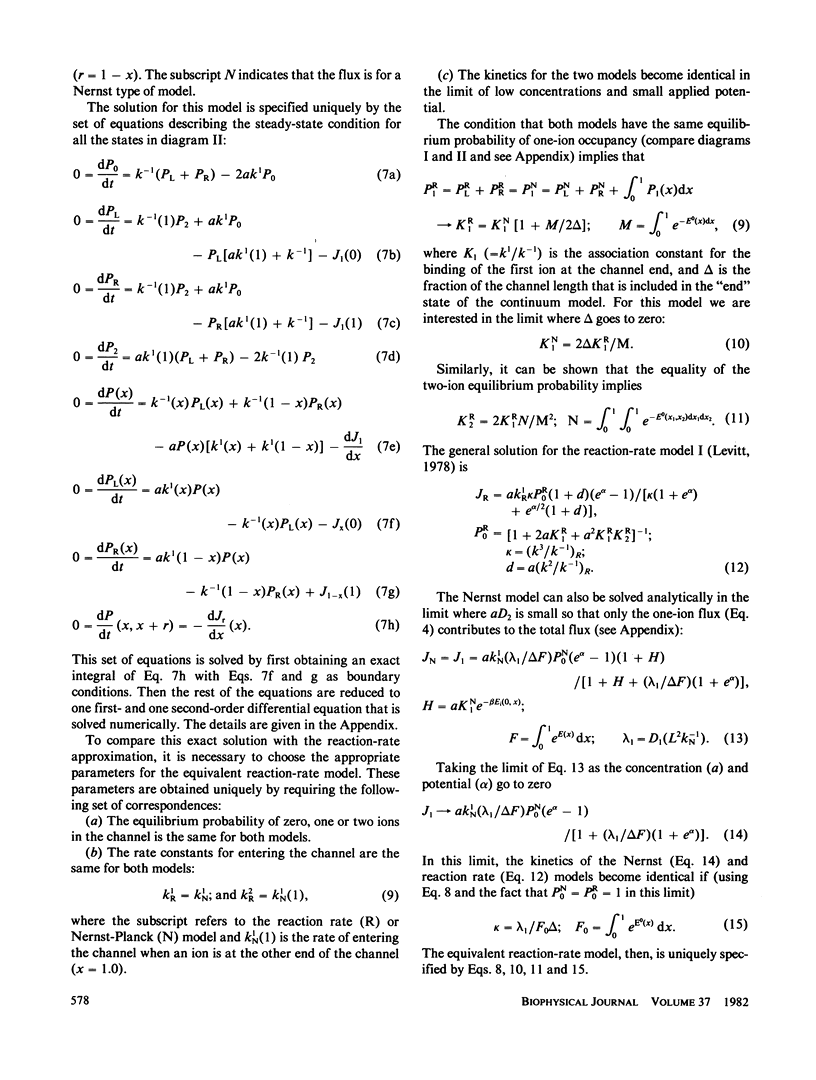
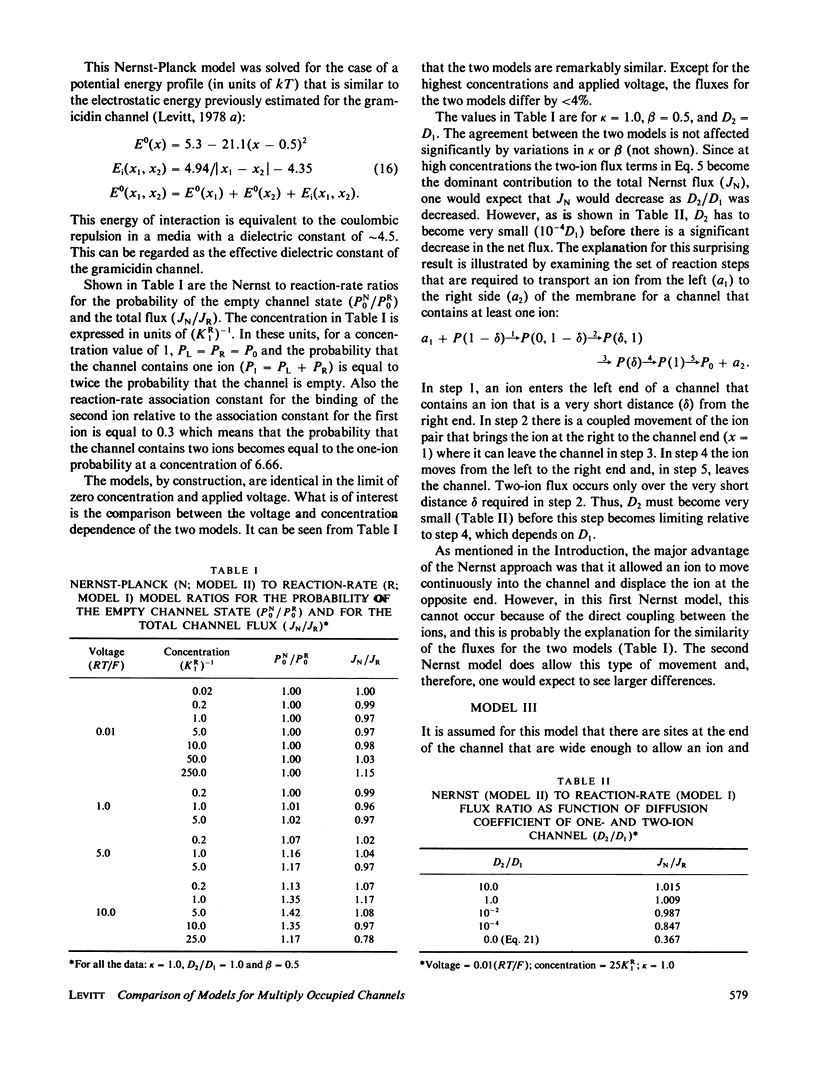
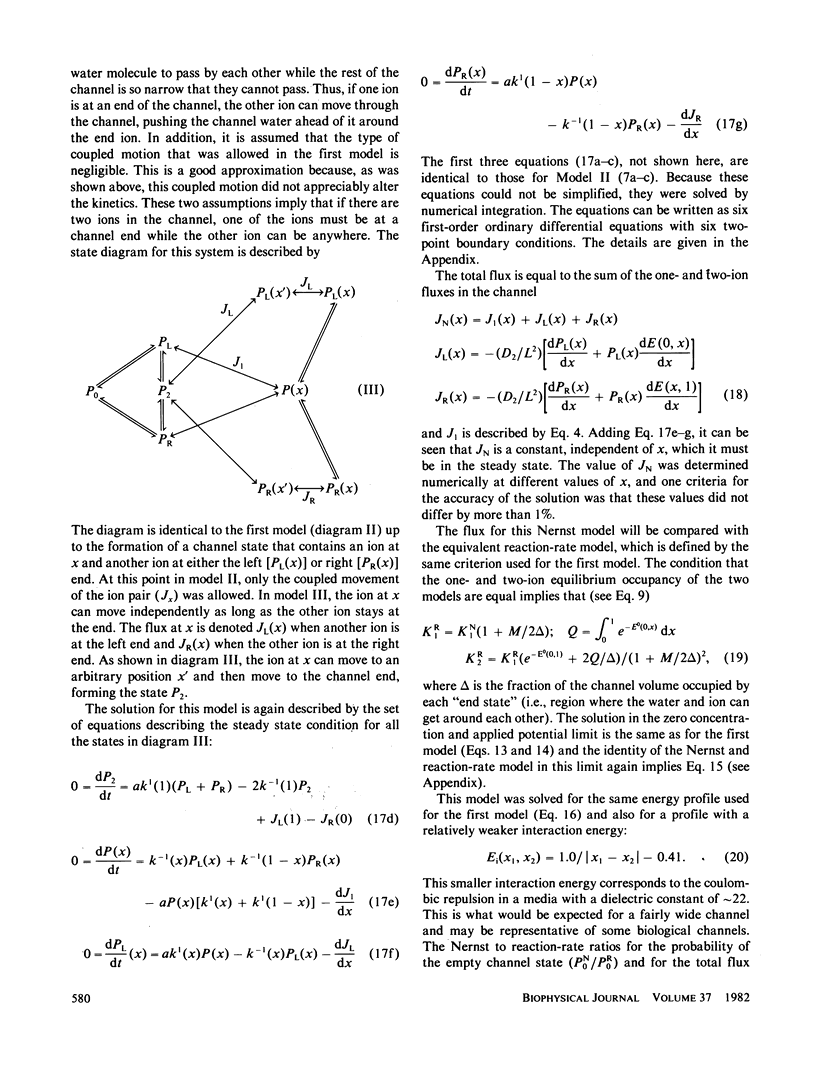
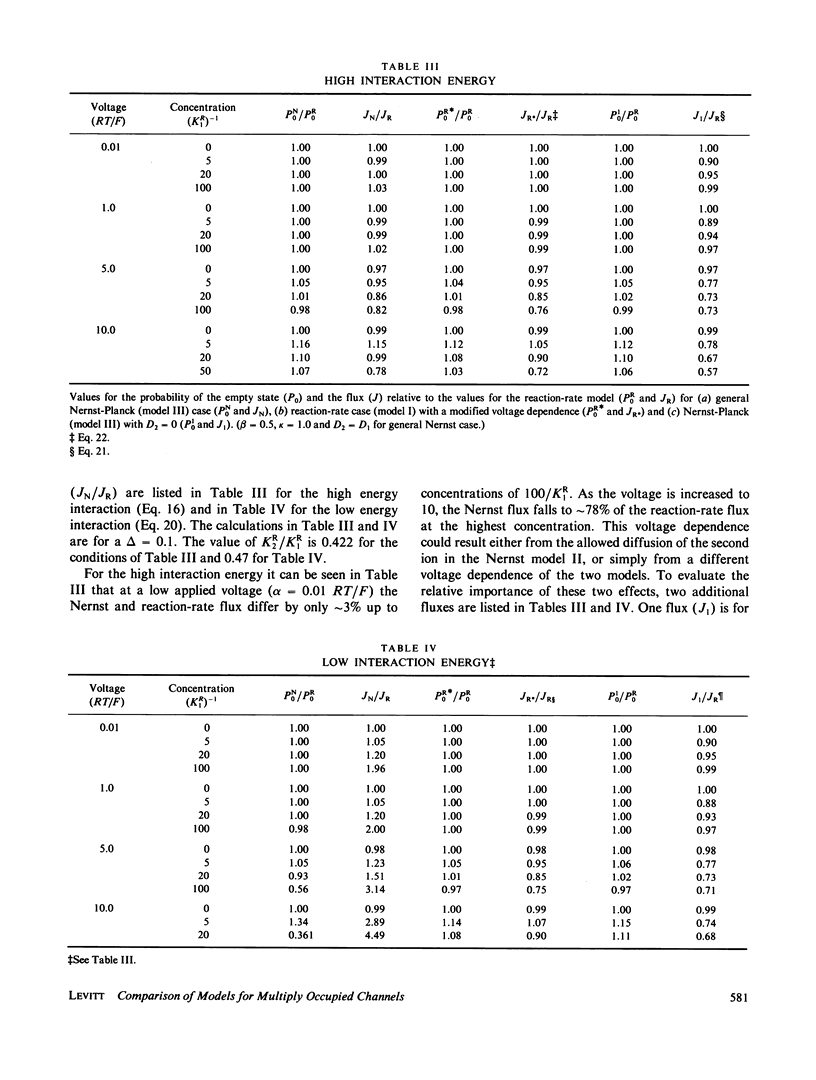
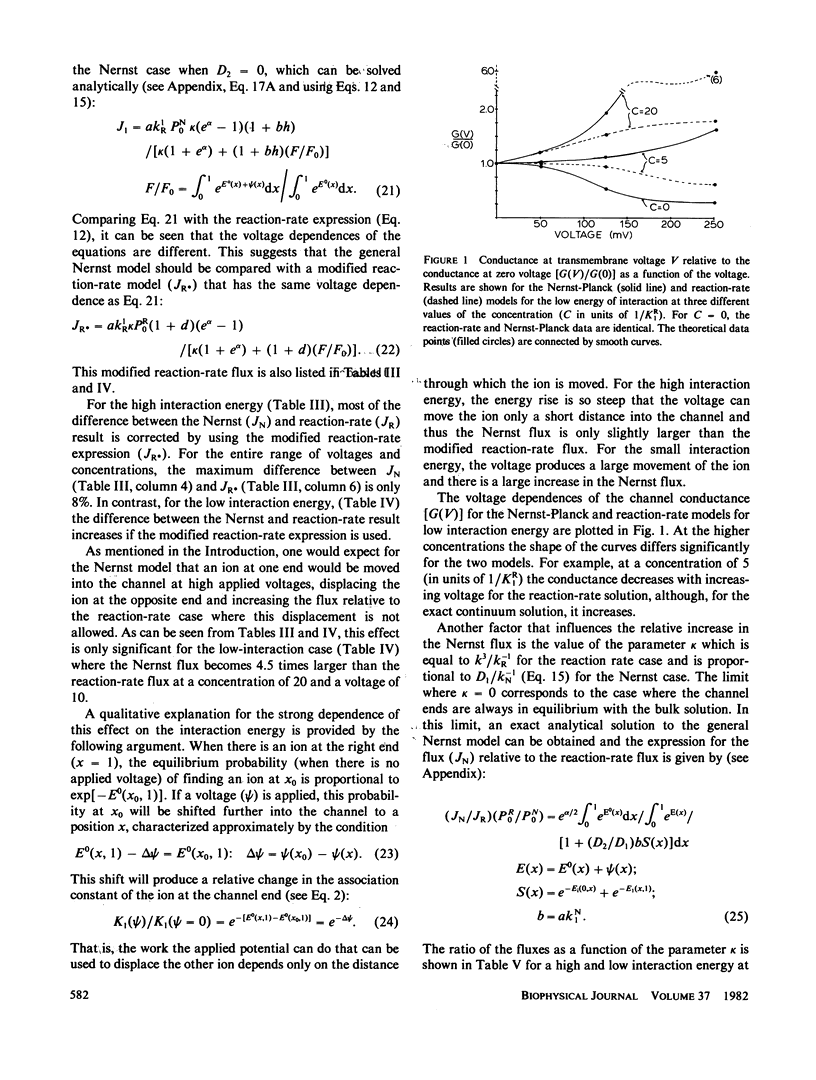
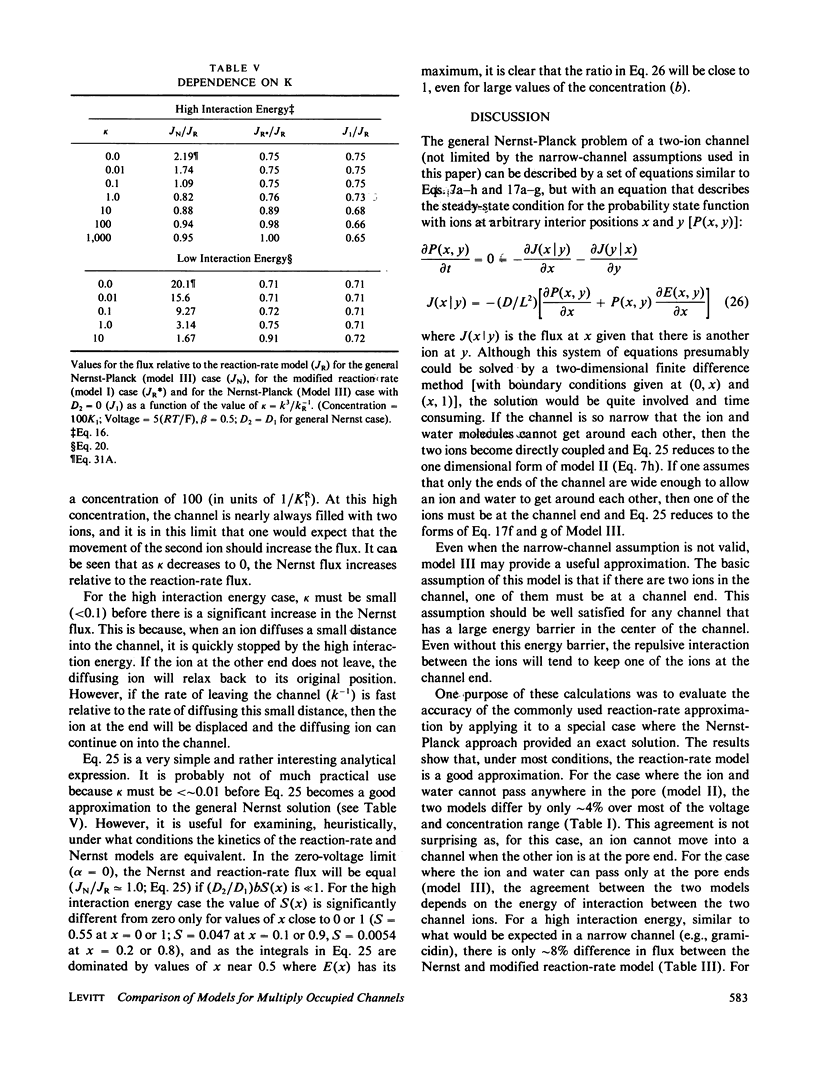
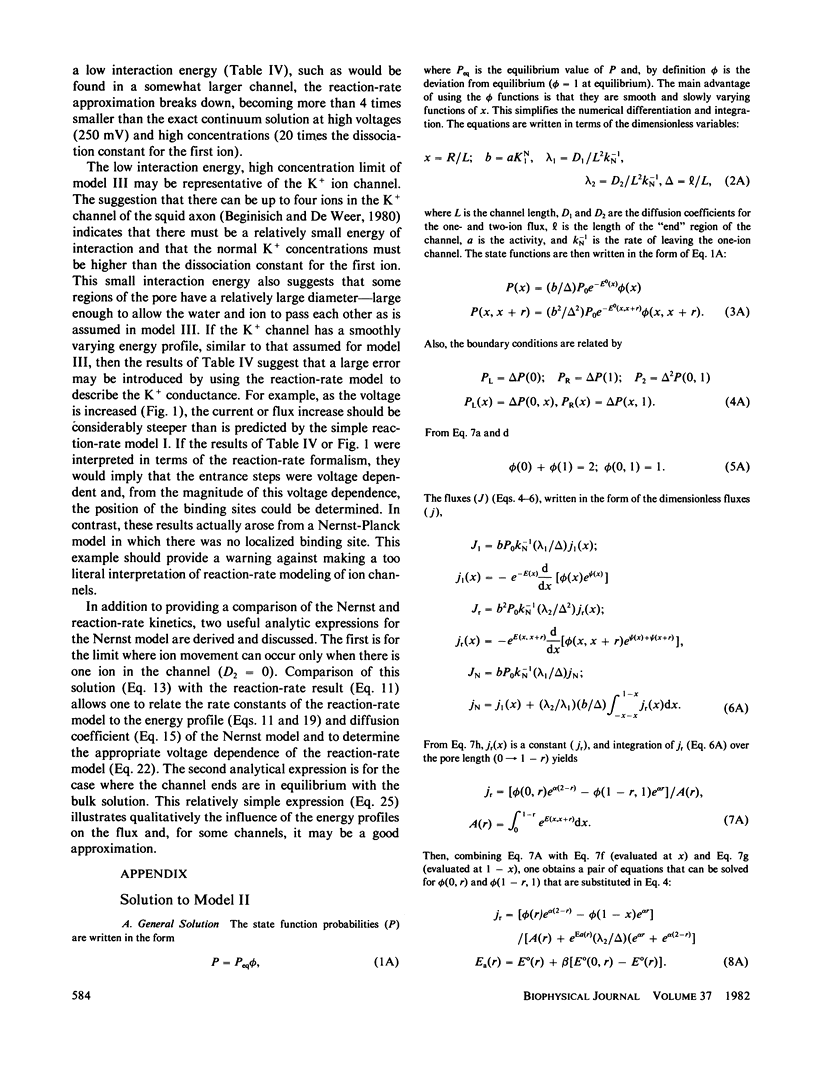
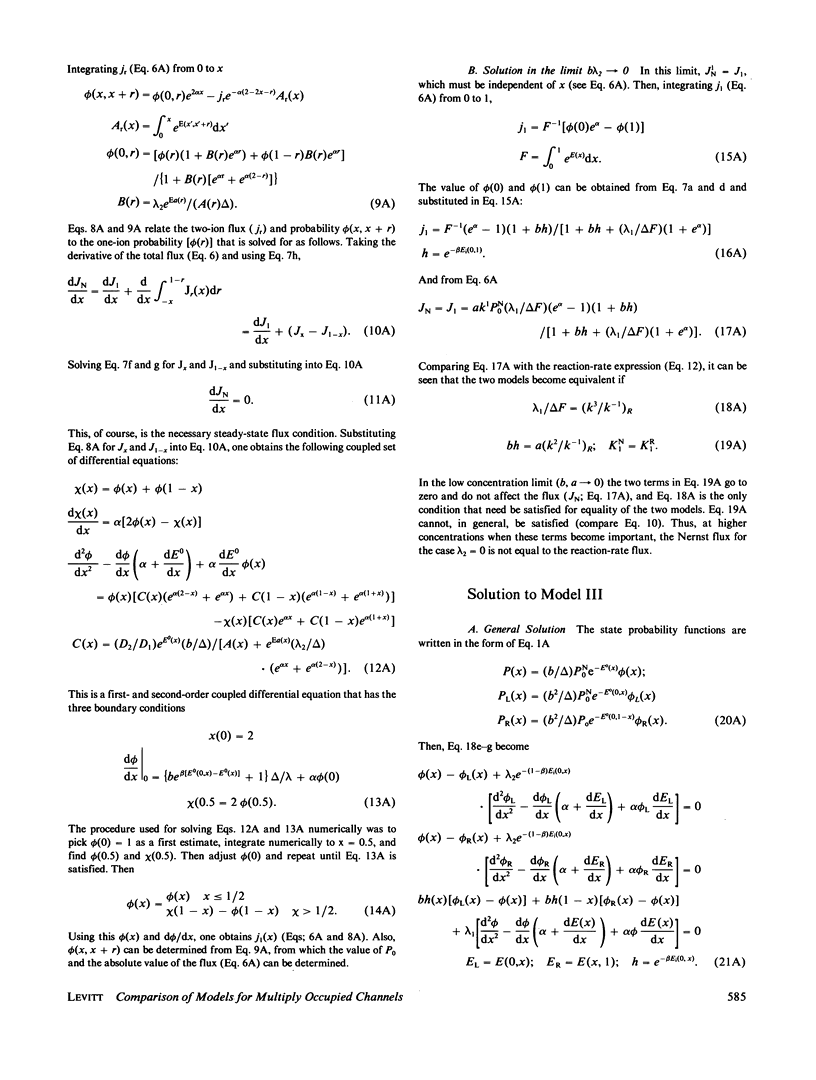
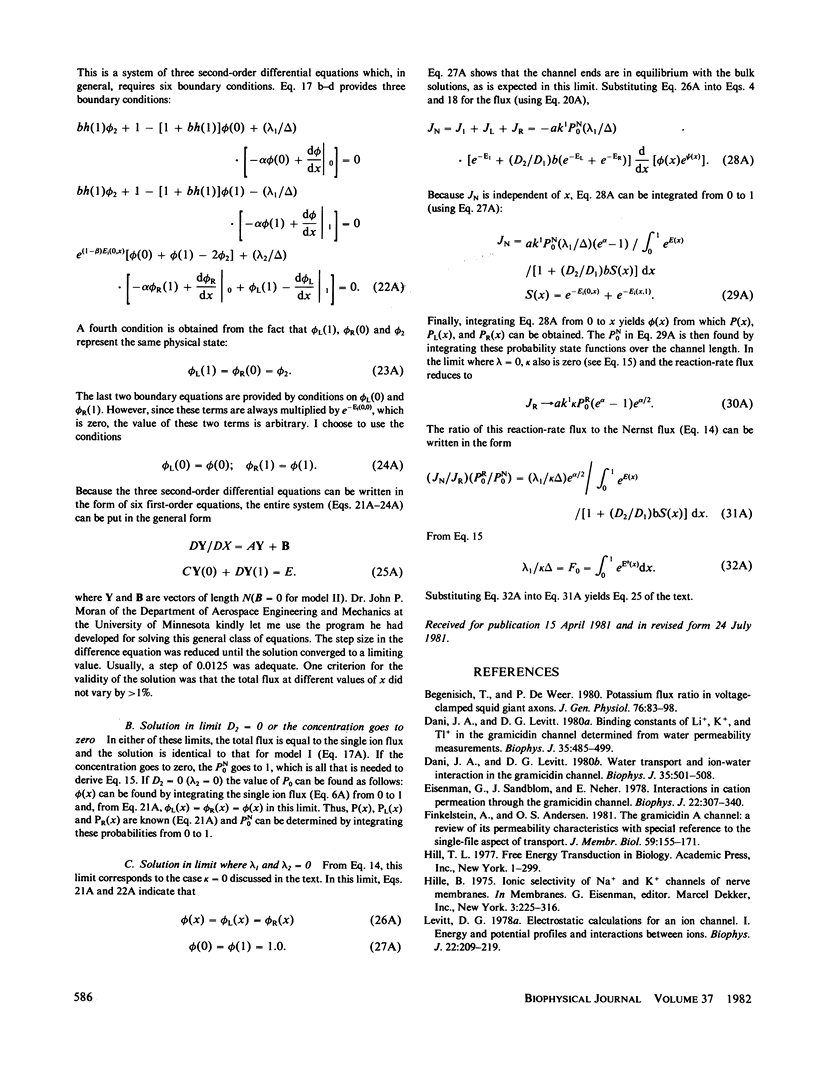
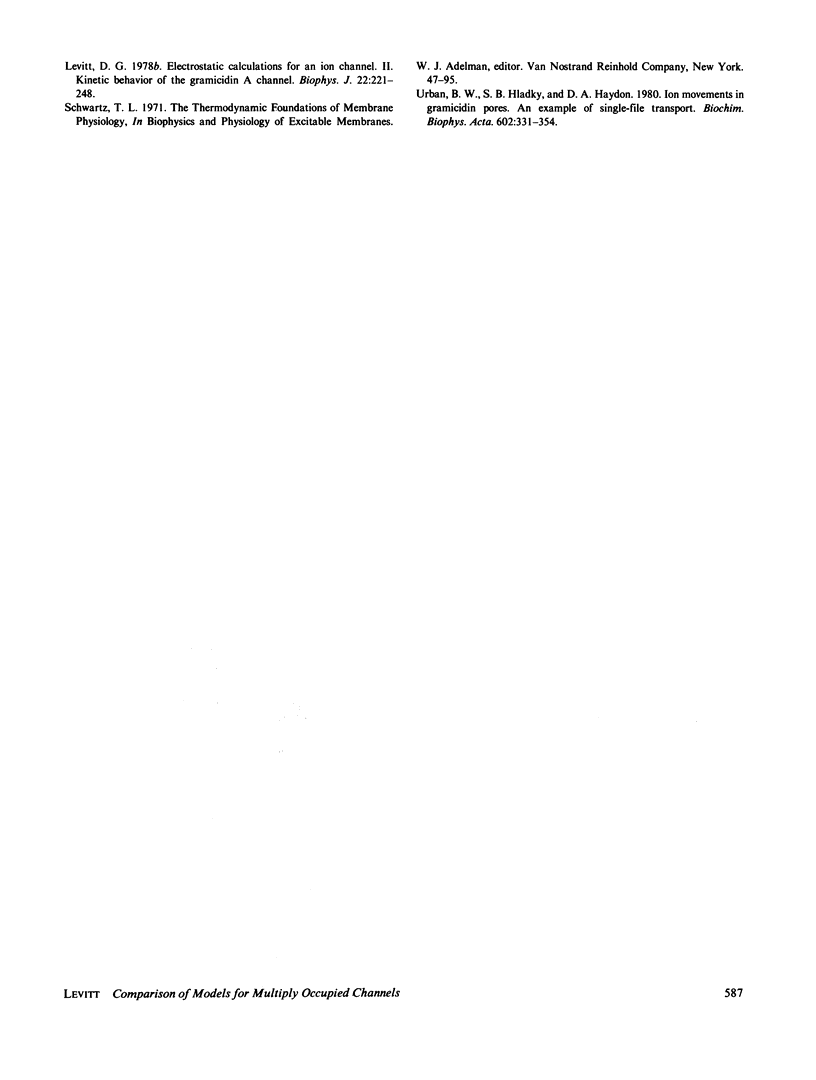
Selected References
These references are in PubMed. This may not be the complete list of references from this article.
- Begenisich T., De Weer P. Potassium flux ratio in voltage-clamped squid giant axons. J Gen Physiol. 1980 Jul;76(1):83–98. doi: 10.1085/jgp.76.1.83. [DOI] [PMC free article] [PubMed] [Google Scholar]
- Dani J. A., Levitt D. G. Binding constants of Li+, K+, and Tl+ in the gramicidin channel determined from water permeability measurements. Biophys J. 1981 Aug;35(2):485–499. doi: 10.1016/S0006-3495(81)84804-7. [DOI] [PMC free article] [PubMed] [Google Scholar]
- Dani J. A., Levitt D. G. Water transport and ion-water interaction in the gramicidin channel. Biophys J. 1981 Aug;35(2):501–508. doi: 10.1016/S0006-3495(81)84805-9. [DOI] [PMC free article] [PubMed] [Google Scholar]
- Eisenman G., Sandblom J., Neher E. Interactions in cation permeation through the gramicidin channel. Cs, Rb, K, Na, Li, Tl, H, and effects of anion binding. Biophys J. 1978 May;22(2):307–340. doi: 10.1016/S0006-3495(78)85491-5. [DOI] [PMC free article] [PubMed] [Google Scholar]
- Finkelstein A., Andersen O. S. The gramicidin A channel: a review of its permeability characteristics with special reference to the single-file aspect of transport. J Membr Biol. 1981 Apr 30;59(3):155–171. doi: 10.1007/BF01875422. [DOI] [PubMed] [Google Scholar]
- Levitt D. G. Electrostatic calculations for an ion channel. I. Energy and potential profiles and interactions between ions. Biophys J. 1978 May;22(2):209–219. doi: 10.1016/S0006-3495(78)85485-X. [DOI] [PMC free article] [PubMed] [Google Scholar]
- Levitt D. G. Electrostatic calculations for an ion channel. II. Kinetic behavior of the gramicidin A channel. Biophys J. 1978 May;22(2):221–248. doi: 10.1016/S0006-3495(78)85486-1. [DOI] [PMC free article] [PubMed] [Google Scholar]
- Urban B. W., Hladky S. B., Haydon D. A. Ion movements in gramicidin pores. An example of single-file transport. Biochim Biophys Acta. 1980 Nov 4;602(2):331–354. doi: 10.1016/0005-2736(80)90316-8. [DOI] [PubMed] [Google Scholar]