Abstract
A numerical model of a muscle fiber as 400 sarcomeres, identical except for their initial lengths, was used to simulate fixed-end tetanic contractions of frog single fibers at sarcomere lengths above the optimum. The sarcomeres were represented by a lumped model, constructed from the passive and active sarcomere length-tension curves, the force-velocity curve, and the observed active elasticity of a single frog muscle fiber. An intersarcomere force was included to prevent large disparities in lengths of neighboring sarcomeres. The model duplicated the fast rise, slow creep rise, peak, and slow decline of tension seen in tetanic contractions of stretched living fibers. Decreasing the initial non-uniformity of sarcomere length reduced the rate of rise of tension during the creep phase, but did not decrease the peak tension reached. Limitations of the model, and other processes that might contribute to the shape of the fixed end tetanic tension record are discussed. Taking account of model and experimental results, it is concluded that the distinctive features of the tension records of fixed end tetanic contraction at lengths beyond optimum can be explained by internal motion within the fiber.
Full text
PDF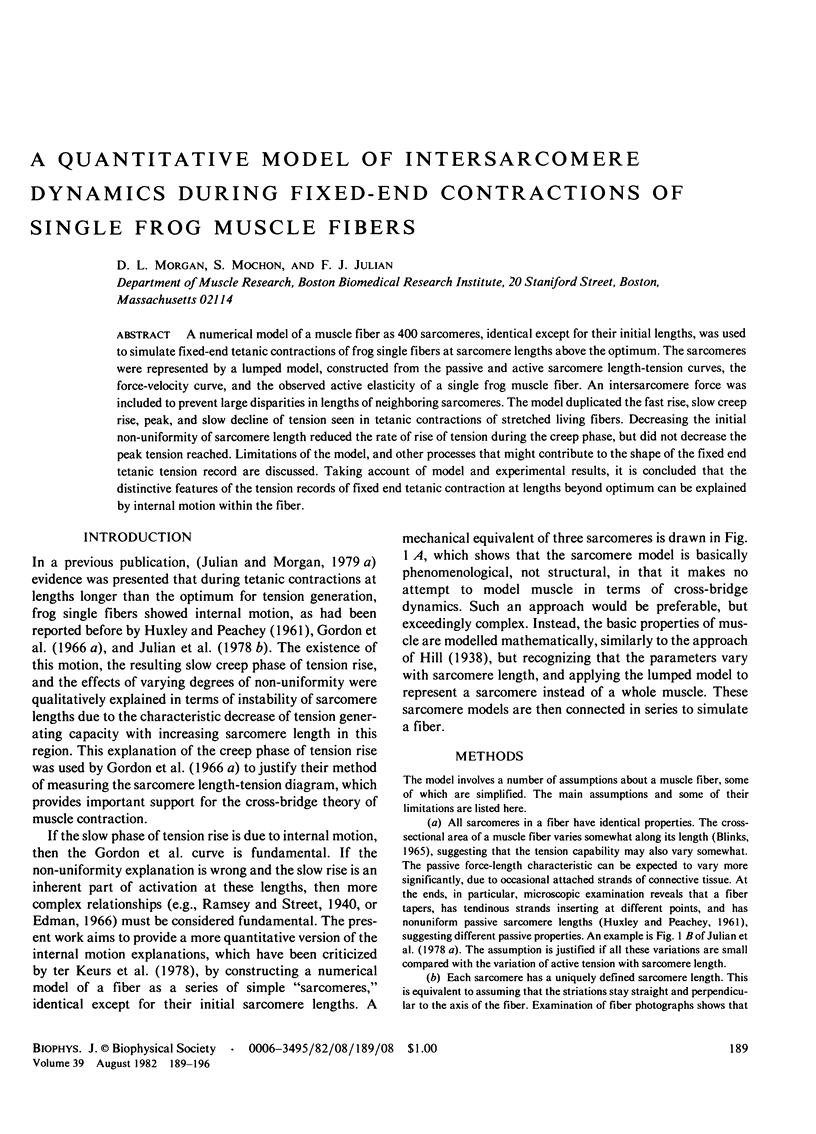
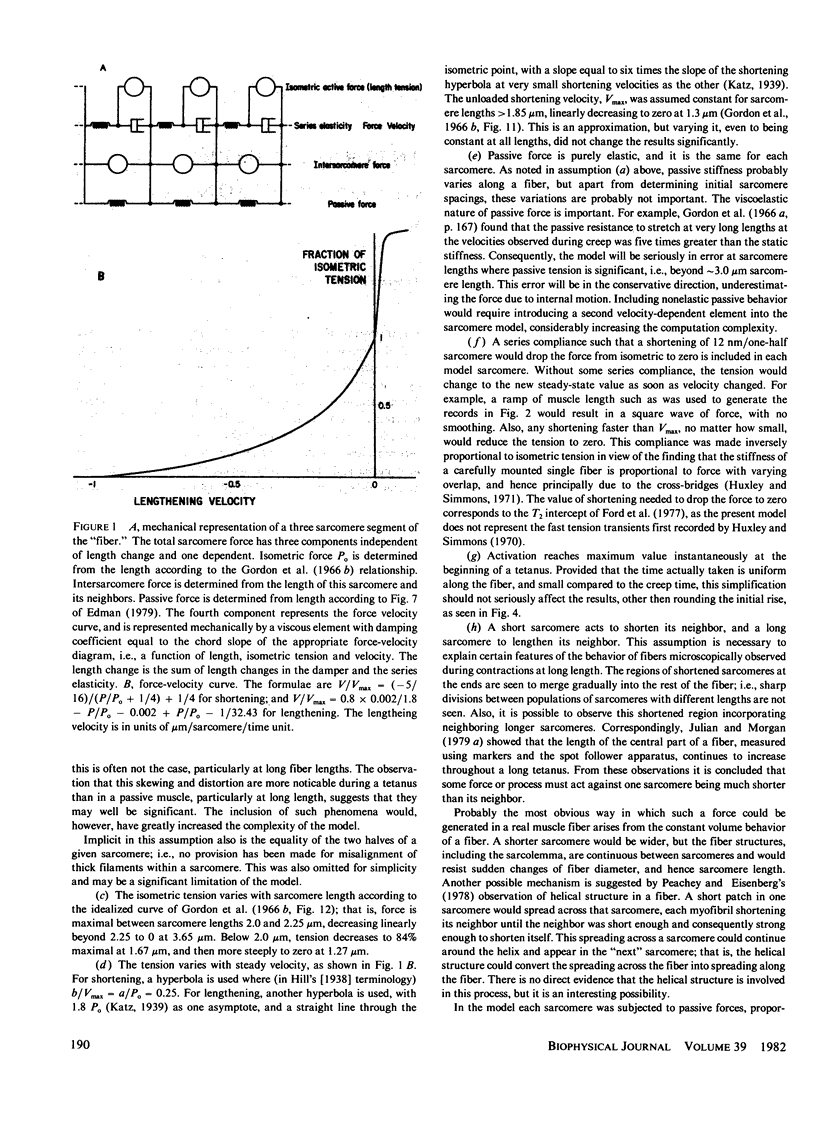
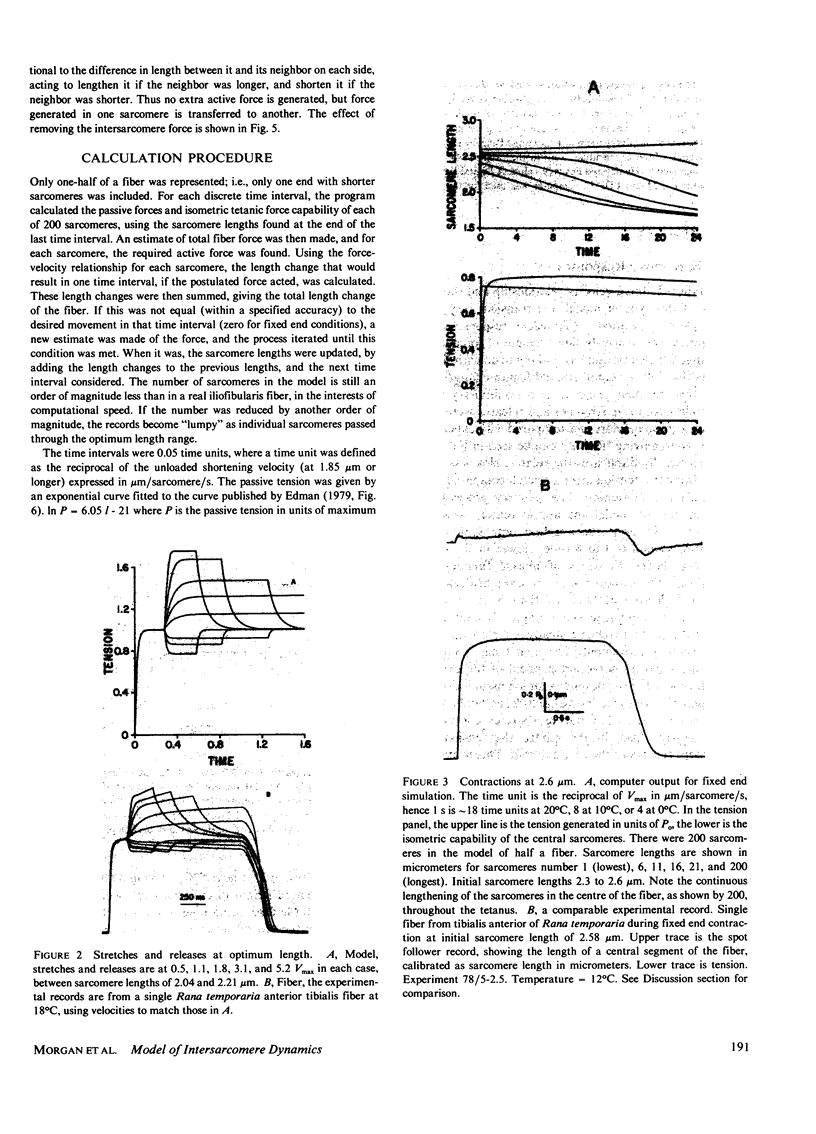
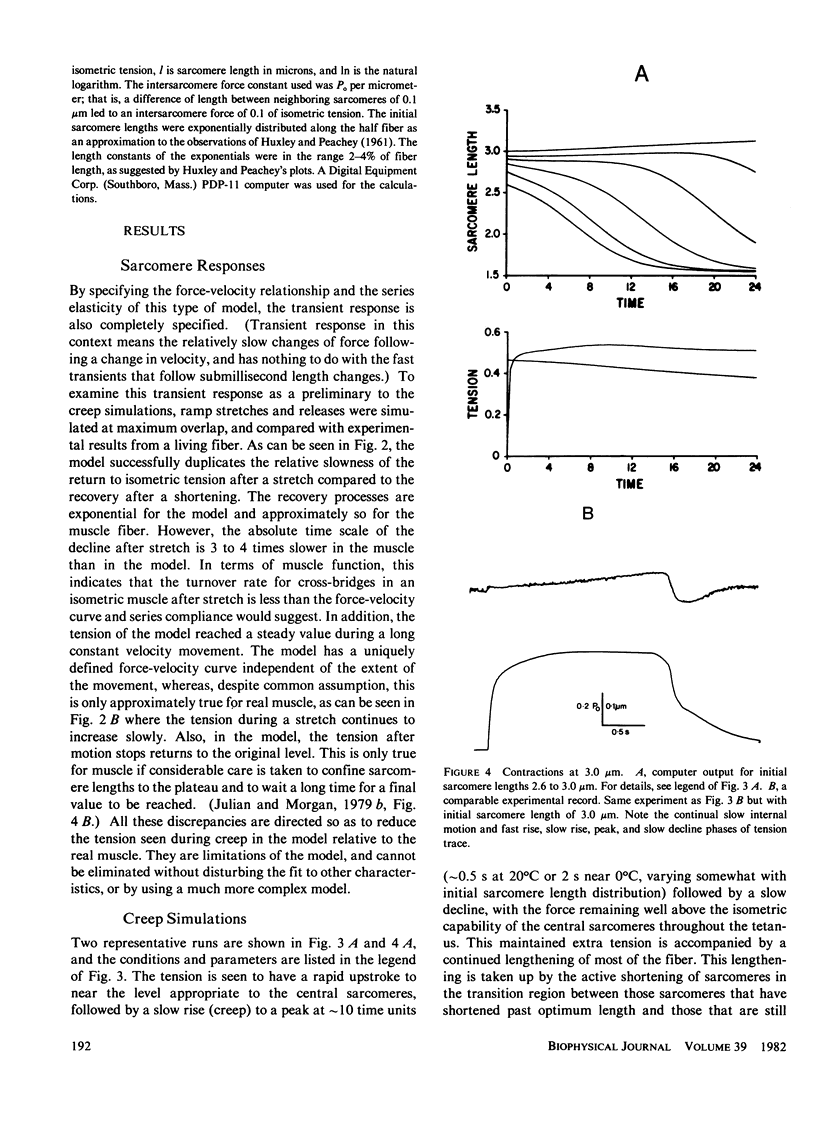
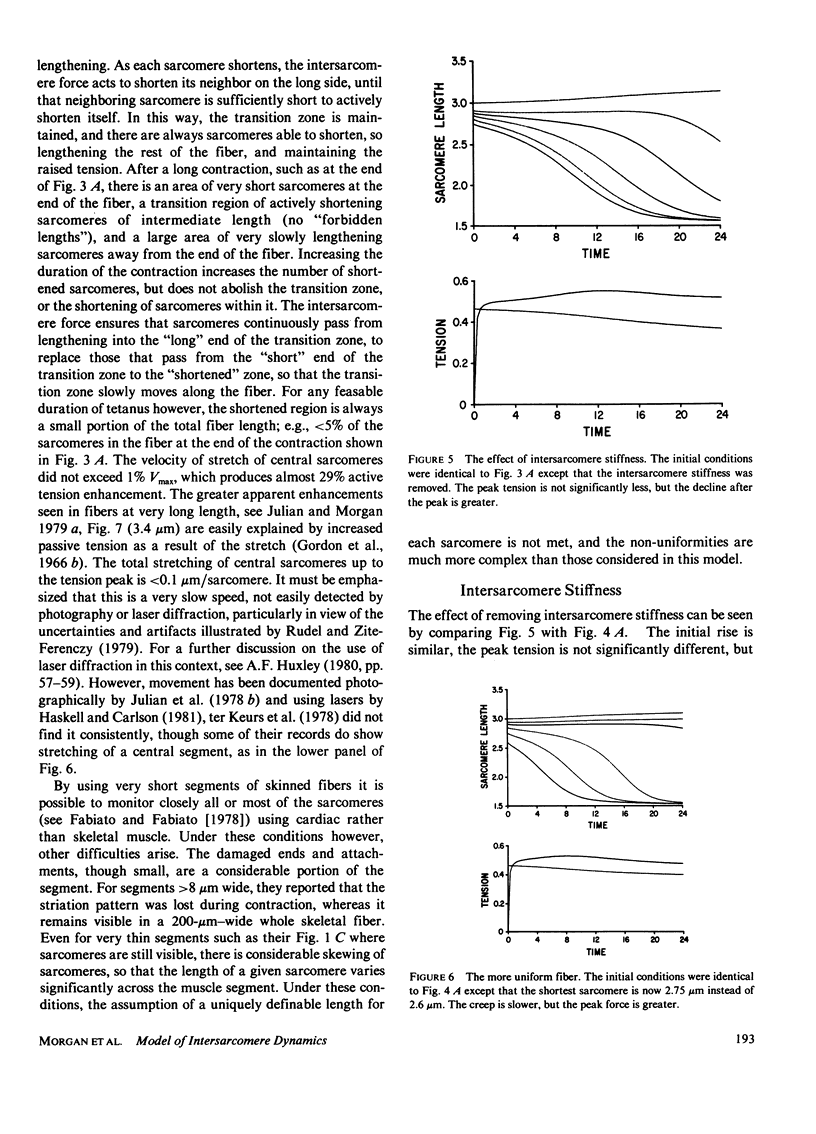
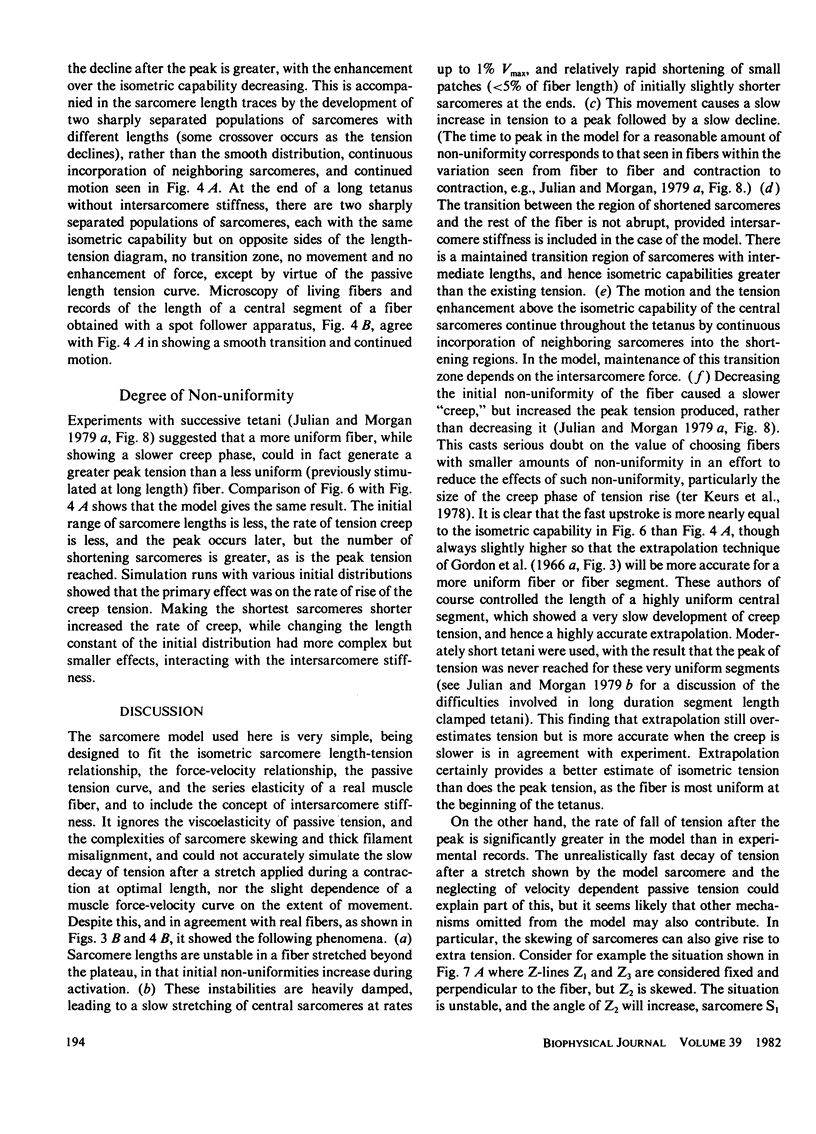
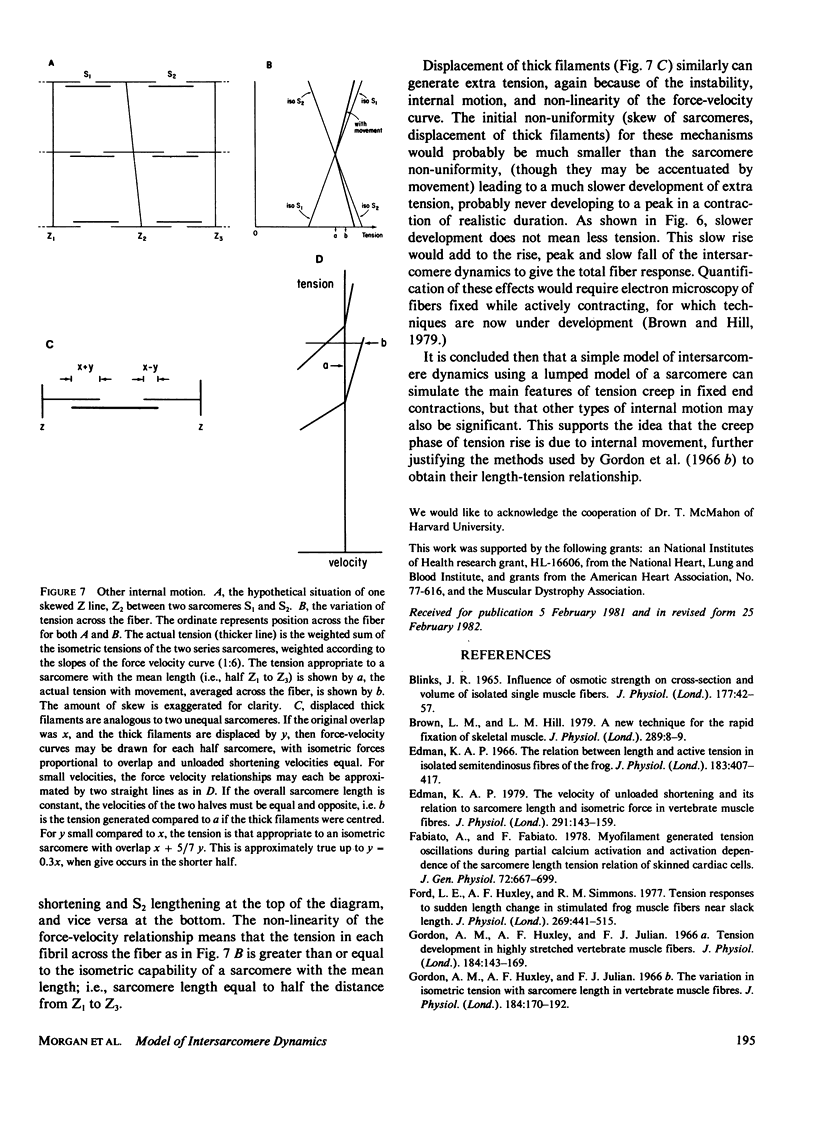
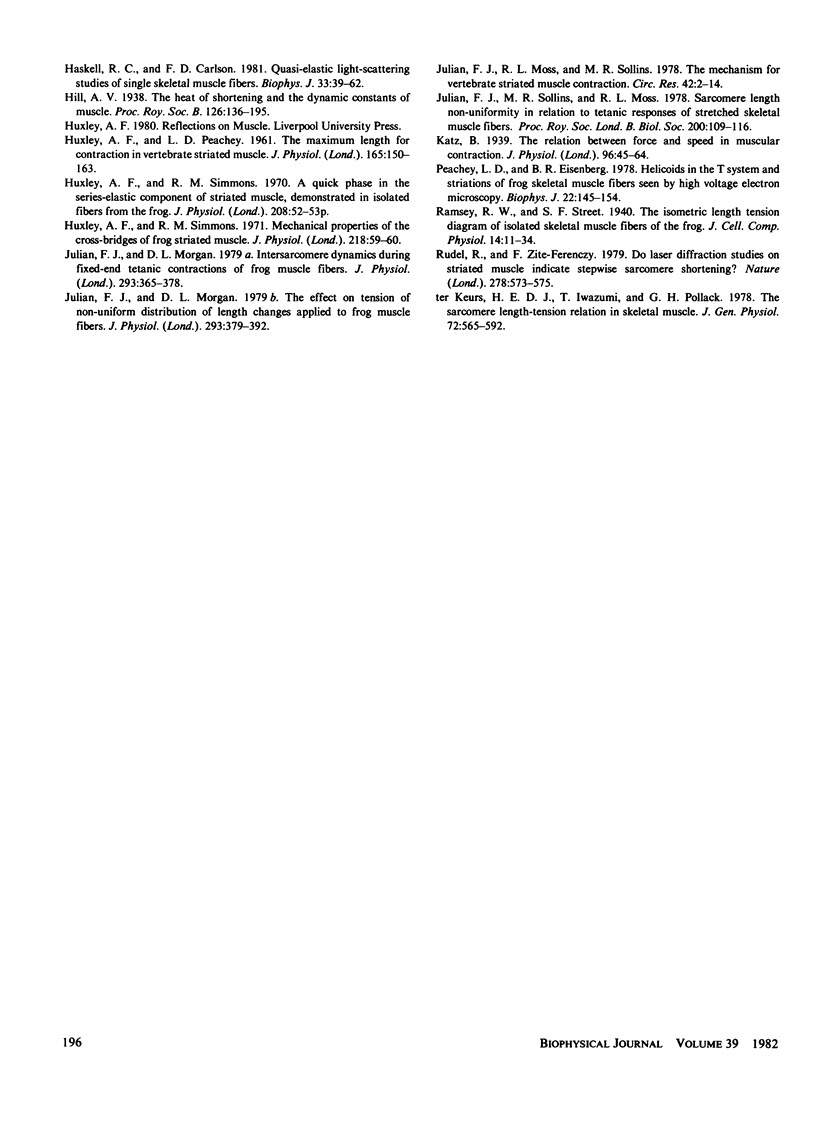
Selected References
These references are in PubMed. This may not be the complete list of references from this article.
- BLINKS J. R. INFLUENCE OF OSMOTIC STRENGTH ON CROSS-SECTION AND VOLUME OF ISOLATED SINGLE MUSCLE FIBRES. J Physiol. 1965 Mar;177:42–57. doi: 10.1113/jphysiol.1965.sp007574. [DOI] [PMC free article] [PubMed] [Google Scholar]
- Edman K. A. The relation between sarcomere length and active tension in isolated semitendinosus fibres of the frog. J Physiol. 1966 Mar;183(2):407–417. doi: 10.1113/jphysiol.1966.sp007873. [DOI] [PMC free article] [PubMed] [Google Scholar]
- Edman K. A. The velocity of unloaded shortening and its relation to sarcomere length and isometric force in vertebrate muscle fibres. J Physiol. 1979 Jun;291:143–159. doi: 10.1113/jphysiol.1979.sp012804. [DOI] [PMC free article] [PubMed] [Google Scholar]
- Fabiato A., Fabiato F. Myofilament-generated tension oscillations during partial calcium activation and activation dependence of the sarcomere length-tension relation of skinned cardiac cells. J Gen Physiol. 1978 Nov;72(5):667–699. doi: 10.1085/jgp.72.5.667. [DOI] [PMC free article] [PubMed] [Google Scholar]
- Ford L. E., Huxley A. F., Simmons R. M. Tension responses to sudden length change in stimulated frog muscle fibres near slack length. J Physiol. 1977 Jul;269(2):441–515. doi: 10.1113/jphysiol.1977.sp011911. [DOI] [PMC free article] [PubMed] [Google Scholar]
- Gordon A. M., Huxley A. F., Julian F. J. Tension development in highly stretched vertebrate muscle fibres. J Physiol. 1966 May;184(1):143–169. doi: 10.1113/jphysiol.1966.sp007908. [DOI] [PMC free article] [PubMed] [Google Scholar]
- HUXLEY A. F., PEACHEY L. D. The maximum length for contraction in vertebrate straiated muscle. J Physiol. 1961 Apr;156:150–165. doi: 10.1113/jphysiol.1961.sp006665. [DOI] [PMC free article] [PubMed] [Google Scholar]
- Haskell R. C., Carlson F. D. Quasi-elastic light-scattering studies of single skeletal muscle fibers. Biophys J. 1981 Jan;33(1):39–62. doi: 10.1016/S0006-3495(81)84871-0. [DOI] [PMC free article] [PubMed] [Google Scholar]
- Julian F. J., Morgan D. L. Intersarcomere dynamics during fixed-end tetanic contractions of frog muscle fibres. J Physiol. 1979 Aug;293:365–378. doi: 10.1113/jphysiol.1979.sp012894. [DOI] [PMC free article] [PubMed] [Google Scholar]
- Julian F. J., Morgan D. L. The effect on tension of non-uniform distribution of length changes applied to frog muscle fibres. J Physiol. 1979 Aug;293:379–392. doi: 10.1113/jphysiol.1979.sp012895. [DOI] [PMC free article] [PubMed] [Google Scholar]
- Julian F. J., Moss R. L., Sollins M. R. The mechanism for vertebrate striated muscle contraction. Circ Res. 1978 Jan;42(1):2–14. doi: 10.1161/01.res.42.1.2. [DOI] [PubMed] [Google Scholar]
- Julian F. J., Sollins M. R., Moss R. L. Sarcomere length non-uniformity in relation to tetanic responses of stretched skeletal muscle fibres. Proc R Soc Lond B Biol Sci. 1978 Jan 24;200(1138):109–116. doi: 10.1098/rspb.1978.0009. [DOI] [PubMed] [Google Scholar]
- Katz B. The relation between force and speed in muscular contraction. J Physiol. 1939 Jun 14;96(1):45–64. doi: 10.1113/jphysiol.1939.sp003756. [DOI] [PMC free article] [PubMed] [Google Scholar]
- Peachey L. D., Eisenberg B. R. Helicoids in the T system and striations of frog skeletal muscle fibers seen by high voltage electron microscopy. Biophys J. 1978 May;22(2):145–154. doi: 10.1016/S0006-3495(78)85480-0. [DOI] [PMC free article] [PubMed] [Google Scholar]
- Rüdel R., Zite-Ferenczy F. Do laser diffraction studies on striated muscle indicate stepwise sarcomere shortening? Nature. 1979 Apr 5;278(5704):573–575. doi: 10.1038/278573a0. [DOI] [PubMed] [Google Scholar]
- ter Keurs H. E., Iwazumi T., Pollack G. H. The sarcomere length-tension relation in skeletal muscle. J Gen Physiol. 1978 Oct;72(4):565–592. doi: 10.1085/jgp.72.4.565. [DOI] [PMC free article] [PubMed] [Google Scholar]