Abstract
When isotonic force steps were applied to activated papillary muscles, the velocity was almost never constant. Early rapid shortening associated with the step persisted for 2-7 ms after the step ends. The early rapid shortening is attributed to lightly damped series elastic recoil and velocity transients of the contractile elements. In most steps, the subsequent velocity declines progressively with shortening, and most of the decline in velocity can be accounted for by compression of a viscoelastic element in parallel with the contractile elements. To demonstrate this, the time course of isotonic velocity was compared with a model in which the force-velocity characteristics of the contractile element were assumed to be constant, and the decline in velocity was due to increasing compression of the viscoelastic element. This model predicted the observed results except that the predicted velocities rose progressively above the measured values for steps to light loads applied late in the twitch, and fell below the velocity trace for heavy loads applied early in the twitch. These deviations would occur if rapid shortening caused deactivation late in the twitch, and if activation were rising early in the twitch. A conditioning step applied to the muscle during the rise of force depressed both isometric force and maximum velocity measured at the peak of force; isometric force was more depressed with later conditioning steps than with earlier steps, while maximum velocity was depressed by about the same extent with both early and late steps. This difference between the effects on isometric force and maximum velocity are explained by a combination of deactivation and viscoelastic load.
Full text
PDF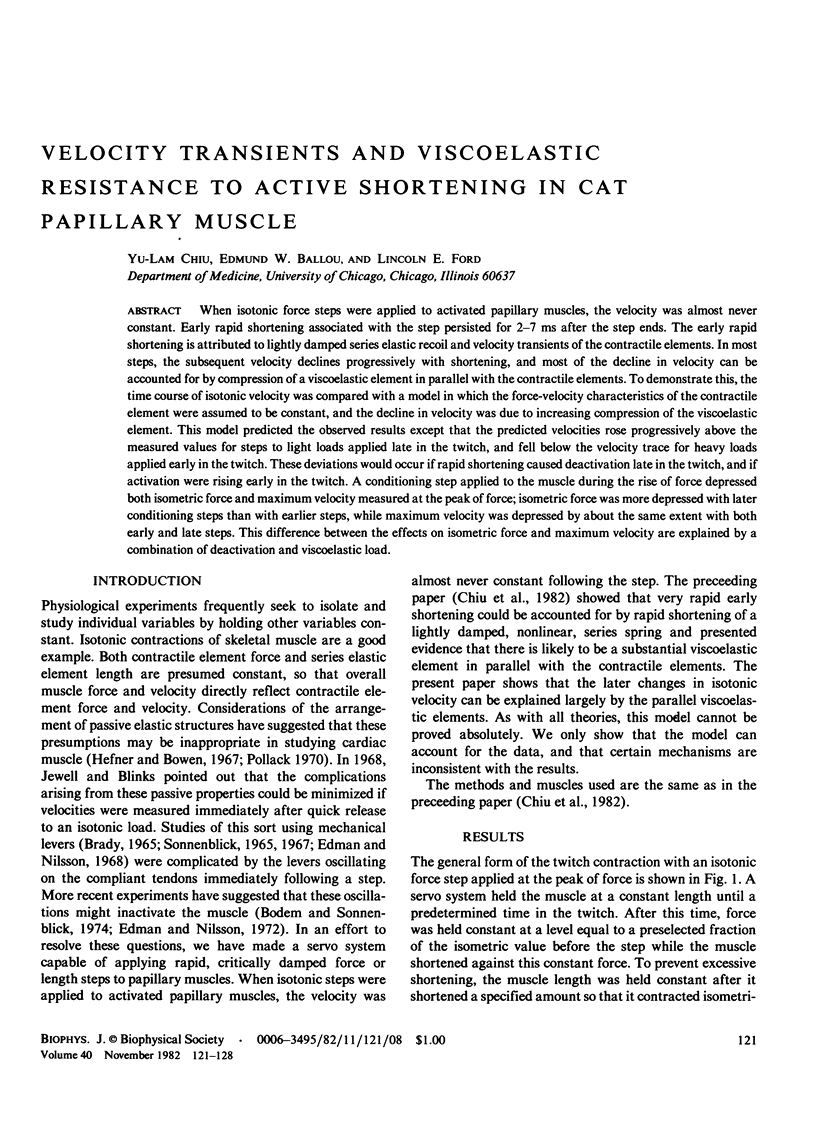
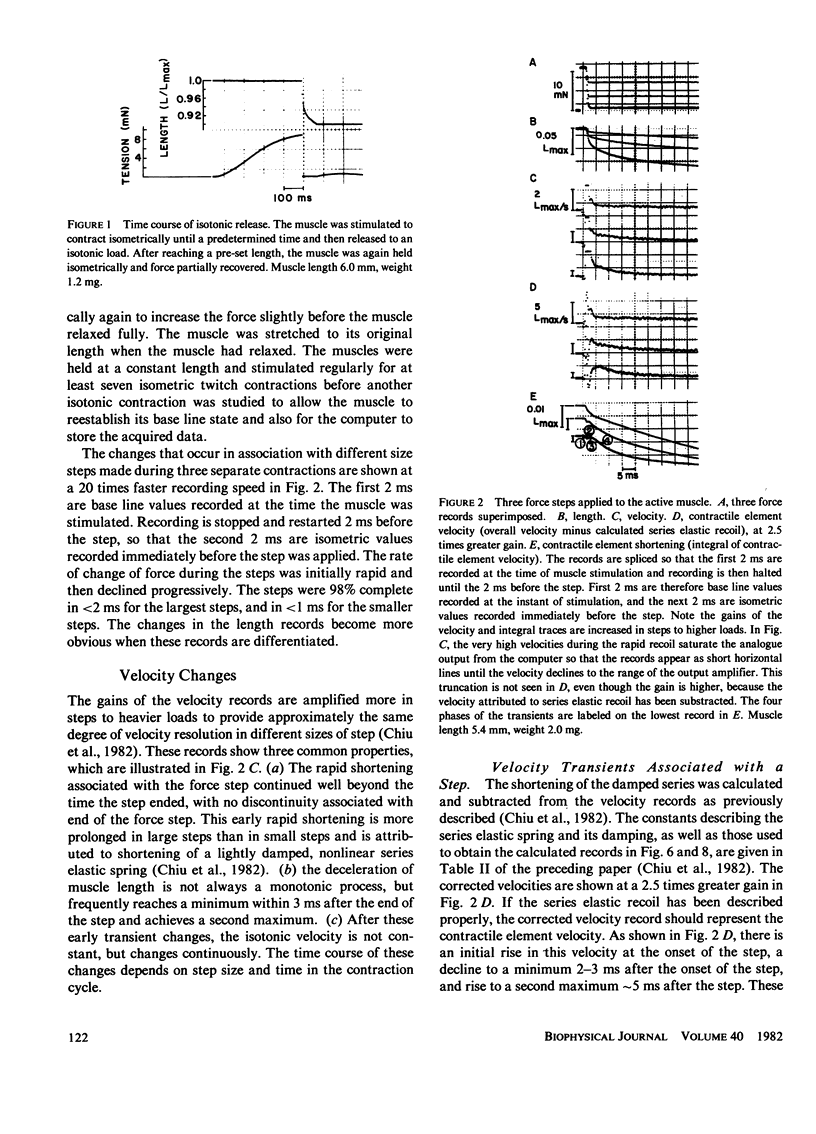
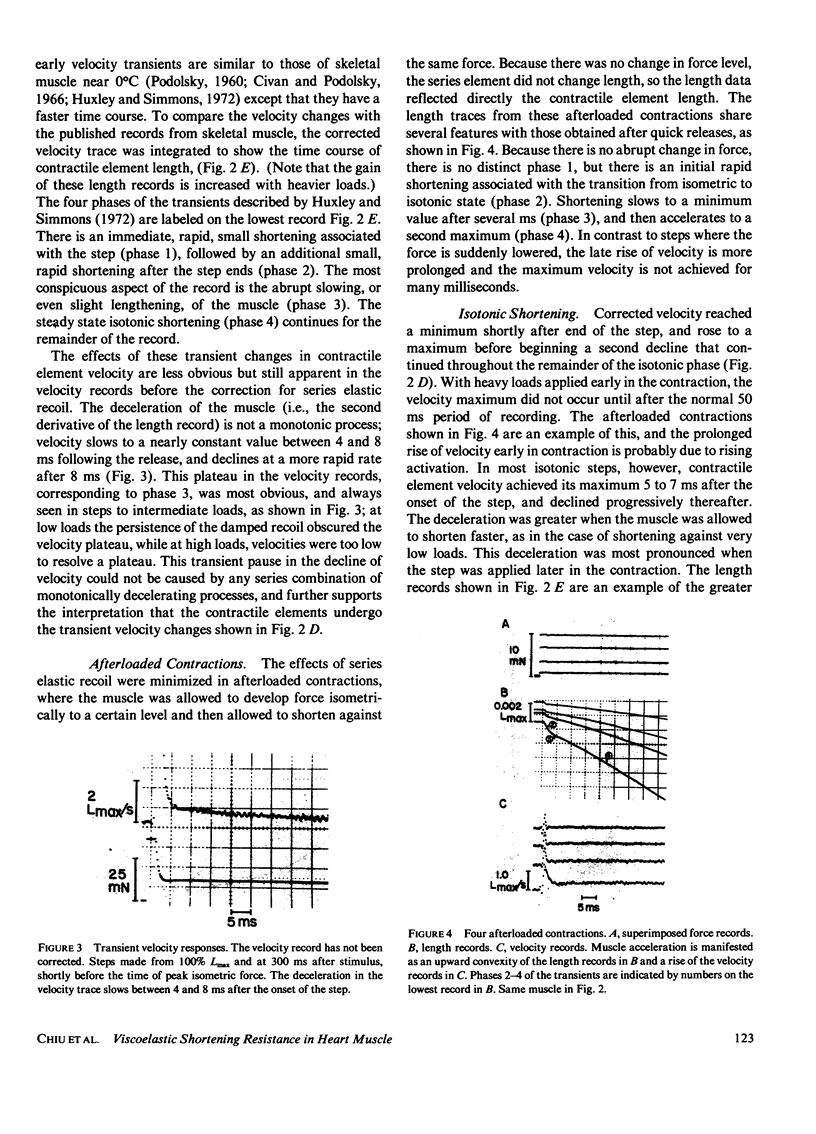
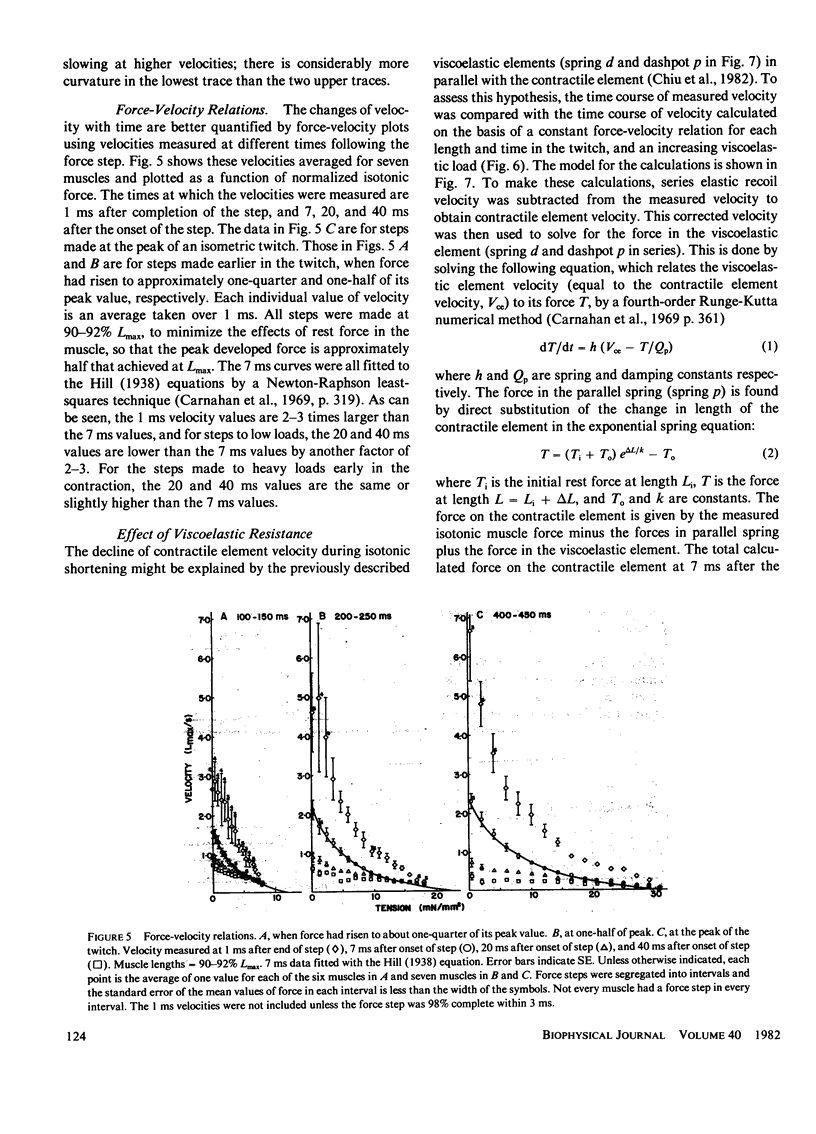
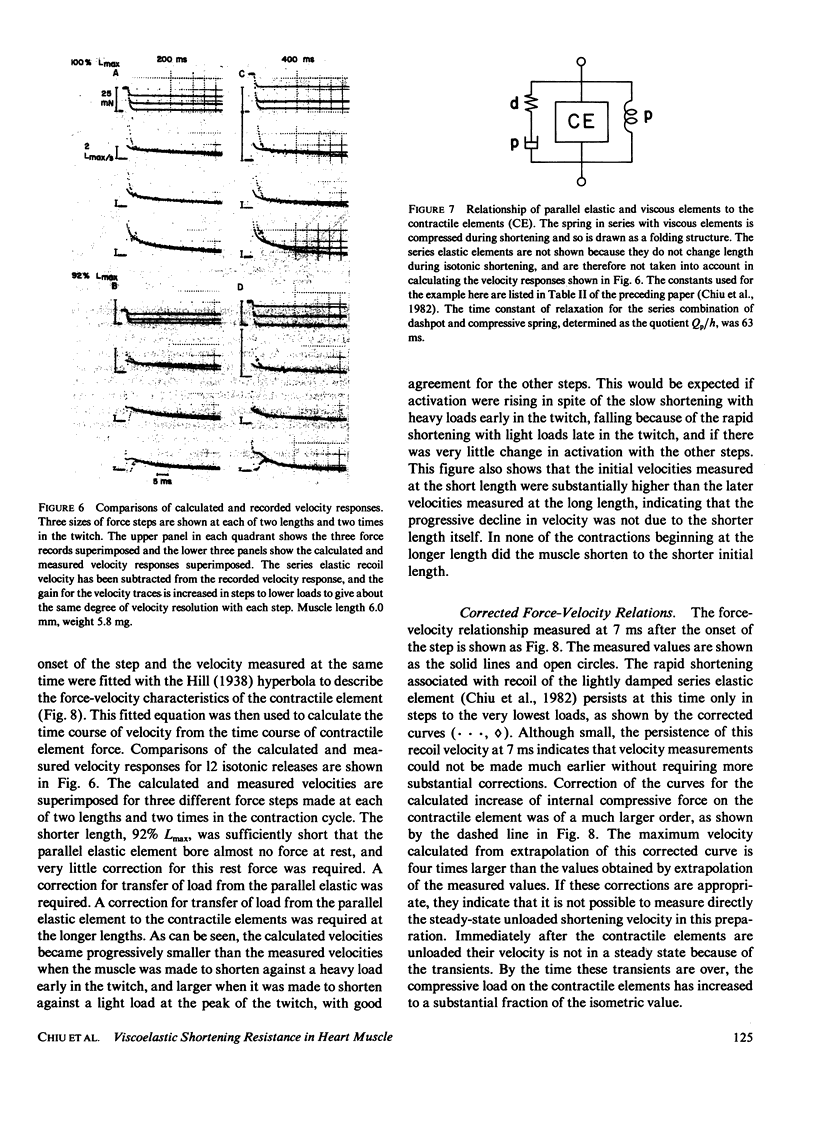
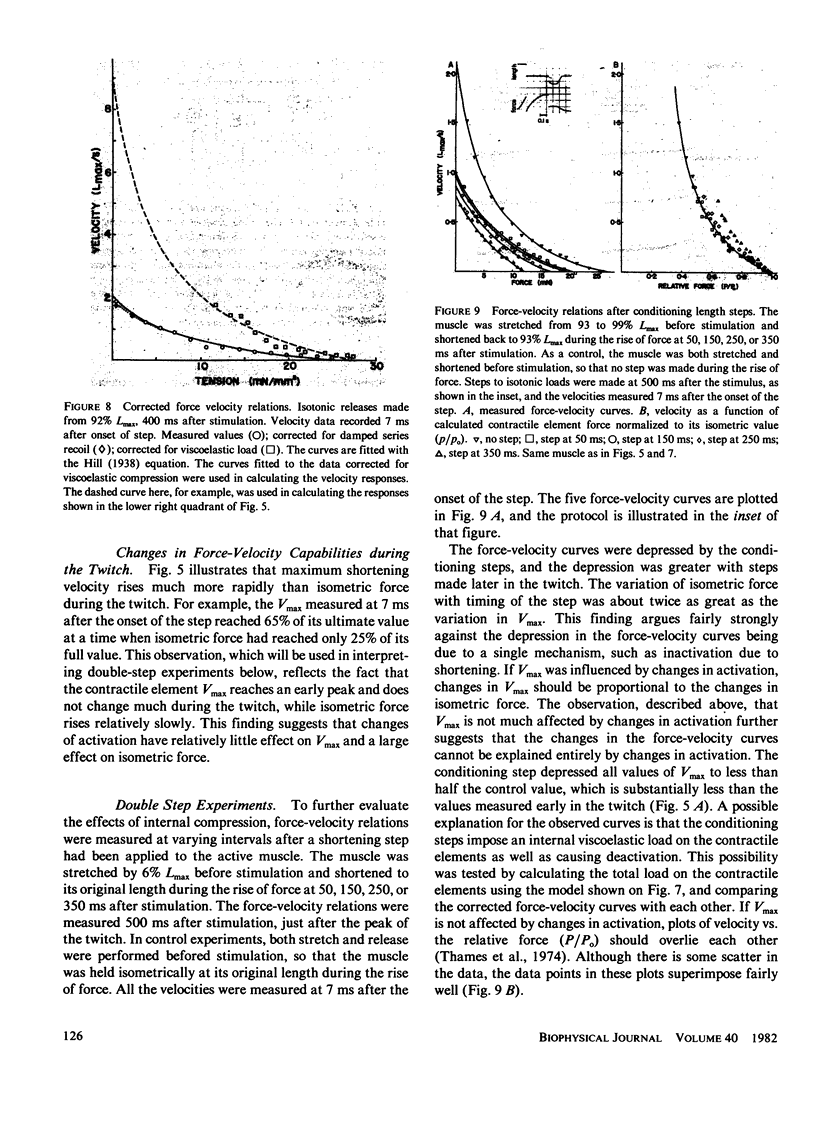
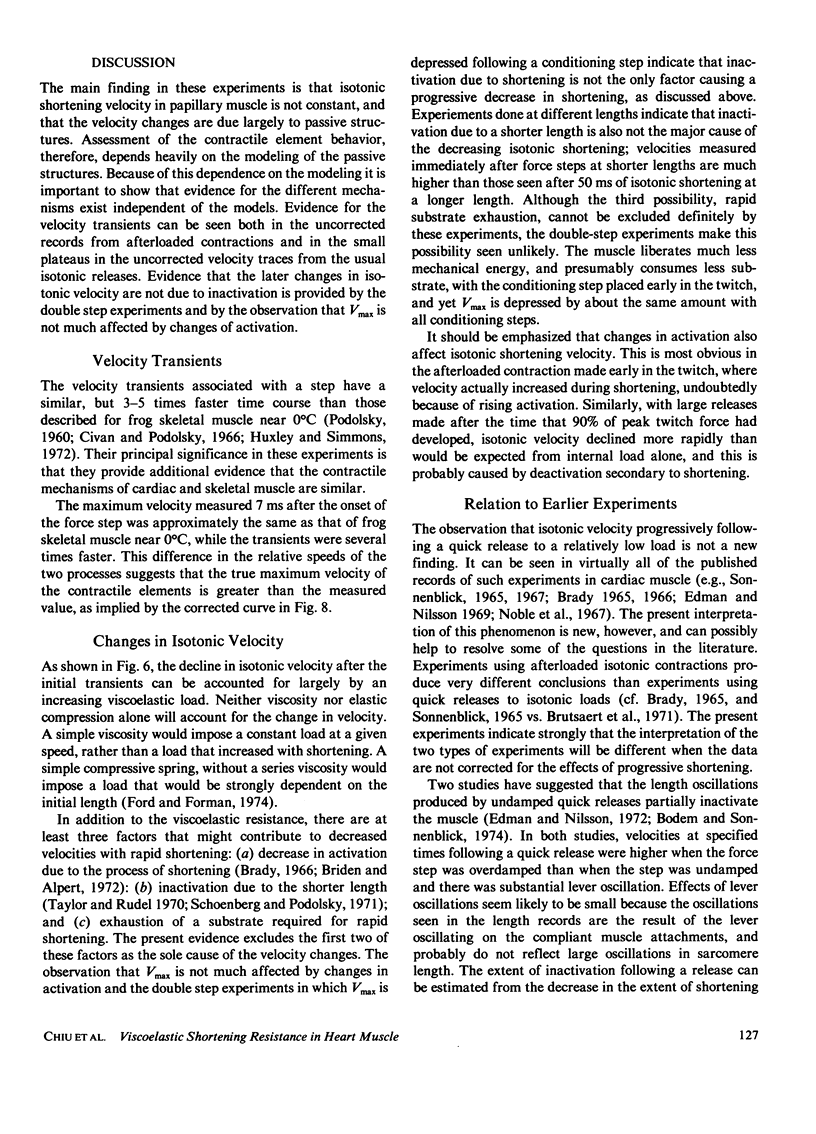
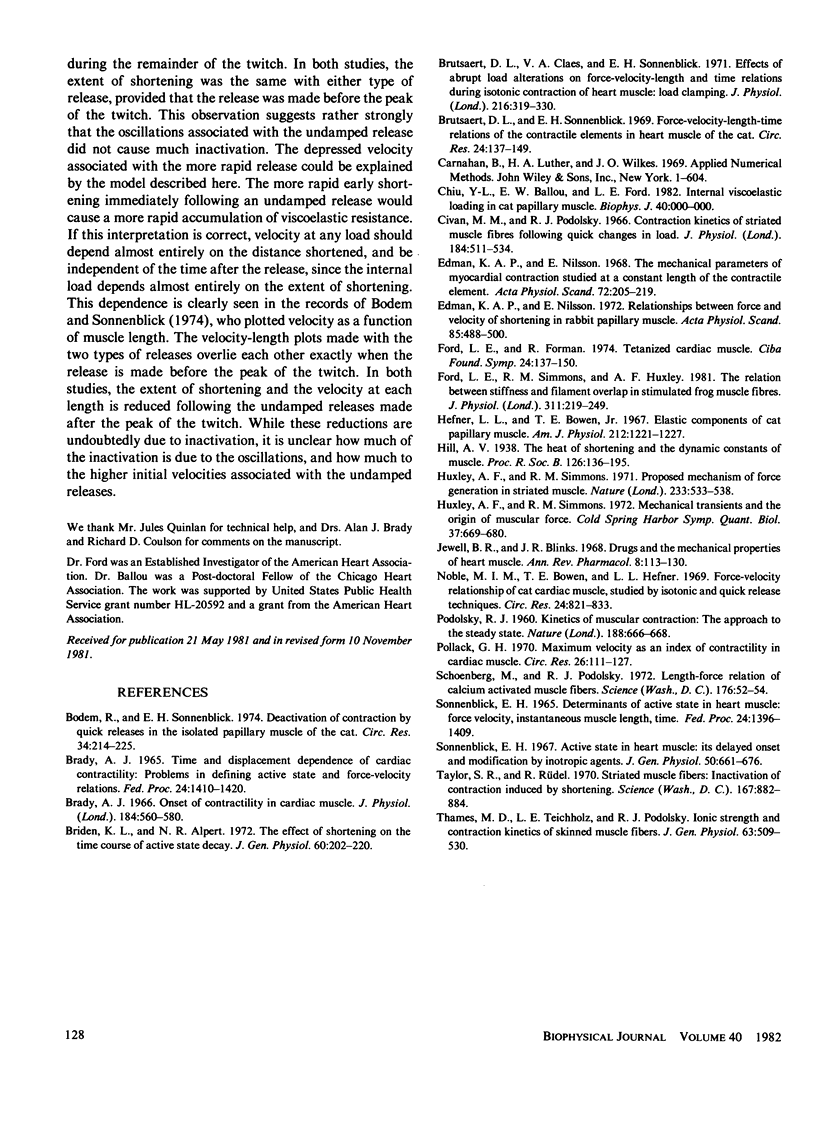
Selected References
These references are in PubMed. This may not be the complete list of references from this article.
- Bodem R., Sonnenblick E. H. Deactivation of contraction by quick releases in the isolated papillary muscle of the cat. Effects of lever damping, caffeine, and tetanization. Circ Res. 1974 Feb;34(2):214–225. doi: 10.1161/01.res.34.2.214. [DOI] [PubMed] [Google Scholar]
- Brady A. J. Onset of contractility in cardiac muscle. J Physiol. 1966 Jun;184(3):560–580. doi: 10.1113/jphysiol.1966.sp007931. [DOI] [PMC free article] [PubMed] [Google Scholar]
- Brady A. J. Time and displacement dependence of cardiac contractility: problems in defining the active state and force-velocity relations. Fed Proc. 1965 Nov-Dec;24(6):1410–1420. [PubMed] [Google Scholar]
- Briden K. L., Alpert N. R. The effect of shortening on the time-course of active state decay. J Gen Physiol. 1972 Aug;60(2):202–220. doi: 10.1085/jgp.60.2.202. [DOI] [PMC free article] [PubMed] [Google Scholar]
- Brutsaert D. L., Claes V. A., Sonnenblick E. H. Effects of abrupt load alterations on force-velocity-length and time relations during isotonic contractions of heart muscle: load clamping. J Physiol. 1971 Jul;216(2):319–330. doi: 10.1113/jphysiol.1971.sp009527. [DOI] [PMC free article] [PubMed] [Google Scholar]
- Brutsaert D. L., Sonnenblick E. H. Force-velocity-length-time relations of the contractile elements in heart muscle of the cat. Circ Res. 1969 Feb;24(2):137–149. doi: 10.1161/01.res.24.2.137. [DOI] [PubMed] [Google Scholar]
- Civan M. M., Podolsky R. J. Contraction kinetics of striated muscle fibres following quick changes in load. J Physiol. 1966 Jun;184(3):511–534. doi: 10.1113/jphysiol.1966.sp007929. [DOI] [PMC free article] [PubMed] [Google Scholar]
- Edman K. A., Nilsson E. Relationships between force and velocity of shortening in rabbit papillary muscle. Acta Physiol Scand. 1972 Aug;85(4):488–500. doi: 10.1111/j.1748-1716.1971.tb05286.x. [DOI] [PubMed] [Google Scholar]
- Edman K. A., Nilsson E. The mechanical parameters of myocardial contraction studied at a constant length of the contractile element. Acta Physiol Scand. 1968 Jan-Feb;72(1):205–219. doi: 10.1111/j.1748-1716.1968.tb03843.x. [DOI] [PubMed] [Google Scholar]
- Ford L. E., Huxley A. F., Simmons R. M. The relation between stiffness and filament overlap in stimulated frog muscle fibres. J Physiol. 1981 Feb;311:219–249. doi: 10.1113/jphysiol.1981.sp013582. [DOI] [PMC free article] [PubMed] [Google Scholar]
- Hefner L. L., Bowen T. E., Jr Elastic components of cat papillary muscle. Am J Physiol. 1967 May;212(5):1221–1227. doi: 10.1152/ajplegacy.1967.212.5.1221. [DOI] [PubMed] [Google Scholar]
- Huxley A. F., Simmons R. M. Proposed mechanism of force generation in striated muscle. Nature. 1971 Oct 22;233(5321):533–538. doi: 10.1038/233533a0. [DOI] [PubMed] [Google Scholar]
- Jewell B. R., Blinks J. R. Drugs and the mechanical properties of heart muscle. Annu Rev Pharmacol. 1968;8:113–130. doi: 10.1146/annurev.pa.08.040168.000553. [DOI] [PubMed] [Google Scholar]
- Noble M. I., Bowen T. E., Hefner L. L. Force-velocity relationship of cat cardiac muscle, studied by isotonic and quick-release techniques. Circ Res. 1969 Jun;24(6):821–833. doi: 10.1161/01.res.24.6.821. [DOI] [PubMed] [Google Scholar]
- PODOLSKY R. J. Kinetics of muscular contraction: the approach to the steady state. Nature. 1960 Nov 19;188:666–668. doi: 10.1038/188666a0. [DOI] [PubMed] [Google Scholar]
- Pollack G. H. Maximum velocity as an index of contractility in cardiac muscle. A critical evaluation. Circ Res. 1970 Jan;26(1):111–127. doi: 10.1161/01.res.26.1.111. [DOI] [PubMed] [Google Scholar]
- Schoenberg M., Podolsky R. J. Length-force relation of calcium activated muscle fibers. Science. 1972 Apr 7;176(4030):52–54. doi: 10.1126/science.176.4030.52. [DOI] [PubMed] [Google Scholar]
- Sonnenblick E. H. Active state in heart muscle. Its delayed onset and modification by inotropic agents. J Gen Physiol. 1967 Jan;50(3):661–676. doi: 10.1085/jgp.50.3.661. [DOI] [PMC free article] [PubMed] [Google Scholar]
- Sonnenblick E. H. Determinants of active state in heart muscle: force, velocity, instantaneous muscle length, time. Fed Proc. 1965 Nov-Dec;24(6):1396–1409. [PubMed] [Google Scholar]