Abstract
To assess the influence of intracellular hemoglobin concentration on red cell viscoelasticity and to better understand changes related to in vivo aging, membrane shear elastic moduli (mu) and time constants for cell shape recovery (tc) were measured for age-fractionated human erythrocytes and derived ghosts. Time constants were also measured for osmotically shrunk cell fractions. Young and old cells had equal mu, but tc was longer for older cells. When young cells were shrunk to equal the volume (and hence hemoglobin concentration and internal viscosity) of old cells, tc increased only slightly. Thus membrane viscosity (eta = mu . tc) increases during aging, regardless of increased internal viscosity. However, further shrinkage of young cells, or slight shrinkage of old cells, caused a sharp increase in tc. Because this increased tc is not explainable by elevated internal viscosity, eta increased, possibly due to a concentration-dependent hemoglobin-membrane interaction. Ghosts had a greater mu than intact cells, with proportionally faster tc; their membrane viscosity was therefore similar to intact cells. However, the ratio of old/young membrane viscosity was less for ghosts than for intact cells, indicating that differences between young and old cell eta may be partly explained by altered hemoglobin-membrane interaction during aging. It is postulated that these changes in viscoelastic behavior influence in vivo survival of senescent cells.
Full text
PDF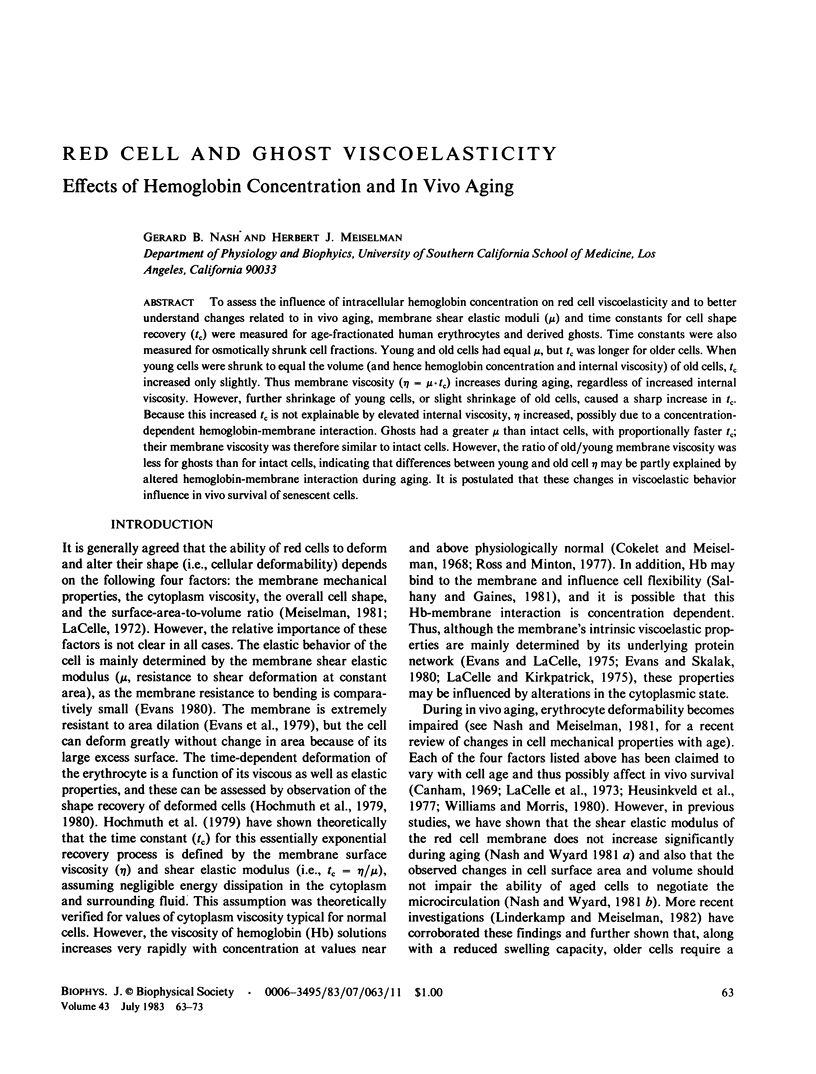
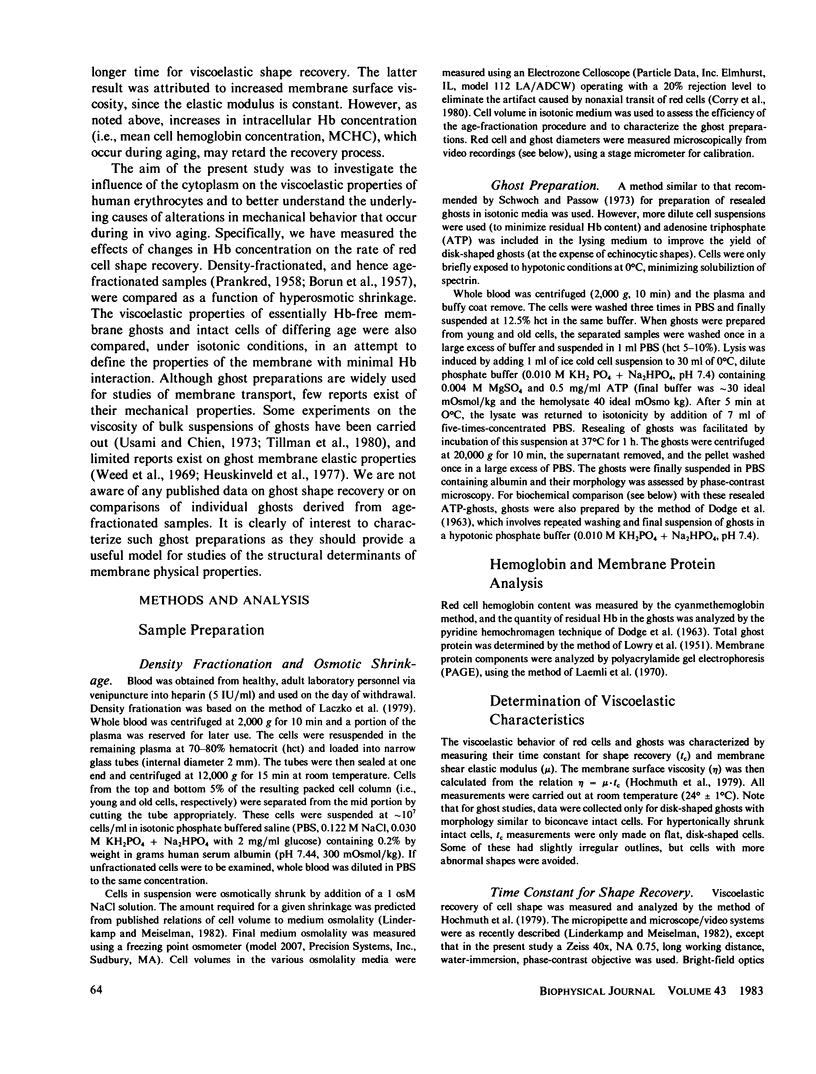
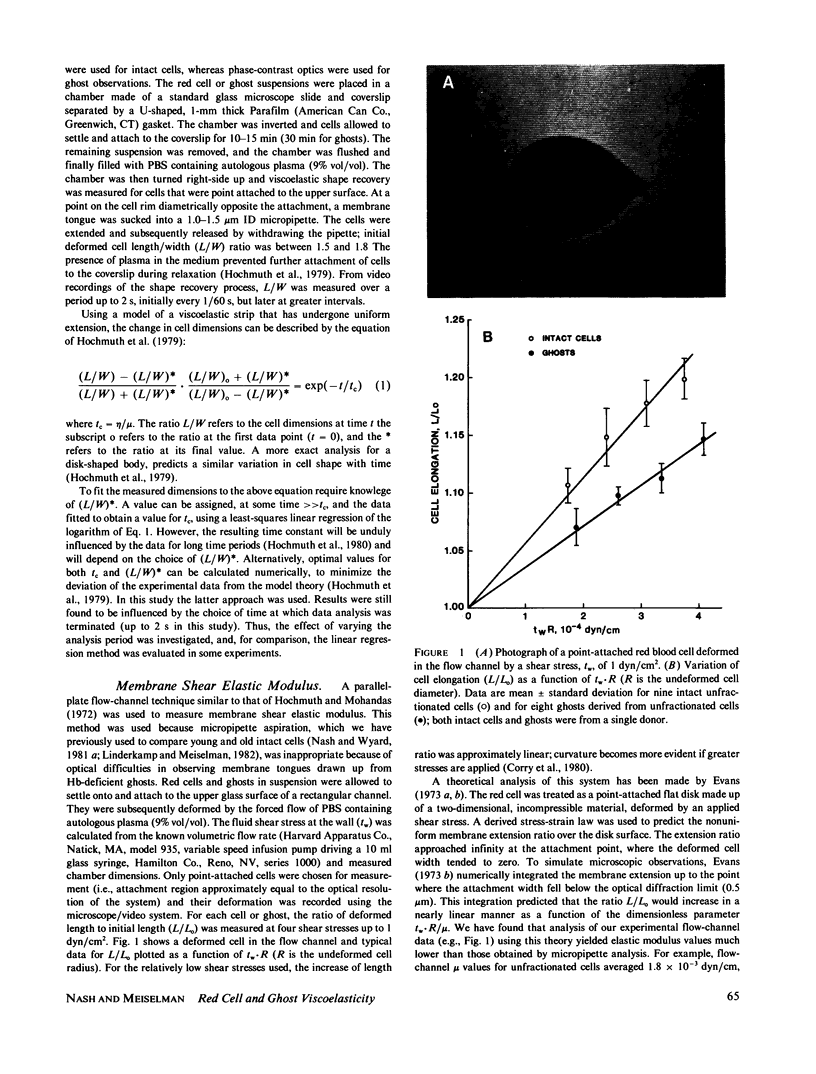
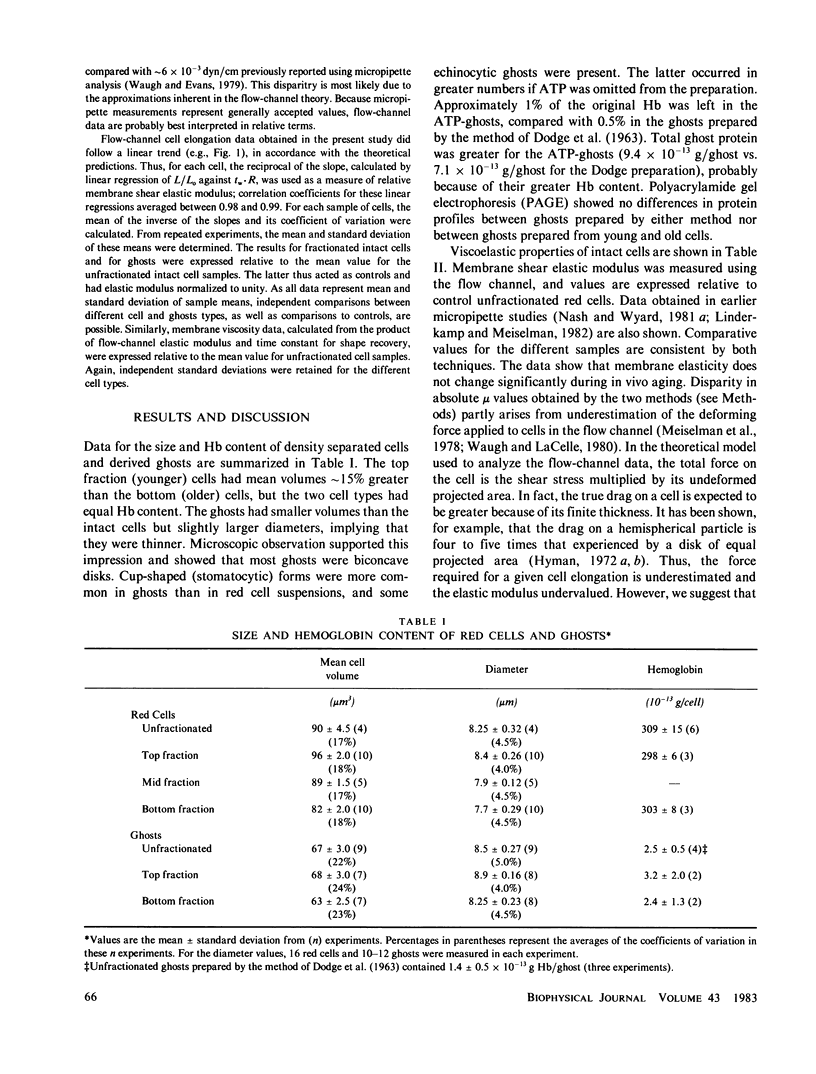
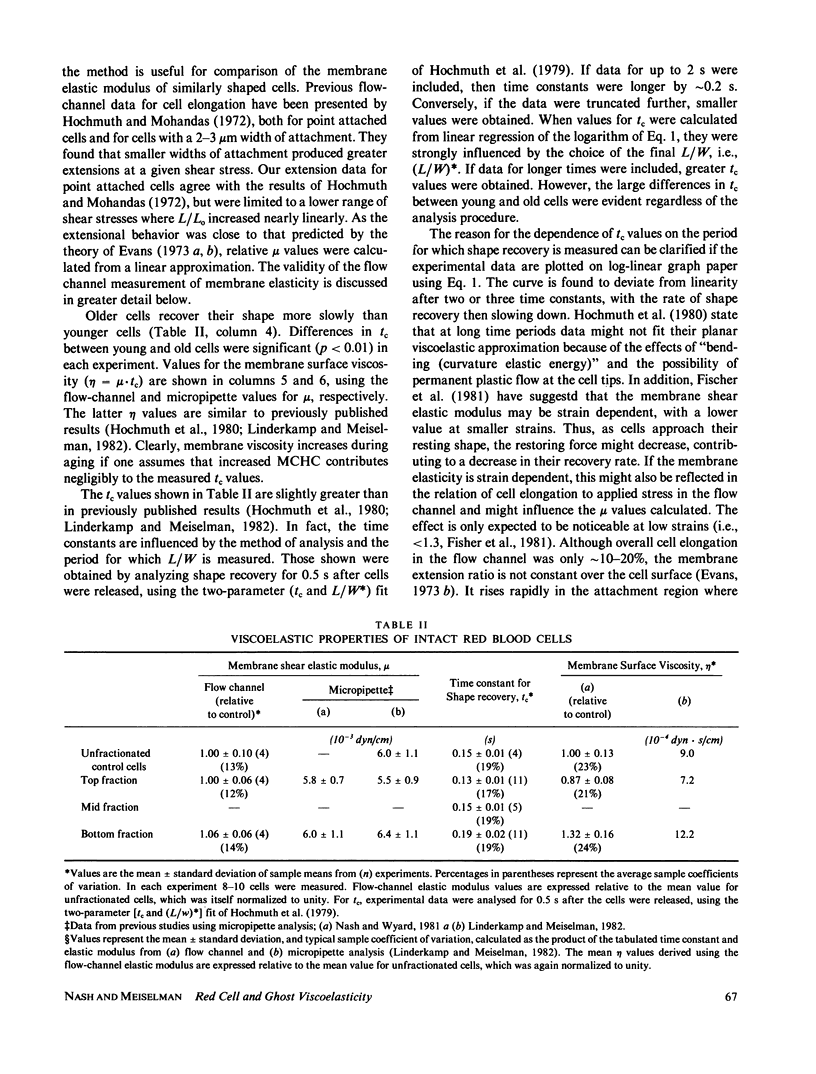
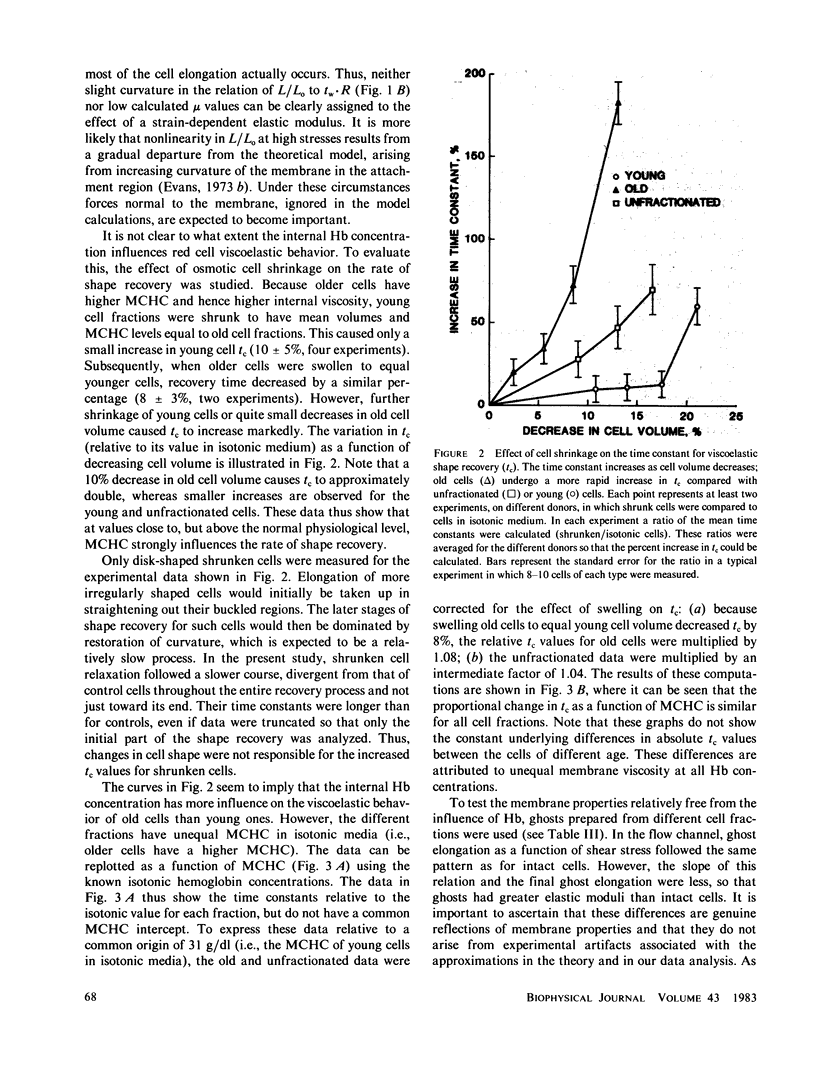
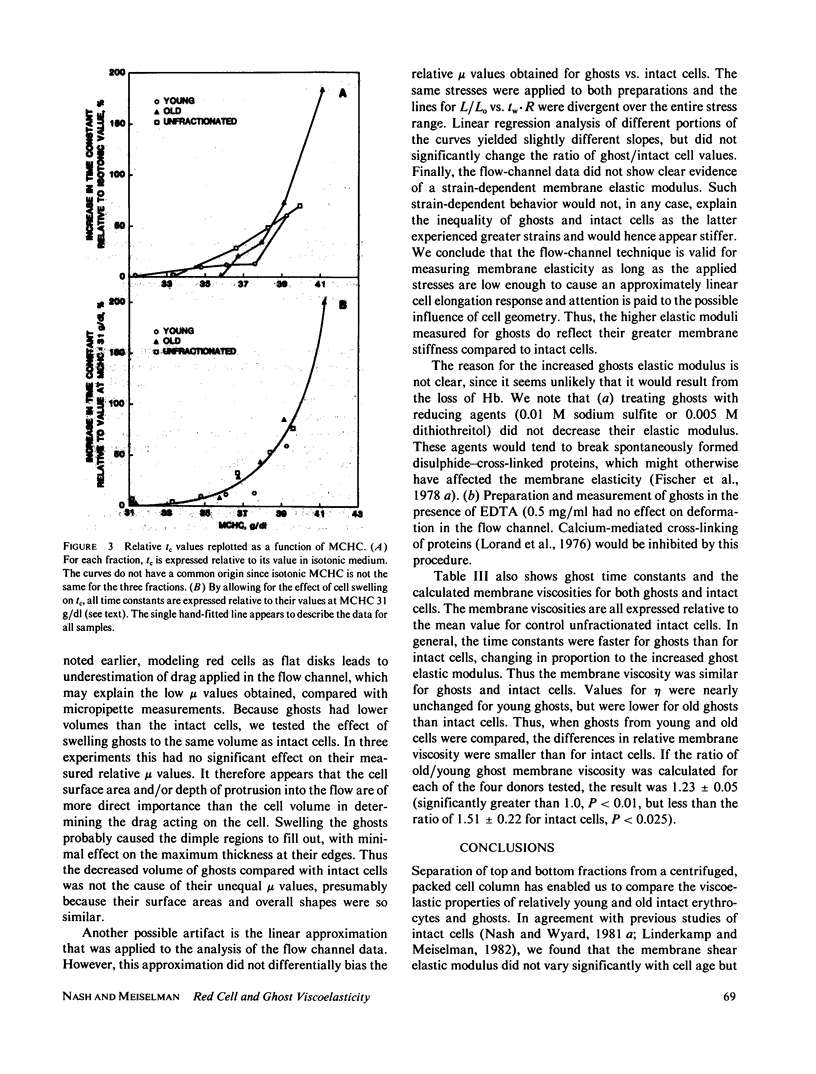
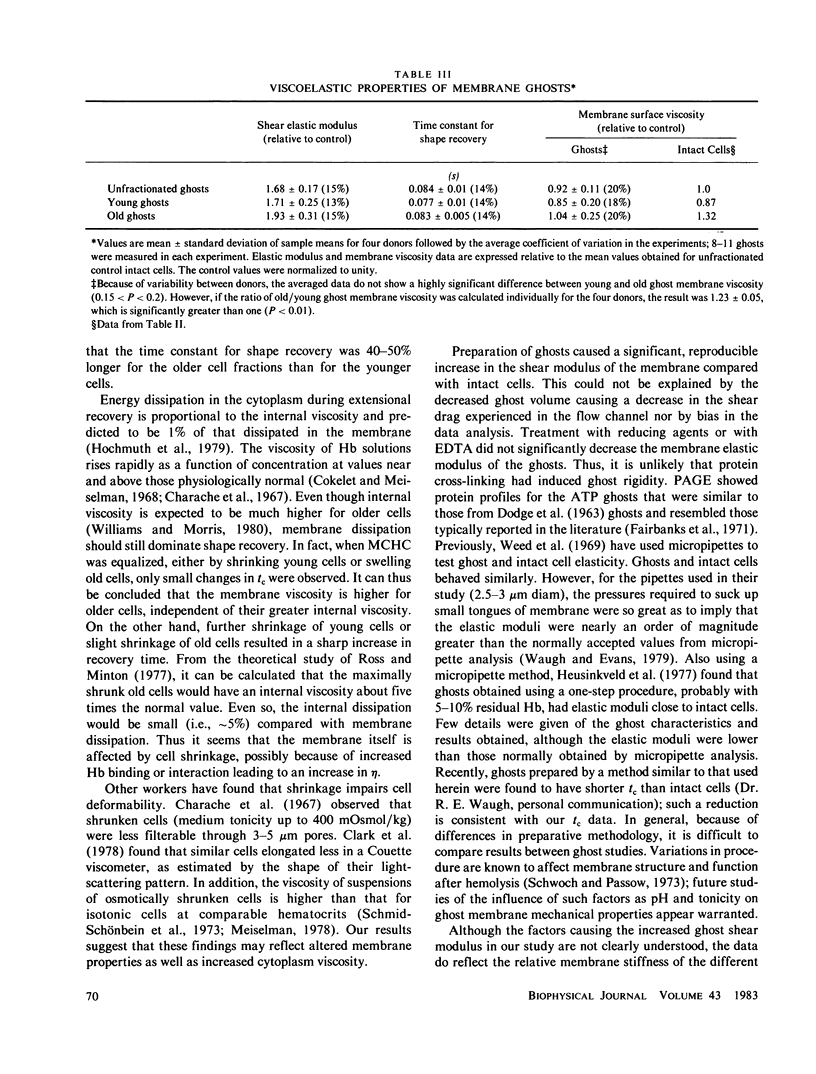
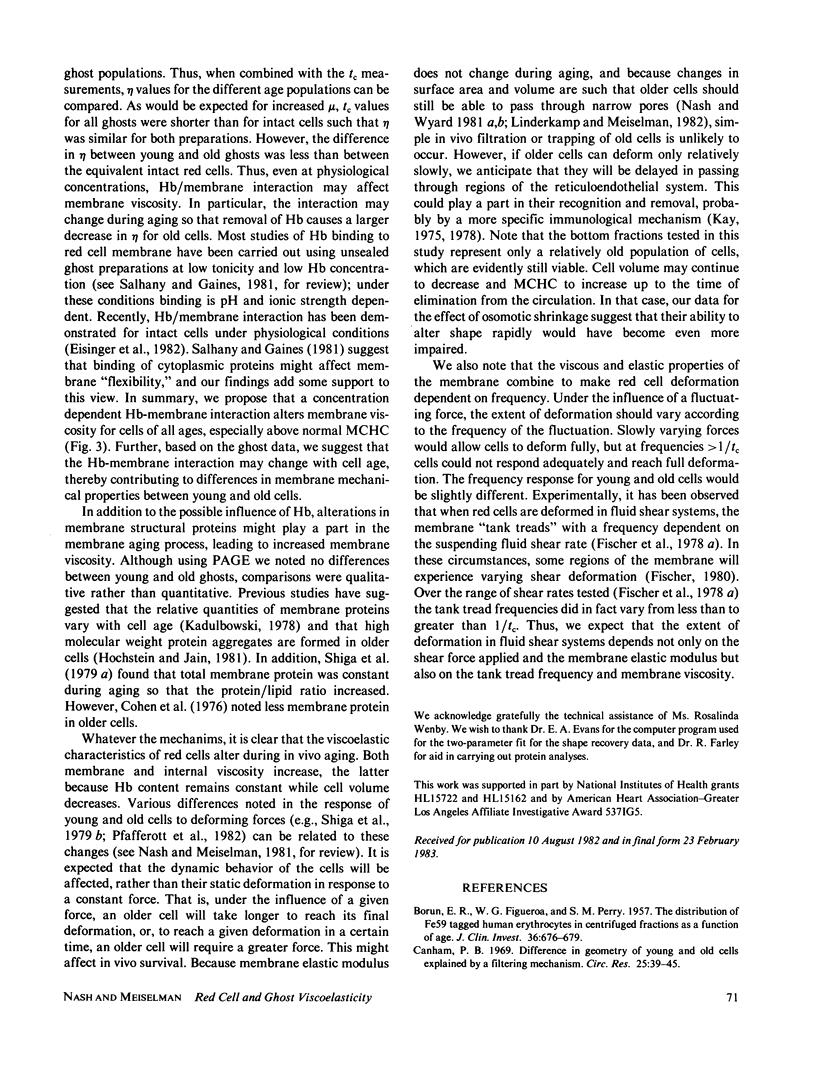
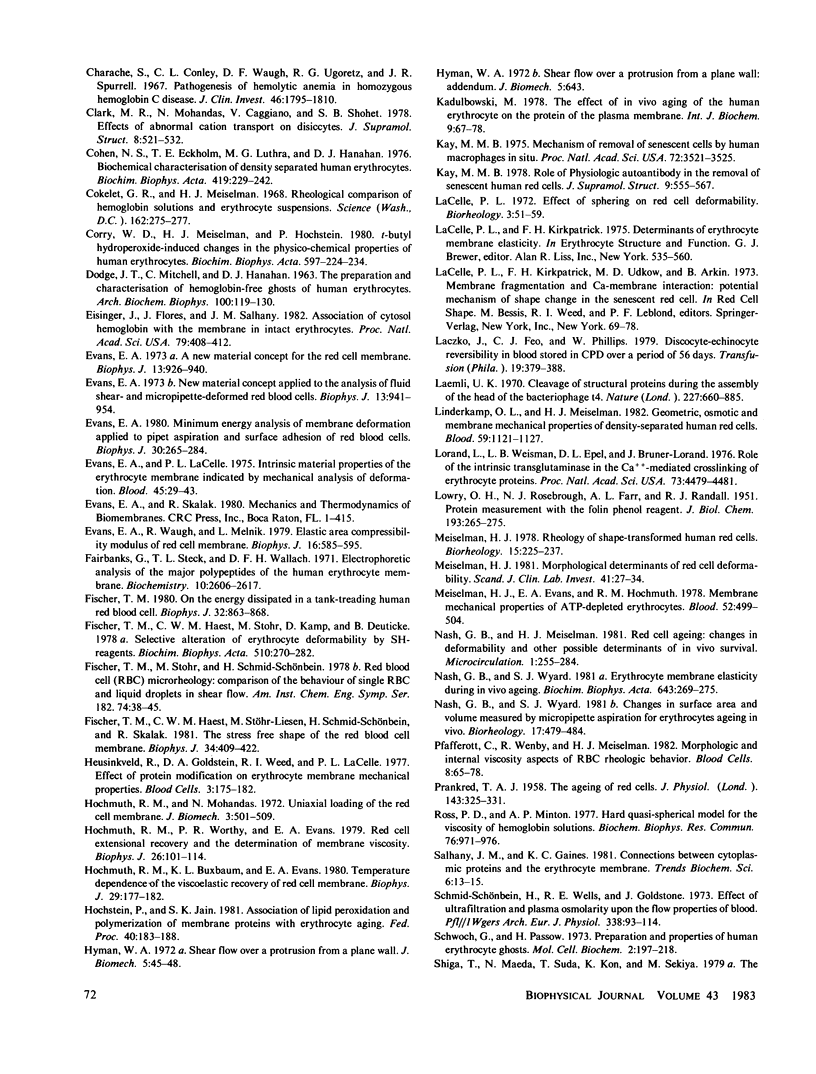
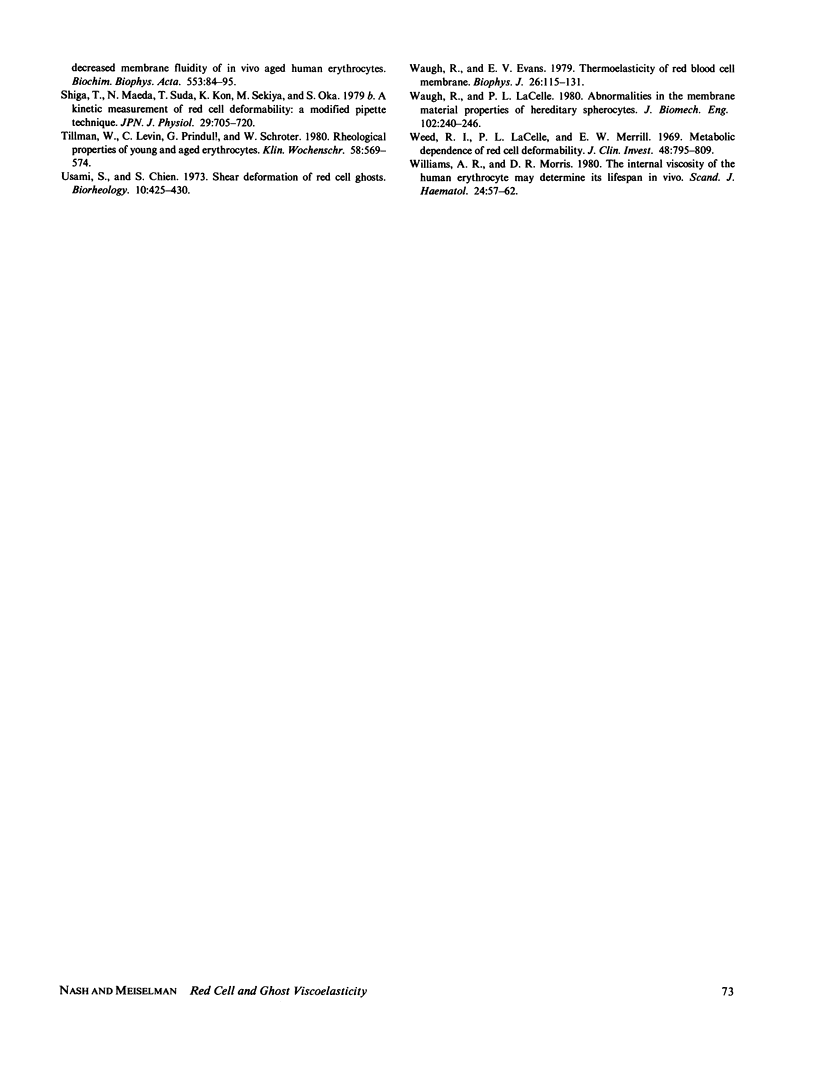
Images in this article
Selected References
These references are in PubMed. This may not be the complete list of references from this article.
- BORUN E. R., FIGUEROA W. G., PERRY S. M. The distribution of Fe59 tagged human erythrocytes in centrifuged specimens as a function of cell age. J Clin Invest. 1957 May;36(5):676–679. doi: 10.1172/JCI103468. [DOI] [PMC free article] [PubMed] [Google Scholar]
- Canham P. B. Difference in geometry of young and old human erythrocytes explained by a filtering mechanism. Circ Res. 1969 Jul;25(1):39–45. doi: 10.1161/01.res.25.1.39. [DOI] [PubMed] [Google Scholar]
- Charache S., Conley C. L., Waugh D. F., Ugoretz R. J., Spurrell J. R. Pathogenesis of hemolytic anemia in homozygous hemoglobin C disease. J Clin Invest. 1967 Nov;46(11):1795–1811. doi: 10.1172/JCI105670. [DOI] [PMC free article] [PubMed] [Google Scholar]
- Clark M. R., Mohandas N., Caggiano V., Shohet S. B. Effects of abnormal cation transport on deformability of desiccytes. J Supramol Struct. 1978;8(4):521–532. doi: 10.1002/jss.400080414. [DOI] [PubMed] [Google Scholar]
- Cohen N. S., Ekholm J. E., Luthra M. G., Hanahan D. J. Biochemical characterization of density-separated human erythrocytes. Biochim Biophys Acta. 1976 Jan 21;419(2):229–242. doi: 10.1016/0005-2736(76)90349-7. [DOI] [PubMed] [Google Scholar]
- Cokelet G. R., Meiselman H. J. Rheological comparison of hemoglobin solutions and erythrocyte suspensions. Science. 1968 Oct 11;162(3850):275–277. doi: 10.1126/science.162.3850.275. [DOI] [PubMed] [Google Scholar]
- Corry W. D., Meiselman H. J., Hochstein P. t-Butyl hydroperoxide-induced changes in the physicochemical properties of human erythrocytes. Biochim Biophys Acta. 1980 Apr 10;597(2):224–234. doi: 10.1016/0005-2736(80)90101-7. [DOI] [PubMed] [Google Scholar]
- DODGE J. T., MITCHELL C., HANAHAN D. J. The preparation and chemical characteristics of hemoglobin-free ghosts of human erythrocytes. Arch Biochem Biophys. 1963 Jan;100:119–130. doi: 10.1016/0003-9861(63)90042-0. [DOI] [PubMed] [Google Scholar]
- Eisinger J., Flores J., Salhany J. M. Association of cytosol hemoglobin with the membrane in intact erythrocytes. Proc Natl Acad Sci U S A. 1982 Jan;79(2):408–412. doi: 10.1073/pnas.79.2.408. [DOI] [PMC free article] [PubMed] [Google Scholar]
- Evans E. A. A new material concept for the red cell membrane. Biophys J. 1973 Sep;13(9):926–940. doi: 10.1016/S0006-3495(73)86035-7. [DOI] [PMC free article] [PubMed] [Google Scholar]
- Evans E. A., La Celle P. L. Intrinsic material properties of the erythrocyte membrane indicated by mechanical analysis of deformation. Blood. 1975 Jan;45(1):29–43. [PubMed] [Google Scholar]
- Evans E. A. Minimum energy analysis of membrane deformation applied to pipet aspiration and surface adhesion of red blood cells. Biophys J. 1980 May;30(2):265–284. doi: 10.1016/S0006-3495(80)85093-4. [DOI] [PMC free article] [PubMed] [Google Scholar]
- Evans E. A. New membrane concept applied to the analysis of fluid shear- and micropipette-deformed red blood cells. Biophys J. 1973 Sep;13(9):941–954. doi: 10.1016/S0006-3495(73)86036-9. [DOI] [PMC free article] [PubMed] [Google Scholar]
- Evans E. A., Waugh R., Melnik L. Elastic area compressibility modulus of red cell membrane. Biophys J. 1976 Jun;16(6):585–595. doi: 10.1016/S0006-3495(76)85713-X. [DOI] [PMC free article] [PubMed] [Google Scholar]
- Fairbanks G., Steck T. L., Wallach D. F. Electrophoretic analysis of the major polypeptides of the human erythrocyte membrane. Biochemistry. 1971 Jun 22;10(13):2606–2617. doi: 10.1021/bi00789a030. [DOI] [PubMed] [Google Scholar]
- Fischer T. M., Haest C. W., Stöhr-Liesen M., Schmid-Schönbein H., Skalak R. The stress-free shape of the red blood cell membrane. Biophys J. 1981 Jun;34(3):409–422. doi: 10.1016/S0006-3495(81)84859-X. [DOI] [PMC free article] [PubMed] [Google Scholar]
- Fischer T. M., Haest C. W., Stöhr M., Kamp D., Deuticke B. Selective alteration of erythrocyte deformabiliby by SH-reagents: evidence for an involvement of spectrin in membrane shear elasticity. Biochim Biophys Acta. 1978 Jul 4;510(2):270–282. doi: 10.1016/0005-2736(78)90027-5. [DOI] [PubMed] [Google Scholar]
- Fischer T. M. On the energy dissipation in a tank-treading human red blood cell. Biophys J. 1980 Nov;32(2):863–868. doi: 10.1016/S0006-3495(80)85022-3. [DOI] [PMC free article] [PubMed] [Google Scholar]
- Hochmuth R. M., Buxbaum K. L., Evans E. A. Temperature dependence of the viscoelastic recovery of red cell membrane. Biophys J. 1980 Jan;29(1):177–182. doi: 10.1016/S0006-3495(80)85124-1. [DOI] [PMC free article] [PubMed] [Google Scholar]
- Hochmuth R. M., Mohandas N. Uniaxial loading of the red-cell membrane. J Biomech. 1972 Sep;5(5):501–509. doi: 10.1016/0021-9290(72)90007-3. [DOI] [PubMed] [Google Scholar]
- Hochmuth R. M., Worthy P. R., Evans E. A. Red cell extensional recovery and the determination of membrane viscosity. Biophys J. 1979 Apr;26(1):101–114. doi: 10.1016/S0006-3495(79)85238-8. [DOI] [PMC free article] [PubMed] [Google Scholar]
- Hochstein P., Jain S. K. Association of lipid peroxidation and polymerization of membrane proteins with erythrocyte aging. Fed Proc. 1981 Feb;40(2):183–188. [PubMed] [Google Scholar]
- Hyman W. A. Shear flow over a protrusion from a plane wall. J Biomech. 1972 Jan;5(1):45–48. doi: 10.1016/0021-9290(72)90018-8. [DOI] [PubMed] [Google Scholar]
- Hyman W. A. Shear flow over a protrusion from a plane wall: addendum. J Biomech. 1972 Nov;5(6):643–643. doi: 10.1016/0021-9290(72)90036-x. [DOI] [PubMed] [Google Scholar]
- Kadlubowski M. The effect of in-vivo ageing of the human erythrocyte on the protein of the plasma membrane. A characterisation. Int J Biochem. 1978;9(2):67–78. doi: 10.1016/0020-711x(78)90014-9. [DOI] [PubMed] [Google Scholar]
- Kay M. M. Mechanism of removal of senescent cells by human macrophages in situ. Proc Natl Acad Sci U S A. 1975 Sep;72(9):3521–3525. doi: 10.1073/pnas.72.9.3521. [DOI] [PMC free article] [PubMed] [Google Scholar]
- Kay M. M. Role of physiologic autoantibody in the removal of senescent human red cells. J Supramol Struct. 1978;9(4):555–567. doi: 10.1002/jss.400090409. [DOI] [PubMed] [Google Scholar]
- LOWRY O. H., ROSEBROUGH N. J., FARR A. L., RANDALL R. J. Protein measurement with the Folin phenol reagent. J Biol Chem. 1951 Nov;193(1):265–275. [PubMed] [Google Scholar]
- LaCelle P. L. Effect of sphering on erythrocyte deformability. Biorheology. 1972 Jun;9(2):51–59. doi: 10.3233/bir-1972-9202. [DOI] [PubMed] [Google Scholar]
- Laczkó J., Feó C. J., Phillips W. Discocyte--echinocyte reversibility in blood stored in CPD over a period of 56 days. Transfusion. 1979 Jul-Aug;19(4):379–388. doi: 10.1046/j.1537-2995.1979.19479250174.x. [DOI] [PubMed] [Google Scholar]
- Linderkamp O., Meiselman H. J. Geometric, osmotic, and membrane mechanical properties of density-separated human red cells. Blood. 1982 Jun;59(6):1121–1127. [PubMed] [Google Scholar]
- Lorand L., Weissmann L. B., Epel D. L., Bruner-Lorand J. Role of the intrinsic transglutaminase in the Ca2+-mediated crosslinking of erythrocyte proteins. Proc Natl Acad Sci U S A. 1976 Dec;73(12):4479–4481. doi: 10.1073/pnas.73.12.4479. [DOI] [PMC free article] [PubMed] [Google Scholar]
- Meiselman H. J., Evans E. A., Hochmuth R. M. Membrane mechanical properties of ATP-depleted human erythrocytes. Blood. 1978 Sep;52(3):499–504. [PubMed] [Google Scholar]
- Meiselman H. J. Morphological determinants of red cell deformability. Scand J Clin Lab Invest Suppl. 1981;156:27–34. doi: 10.3109/00365518109097426. [DOI] [PubMed] [Google Scholar]
- Meiselman H. J. Rheology of shape-transformed human red cells. Biorheology. 1978;15(3-4):225–237. doi: 10.3233/bir-1978-153-410. [DOI] [PubMed] [Google Scholar]
- Nash G. B., Wyard S. J. Changes in surface area and volume measured by micropipette aspiration for erythrocytes ageing in vivo. Biorheology. 1980;17(5-6):479–484. [PubMed] [Google Scholar]
- Nash G. B., Wyard S. J. Erythrocyte membrane elasticity during in vivo ageing. Biochim Biophys Acta. 1981 May 6;643(2):269–275. doi: 10.1016/0005-2736(81)90072-9. [DOI] [PubMed] [Google Scholar]
- Pfafferott C., Wenby R., Meiselman H. J. Morphologic and internal viscosity aspects of RBC rheologic behavior. Blood Cells. 1982;8(1):65–78. [PubMed] [Google Scholar]
- Ross P. D., Minton A. P. Hard quasispherical model for the viscosity of hemoglobin solutions. Biochem Biophys Res Commun. 1977 Jun 20;76(4):971–976. doi: 10.1016/0006-291x(77)90950-0. [DOI] [PubMed] [Google Scholar]
- Schmid-Schönbein H., Wells R. E., Goldstone J. Effect of ultrafiltration and plasma osmolarity upon the flow properties of blood: A possible mechanism for control of blood flow in the renal medullary vasa recta. Pflugers Arch. 1973 Jan 22;338(2):93–144. doi: 10.1007/BF00592746. [DOI] [PubMed] [Google Scholar]
- Schwoch G., Passow H. Preparation and properties of human erythrocyte ghosts. Mol Cell Biochem. 1973 Dec 15;2(2):197–218. doi: 10.1007/BF01795474. [DOI] [PubMed] [Google Scholar]
- Tillmann W., Levin C., Prindull G., Schröter W. Rheological properties of young and aged human erythrocytes. Klin Wochenschr. 1980 Jun 2;58(11):569–574. doi: 10.1007/BF01477168. [DOI] [PubMed] [Google Scholar]
- Usami S., Chien S. Shear deformation of red cell ghosts. Biorheology. 1973 Sep;10(3):425–430. doi: 10.3233/bir-1973-10316. [DOI] [PubMed] [Google Scholar]
- Waugh R., Evans E. A. Thermoelasticity of red blood cell membrane. Biophys J. 1979 Apr;26(1):115–131. doi: 10.1016/S0006-3495(79)85239-X. [DOI] [PMC free article] [PubMed] [Google Scholar]
- Weed R. I., LaCelle P. L., Merrill E. W. Metabolic dependence of red cell deformability. J Clin Invest. 1969 May;48(5):795–809. doi: 10.1172/JCI106038. [DOI] [PMC free article] [PubMed] [Google Scholar]
- Williams A. R., Morris D. R. The internal viscosity of the human erythrocyte may determine its lifespan in vivo. Scand J Haematol. 1980 Jan;24(1):57–62. doi: 10.1111/j.1600-0609.1980.tb01318.x. [DOI] [PubMed] [Google Scholar]