Abstract
To obtain the open or closed time interval distributions of patch clamp signals, several workers have used a half-amplitude minimum time interval criterion. Within this framework, no transition between states of different conductance levels is considered to have taken place if it leads to a time interval smaller than a certain critical value. This procedure modifies substantially the open or closed time interval distribution of the random signal to be analyzed, since time intervals well above the time resolution of the recording system may be interrupted by short gaps that may or may not satisfy the minimum time interval criterion. We present here a general theoretical framework by means of which the effect of time interval omission on time interval distributions can be taken into account. Based on the mathematical formalism provided by the Kolmogorov forward equation, special matrix operators are first defined. The general solution to the time omission problem in its integral form is then derived. In view of the poor computational feasibility of the resulting solution, a first-order approximation is also presented. This approximation consists essentially in neglecting the contribution of the undetected gaps to the total length of the resulting time interval. The exact and approximate solutions are then applied to two special kinetic schemes commonly found in single-channel studies, namely the O-C and C-O-C models. The applicability of the proposed formalism to the time interval distribution problem of a damped random signal is finally discussed.
Full text
PDF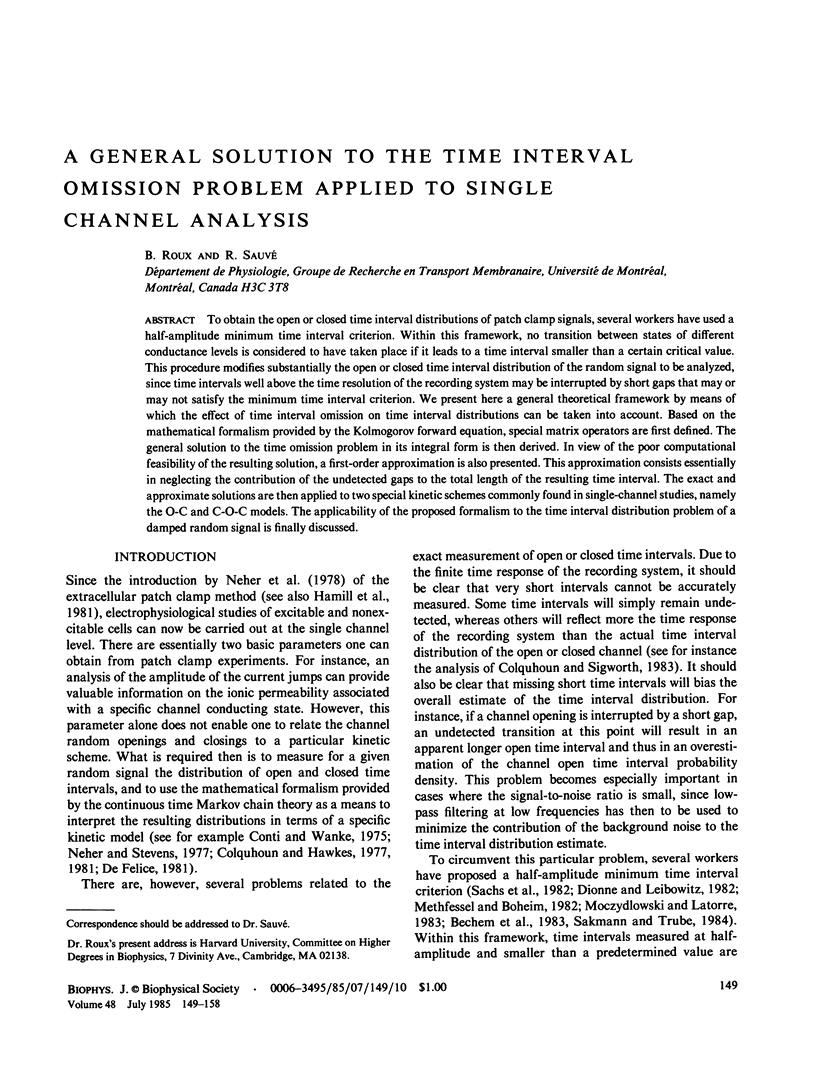
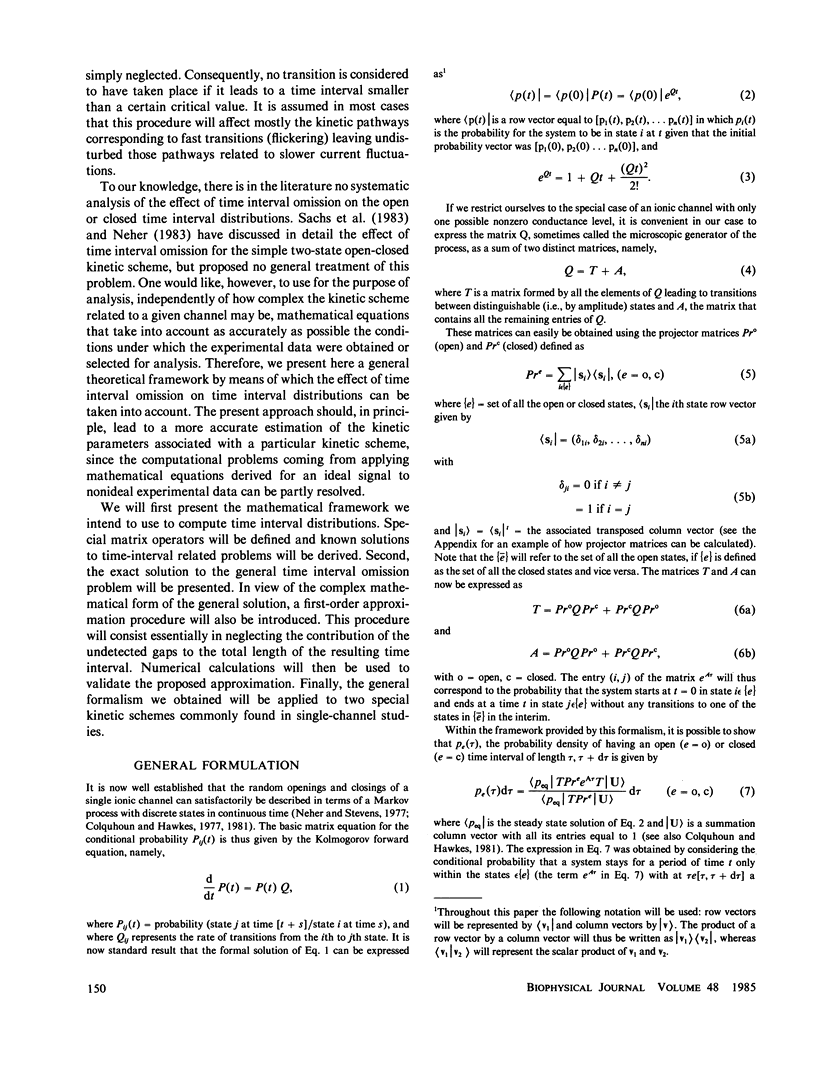
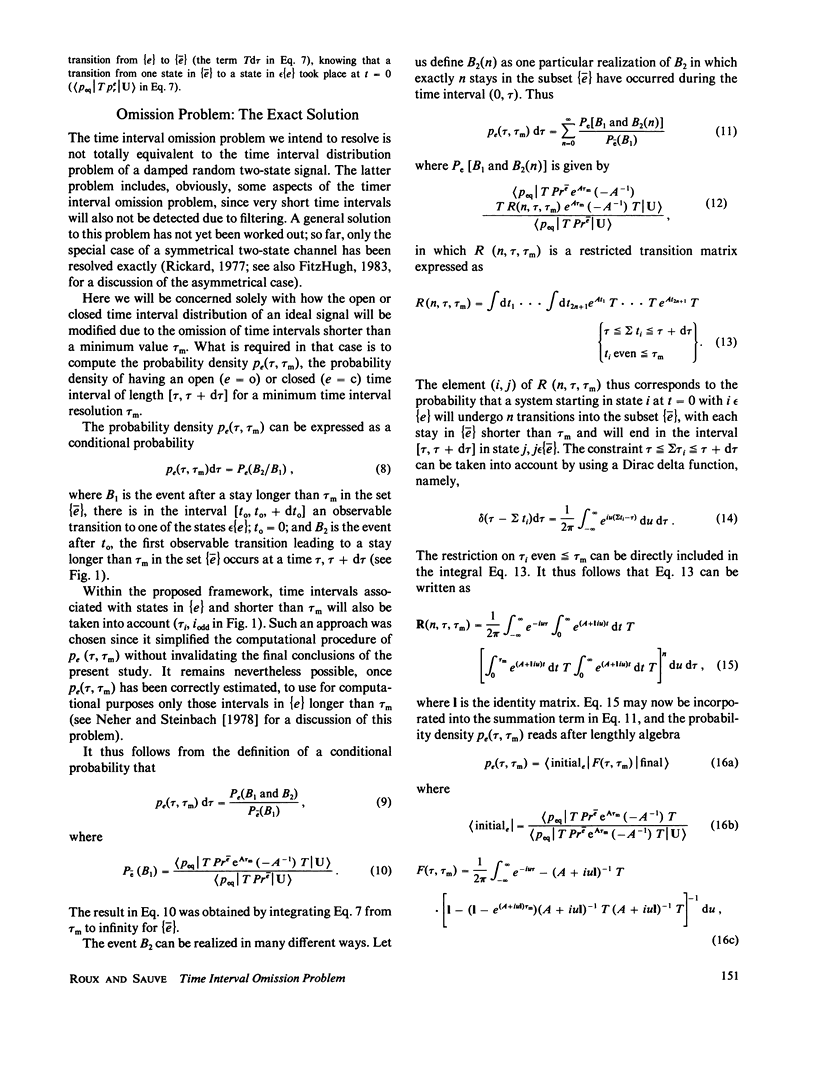
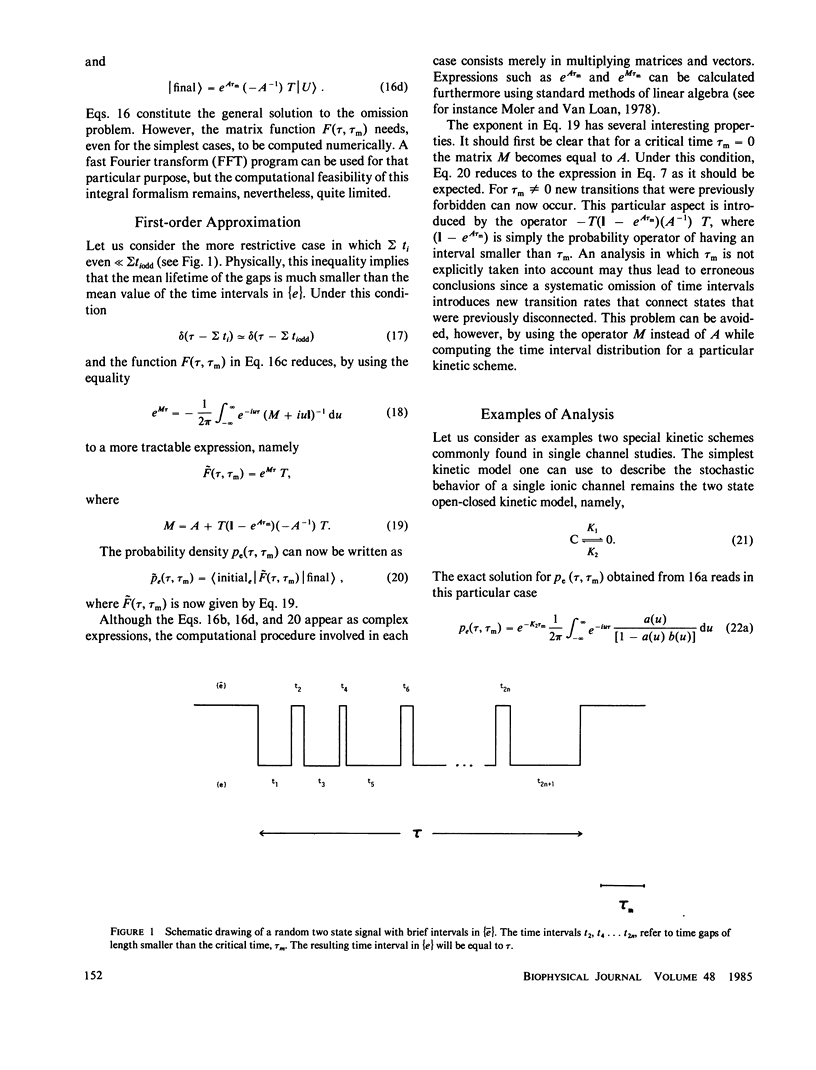
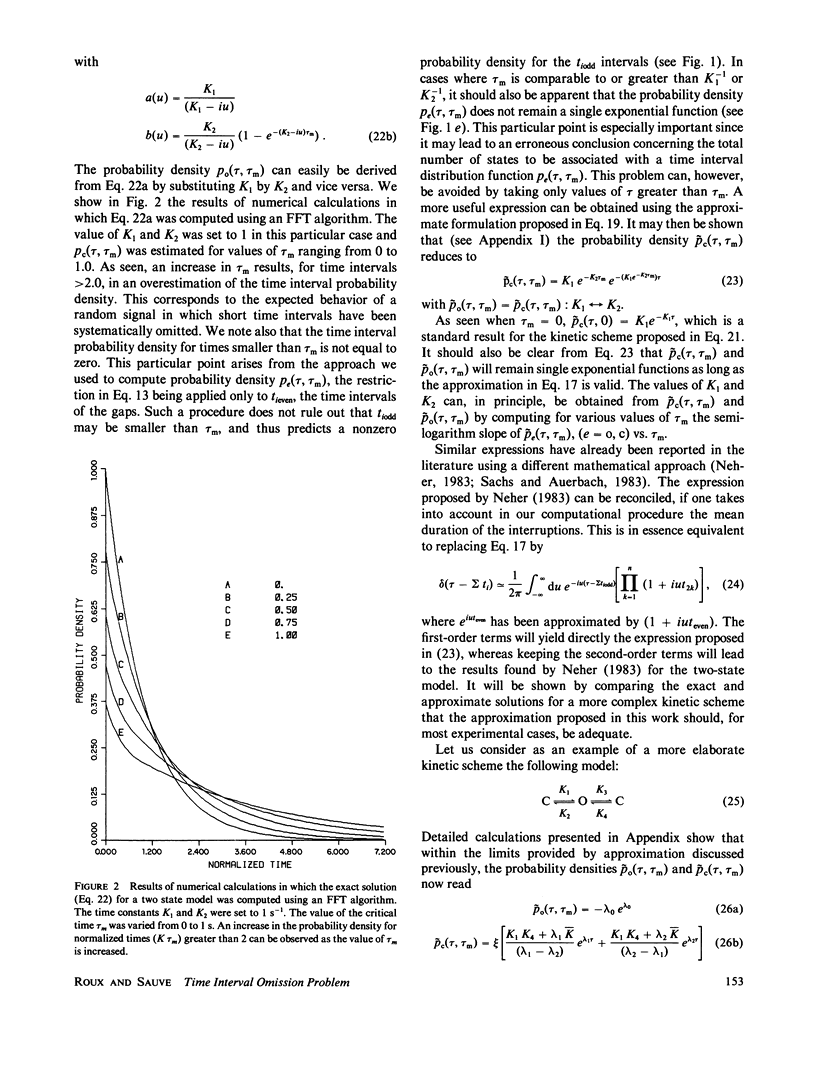
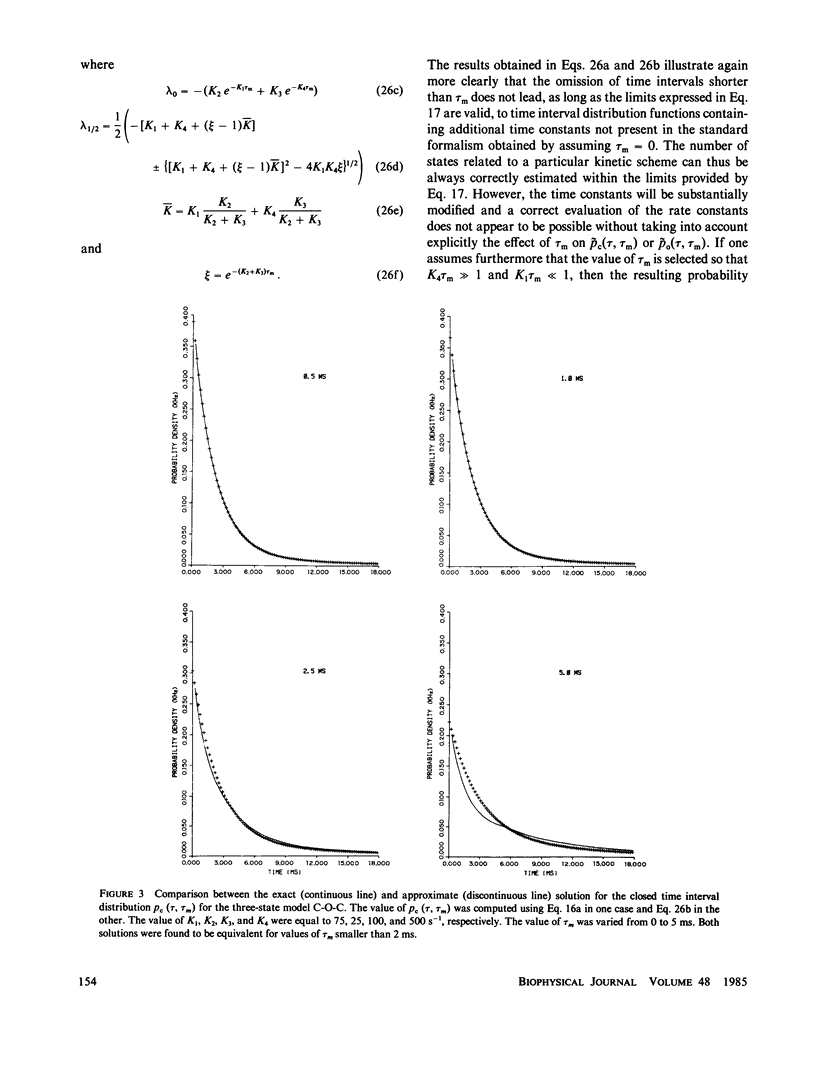
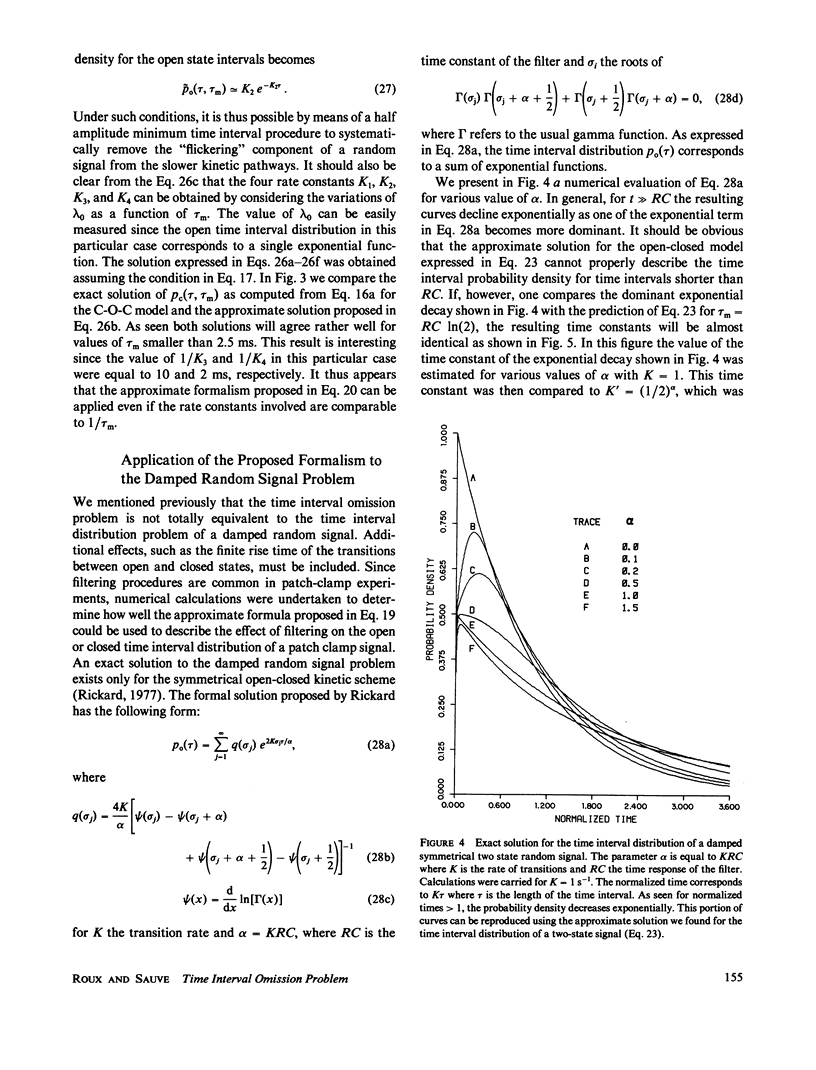
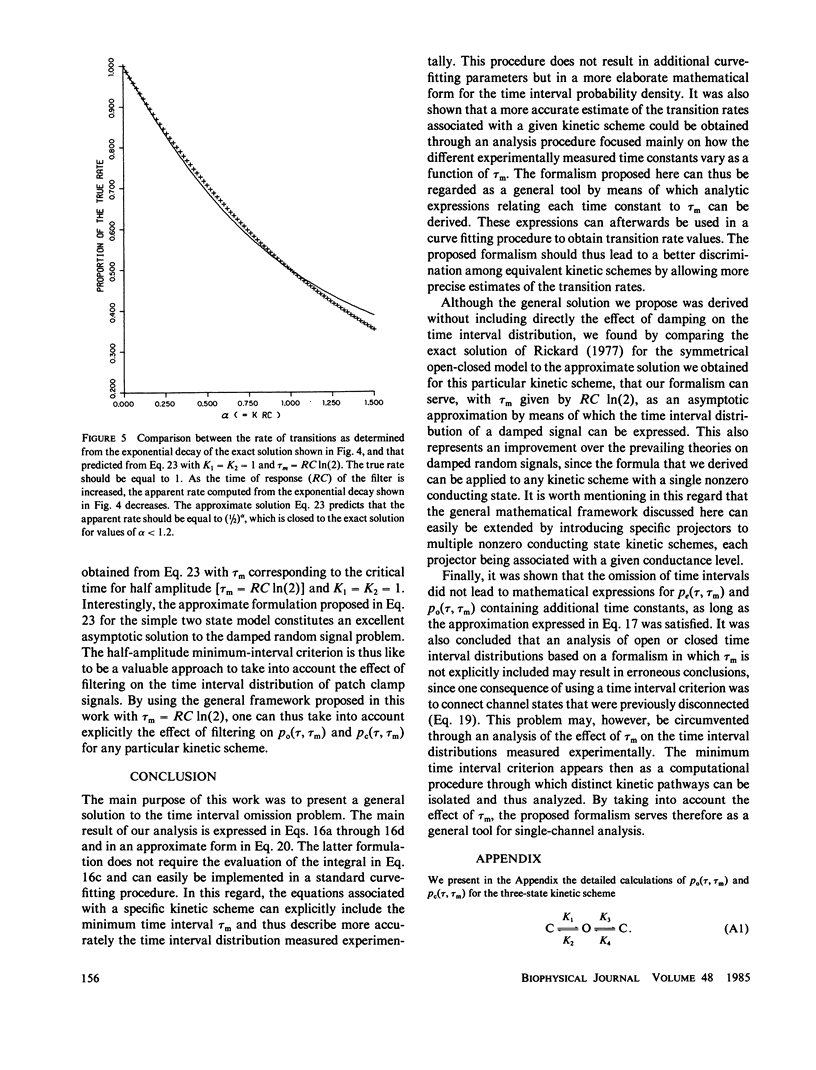
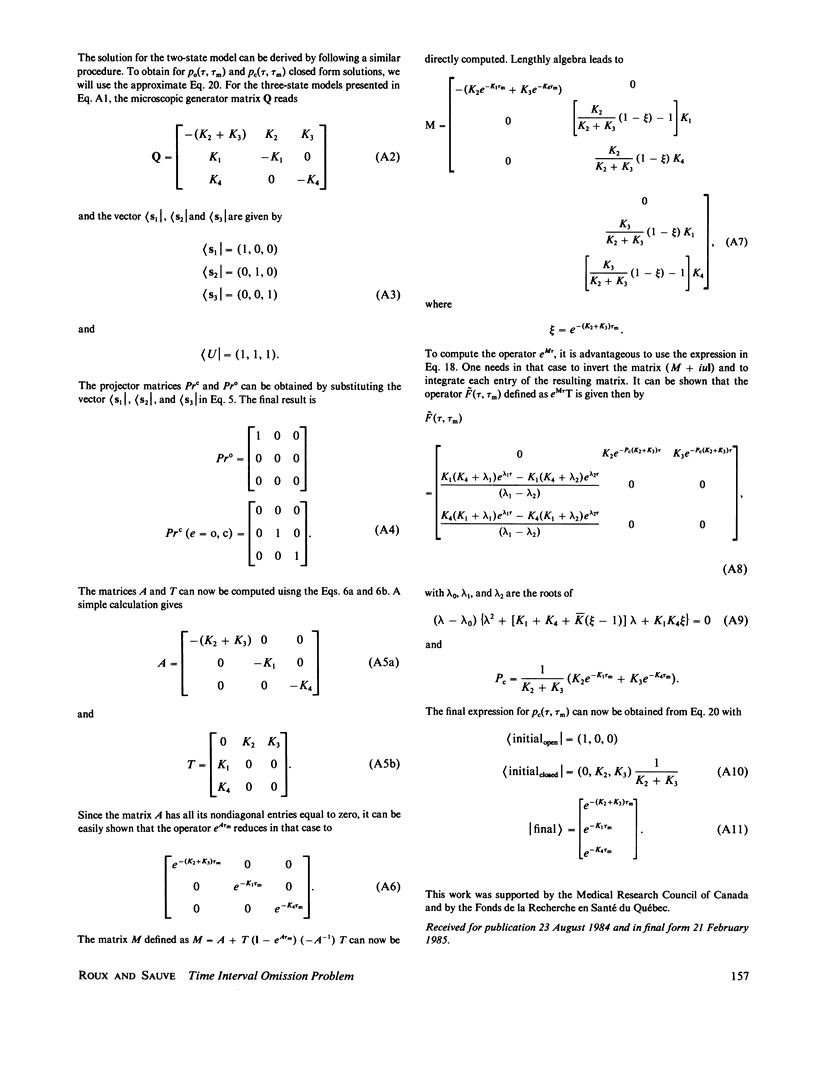
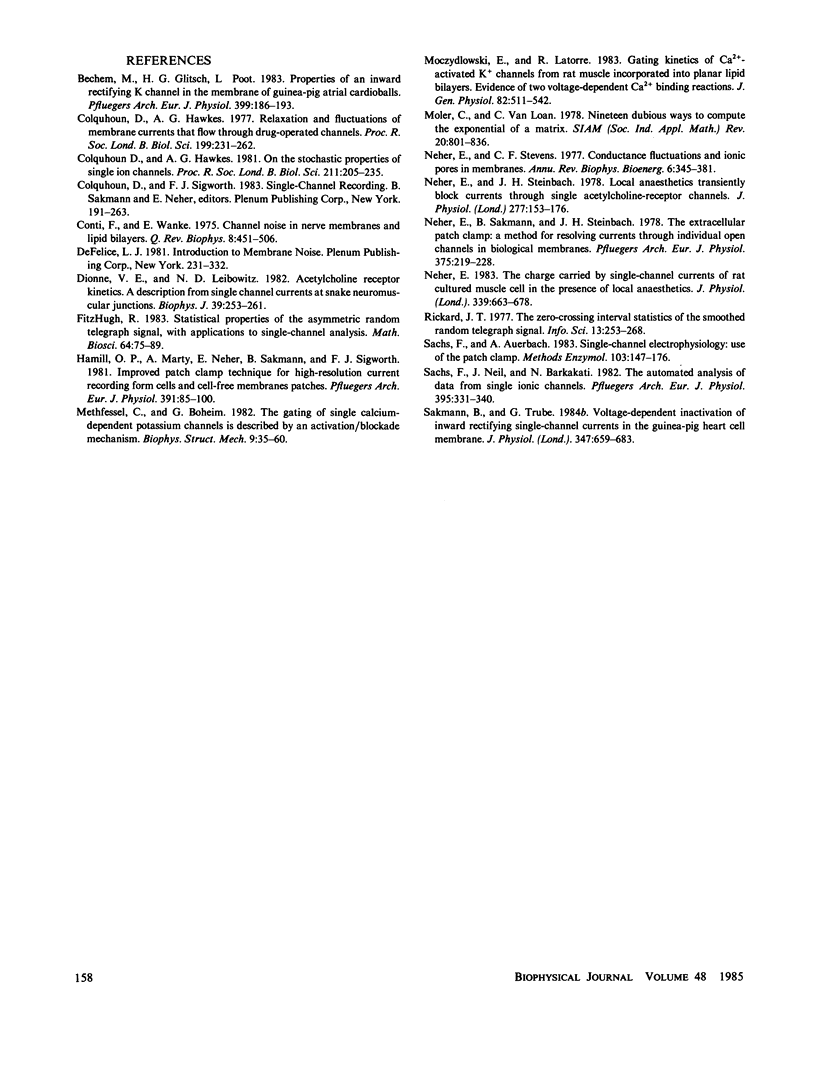
Selected References
These references are in PubMed. This may not be the complete list of references from this article.
- Bechem M., Glitsch H. G., Pott L. Properties of an inward rectifying K channel in the membrane of guinea-pig atrial cardioballs. Pflugers Arch. 1983 Nov;399(3):186–193. doi: 10.1007/BF00656713. [DOI] [PubMed] [Google Scholar]
- Colquhoun D., Hawkes A. G. On the stochastic properties of single ion channels. Proc R Soc Lond B Biol Sci. 1981 Mar 6;211(1183):205–235. doi: 10.1098/rspb.1981.0003. [DOI] [PubMed] [Google Scholar]
- Colquhoun D., Hawkes A. G. Relaxation and fluctuations of membrane currents that flow through drug-operated channels. Proc R Soc Lond B Biol Sci. 1977 Nov 14;199(1135):231–262. doi: 10.1098/rspb.1977.0137. [DOI] [PubMed] [Google Scholar]
- Conti F., Wanke E. Channel noise in nerve membranes and lipid bilayers. Q Rev Biophys. 1975 Nov;8(4):451–506. doi: 10.1017/s0033583500001967. [DOI] [PubMed] [Google Scholar]
- Dionne V. E., Leibowitz M. D. Acetylcholine receptor kinetics. A description from single-channel currents at snake neuromuscular junctions. Biophys J. 1982 Sep;39(3):253–261. doi: 10.1016/S0006-3495(82)84515-3. [DOI] [PMC free article] [PubMed] [Google Scholar]
- Hamill O. P., Marty A., Neher E., Sakmann B., Sigworth F. J. Improved patch-clamp techniques for high-resolution current recording from cells and cell-free membrane patches. Pflugers Arch. 1981 Aug;391(2):85–100. doi: 10.1007/BF00656997. [DOI] [PubMed] [Google Scholar]
- Methfessel C., Boheim G. The gating of single calcium-dependent potassium channels is described by an activation/blockade mechanism. Biophys Struct Mech. 1982;9(1):35–60. doi: 10.1007/BF00536014. [DOI] [PubMed] [Google Scholar]
- Moczydlowski E., Latorre R. Gating kinetics of Ca2+-activated K+ channels from rat muscle incorporated into planar lipid bilayers. Evidence for two voltage-dependent Ca2+ binding reactions. J Gen Physiol. 1983 Oct;82(4):511–542. doi: 10.1085/jgp.82.4.511. [DOI] [PMC free article] [PubMed] [Google Scholar]
- Neher E., Sakmann B., Steinbach J. H. The extracellular patch clamp: a method for resolving currents through individual open channels in biological membranes. Pflugers Arch. 1978 Jul 18;375(2):219–228. doi: 10.1007/BF00584247. [DOI] [PubMed] [Google Scholar]
- Neher E., Steinbach J. H. Local anaesthetics transiently block currents through single acetylcholine-receptor channels. J Physiol. 1978 Apr;277:153–176. doi: 10.1113/jphysiol.1978.sp012267. [DOI] [PMC free article] [PubMed] [Google Scholar]
- Neher E., Stevens C. F. Conductance fluctuations and ionic pores in membranes. Annu Rev Biophys Bioeng. 1977;6:345–381. doi: 10.1146/annurev.bb.06.060177.002021. [DOI] [PubMed] [Google Scholar]
- Neher E. The charge carried by single-channel currents of rat cultured muscle cells in the presence of local anaesthetics. J Physiol. 1983 Jun;339:663–678. doi: 10.1113/jphysiol.1983.sp014741. [DOI] [PMC free article] [PubMed] [Google Scholar]
- Sachs F., Auerbach A. Single-channel electrophysiology: use of the patch clamp. Methods Enzymol. 1983;103:147–176. doi: 10.1016/s0076-6879(83)03011-6. [DOI] [PubMed] [Google Scholar]
- Sachs F., Neil J., Barkakati N. The automated analysis of data from single ionic channels. Pflugers Arch. 1982 Dec;395(4):331–340. doi: 10.1007/BF00580798. [DOI] [PubMed] [Google Scholar]
- Sakmann B., Trube G. Voltage-dependent inactivation of inward-rectifying single-channel currents in the guinea-pig heart cell membrane. J Physiol. 1984 Feb;347:659–683. doi: 10.1113/jphysiol.1984.sp015089. [DOI] [PMC free article] [PubMed] [Google Scholar]