Abstract
Force development by skinned frog semitendinosus fibers was studied at various levels of lateral compression to compare the results with intact fibers and to evaluate the limits on cross-bridge movements during isometric contraction. The skinned fibers were compressed osmotically using a high molecular weight polymer, dextran T500. Ca-activated force remained constant down to 58% of the fiber width (w0) after skinning, corresponding to a nearly twofold change in separation between the thin and thick filaments in the myofilament lattice. This agrees with the earlier result on intact fibers, and gives additional evidence that the cross-bridge mechanism for force generation is relatively insensitive to large changes in interfilament separation. Further compression, below 0.58 w0, produced a sharp drop in force, and the force was practically zero at a fiber width of 50%. The effect at high compression was the same at all pCa's, which indicates that the Ca sensitivity of the myofilaments is unaffected by radial compression. The stiffness of the fiber remained high in rigor in the presence of dextran, which indicates that the rigor cross-bridge attachment is not inhibited, and actually may be improved, with decreases in the interfilament space. Also, the drop in active force with the highest compression was similar when the compressed fibers were put in rigor before contraction, which suggests that the force drop also was not due to a hindrance to cross-bridge attachment. The results appear to exclude large motions such as tilting and rocking of the bridge as a rigid molecule, but suggest that at least some molecular movement is essential for force development; they also raise the possibility that there is a critical interfilament separation in the fiber, below which the cross-bridge cannot function.
Full text
PDF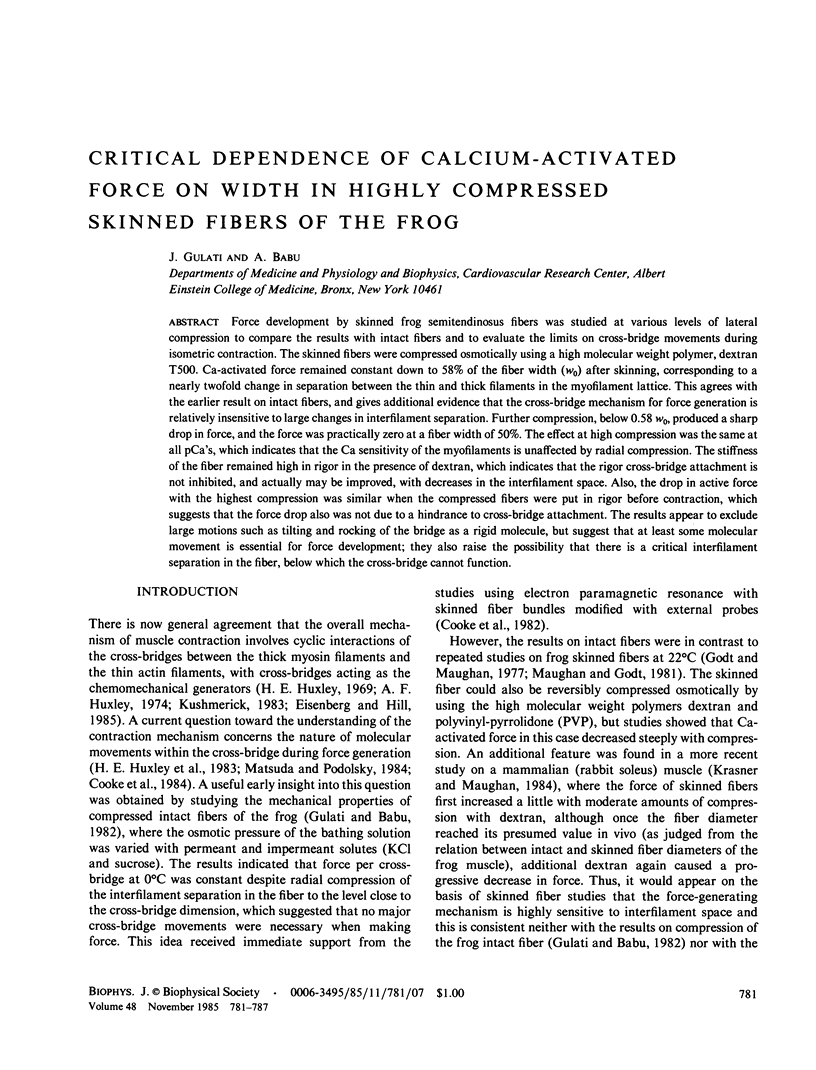
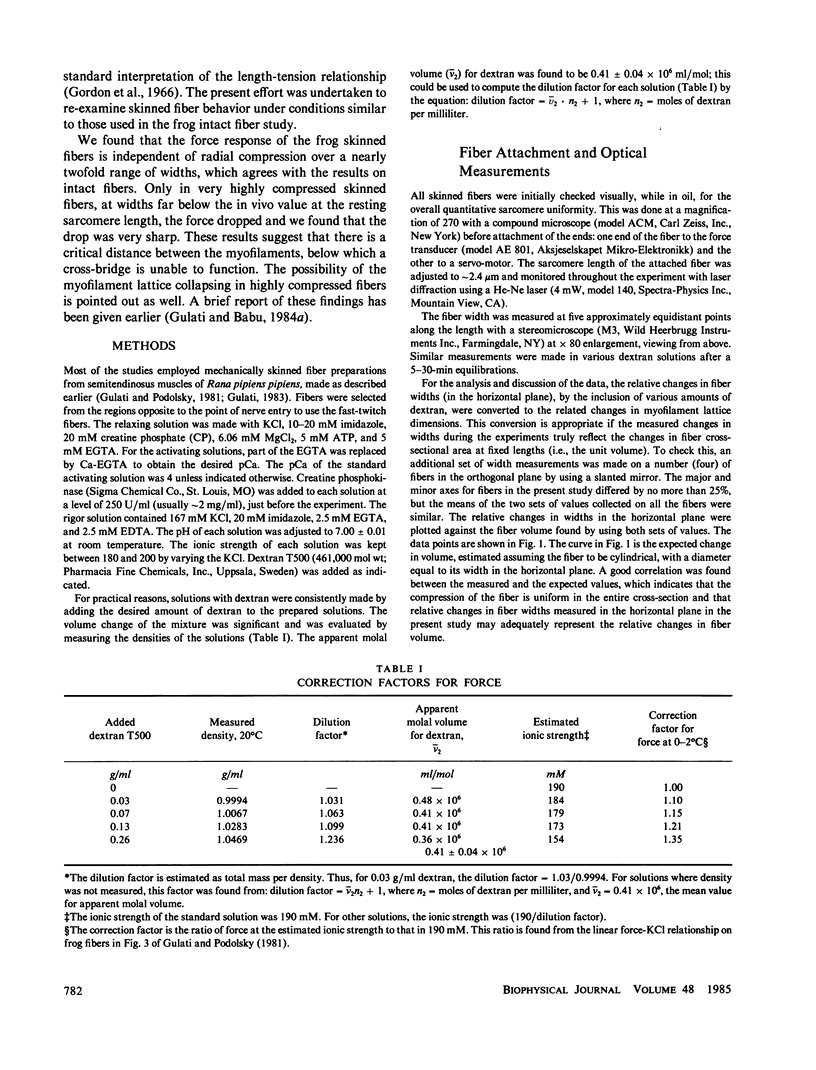
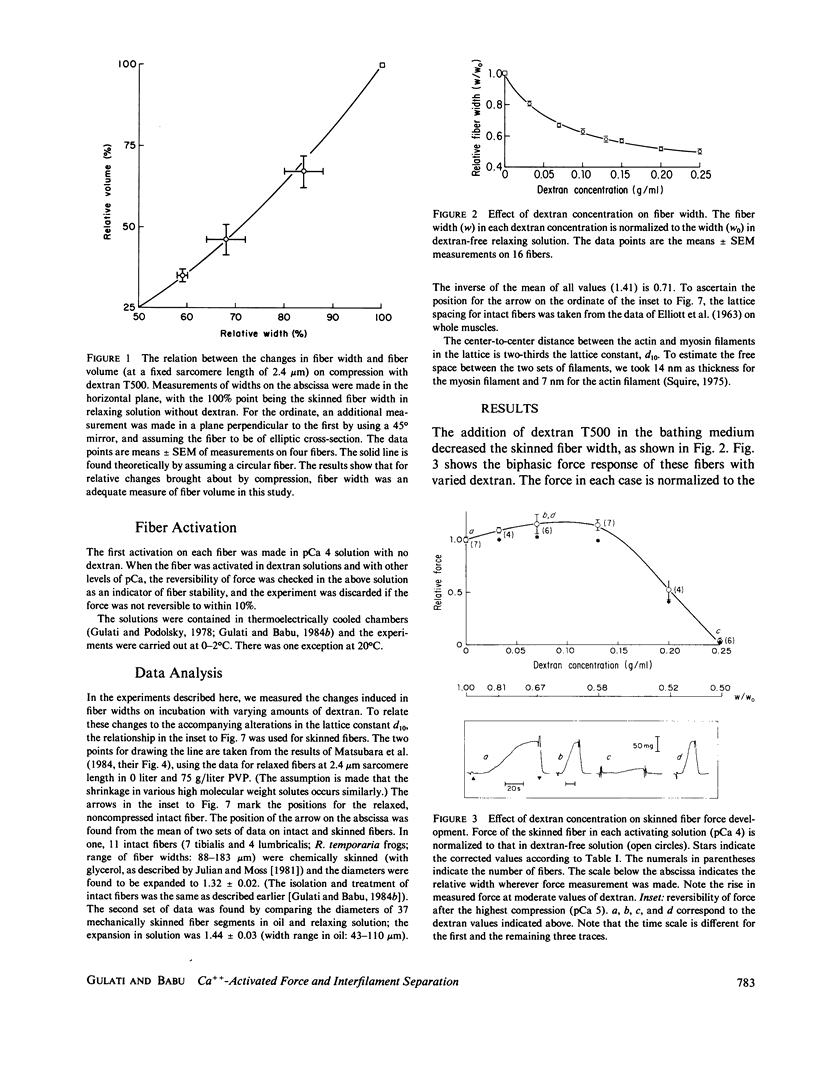
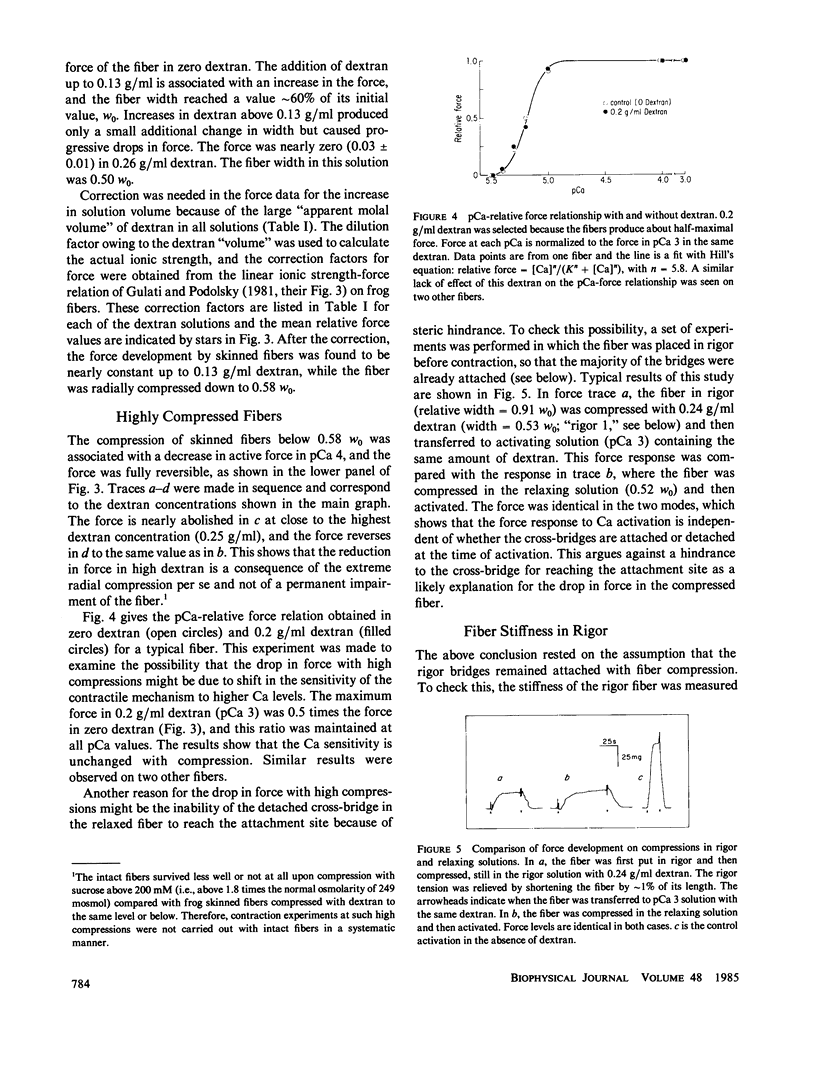
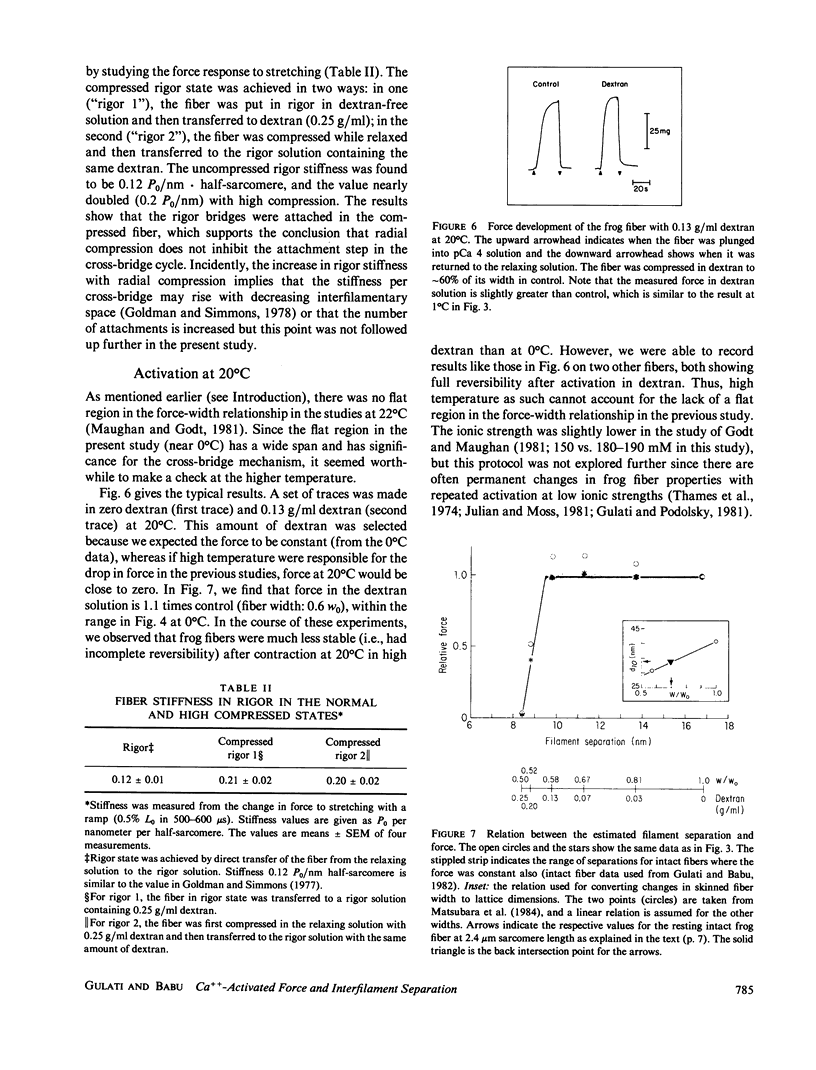
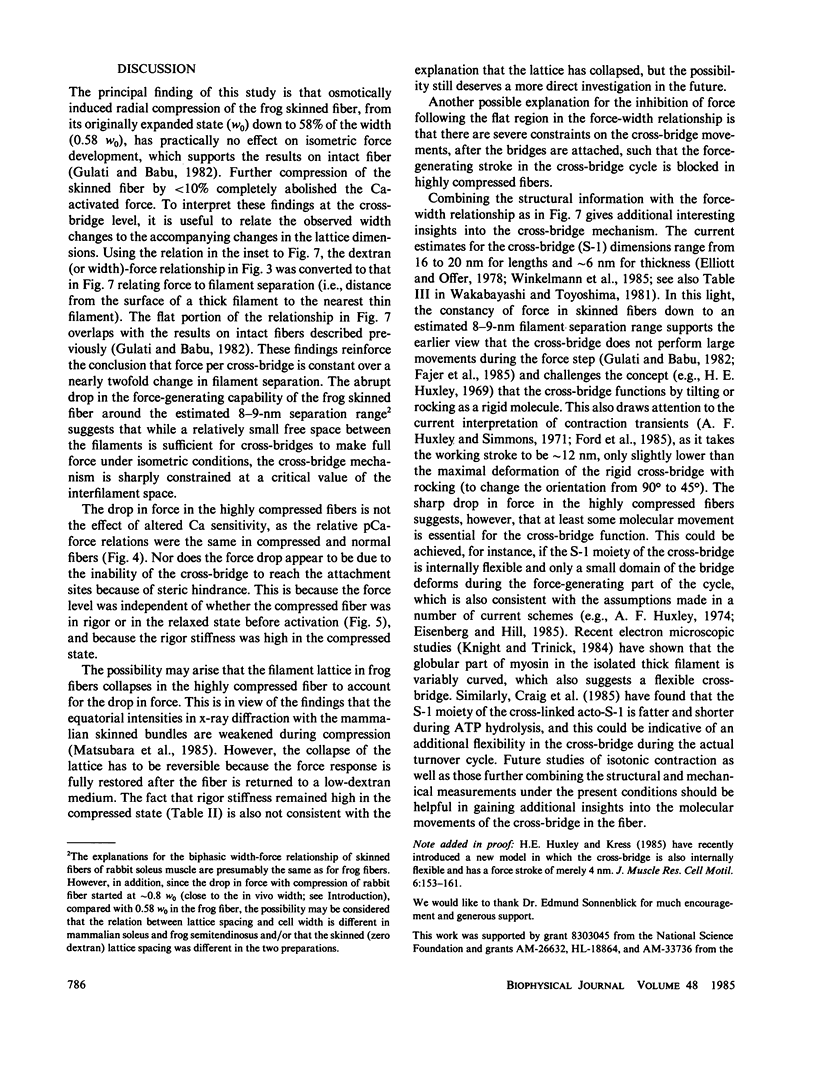
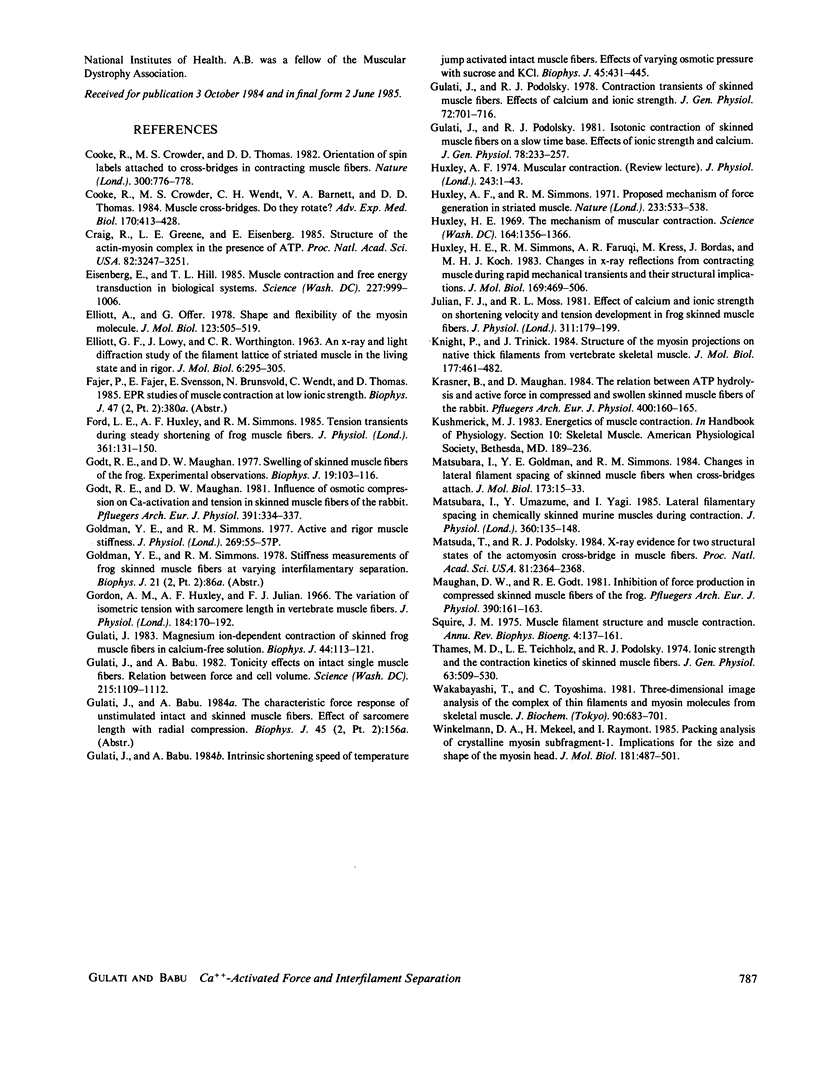
Images in this article
Selected References
These references are in PubMed. This may not be the complete list of references from this article.
- Cooke R., Crowder M. S., Thomas D. D. Orientation of spin labels attached to cross-bridges in contracting muscle fibres. Nature. 1982 Dec 23;300(5894):776–778. doi: 10.1038/300776a0. [DOI] [PubMed] [Google Scholar]
- Cooke R., Crowder M. S., Wendt C. H., Barnett V. A., Thomas D. D. Muscle cross-bridges: do they rotate? Adv Exp Med Biol. 1984;170:413–427. doi: 10.1007/978-1-4684-4703-3_37. [DOI] [PubMed] [Google Scholar]
- Craig R., Greene L. E., Eisenberg E. Structure of the actin-myosin complex in the presence of ATP. Proc Natl Acad Sci U S A. 1985 May;82(10):3247–3251. doi: 10.1073/pnas.82.10.3247. [DOI] [PMC free article] [PubMed] [Google Scholar]
- Eisenberg E., Hill T. L. Muscle contraction and free energy transduction in biological systems. Science. 1985 Mar 1;227(4690):999–1006. doi: 10.1126/science.3156404. [DOI] [PubMed] [Google Scholar]
- Elliott A., Offer G. Shape and flexibility of the myosin molecule. J Mol Biol. 1978 Aug 25;123(4):505–519. doi: 10.1016/0022-2836(78)90204-8. [DOI] [PubMed] [Google Scholar]
- Ford L. E., Huxley A. F., Simmons R. M. Tension transients during steady shortening of frog muscle fibres. J Physiol. 1985 Apr;361:131–150. doi: 10.1113/jphysiol.1985.sp015637. [DOI] [PMC free article] [PubMed] [Google Scholar]
- Godt R. E., Maughan D. W. Influence of osmotic compression on calcium activation and tension in skinned muscle fibers of the rabbit. Pflugers Arch. 1981 Oct;391(4):334–337. doi: 10.1007/BF00581519. [DOI] [PubMed] [Google Scholar]
- Godt R. E., Maughan D. W. Swelling of skinned muscle fibers of the frog. Experimental observations. Biophys J. 1977 Aug;19(2):103–116. doi: 10.1016/S0006-3495(77)85573-2. [DOI] [PMC free article] [PubMed] [Google Scholar]
- Gordon A. M., Huxley A. F., Julian F. J. The variation in isometric tension with sarcomere length in vertebrate muscle fibres. J Physiol. 1966 May;184(1):170–192. doi: 10.1113/jphysiol.1966.sp007909. [DOI] [PMC free article] [PubMed] [Google Scholar]
- Gulati J., Babu A. Intrinsic shortening speed of temperature-jump-activated intact muscle fibers. Effects of varying osmotic pressure with sucrose and KCl. Biophys J. 1984 Feb;45(2):431–445. doi: 10.1016/S0006-3495(84)84166-1. [DOI] [PMC free article] [PubMed] [Google Scholar]
- Gulati J., Babu A. Tonicity effects on intact single muscle fibers: relation between force and cell volume. Science. 1982 Feb 26;215(4536):1109–1112. doi: 10.1126/science.6977845. [DOI] [PubMed] [Google Scholar]
- Gulati J. Magnesium ion-dependent contraction of skinned frog muscle fibers in calcium-free solution. Biophys J. 1983 Oct;44(1):113–121. doi: 10.1016/S0006-3495(83)84283-0. [DOI] [PMC free article] [PubMed] [Google Scholar]
- Gulati J., Podolsky R. J. Contraction transients of skinned muscle fibers: effects of calcium and ionic strength. J Gen Physiol. 1978 Nov;72(5):701–715. doi: 10.1085/jgp.72.5.701. [DOI] [PMC free article] [PubMed] [Google Scholar]
- Gulati J., Podolsky R. J. Isotonic contraction of skinned muscle fibers on a slow time base: effects of ionic strength and calcium. J Gen Physiol. 1981 Sep;78(3):233–257. doi: 10.1085/jgp.78.3.233. [DOI] [PMC free article] [PubMed] [Google Scholar]
- Huxley A. F. Muscular contraction. J Physiol. 1974 Nov;243(1):1–43. [PMC free article] [PubMed] [Google Scholar]
- Huxley A. F., Simmons R. M. Proposed mechanism of force generation in striated muscle. Nature. 1971 Oct 22;233(5321):533–538. doi: 10.1038/233533a0. [DOI] [PubMed] [Google Scholar]
- Huxley H. E., Kress M. Crossbridge behaviour during muscle contraction. J Muscle Res Cell Motil. 1985 Apr;6(2):153–161. doi: 10.1007/BF00713057. [DOI] [PubMed] [Google Scholar]
- Huxley H. E., Simmons R. M., Faruqi A. R., Kress M., Bordas J., Koch M. H. Changes in the X-ray reflections from contracting muscle during rapid mechanical transients and their structural implications. J Mol Biol. 1983 Sep 15;169(2):469–506. doi: 10.1016/s0022-2836(83)80062-x. [DOI] [PubMed] [Google Scholar]
- Huxley H. E. The mechanism of muscular contraction. Science. 1969 Jun 20;164(3886):1356–1365. doi: 10.1126/science.164.3886.1356. [DOI] [PubMed] [Google Scholar]
- Julian F. J., Moss R. L. Effects of calcium and ionic strength on shortening velocity and tension development in frog skinned muscle fibres. J Physiol. 1981 Feb;311:179–199. doi: 10.1113/jphysiol.1981.sp013580. [DOI] [PMC free article] [PubMed] [Google Scholar]
- Knight P., Trinick J. Structure of the myosin projections on native thick filaments from vertebrate skeletal muscle. J Mol Biol. 1984 Aug 15;177(3):461–482. doi: 10.1016/0022-2836(84)90295-x. [DOI] [PubMed] [Google Scholar]
- Krasner B., Maughan D. The relationship between ATP hydrolysis and active force in compressed and swollen skinned muscle fibers of the rabbit. Pflugers Arch. 1984 Feb;400(2):160–165. doi: 10.1007/BF00585033. [DOI] [PubMed] [Google Scholar]
- Matsubara I., Goldman Y. E., Simmons R. M. Changes in the lateral filament spacing of skinned muscle fibres when cross-bridges attach. J Mol Biol. 1984 Feb 15;173(1):15–33. doi: 10.1016/0022-2836(84)90401-7. [DOI] [PubMed] [Google Scholar]
- Matsubara I., Umazume Y., Yagi N. Lateral filamentary spacing in chemically skinned murine muscles during contraction. J Physiol. 1985 Mar;360:135–148. doi: 10.1113/jphysiol.1985.sp015608. [DOI] [PMC free article] [PubMed] [Google Scholar]
- Matsuda T., Podolsky R. J. X-ray evidence for two structural states of the actomyosin cross-bridge in muscle fibers. Proc Natl Acad Sci U S A. 1984 Apr;81(8):2364–2368. doi: 10.1073/pnas.81.8.2364. [DOI] [PMC free article] [PubMed] [Google Scholar]
- Maughan D. W., Godt R. E. Inhibition of force production in compressed skinned muscle fibers of the frog. Pflugers Arch. 1981 May;390(2):161–163. doi: 10.1007/BF00590200. [DOI] [PubMed] [Google Scholar]
- Squire J. M. Muscle filament structure and muscle contraction. Annu Rev Biophys Bioeng. 1975;4(00):137–163. doi: 10.1146/annurev.bb.04.060175.001033. [DOI] [PubMed] [Google Scholar]
- Thames M. D., Teichholz L. E., Podolsky R. J. Ionic strength and the contraction kinetics of skinned muscle fibers. J Gen Physiol. 1974 Apr;63(4):509–530. doi: 10.1085/jgp.63.4.509. [DOI] [PMC free article] [PubMed] [Google Scholar]
- Wakabayashi T., Toyoshima C. Three-dimensional image analysis of the complex of thin filaments and myosin molecules from skeletal muscle. II. The multi-domain structure of actin-myosin S1 complex. J Biochem. 1981 Sep;90(3):683–701. doi: 10.1093/oxfordjournals.jbchem.a133523. [DOI] [PubMed] [Google Scholar]
- Winkelmann D. A., Mekeel H., Rayment I. Packing analysis of crystalline myosin subfragment-1. Implications for the size and shape of the myosin head. J Mol Biol. 1985 Feb 20;181(4):487–501. doi: 10.1016/0022-2836(85)90422-x. [DOI] [PubMed] [Google Scholar]