Abstract
The interaction of QX222, a quaternary ammonium derivative of lidocaine, with the Na channel was studied in internally perfused squid axons under voltage-clamped conditions. A use-dependent block was observed in response to repetitive depolarizing pulses. The time constant for block development and the steady state level of the block were increased with increasing frequency of stimulation from 0.1 to 10 Hz. Use-dependent block can be viewed as a net increase in the drug incorporation into Na channels with successive pulses. That is, net drug uptake by Na channels occurs during the depolarizing phase and net drug release occurs during the interpulse interval. The observed uptake rate of use-dependent block is shown to be a linear combination of the uptake rates associated with the depolarizing and resting potentials. Also, the steady state fraction of blocked channels is shown to be a linear combination of the state-dependent blockade equilibria. Drug-channel interactions are assumed to be dependent on gated control of the diffusion path between drug pool and the interior channel binding site. Drug ingress to the binding site can be inhibited by the channel gates (receptor guarding), while drug bound to the channel may become trapped by closure of the channel gates (trapping). On the basis of these assumptions, a simple procedure is proposed for estimating apparent rate constants governing the drug-channel binding reactions for two cases of channel blockade. The estimated forward (k) and backward (1) rate constants are: 2.45 x I05 M-1 s- and 0.23 x 103 s-1, respectively, for k and I for the case when the drug is trapped by both activation and inactivation gates, and 3.58 x 105 M-l s-l and 4.15 x 10-3 S-l for the case when the drug is not trapped. While these two schemes make a similar prediction with respect to the resulting uptake rates, their prediction of the steady state level of block differs. The observed steady state level of block could quantitatively be predicted by the trapped scheme but not by the untrapped scheme, thus providing a means for discriminating between these two schemes. In addition, the trapped scheme, but not the untrapped scheme, could provide an explanation for the observed voltage dependence of the slow recovery from use-dependent block.
Full text
PDF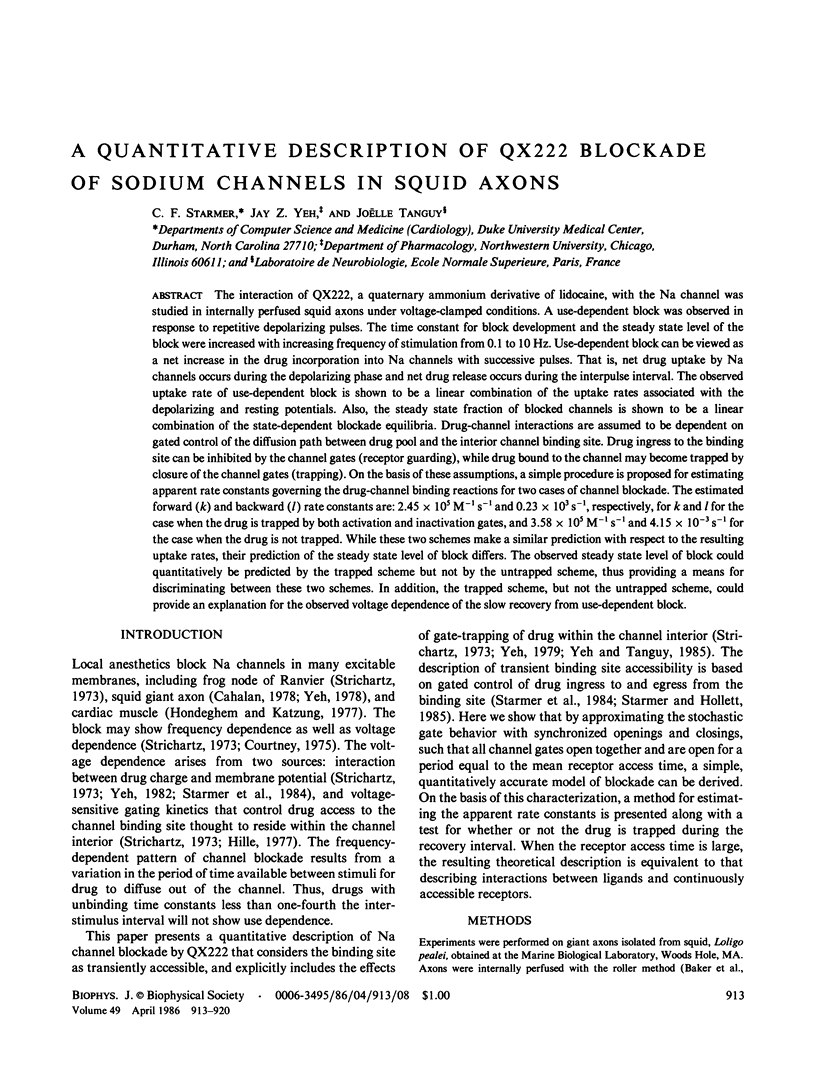
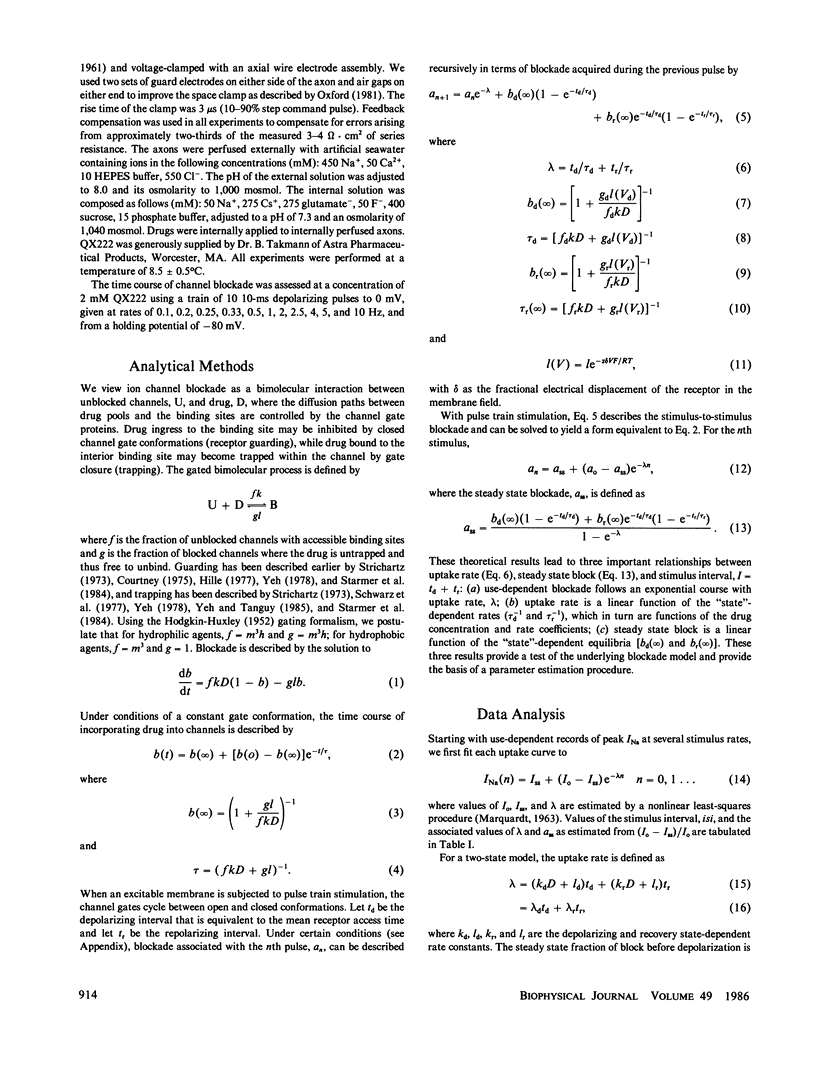
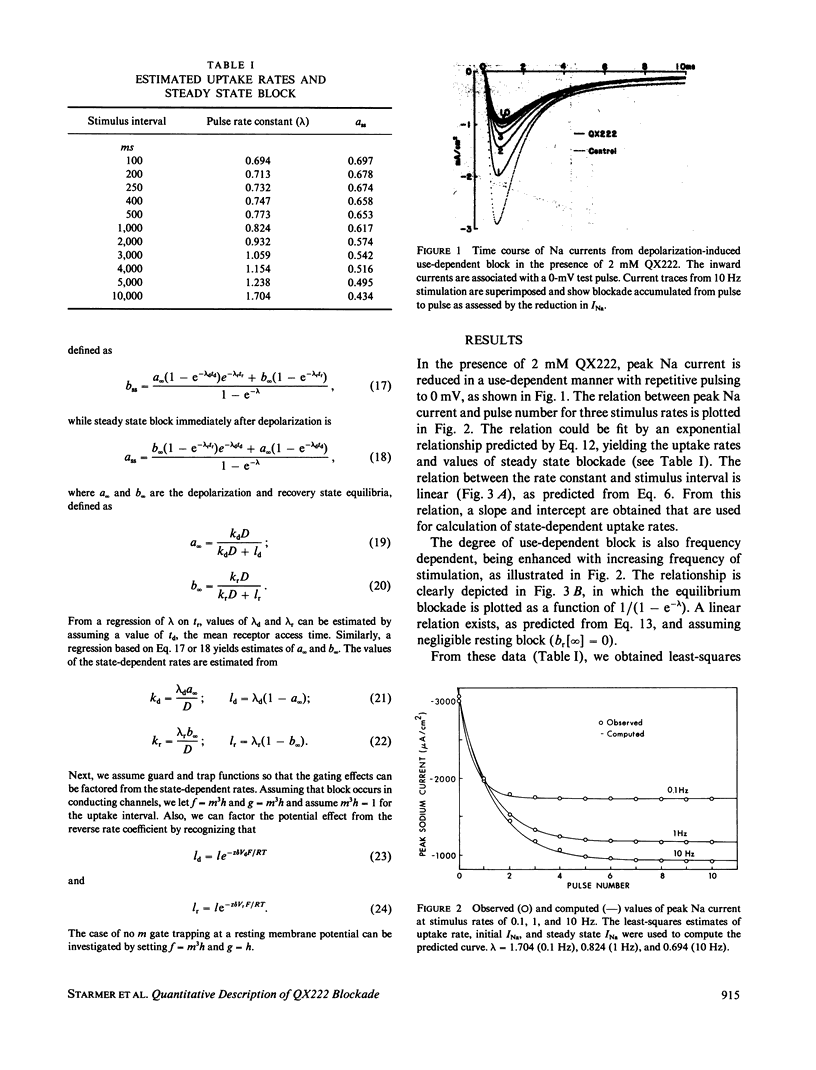
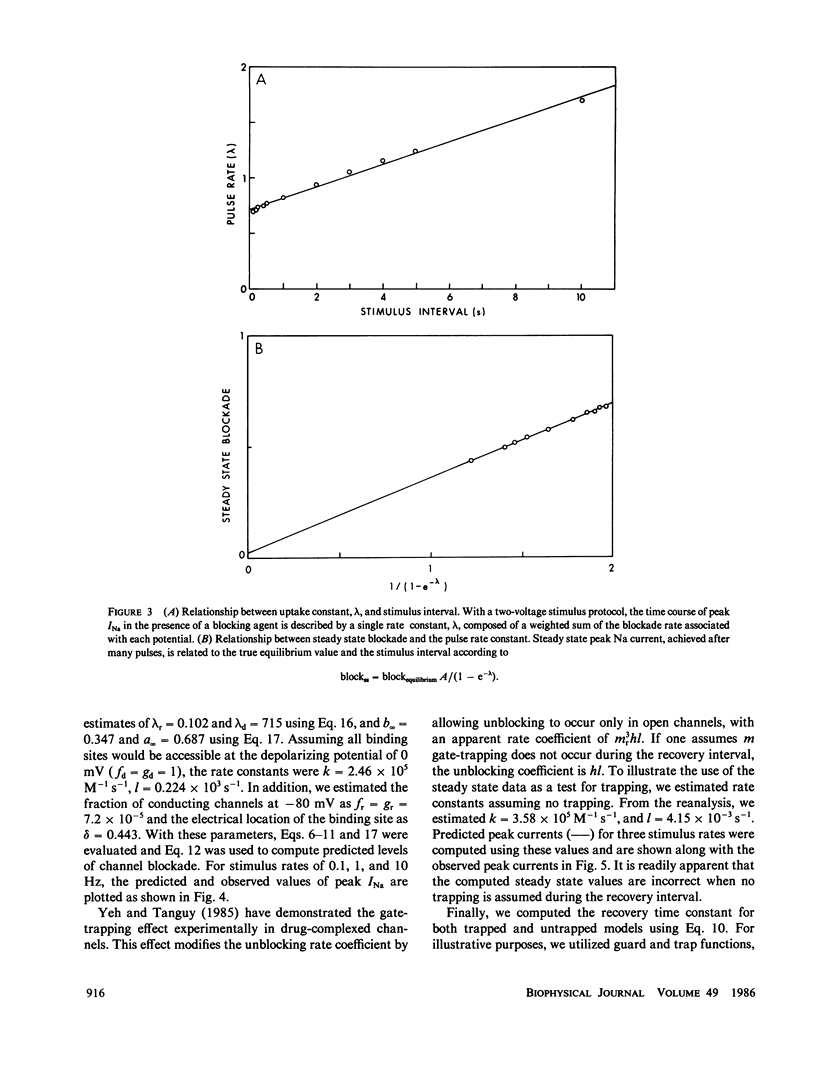
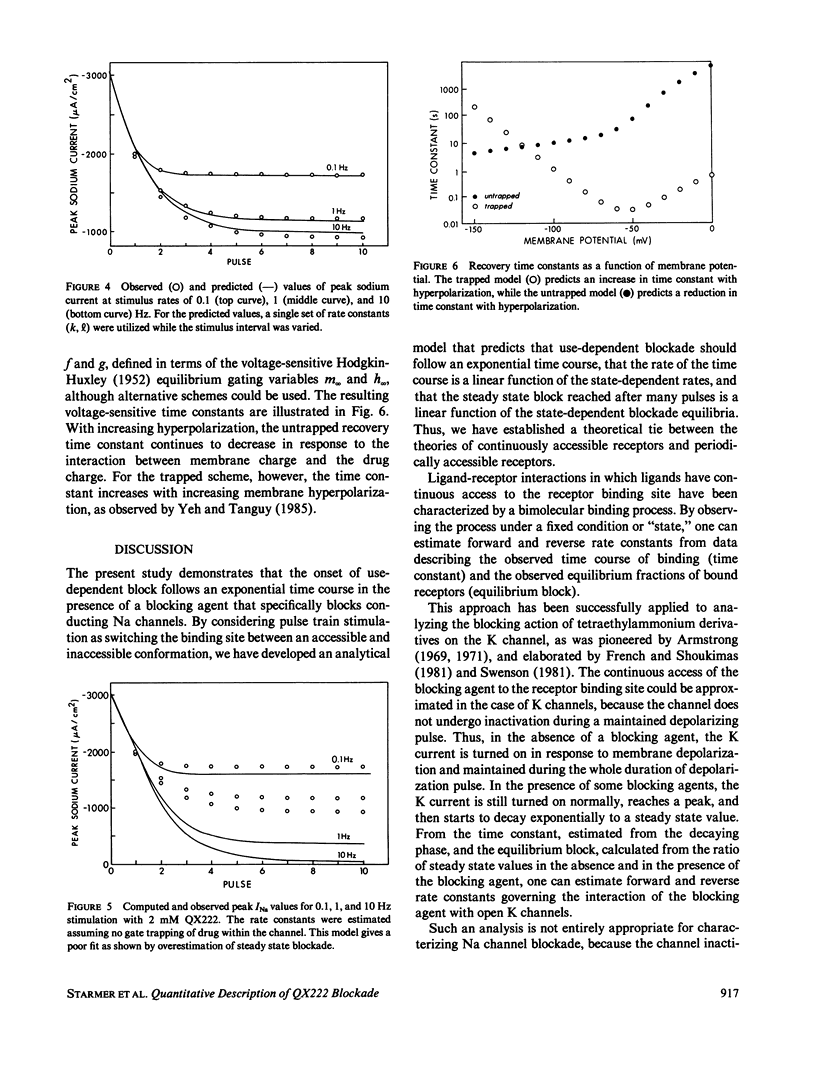
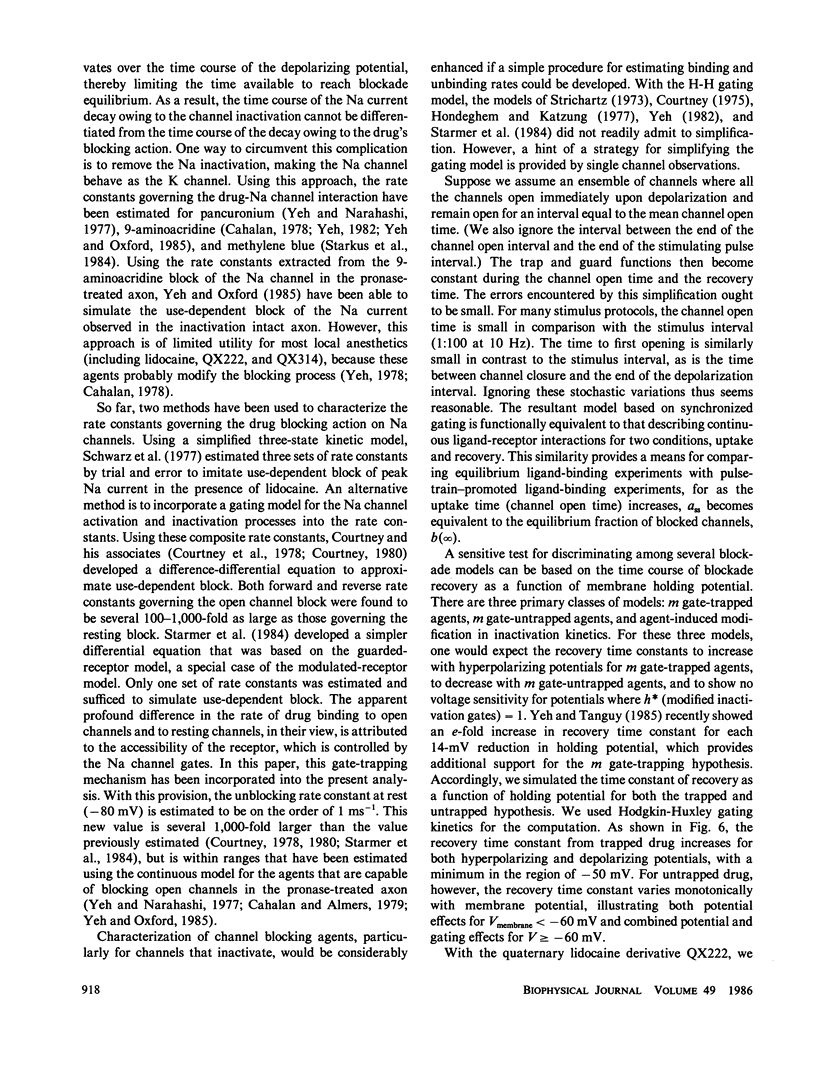
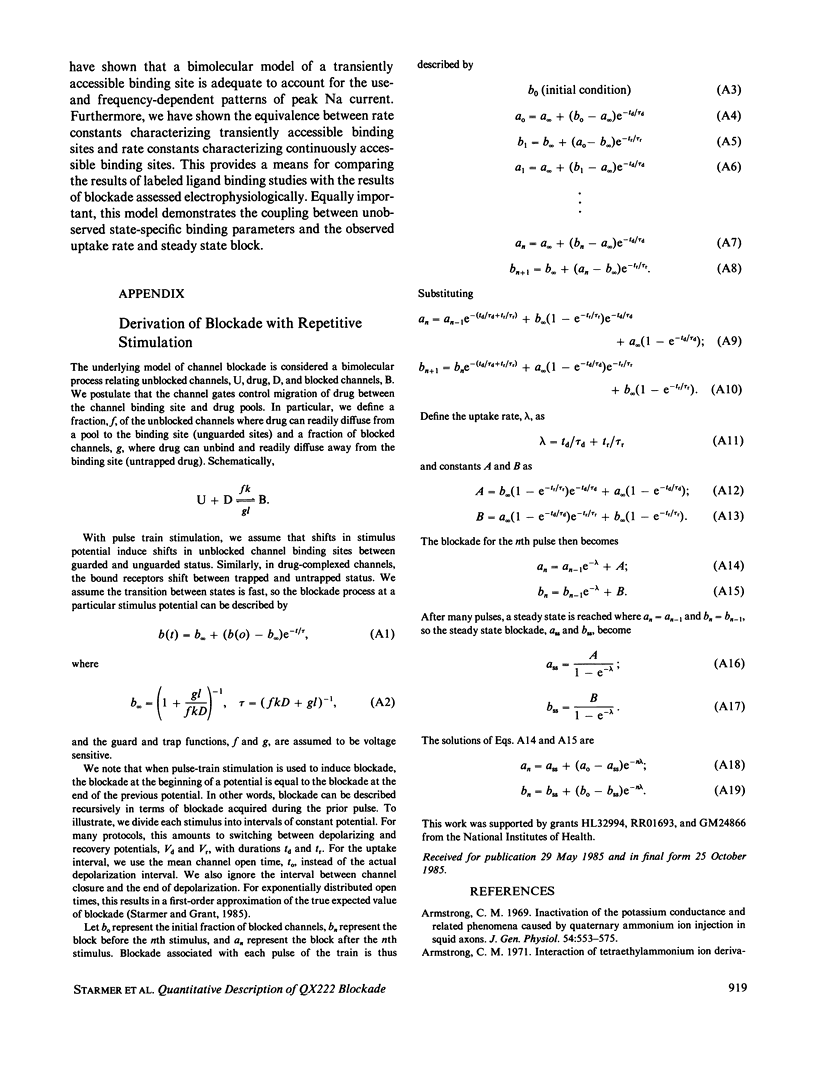
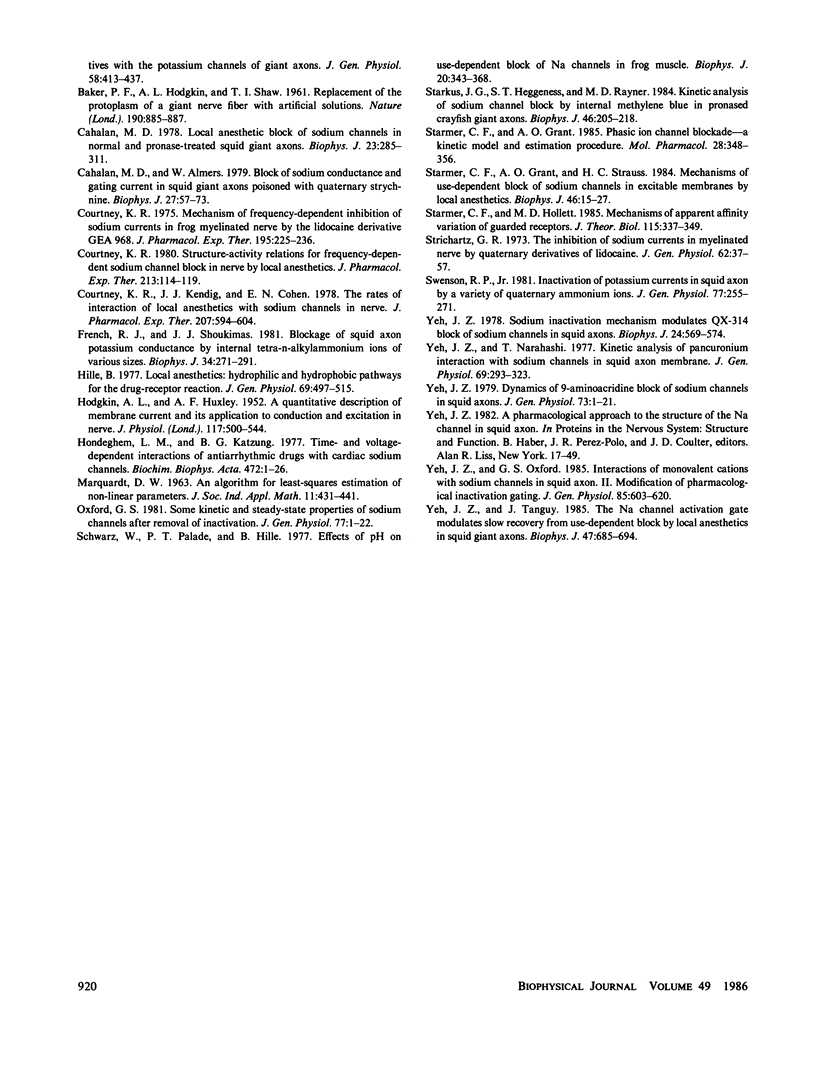
Selected References
These references are in PubMed. This may not be the complete list of references from this article.
- Armstrong C. M. Inactivation of the potassium conductance and related phenomena caused by quaternary ammonium ion injection in squid axons. J Gen Physiol. 1969 Nov;54(5):553–575. doi: 10.1085/jgp.54.5.553. [DOI] [PMC free article] [PubMed] [Google Scholar]
- Armstrong C. M. Interaction of tetraethylammonium ion derivatives with the potassium channels of giant axons. J Gen Physiol. 1971 Oct;58(4):413–437. doi: 10.1085/jgp.58.4.413. [DOI] [PMC free article] [PubMed] [Google Scholar]
- BAKER P. F., HODGKIN A. L., SHAW T. I. Replacement of the protoplasm of a giant nerve fibre with artificial solutions. Nature. 1961 Jun 3;190:885–887. doi: 10.1038/190885a0. [DOI] [PubMed] [Google Scholar]
- Cahalan M. D., Almers W. Block of sodium conductance and gating current in squid giant axons poisoned with quaternary strychnine. Biophys J. 1979 Jul;27(1):57–73. doi: 10.1016/S0006-3495(79)85202-9. [DOI] [PMC free article] [PubMed] [Google Scholar]
- Cahalan M. D. Local anesthetic block of sodium channels in normal and pronase-treated squid giant axons. Biophys J. 1978 Aug;23(2):285–311. doi: 10.1016/S0006-3495(78)85449-6. [DOI] [PMC free article] [PubMed] [Google Scholar]
- Courtney K. R., Kendig J. J., Cohen E. N. The rates of interaction of local anesthetics with sodium channels in nerve. J Pharmacol Exp Ther. 1978 Nov;207(2):594–604. [PubMed] [Google Scholar]
- Courtney K. R. Mechanism of frequency-dependent inhibition of sodium currents in frog myelinated nerve by the lidocaine derivative GEA. J Pharmacol Exp Ther. 1975 Nov;195(2):225–236. [PubMed] [Google Scholar]
- Courtney K. R. Structure-activity relations for frequency-dependent sodium channel block in nerve by local anesthetics. J Pharmacol Exp Ther. 1980 Apr;213(1):114–119. [PubMed] [Google Scholar]
- French R. J., Shoukimas J. J. Blockage of squid axon potassium conductance by internal tetra-N-alkylammonium ions of various sizes. Biophys J. 1981 May;34(2):271–291. doi: 10.1016/S0006-3495(81)84849-7. [DOI] [PMC free article] [PubMed] [Google Scholar]
- HODGKIN A. L., HUXLEY A. F. A quantitative description of membrane current and its application to conduction and excitation in nerve. J Physiol. 1952 Aug;117(4):500–544. doi: 10.1113/jphysiol.1952.sp004764. [DOI] [PMC free article] [PubMed] [Google Scholar]
- Hille B. Local anesthetics: hydrophilic and hydrophobic pathways for the drug-receptor reaction. J Gen Physiol. 1977 Apr;69(4):497–515. doi: 10.1085/jgp.69.4.497. [DOI] [PMC free article] [PubMed] [Google Scholar]
- Lambert P. A., Hancock I. C., Baddiley J. Occurrence and function of membrane teichoic acids. Biochim Biophys Acta. 1977 May 31;472(1):1–12. doi: 10.1016/0304-4157(77)90012-0. [DOI] [PubMed] [Google Scholar]
- Oxford G. S. Some kinetic and steady-state properties of sodium channels after removal of inactivation. J Gen Physiol. 1981 Jan;77(1):1–22. doi: 10.1085/jgp.77.1.1. [DOI] [PMC free article] [PubMed] [Google Scholar]
- Schwarz W., Palade P. T., Hille B. Local anesthetics. Effect of pH on use-dependent block of sodium channels in frog muscle. Biophys J. 1977 Dec;20(3):343–368. doi: 10.1016/S0006-3495(77)85554-9. [DOI] [PMC free article] [PubMed] [Google Scholar]
- Starkus J. G., Heggeness S. T., Rayner M. D. Kinetic analysis of sodium channel block by internal methylene blue in pronased crayfish giant axons. Biophys J. 1984 Aug;46(2):205–218. doi: 10.1016/S0006-3495(84)84014-X. [DOI] [PMC free article] [PubMed] [Google Scholar]
- Starmer C. F., Grant A. O. Phasic ion channel blockade. A kinetic model and parameter estimation procedure. Mol Pharmacol. 1985 Oct;28(4):348–356. [PubMed] [Google Scholar]
- Starmer C. F., Grant A. O., Strauss H. C. Mechanisms of use-dependent block of sodium channels in excitable membranes by local anesthetics. Biophys J. 1984 Jul;46(1):15–27. doi: 10.1016/S0006-3495(84)83994-6. [DOI] [PMC free article] [PubMed] [Google Scholar]
- Starmer C. F., Hollett M. D. Mechanisms of apparent affinity variation of guarded receptors. J Theor Biol. 1985 Aug 7;115(3):337–349. doi: 10.1016/s0022-5193(85)80196-x. [DOI] [PubMed] [Google Scholar]
- Strichartz G. R. The inhibition of sodium currents in myelinated nerve by quaternary derivatives of lidocaine. J Gen Physiol. 1973 Jul;62(1):37–57. doi: 10.1085/jgp.62.1.37. [DOI] [PMC free article] [PubMed] [Google Scholar]
- Swenson R. P., Jr Inactivation of potassium current in squid axon by a variety of quaternary ammonium ions. J Gen Physiol. 1981 Mar;77(3):255–271. doi: 10.1085/jgp.77.3.255. [DOI] [PMC free article] [PubMed] [Google Scholar]
- Yeh J. Z. A pharmacological approach to the structure of the Na channel in squid axon. Prog Clin Biol Res. 1982;79:17–49. [PubMed] [Google Scholar]
- Yeh J. Z. Dynamics of 9-aminoacridine block of sodium channels in squid axons. J Gen Physiol. 1979 Jan;73(1):1–21. doi: 10.1085/jgp.73.1.1. [DOI] [PMC free article] [PubMed] [Google Scholar]
- Yeh J. Z., Narahashi T. Kinetic analysis of pancuronium interaction with sodium channels in squid axon membranes. J Gen Physiol. 1977 Mar;69(3):293–323. doi: 10.1085/jgp.69.3.293. [DOI] [PMC free article] [PubMed] [Google Scholar]
- Yeh J. Z., Oxford G. S. Interactions of monovalent cations with sodium channels in squid axon. II. Modification of pharmacological inactivation gating. J Gen Physiol. 1985 Apr;85(4):603–620. doi: 10.1085/jgp.85.4.603. [DOI] [PMC free article] [PubMed] [Google Scholar]
- Yeh J. Z. Sodium inactivation mechanism modulates QX-314 block of sodium channels in squid axons. Biophys J. 1978 Nov;24(2):569–574. doi: 10.1016/S0006-3495(78)85403-4. [DOI] [PMC free article] [PubMed] [Google Scholar]
- Yeh J. Z., Tanguy J. Na channel activation gate modulates slow recovery from use-dependent block by local anesthetics in squid giant axons. Biophys J. 1985 May;47(5):685–694. doi: 10.1016/S0006-3495(85)83965-5. [DOI] [PMC free article] [PubMed] [Google Scholar]