Abstract
Physical studies of human erythrocyte spectrin indicate that isolated spectrin dimers and tetramers in solution are worm-like coils with a persistence length of approximately 20 nm. This finding, the known polyelectrolytic nature of spectrin, and other structural information about spectrin and the membrane skeleton molecular organization have lead us to the hypothesis that the human erythrocyte membrane skeleton constitutes a two-dimensional ionic gel (swollen ionic elastomer). This concept is incorporated in what we refer to as the protein gel-lipid bilayer membrane model. The model accounts quantitatively for red elastic shear modulus and the maximum elastic extension ratio reported for the human erythrocytes membrane. Gel theory further predicts that depending on the environmental conditions, the membrane skeleton modulus of area compression may be small or large relative to the membrane elastic shear modulus. Our analyses show that the ratio between these two parameters affects both the geometry and the stability of the favored cell shapes and that the higher the membrane skeleton compressibility the smaller the values of the gel tension needed to induce cell shape transformations. The main virtue of the protein gel-lipid bilayer membrane model is that it offers a novel theoretical and molecular basis for the various mechanical properties of the membrane skeleton such as the membrane skeleton modulus of area compression and osmotic tension, and the effects of these properties on local membrane skeleton density, cell shape, and shape transformations.
Full text
PDF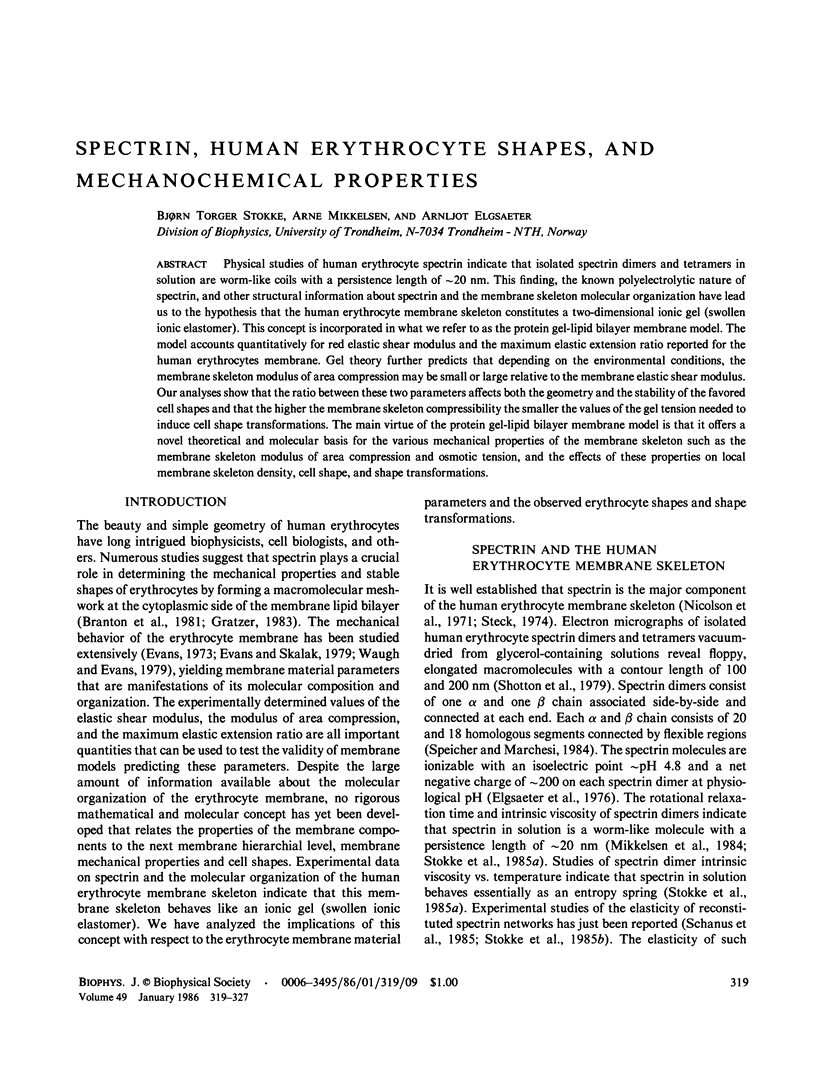
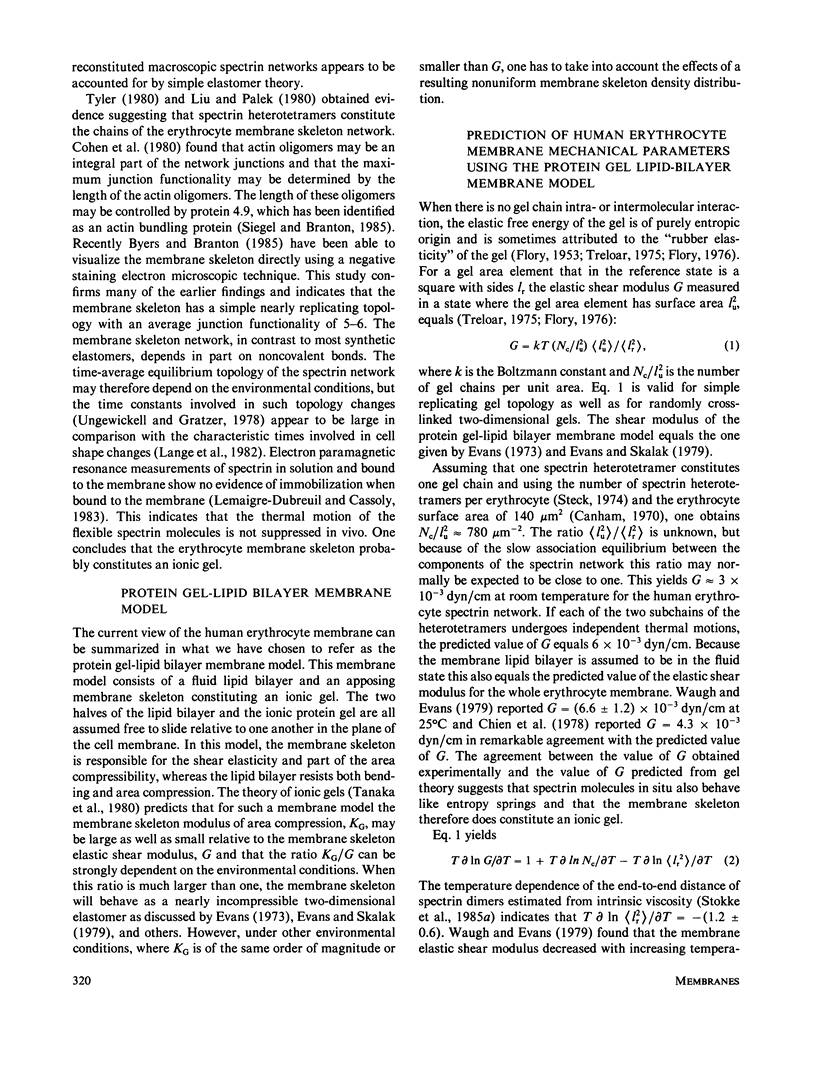
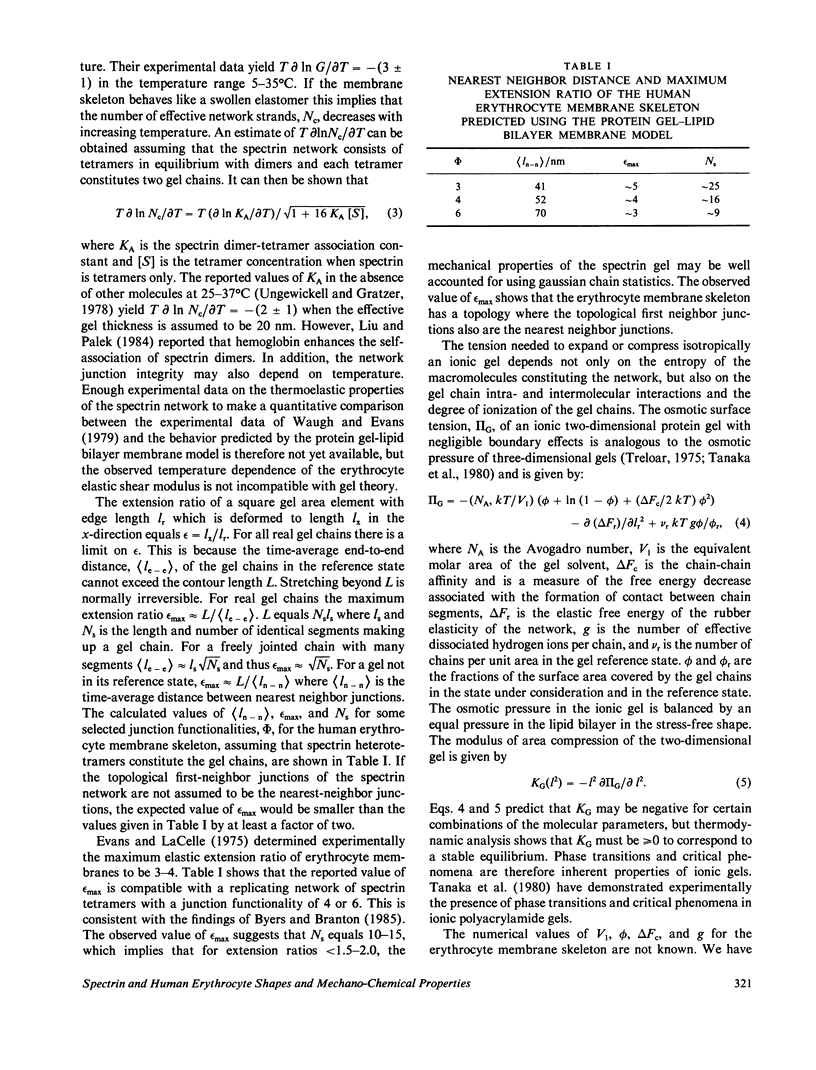
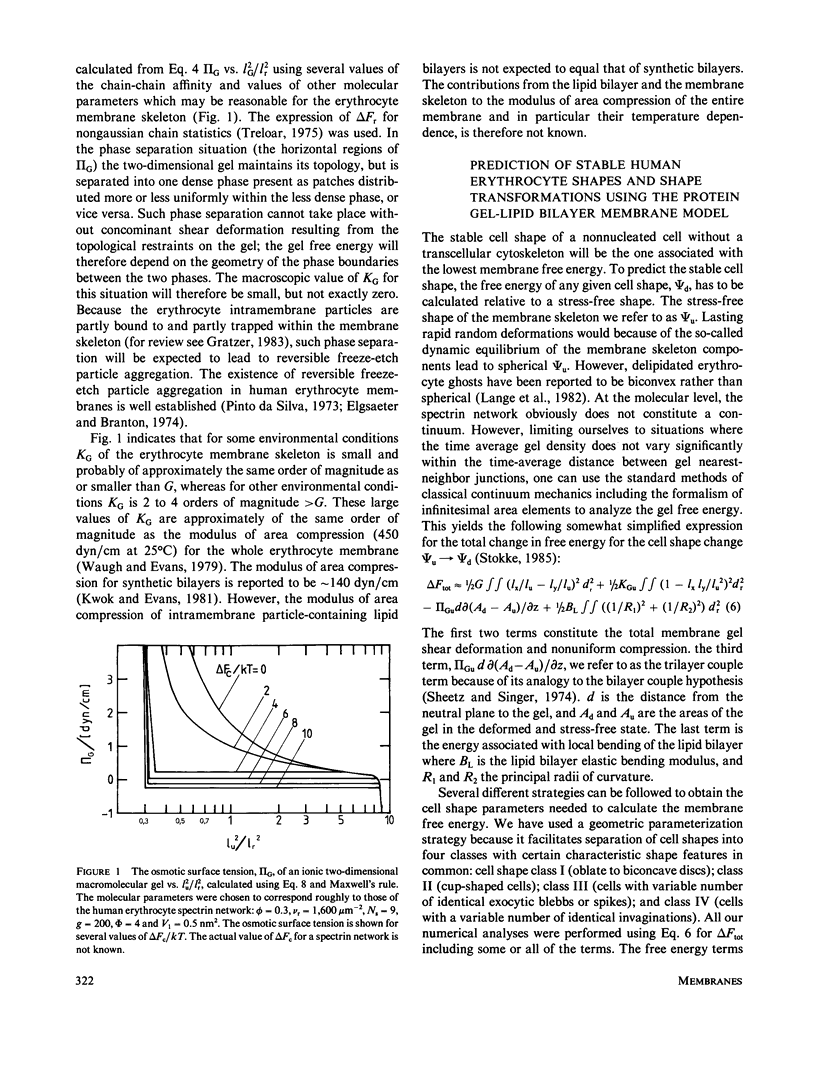
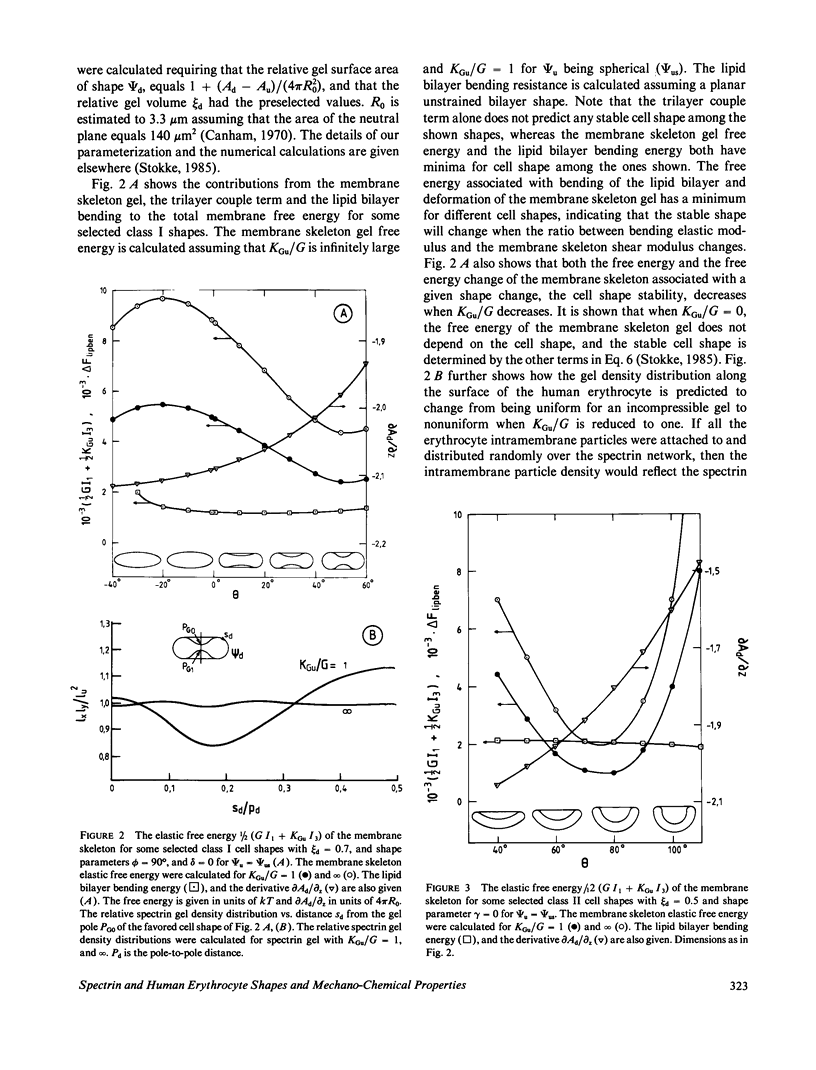
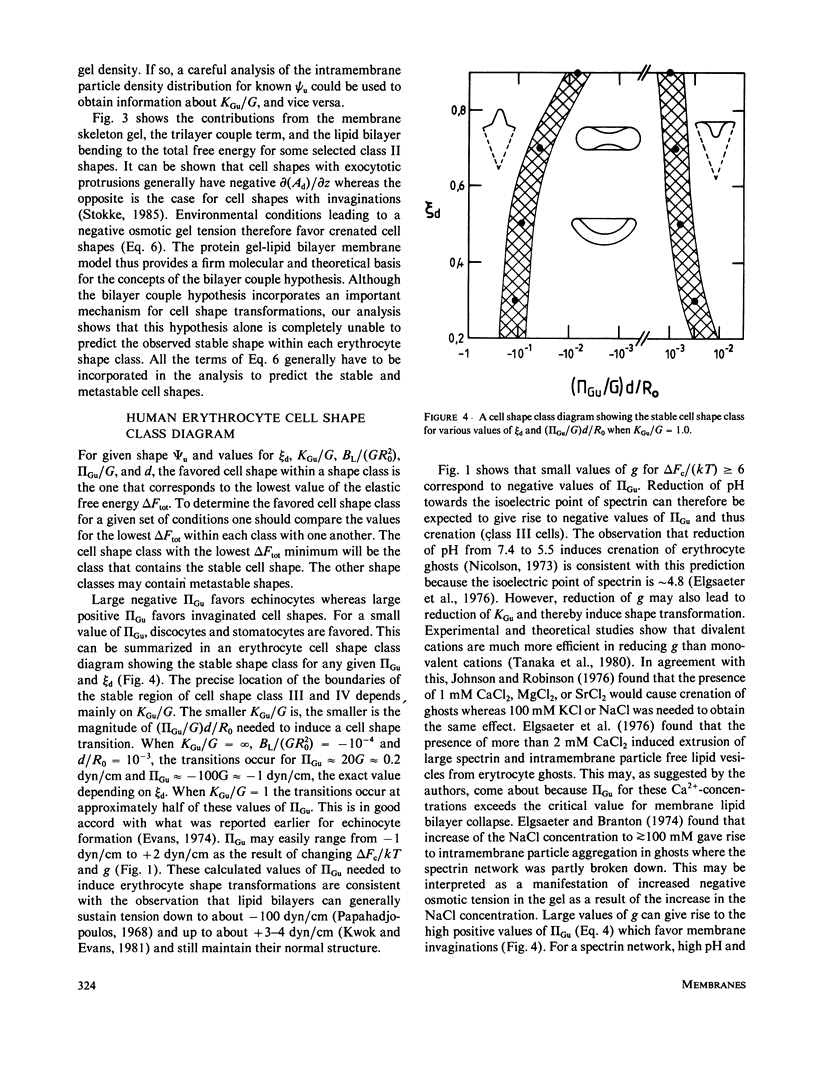
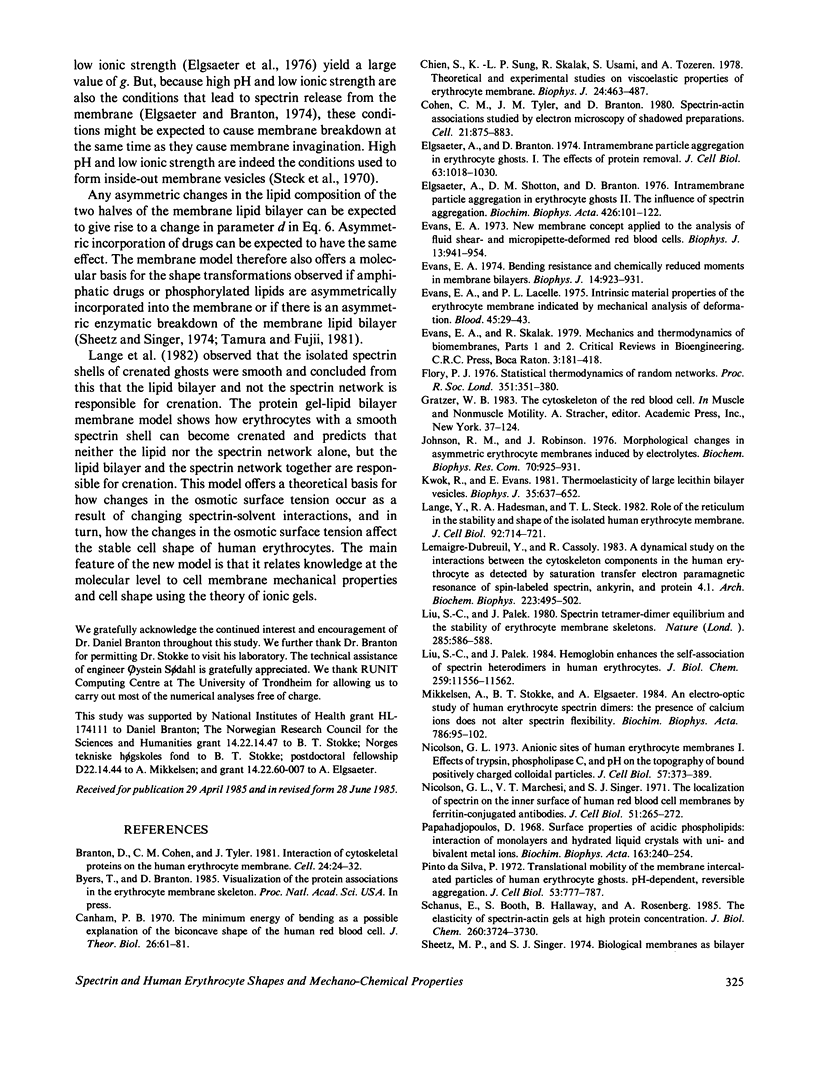
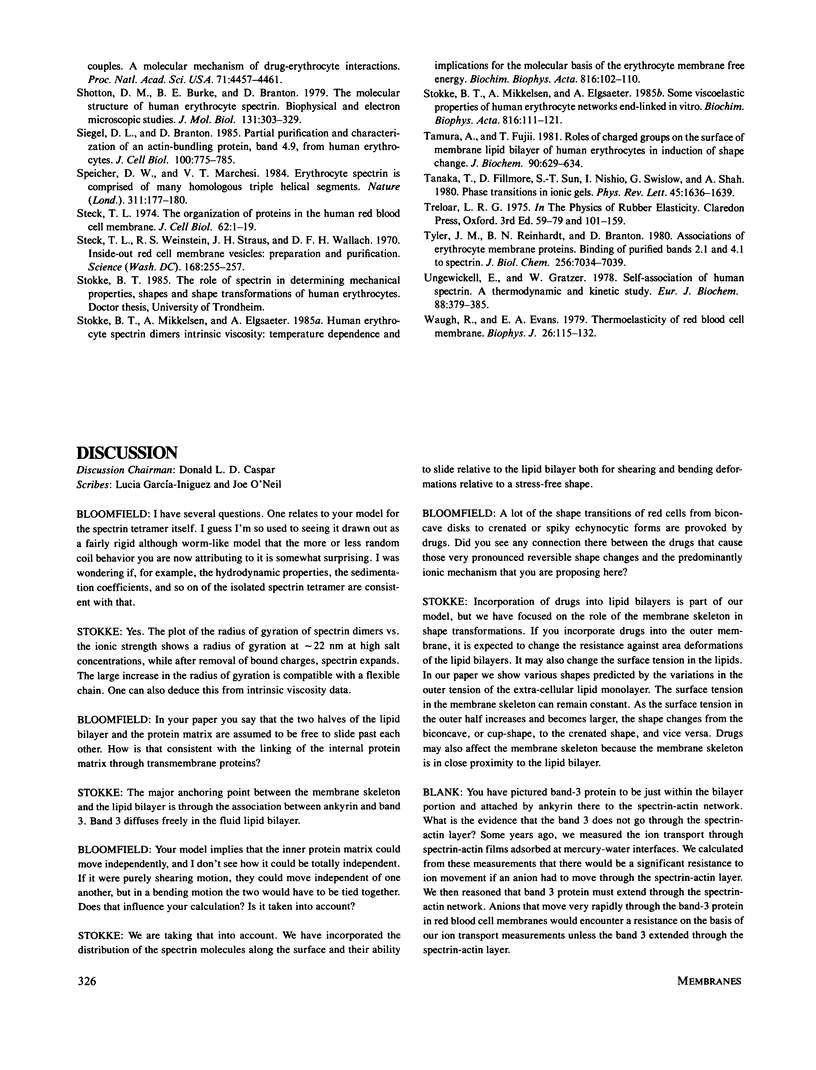
Images in this article
Selected References
These references are in PubMed. This may not be the complete list of references from this article.
- Branton D., Cohen C. M., Tyler J. Interaction of cytoskeletal proteins on the human erythrocyte membrane. Cell. 1981 Apr;24(1):24–32. doi: 10.1016/0092-8674(81)90497-9. [DOI] [PubMed] [Google Scholar]
- Canham P. B. The minimum energy of bending as a possible explanation of the biconcave shape of the human red blood cell. J Theor Biol. 1970 Jan;26(1):61–81. doi: 10.1016/s0022-5193(70)80032-7. [DOI] [PubMed] [Google Scholar]
- Chien S., Sung K. L., Skalak R., Usami S., Tözeren A. Theoretical and experimental studies on viscoelastic properties of erythrocyte membrane. Biophys J. 1978 Nov;24(2):463–487. doi: 10.1016/S0006-3495(78)85395-8. [DOI] [PMC free article] [PubMed] [Google Scholar]
- Cohen C. M., Tyler J. M., Branton D. Spectrin-actin associations studied by electron microscopy of shadowed preparations. Cell. 1980 Oct;21(3):875–883. doi: 10.1016/0092-8674(80)90451-1. [DOI] [PubMed] [Google Scholar]
- Dubreuil Y. L., Cassoly R. A dynamical study on the interactions between the cytoskeleton components in the human erythrocyte as detected by saturation transfer electron paramagnetic resonance of spin-labeled spectrin, ankyrin, and protein 4.1. Arch Biochem Biophys. 1983 Jun;223(2):495–502. doi: 10.1016/0003-9861(83)90614-8. [DOI] [PubMed] [Google Scholar]
- Elgsaeter A., Branton D. Intramembrane particle aggregation in erythrocyte ghosts. I. The effects of protein removal. J Cell Biol. 1974 Dec;63(3):1018–1036. doi: 10.1083/jcb.63.3.1018. [DOI] [PMC free article] [PubMed] [Google Scholar]
- Elgsaeter A., Shotton D. M., Branton D. Intramembrane particle aggregation in erythrocyte ghosts. II. The influence of spectrin aggregation. Biochim Biophys Acta. 1976 Feb 19;426(1):101–122. doi: 10.1016/0005-2736(76)90433-8. [DOI] [PubMed] [Google Scholar]
- Evans E. A. Bending resistance and chemically induced moments in membrane bilayers. Biophys J. 1974 Dec;14(12):923–931. doi: 10.1016/S0006-3495(74)85959-X. [DOI] [PMC free article] [PubMed] [Google Scholar]
- Evans E. A., La Celle P. L. Intrinsic material properties of the erythrocyte membrane indicated by mechanical analysis of deformation. Blood. 1975 Jan;45(1):29–43. [PubMed] [Google Scholar]
- Evans E. A. New membrane concept applied to the analysis of fluid shear- and micropipette-deformed red blood cells. Biophys J. 1973 Sep;13(9):941–954. doi: 10.1016/S0006-3495(73)86036-9. [DOI] [PMC free article] [PubMed] [Google Scholar]
- Evans E. A., Skalak R. Mechanics and thermodynamics of biomembranes: part 2. CRC Crit Rev Bioeng. 1979 Nov;3(4):331–418. [PubMed] [Google Scholar]
- Johnson R. M., Robinson J. Morphological changes in asymmetric erythrocyte membranes induced by electrolytes. Biochem Biophys Res Commun. 1976 Jun 7;70(3):925–931. doi: 10.1016/0006-291x(76)90680-x. [DOI] [PubMed] [Google Scholar]
- Kwok R., Evans E. Thermoelasticity of large lecithin bilayer vesicles. Biophys J. 1981 Sep;35(3):637–652. doi: 10.1016/S0006-3495(81)84817-5. [DOI] [PMC free article] [PubMed] [Google Scholar]
- Lange Y., Hadesman R. A., Steck T. L. Role of the reticulum in the stability and shape of the isolated human erythrocyte membrane. J Cell Biol. 1982 Mar;92(3):714–721. doi: 10.1083/jcb.92.3.714. [DOI] [PMC free article] [PubMed] [Google Scholar]
- Liu S. C., Palek J. Hemoglobin enhances the self-association of spectrin heterodimers in human erythrocytes. J Biol Chem. 1984 Sep 25;259(18):11556–11562. [PubMed] [Google Scholar]
- Liu S. C., Palek J. Spectrin tetramer-dimer equilibrium and the stability of erythrocyte membrane skeletons. Nature. 1980 Jun 19;285(5766):586–588. doi: 10.1038/285586a0. [DOI] [PubMed] [Google Scholar]
- Mikkelsen A., Stokke B. T., Elgsaeter A. An electro-optic study of human erythrocyte spectrin dimers. The presence of calcium ions does not alter spectrin flexibility. Biochim Biophys Acta. 1984 Apr 27;786(1-2):95–102. doi: 10.1016/0167-4838(84)90158-4. [DOI] [PubMed] [Google Scholar]
- Nicolson G. L. Anionic sites of human erythrocyte membranes. I. Effects of trypsin, phospholipase C, and pH on the topography of bound positively charged colloidal particles. J Cell Biol. 1973 May;57(2):373–387. doi: 10.1083/jcb.57.2.373. [DOI] [PMC free article] [PubMed] [Google Scholar]
- Nicolson G. L., Marchesi V. T., Singer S. J. The localization of spectrin on the inner surface of human red blood cell membranes by ferritin-conjugated antibodies. J Cell Biol. 1971 Oct;51(1):265–272. doi: 10.1083/jcb.51.1.265. [DOI] [PMC free article] [PubMed] [Google Scholar]
- Papahadjopoulos D. Surface properties of acidic phospholipids: interaction of monolayers and hydrated liquid crystals with uni- and bi-valent metal ions. Biochim Biophys Acta. 1968 Sep 17;163(2):240–254. doi: 10.1016/0005-2736(68)90103-x. [DOI] [PubMed] [Google Scholar]
- Pinto da Silva P. Translational mobility of the membrane intercalated particles of human erythrocyte ghosts. pH-dependent, reversible aggregation. J Cell Biol. 1972 Jun;53(3):777–787. doi: 10.1083/jcb.53.3.777. [DOI] [PMC free article] [PubMed] [Google Scholar]
- Schanus E., Booth S., Hallaway B., Rosenberg A. The elasticity of spectrin-actin gels at high protein concentration. J Biol Chem. 1985 Mar 25;260(6):3724–3730. [PubMed] [Google Scholar]
- Sheetz M. P., Singer S. J. Biological membranes as bilayer couples. A molecular mechanism of drug-erythrocyte interactions. Proc Natl Acad Sci U S A. 1974 Nov;71(11):4457–4461. doi: 10.1073/pnas.71.11.4457. [DOI] [PMC free article] [PubMed] [Google Scholar]
- Shotton D. M., Burke B. E., Branton D. The molecular structure of human erythrocyte spectrin. Biophysical and electron microscopic studies. J Mol Biol. 1979 Jun 25;131(2):303–329. doi: 10.1016/0022-2836(79)90078-0. [DOI] [PubMed] [Google Scholar]
- Siegel D. L., Branton D. Partial purification and characterization of an actin-bundling protein, band 4.9, from human erythrocytes. J Cell Biol. 1985 Mar;100(3):775–785. doi: 10.1083/jcb.100.3.775. [DOI] [PMC free article] [PubMed] [Google Scholar]
- Speicher D. W., Marchesi V. T. Erythrocyte spectrin is comprised of many homologous triple helical segments. Nature. 1984 Sep 13;311(5982):177–180. doi: 10.1038/311177a0. [DOI] [PubMed] [Google Scholar]
- Steck T. L. The organization of proteins in the human red blood cell membrane. A review. J Cell Biol. 1974 Jul;62(1):1–19. doi: 10.1083/jcb.62.1.1. [DOI] [PMC free article] [PubMed] [Google Scholar]
- Steck T. L., Weinstein R. S., Straus J. H., Wallach D. F. Inside-out red cell membrane vesicles: preparation and purification. Science. 1970 Apr 10;168(3928):255–257. doi: 10.1126/science.168.3928.255. [DOI] [PubMed] [Google Scholar]
- Stokke B. T., Mikkelsen A., Elgsaeter A. Human erythrocyte spectrin dimer intrinsic viscosity: temperature dependence and implications for the molecular basis of the erythrocyte membrane free energy. Biochim Biophys Acta. 1985 Jun 11;816(1):102–110. doi: 10.1016/0005-2736(85)90398-0. [DOI] [PubMed] [Google Scholar]
- Stokke B. T., Mikkelsen A., Elgsaeter A. Some viscoelastic properties of human erythrocyte spectrin networks end-linked in vitro. Biochim Biophys Acta. 1985 Jun 11;816(1):111–121. doi: 10.1016/0005-2736(85)90399-2. [DOI] [PubMed] [Google Scholar]
- Tamura A., Fujii T. Roles of charged groups on the surface of membrane lipid bilayer of human erythrocytes in induction of shape change. J Biochem. 1981 Sep;90(3):629–634. doi: 10.1093/oxfordjournals.jbchem.a133516. [DOI] [PubMed] [Google Scholar]
- Tyler J. M., Reinhardt B. N., Branton D. Associations of erythrocyte membrane proteins. Binding of purified bands 2.1 and 4.1 to spectrin. J Biol Chem. 1980 Jul 25;255(14):7034–7039. [PubMed] [Google Scholar]
- Ungewickell E., Gratzer W. Self-association of human spectrin. A thermodynamic and kinetic study. Eur J Biochem. 1978 Aug 1;88(2):379–385. doi: 10.1111/j.1432-1033.1978.tb12459.x. [DOI] [PubMed] [Google Scholar]
- Waugh R., Evans E. A. Thermoelasticity of red blood cell membrane. Biophys J. 1979 Apr;26(1):115–131. doi: 10.1016/S0006-3495(79)85239-X. [DOI] [PMC free article] [PubMed] [Google Scholar]