Abstract
We subjected single skeletal muscle cells from frog semitendinosus to sinusoidal oscillations that simulated the strain experienced as the cells near the end of passive extension and begin active contraction in slow swimming. Other cells from which the basement membrane was removed by enzymatic and mechanical procedures were tested identically. Effectiveness of the basement membrane removal technique was evaluated by electron microscopy, by an electrophoretic and lectin-binding assay for depletion of cell surface glycoproteins, and by confirmation by means of electrophoretic and immunologic analyses that major intracellular, cytoskeletal proteins were not disrupted. Measurements of maximum stress, maximum strain, and phase lag between these maxima enabled the complex modulus (dynamic stiffness) and loss tangent (relative viscous losses to elastic energy storage) to be calculated for each mechanically tested preparation. We also calculated the amounts of energy stored and dissipated in each preparation. These calculations indicate that cells with intact basement membranes have complex moduli significantly greater than those of cells without basement membranes, and that cells with basement membrane store significantly more elastic energy than basement membrane depleted cells. However, when subjected to identical sinusoidal strains, energy dissipation in cells with intact basement membranes is over three times greater than dissipation in cells without basement membrane. The relative magnitudes of energy losses to energy storage, called the specific loss, is nearly three times greater for intact cells than for basement membrane depleted cells. Basement membranes may thereby serve as a brake for slowing passive extension of muscle before contraction begins.
Full text
PDF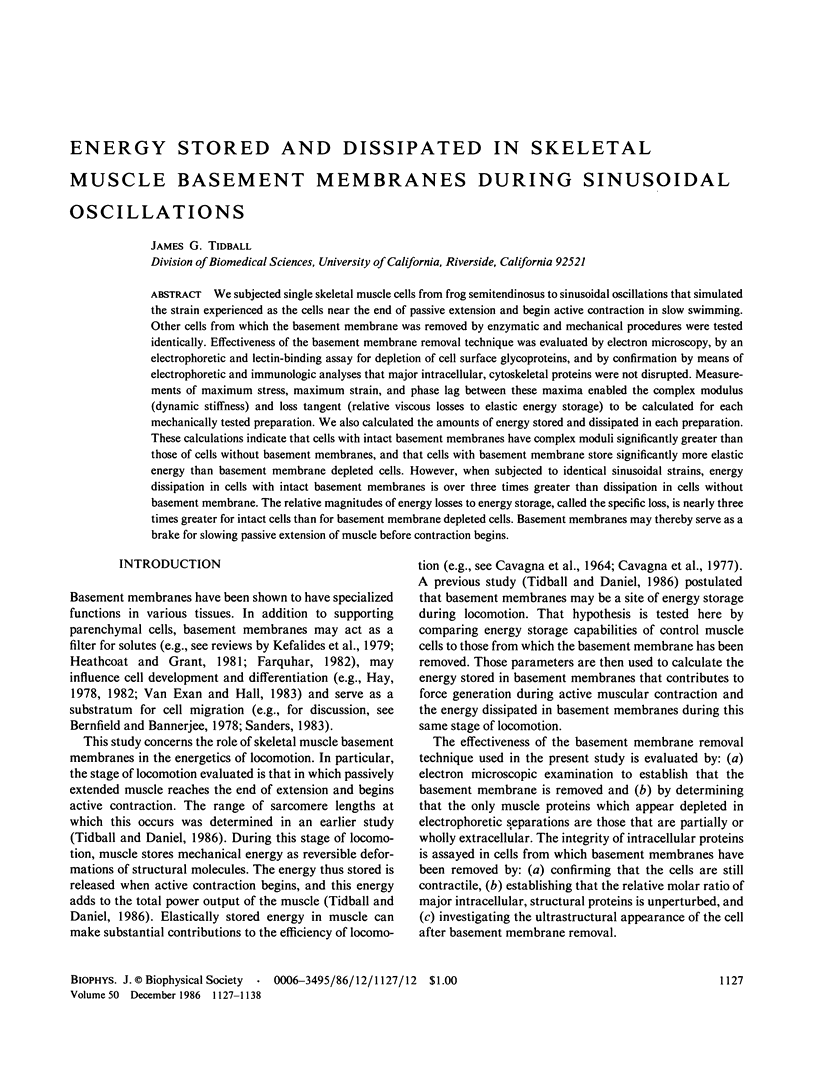
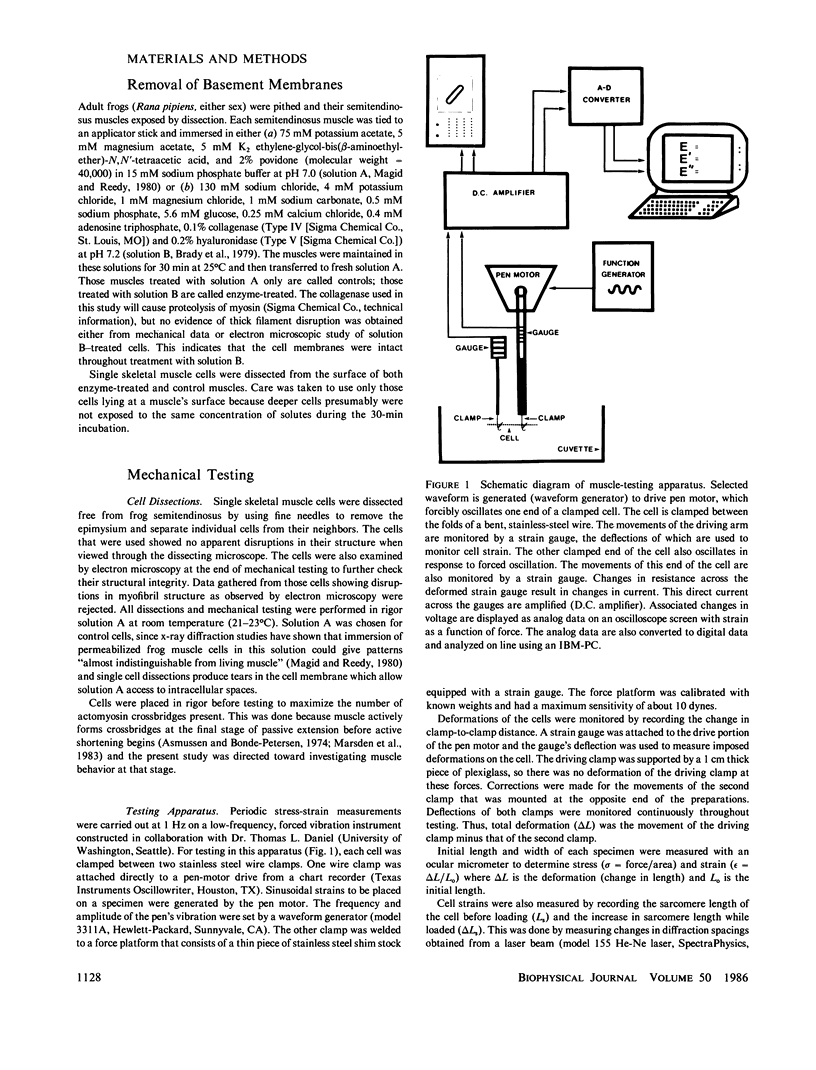
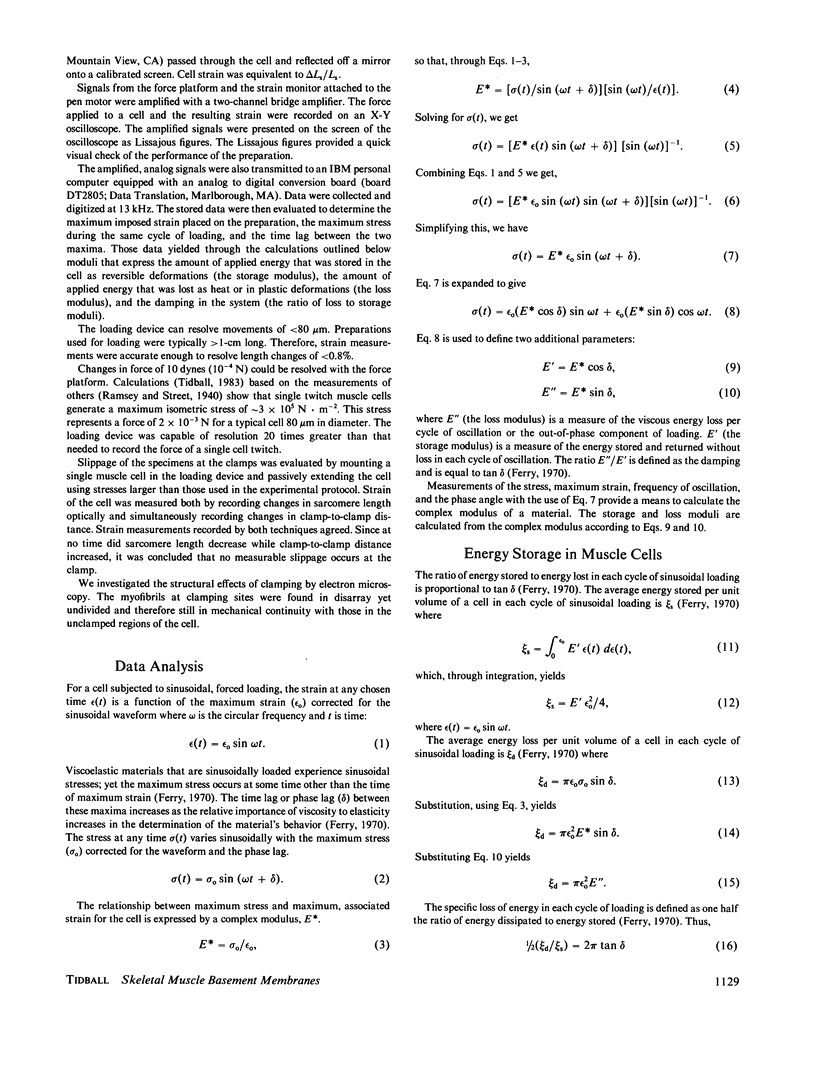
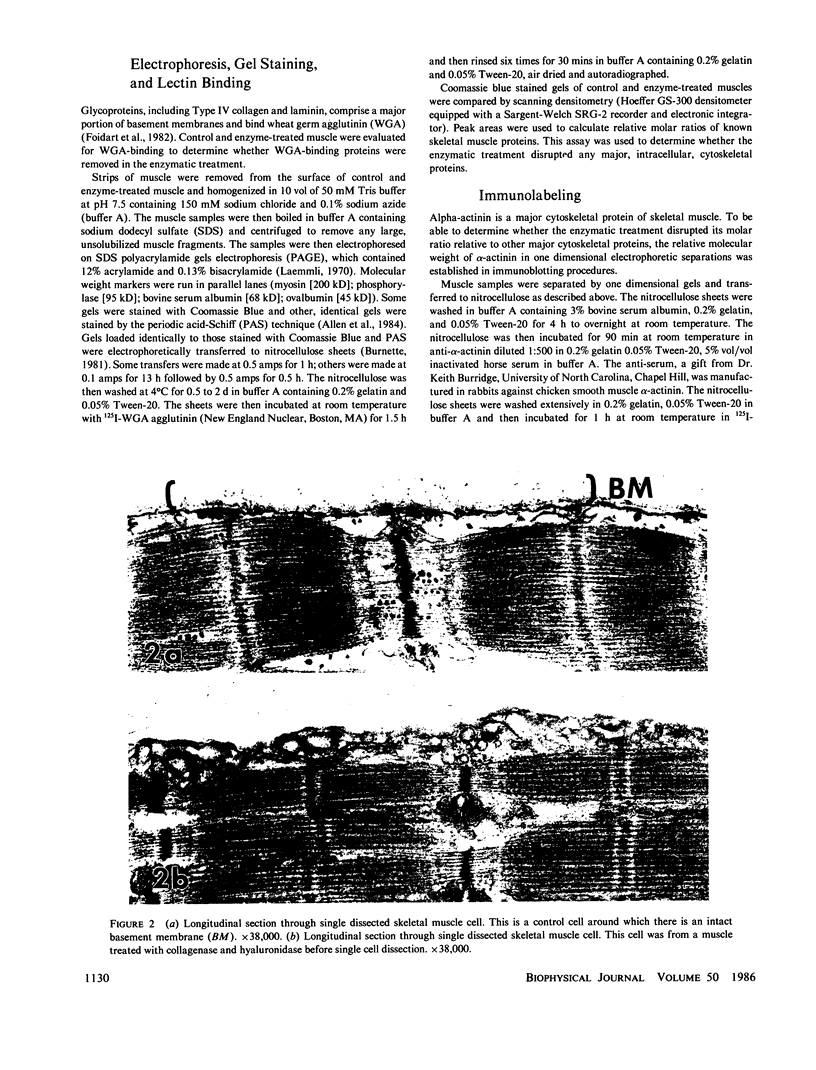
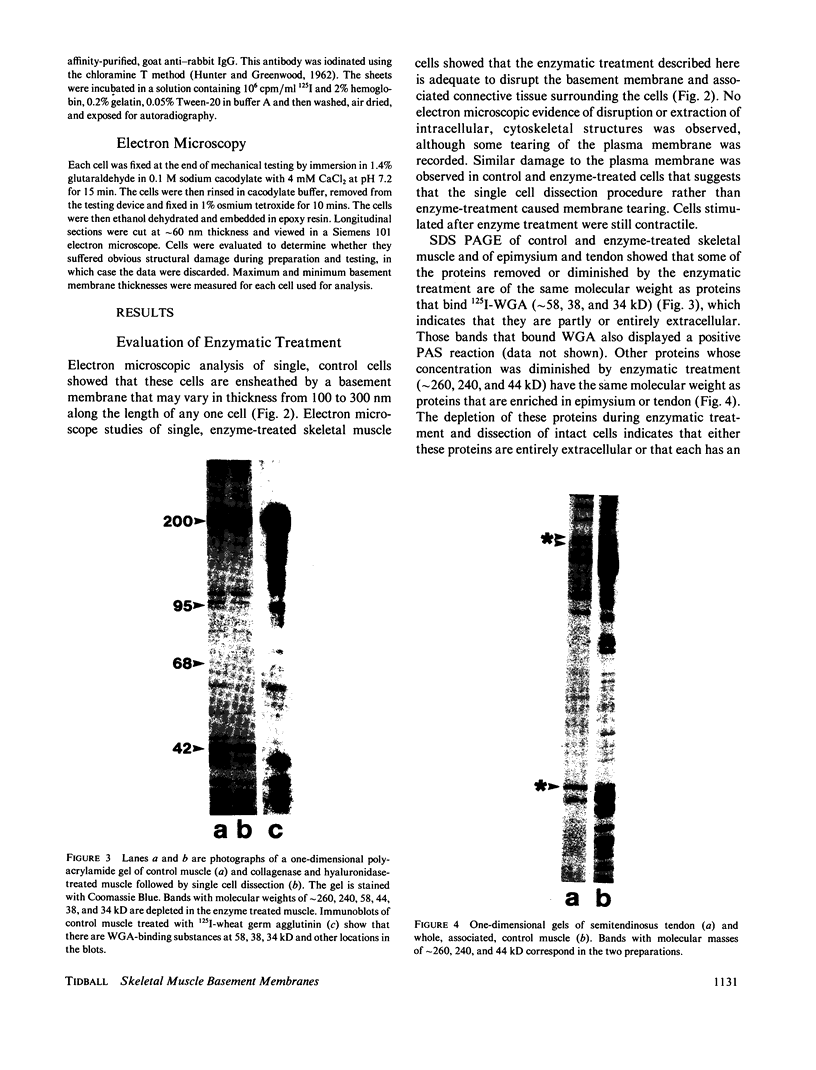
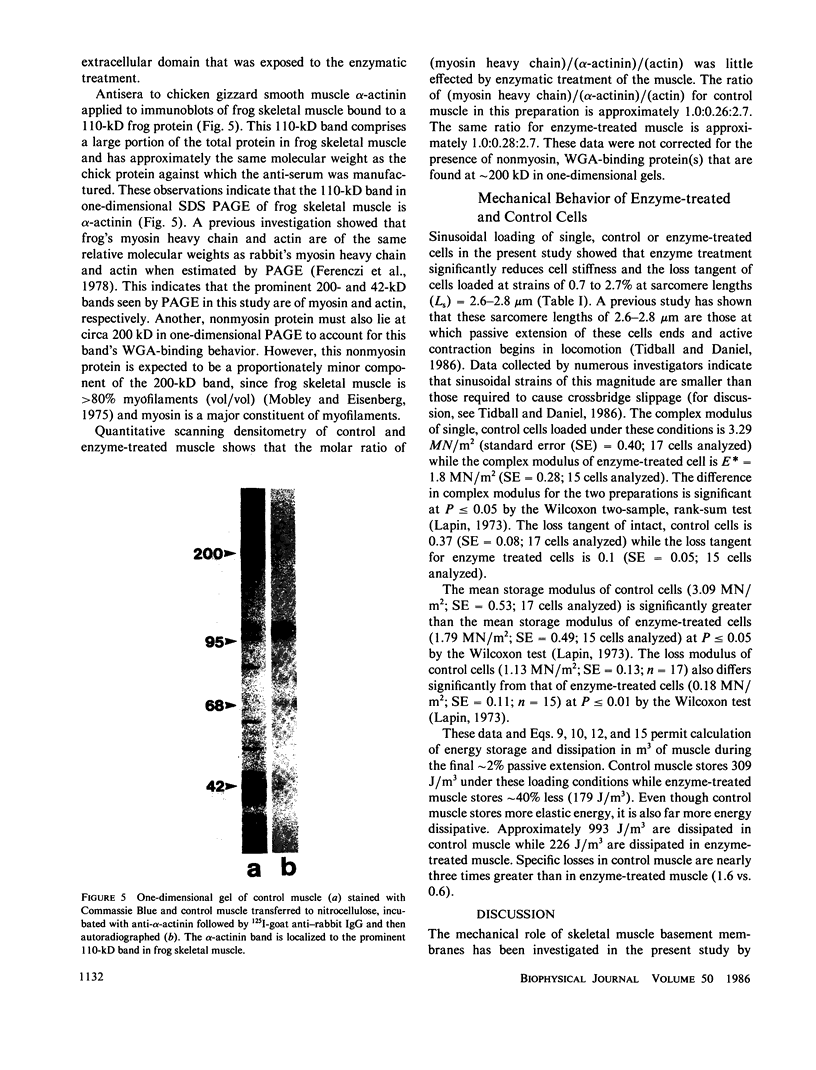
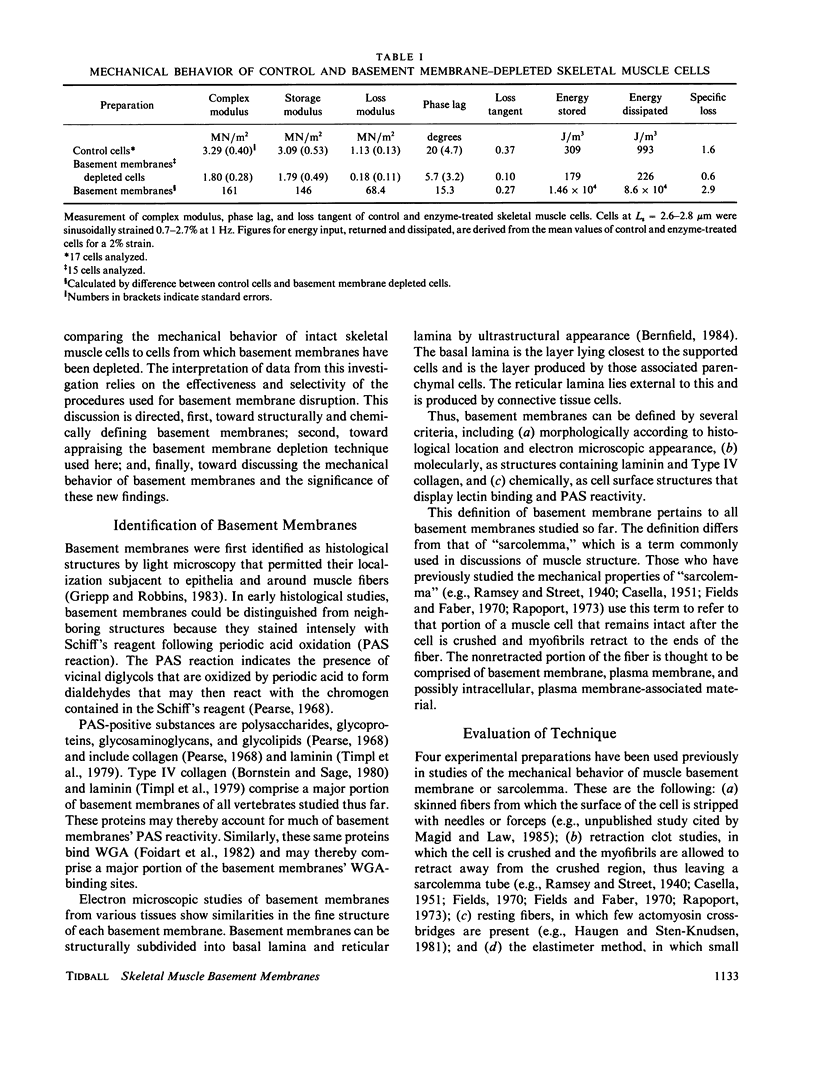
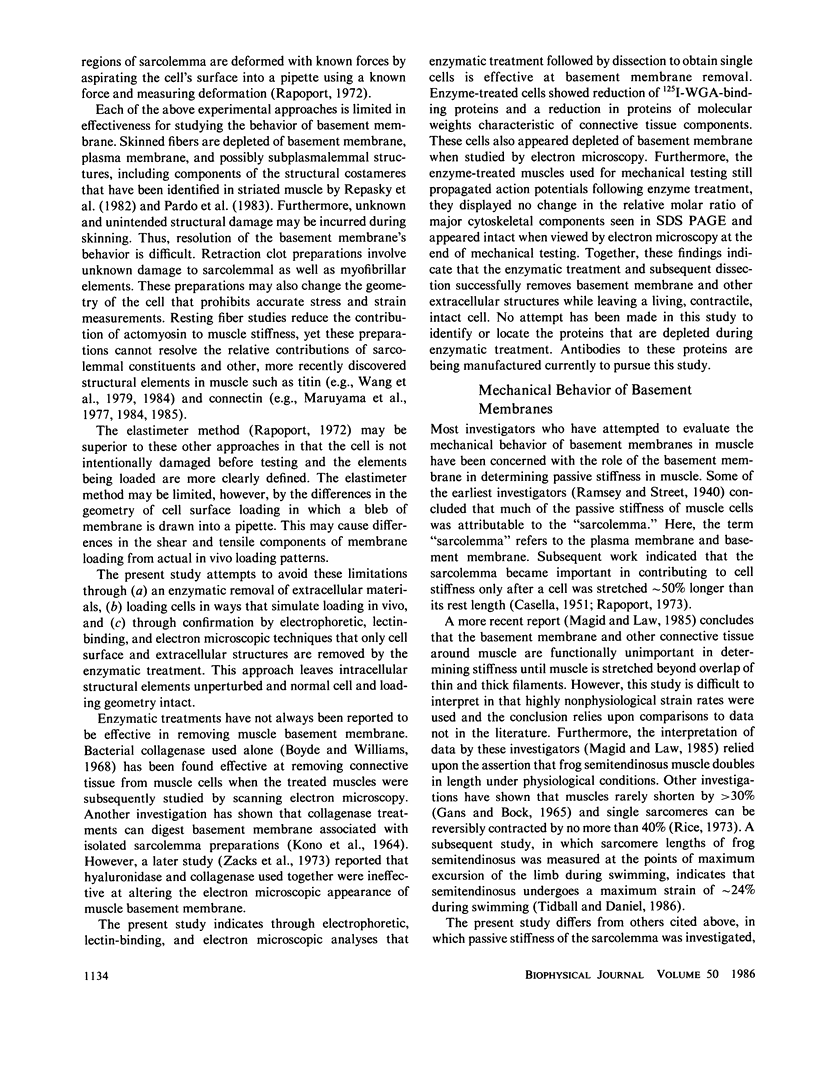
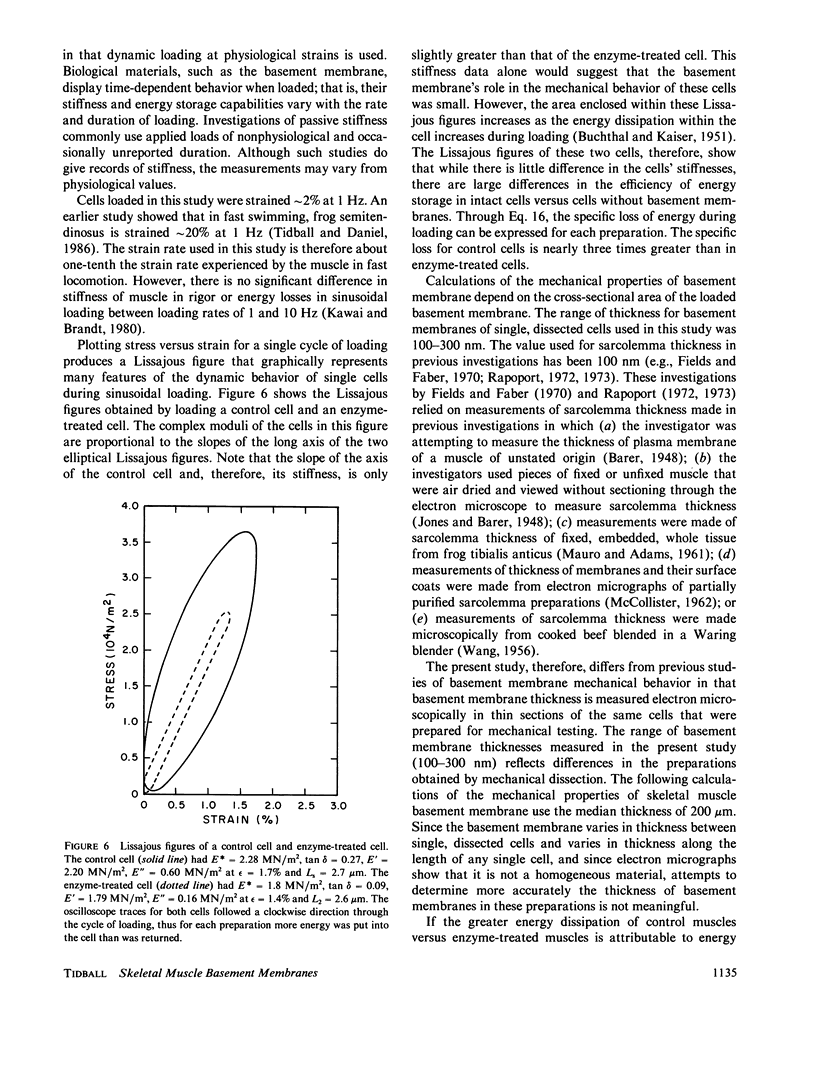
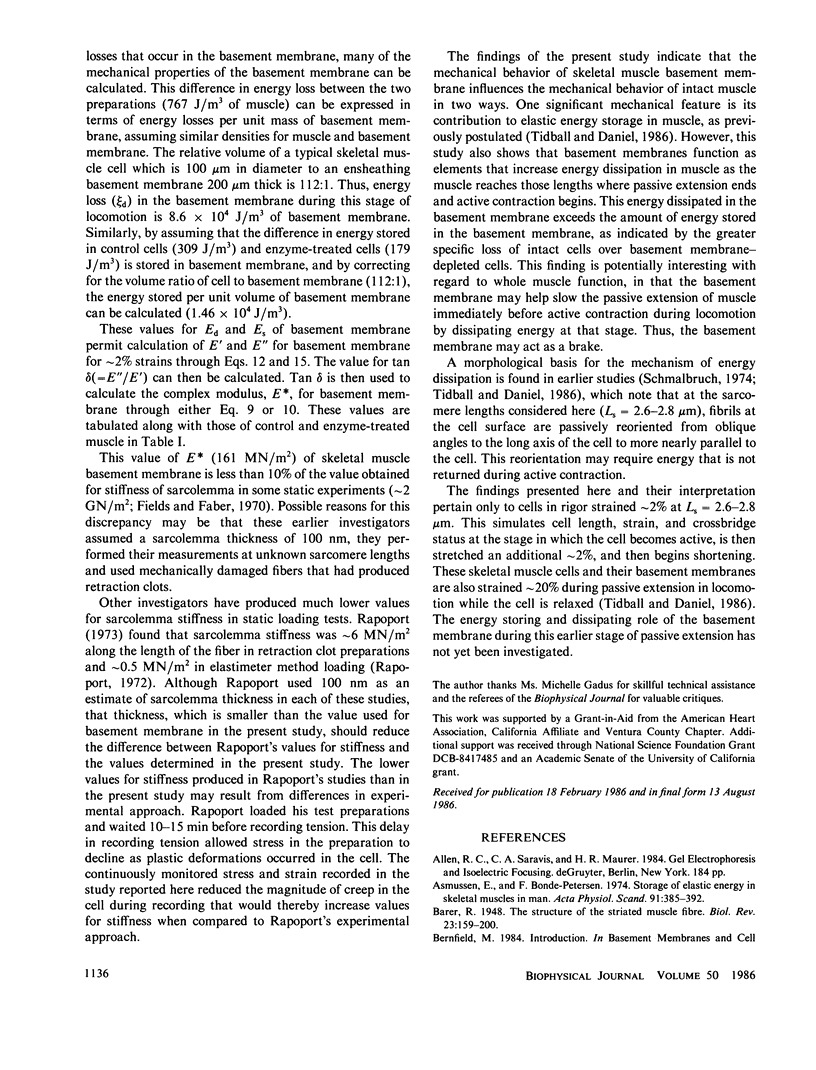
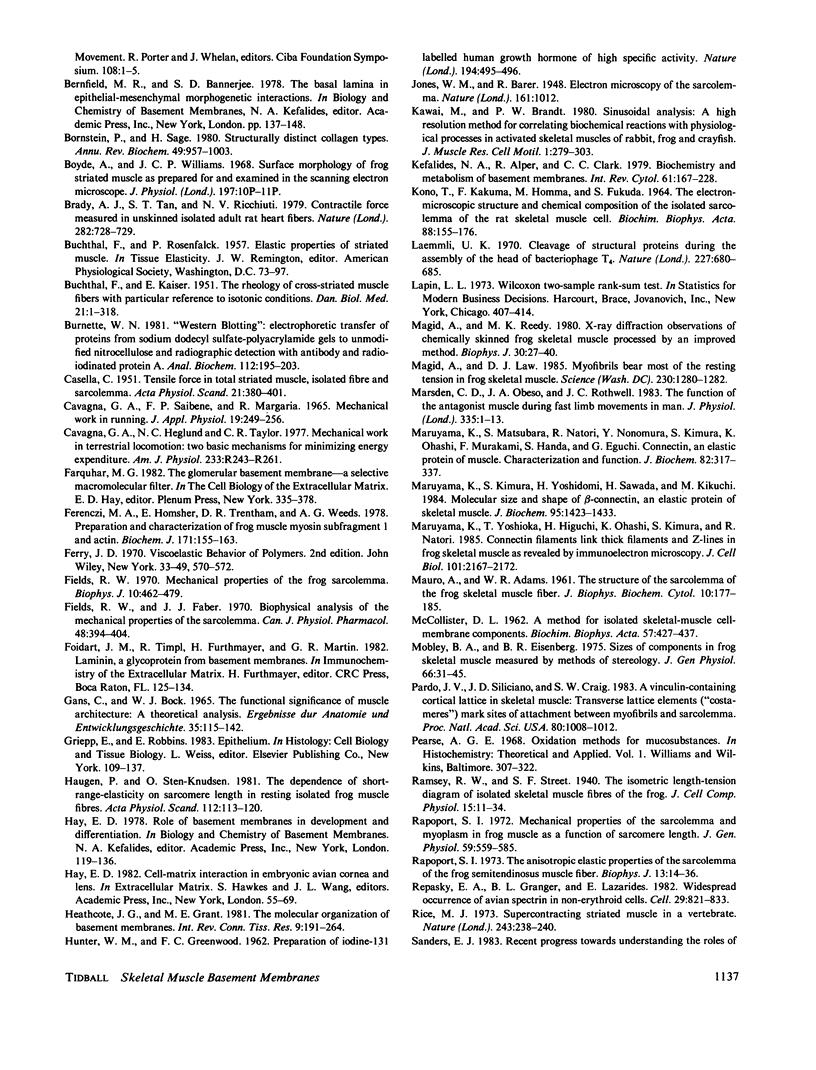
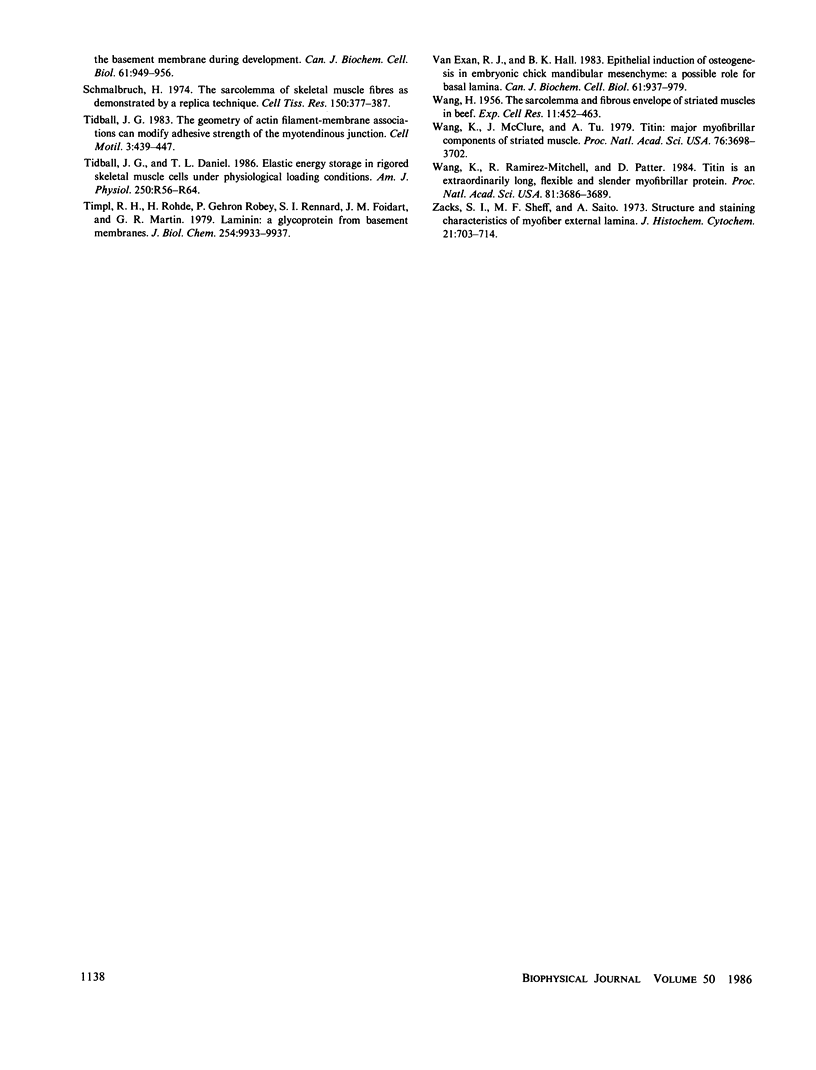
Images in this article
Selected References
These references are in PubMed. This may not be the complete list of references from this article.
- Asmussen E., Bonde-Petersen F. Storage of elastic energy in skeletal muscles in man. Acta Physiol Scand. 1974 Jul;91(3):385–392. doi: 10.1111/j.1748-1716.1974.tb05693.x. [DOI] [PubMed] [Google Scholar]
- BARER R. The structure of the striated muscle fibre. Biol Rev Camb Philos Soc. 1948 Apr;23(2):159–200. doi: 10.1111/j.1469-185x.1948.tb00461.x. [DOI] [PubMed] [Google Scholar]
- Bornstein P., Sage H. Structurally distinct collagen types. Annu Rev Biochem. 1980;49:957–1003. doi: 10.1146/annurev.bi.49.070180.004521. [DOI] [PubMed] [Google Scholar]
- Brady A. J., Tan S. T., Ricchiuti N. V. Contractile force measured in unskinned isolated adult rat heart fibres. Nature. 1979 Dec 13;282(5740):728–729. doi: 10.1038/282728a0. [DOI] [PubMed] [Google Scholar]
- Burnette W. N. "Western blotting": electrophoretic transfer of proteins from sodium dodecyl sulfate--polyacrylamide gels to unmodified nitrocellulose and radiographic detection with antibody and radioiodinated protein A. Anal Biochem. 1981 Apr;112(2):195–203. doi: 10.1016/0003-2697(81)90281-5. [DOI] [PubMed] [Google Scholar]
- CASELLA C. Tensile force in total striated muscle, isolated fibre and sarcolemma. Acta Physiol Scand. 1950 Dec;21(4):380–401. doi: 10.1111/j.1748-1716.1950.tb00744.x. [DOI] [PubMed] [Google Scholar]
- CAVAGNA G. A., SAIBENE F. P., MARGARIA R. MECHANICAL WORK IN RUNNING. J Appl Physiol. 1964 Mar;19:249–256. doi: 10.1152/jappl.1964.19.2.249. [DOI] [PubMed] [Google Scholar]
- Cavagna G. A., Heglund N. C., Taylor C. R. Mechanical work in terrestrial locomotion: two basic mechanisms for minimizing energy expenditure. Am J Physiol. 1977 Nov;233(5):R243–R261. doi: 10.1152/ajpregu.1977.233.5.R243. [DOI] [PubMed] [Google Scholar]
- Ferenczi M. A., Homsher E., Trentham D. R., Weeds A. G. Preparation and characterization of frog muscle myosin subfragment 1 and actin. Biochem J. 1978 Apr 1;171(1):155–163. doi: 10.1042/bj1710155. [DOI] [PMC free article] [PubMed] [Google Scholar]
- Fields R. W., Faber J. J. Biophysical analysis of the mechanical properties of the sarcolemma. Can J Physiol Pharmacol. 1970 Jun;48(6):394–404. doi: 10.1139/y70-062. [DOI] [PubMed] [Google Scholar]
- Fields R. W. Mechanical properties of the frog sarcolemma. Biophys J. 1970 May;10(5):462–479. doi: 10.1016/S0006-3495(70)86312-3. [DOI] [PMC free article] [PubMed] [Google Scholar]
- Gans C., Bock W. J. The functional significance of muscle architecture--a theoretical analysis. Ergeb Anat Entwicklungsgesch. 1965;38:115–142. [PubMed] [Google Scholar]
- HUNTER W. M., GREENWOOD F. C. Preparation of iodine-131 labelled human growth hormone of high specific activity. Nature. 1962 May 5;194:495–496. doi: 10.1038/194495a0. [DOI] [PubMed] [Google Scholar]
- Haugen P., Sten-Knudsen O. The dependence of the short-range elasticity on sarcomere length in resting isolated frog muscle fibres. Acta Physiol Scand. 1981 Jun;112(2):113–120. doi: 10.1111/j.1748-1716.1981.tb06793.x. [DOI] [PubMed] [Google Scholar]
- Heathcote J. G., Grant M. E. The molecular organization of basement membranes. Int Rev Connect Tissue Res. 1981;9:191–264. doi: 10.1016/b978-0-12-363709-3.50011-5. [DOI] [PubMed] [Google Scholar]
- JONES W. M., BARER R. Electron microscopy of the sarcolemma. Nature. 1948 Jun 26;161(4104):1012–1012. doi: 10.1038/1611012a0. [DOI] [PubMed] [Google Scholar]
- KONO T., KAKUMA F., HOMMA M., FUKUDA S. THE ELECTRON-MICROSCOPIC STRUCTURE AND CHEMICAL COMPOSITION OF THE ISOLATED SARCOLEMMA OF THE RAT SKELETAL MUSCLE CELL. Biochim Biophys Acta. 1964 Jul 29;88:155–176. doi: 10.1016/0926-6577(64)90163-9. [DOI] [PubMed] [Google Scholar]
- Kawai M., Brandt P. W. Sinusoidal analysis: a high resolution method for correlating biochemical reactions with physiological processes in activated skeletal muscles of rabbit, frog and crayfish. J Muscle Res Cell Motil. 1980 Sep;1(3):279–303. doi: 10.1007/BF00711932. [DOI] [PubMed] [Google Scholar]
- Kefalides N. A., Alper R., Clark C. C. Biochemistry and metabolism of basement membranes. Int Rev Cytol. 1979;61:167–228. doi: 10.1016/s0074-7696(08)61998-1. [DOI] [PubMed] [Google Scholar]
- Laemmli U. K. Cleavage of structural proteins during the assembly of the head of bacteriophage T4. Nature. 1970 Aug 15;227(5259):680–685. doi: 10.1038/227680a0. [DOI] [PubMed] [Google Scholar]
- MAURO A., ADAMS W. R. The structure of the sarcolemma of the frog skeletal muscle fiber. J Biophys Biochem Cytol. 1961 Aug;10(4):177–185. doi: 10.1083/jcb.10.4.177. [DOI] [PMC free article] [PubMed] [Google Scholar]
- Magid A., Law D. J. Myofibrils bear most of the resting tension in frog skeletal muscle. Science. 1985 Dec 13;230(4731):1280–1282. doi: 10.1126/science.4071053. [DOI] [PubMed] [Google Scholar]
- Magid A., Reedy M. K. X-ray diffraction observations of chemically skinned frog skeletal muscle processed by an improved method. Biophys J. 1980 Apr;30(1):27–40. doi: 10.1016/S0006-3495(80)85074-0. [DOI] [PMC free article] [PubMed] [Google Scholar]
- Maruyama K., Kimura S., Yoshidomi H., Sawada H., Kikuchi M. Molecular size and shape of beta-connectin, an elastic protein of striated muscle. J Biochem. 1984 May;95(5):1423–1433. doi: 10.1093/oxfordjournals.jbchem.a134750. [DOI] [PubMed] [Google Scholar]
- Maruyama K., Yoshioka T., Higuchi H., Ohashi K., Kimura S., Natori R. Connectin filaments link thick filaments and Z lines in frog skeletal muscle as revealed by immunoelectron microscopy. J Cell Biol. 1985 Dec;101(6):2167–2172. doi: 10.1083/jcb.101.6.2167. [DOI] [PMC free article] [PubMed] [Google Scholar]
- Mobley B. A., Eisenberg B. R. Sizes of components in frog skeletal muscle measured by methods of stereology. J Gen Physiol. 1975 Jul;66(1):31–45. doi: 10.1085/jgp.66.1.31. [DOI] [PMC free article] [PubMed] [Google Scholar]
- Pardo J. V., Siliciano J. D., Craig S. W. A vinculin-containing cortical lattice in skeletal muscle: transverse lattice elements ("costameres") mark sites of attachment between myofibrils and sarcolemma. Proc Natl Acad Sci U S A. 1983 Feb;80(4):1008–1012. doi: 10.1073/pnas.80.4.1008. [DOI] [PMC free article] [PubMed] [Google Scholar]
- Rapoport S. I. Mechanical properties of the sarcolemma and myoplasm in frog muscle as a function of sarcomere length. J Gen Physiol. 1972 May;59(5):559–585. doi: 10.1085/jgp.59.5.559. [DOI] [PMC free article] [PubMed] [Google Scholar]
- Rapoport S. I. The anisotropic elastic properties of the sarcolemma of the frog semitendinosus muscle fiber. Biophys J. 1973 Jan;13(1):14–36. doi: 10.1016/S0006-3495(73)85967-3. [DOI] [PMC free article] [PubMed] [Google Scholar]
- Repasky E. A., Granger B. L., Lazarides E. Widespread occurrence of avian spectrin in nonerythroid cells. Cell. 1982 Jul;29(3):821–833. doi: 10.1016/0092-8674(82)90444-5. [DOI] [PubMed] [Google Scholar]
- Rice M. J. Supercontracting striated muscle in a vertebrate. Nature. 1973 May 25;243(5404):238–240. doi: 10.1038/243238a0. [DOI] [PubMed] [Google Scholar]
- Sanders E. J. Recent progress towards understanding the roles of the basement membrane in development. Can J Biochem Cell Biol. 1983 Aug;61(8):949–956. doi: 10.1139/o83-121. [DOI] [PubMed] [Google Scholar]
- Schmalbruch H. The sarcolemma of skeletal muscle fibres as demonstrated by a replica technique. Cell Tissue Res. 1974;150(3):377–387. doi: 10.1007/BF00220144. [DOI] [PubMed] [Google Scholar]
- Tidball J. G., Daniel T. L. Elastic energy storage in rigored skeletal muscle cells under physiological loading conditions. Am J Physiol. 1986 Jan;250(1 Pt 2):R56–R64. doi: 10.1152/ajpregu.1986.250.1.R56. [DOI] [PubMed] [Google Scholar]
- Tidball J. G. The geometry of actin filament-membrane associations can modify adhesive strength of the myotendinous junction. Cell Motil. 1983;3(5-6):439–447. doi: 10.1002/cm.970030512. [DOI] [PubMed] [Google Scholar]
- Timpl R., Rohde H., Robey P. G., Rennard S. I., Foidart J. M., Martin G. R. Laminin--a glycoprotein from basement membranes. J Biol Chem. 1979 Oct 10;254(19):9933–9937. [PubMed] [Google Scholar]
- Van Exan R. J., Hall B. K. Epithelial induction of osteogenesis in embryonic chick mandibular mesenchyme: a possible role for basal lamina. Can J Biochem Cell Biol. 1983 Aug;61(8):967–979. doi: 10.1139/o83-123. [DOI] [PubMed] [Google Scholar]
- WANG H. The sarcolemma and fibrous envelope of striated muscles in beef. Exp Cell Res. 1956 Aug;11(2):452–463. doi: 10.1016/0014-4827(56)90121-5. [DOI] [PubMed] [Google Scholar]
- Wang K., McClure J., Tu A. Titin: major myofibrillar components of striated muscle. Proc Natl Acad Sci U S A. 1979 Aug;76(8):3698–3702. doi: 10.1073/pnas.76.8.3698. [DOI] [PMC free article] [PubMed] [Google Scholar]
- Wang K., Ramirez-Mitchell R., Palter D. Titin is an extraordinarily long, flexible, and slender myofibrillar protein. Proc Natl Acad Sci U S A. 1984 Jun;81(12):3685–3689. doi: 10.1073/pnas.81.12.3685. [DOI] [PMC free article] [PubMed] [Google Scholar]
- Zacks S. I., Sheff M. F., Saito A. Structure and staining characteristics of myofiber external lamina. J Histochem Cytochem. 1973 Aug;21(8):703–714. doi: 10.1177/21.8.703. [DOI] [PubMed] [Google Scholar]