Abstract
Time-resolved neutron diffraction on retinal rod outer segments are performed to reinvestigate the origin of the light-induced structural change observed by Saibil et al. (Saibil, H., M. Chabre, and D. L. Worcester, 1976, Nature (Lond.), 262:266-270). Photoactivating rhodopsin triggers in rods a cascade of GTP-dependent and transducin-mediated reactions controlling cyclic-GMP hydrolysis. Infrared light-scattering studies (Kühn, H., N. Bennett, M. Michel-Villaz, and M. Chabre, 1981, Proc. Natl. Acad. Sci. USA, 78:6873-6877; Vuong, T. M., M. Chabre, and L. Stryer, 1984, Nature (Lond.), 311:659-661) demonstrated the existence of structural changes that correspond to this cascade rather than to rhodopsin photoactivation. We thus look for neutron diffraction changes of similar origins. With 1-min time resolution, intensity changes are observed mainly for orders 2 and 4. The illumination and GTP dependence of these changes indicates an involvement of transducin. Without GTP, they are linear with the amount of photoexcited rhodopsin, saturate at 10% photolysis, and thus correlate well with the light-scattering "binding signal." With GTP, light sensitivity is higher and saturation occurs below 0.5% photolysis, as for the "dissociation signal" of light scattering. In both cases, lattice compressions of 0.2-0.3% are observed. With 4-s time resolution the intensity change with GTP present precedes the lattice compression. The fast intensity change is probably due to the displacement of transducin alpha-subunits away from the disc membrane and the slower lattice shrinkage to an osmotic readjustment of the rod.
Full text
PDF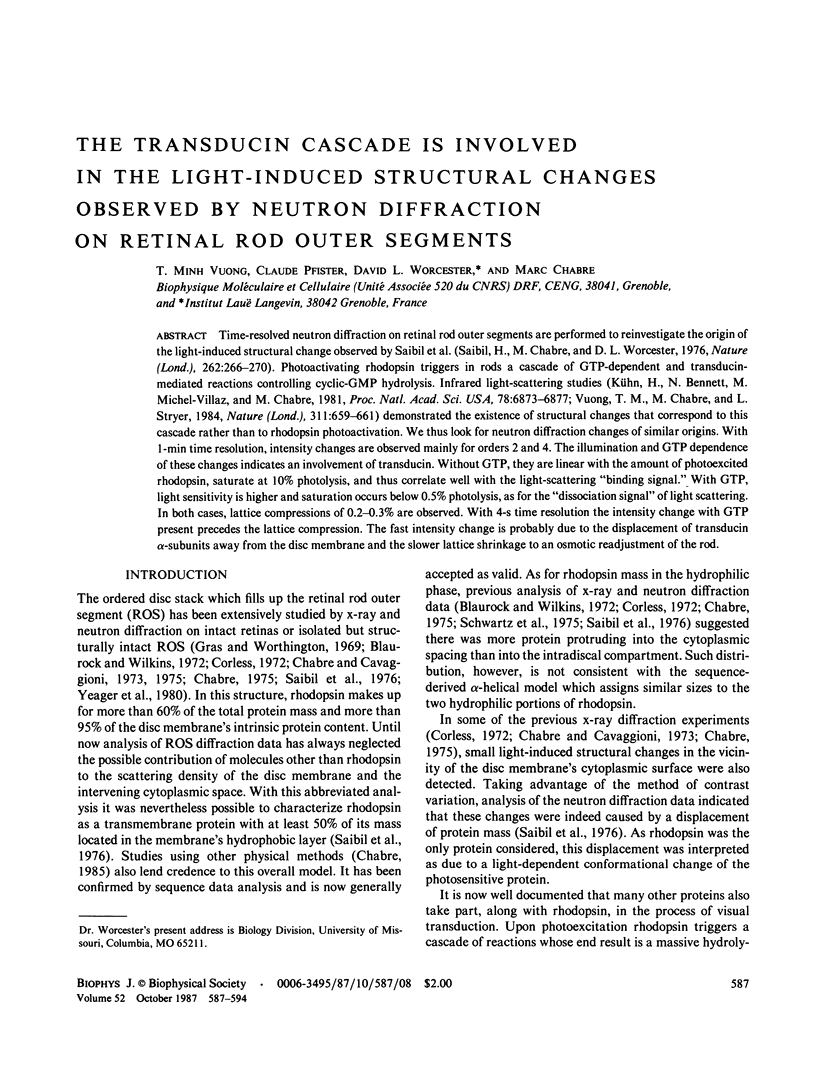
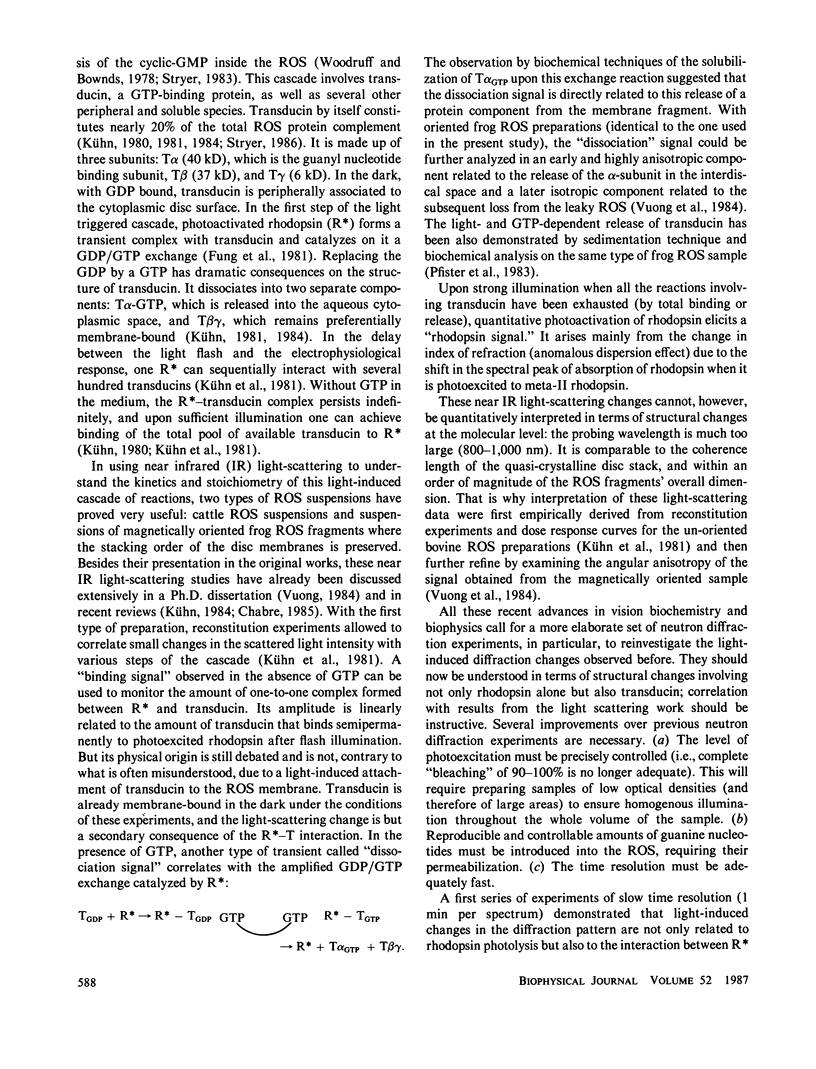
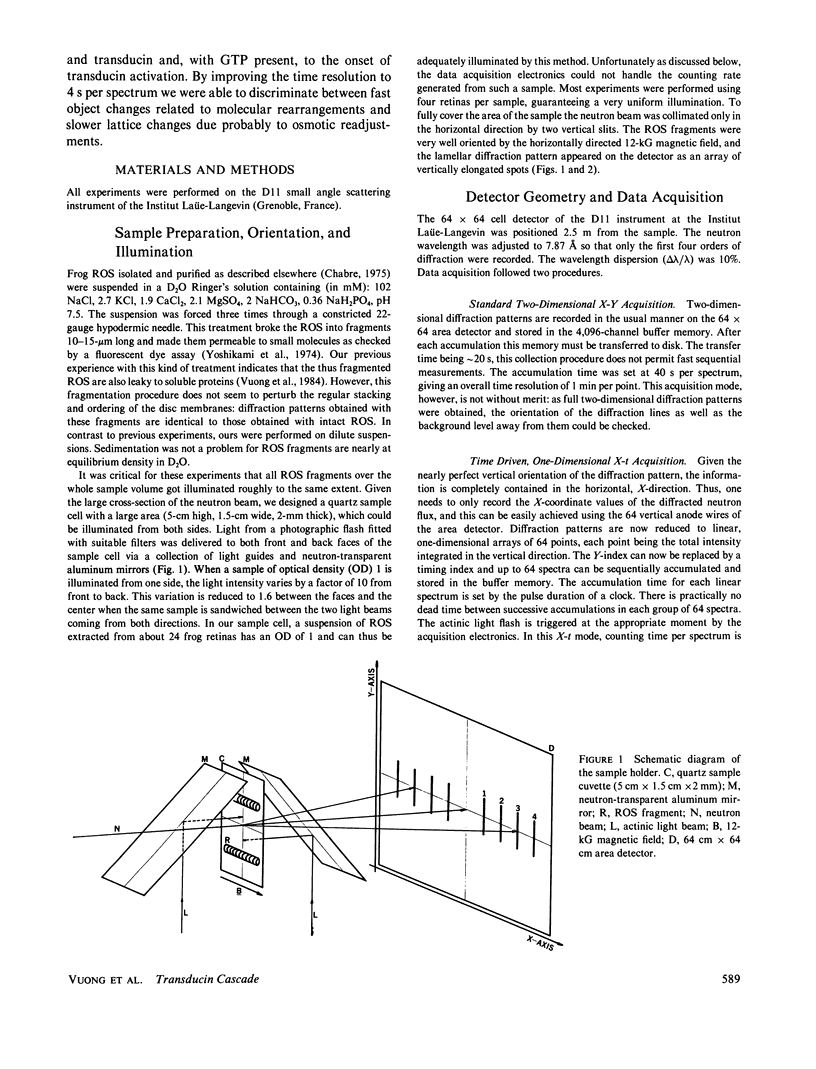
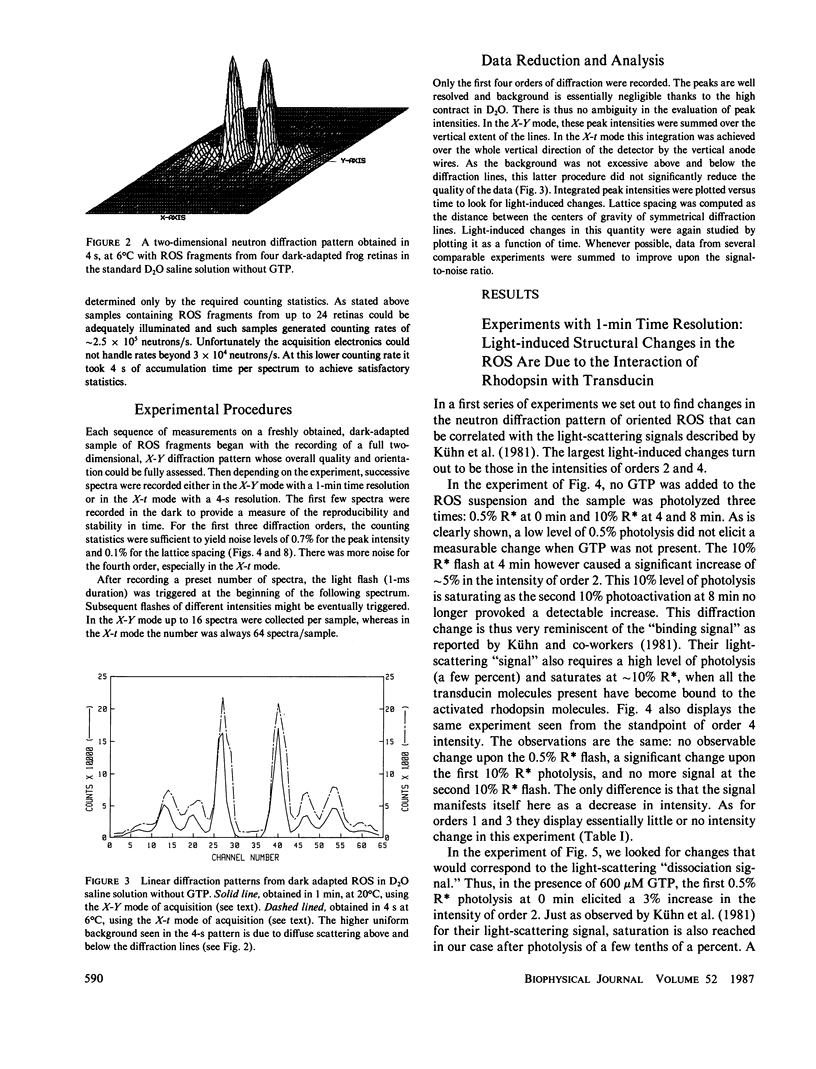
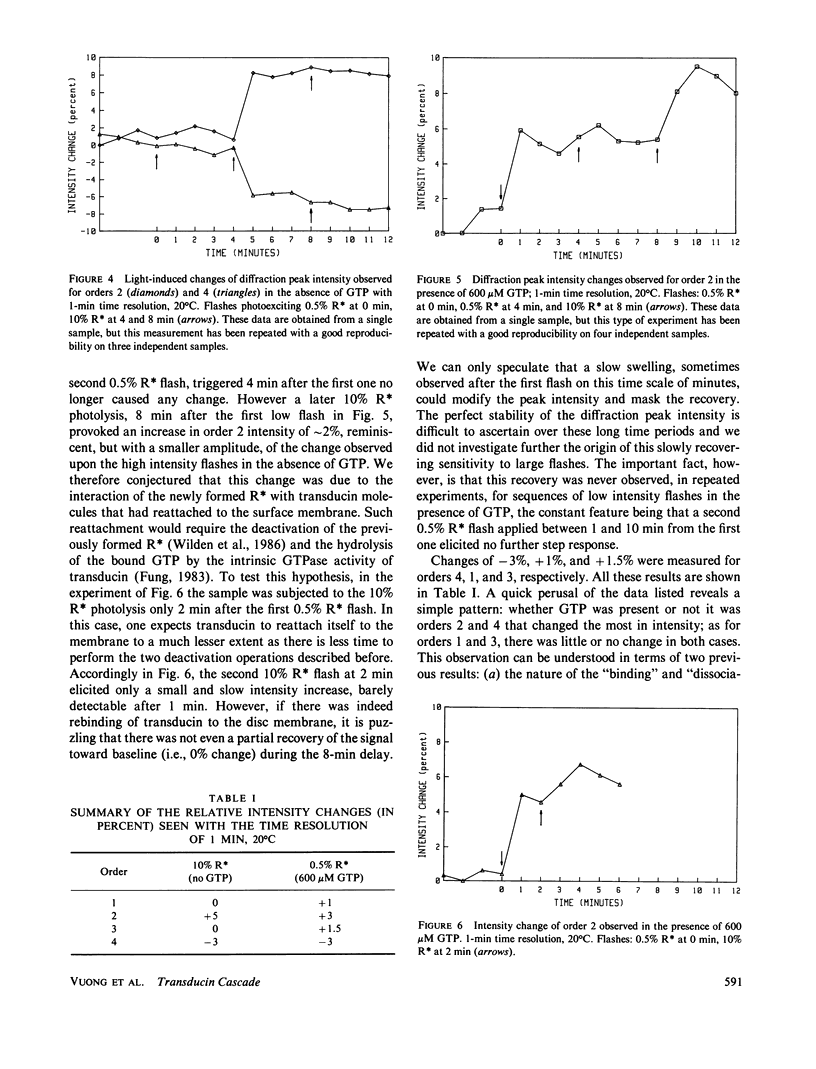
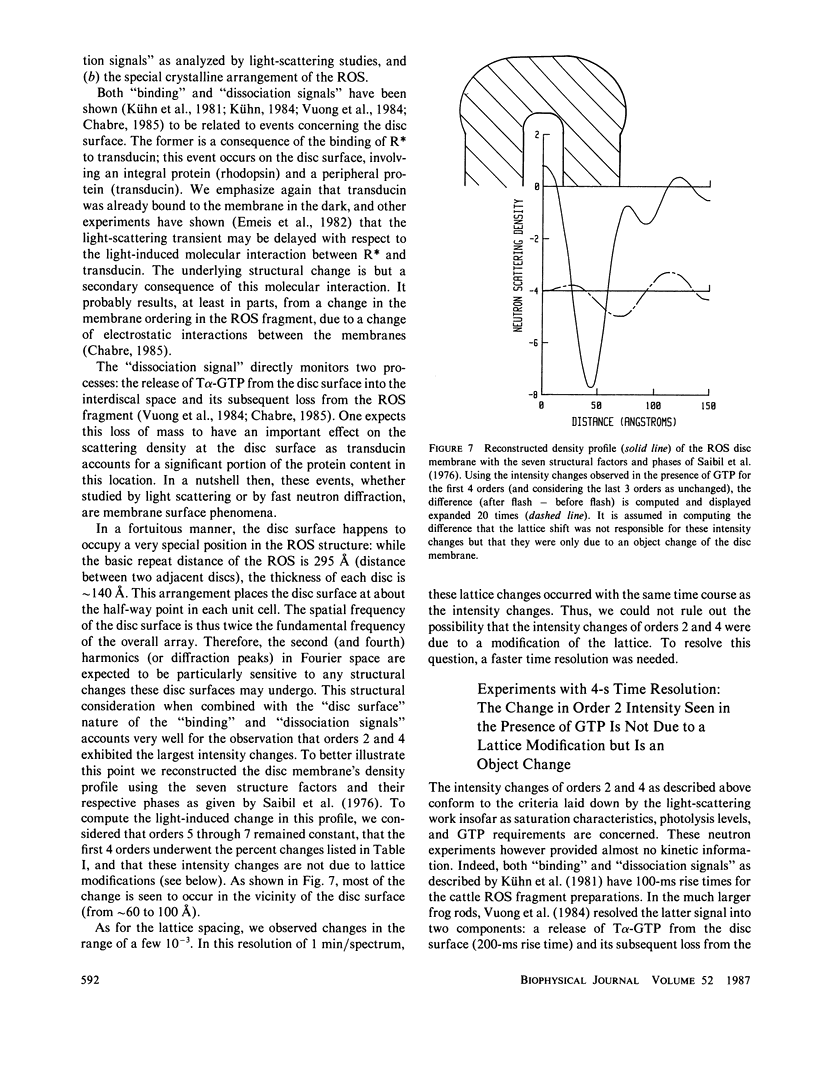
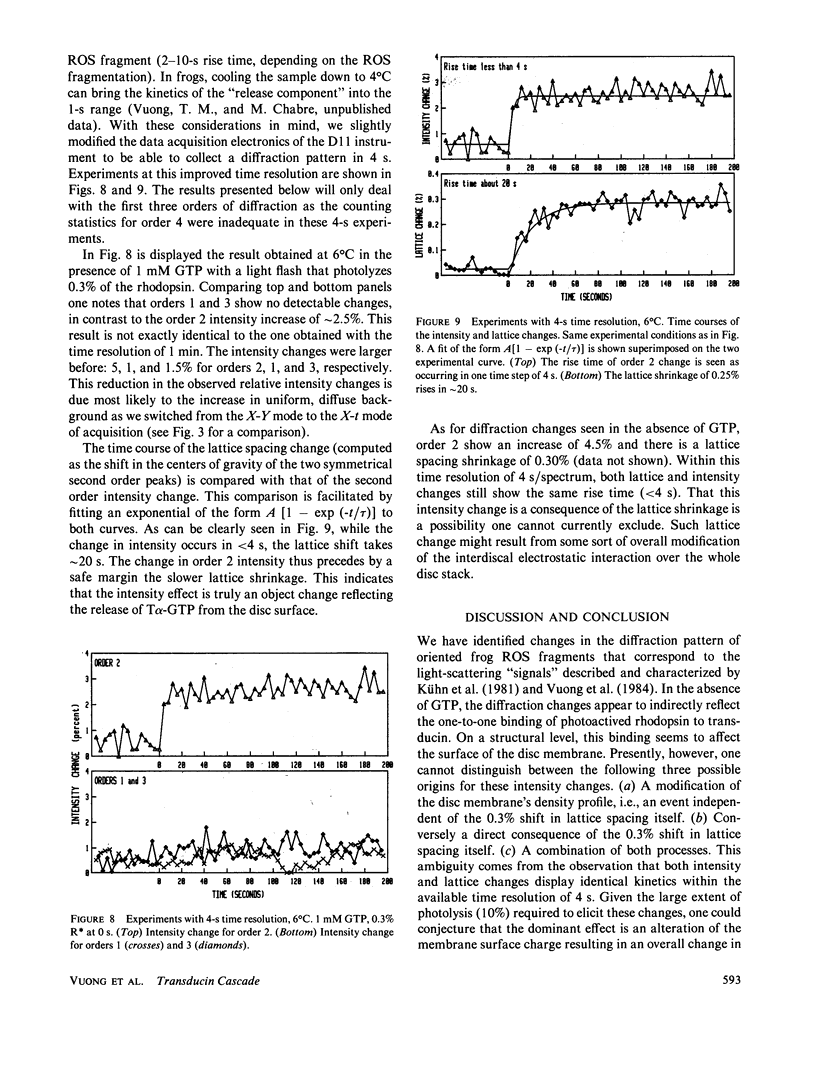
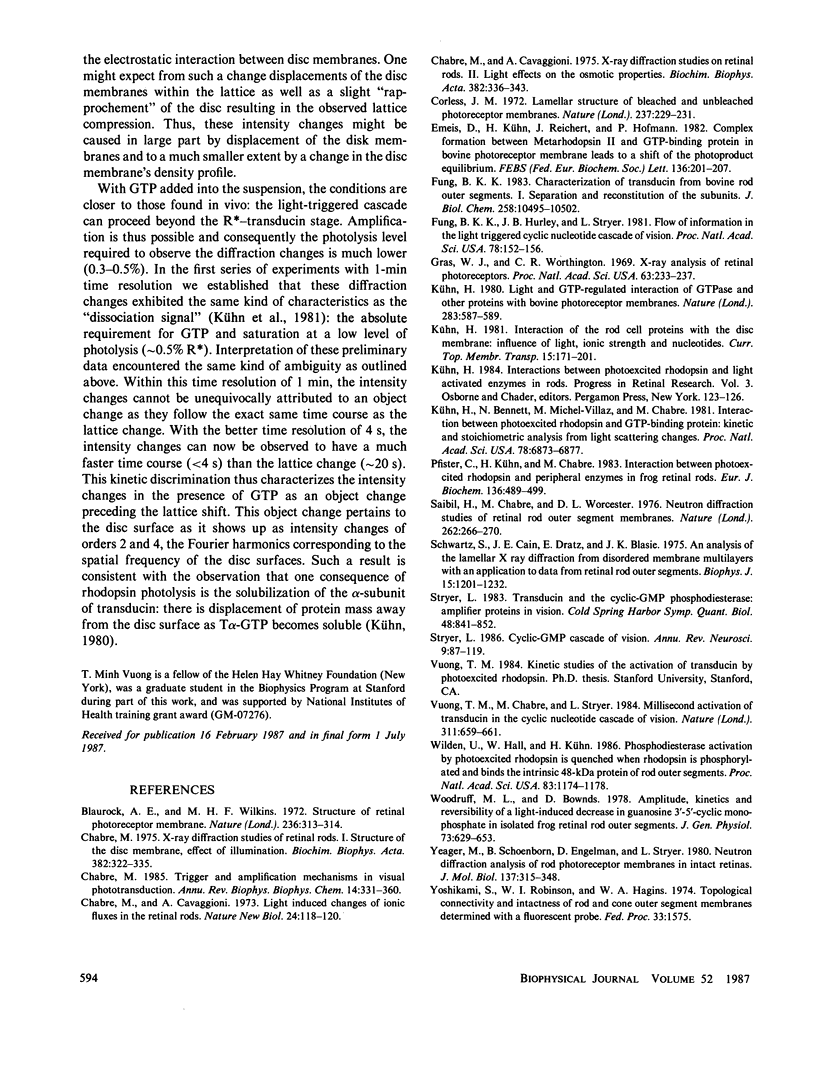
Selected References
These references are in PubMed. This may not be the complete list of references from this article.
- Blaurock A. E., Wilkins M. H. Structure of retinal photoreceptor membranes. Nature. 1972 Apr 7;236(5345):313–314. doi: 10.1038/236313a0. [DOI] [PubMed] [Google Scholar]
- Chabre M., Cavaggioni A. Light induced changes in ionic flux in the retinal rod. Nat New Biol. 1973 Jul 25;244(134):118–120. doi: 10.1038/newbio244118a0. [DOI] [PubMed] [Google Scholar]
- Chabre M., Cavaggioni A. X-ray diffraction studies of retinal rods. II. Light effect on the osmotic properties. Biochim Biophys Acta. 1975 Mar 25;382(3):336–343. doi: 10.1016/0005-2736(75)90275-8. [DOI] [PubMed] [Google Scholar]
- Chabre M. Trigger and amplification mechanisms in visual phototransduction. Annu Rev Biophys Biophys Chem. 1985;14:331–360. doi: 10.1146/annurev.bb.14.060185.001555. [DOI] [PubMed] [Google Scholar]
- Chabre M. X-ray diffraction studies of retinal rods. I. Structure of the disc membrane, effect of illumination. Biochim Biophys Acta. 1975 Mar 25;382(3):322–335. doi: 10.1016/0005-2736(75)90274-6. [DOI] [PubMed] [Google Scholar]
- Corless J. M. Lamellar structure of bleached and unbleached rod photoreceptor membranes. Nature. 1972 May 26;237(5352):229–231. doi: 10.1038/237229a0. [DOI] [PubMed] [Google Scholar]
- Fung B. K. Characterization of transducin from bovine retinal rod outer segments. I. Separation and reconstitution of the subunits. J Biol Chem. 1983 Sep 10;258(17):10495–10502. [PubMed] [Google Scholar]
- Fung B. K., Hurley J. B., Stryer L. Flow of information in the light-triggered cyclic nucleotide cascade of vision. Proc Natl Acad Sci U S A. 1981 Jan;78(1):152–156. doi: 10.1073/pnas.78.1.152. [DOI] [PMC free article] [PubMed] [Google Scholar]
- Gras W. J., Worthington C. R. X-ray analysis of retinal photoreceptors. Proc Natl Acad Sci U S A. 1969 Jun;63(2):233–238. doi: 10.1073/pnas.63.2.233. [DOI] [PMC free article] [PubMed] [Google Scholar]
- Kühn H., Bennett N., Michel-Villaz M., Chabre M. Interactions between photoexcited rhodopsin and GTP-binding protein: kinetic and stoichiometric analyses from light-scattering changes. Proc Natl Acad Sci U S A. 1981 Nov;78(11):6873–6877. doi: 10.1073/pnas.78.11.6873. [DOI] [PMC free article] [PubMed] [Google Scholar]
- Kühn H. Light- and GTP-regulated interaction of GTPase and other proteins with bovine photoreceptor membranes. Nature. 1980 Feb 7;283(5747):587–589. doi: 10.1038/283587a0. [DOI] [PubMed] [Google Scholar]
- Pfister C., Kühn H., Chabre M. Interaction between photoexcited rhodopsin and peripheral enzymes in frog retinal rods. Influence on the postmetarhodopsin II decay and phosphorylation rate of rhodopsin. Eur J Biochem. 1983 Nov 15;136(3):489–499. doi: 10.1111/j.1432-1033.1983.tb07767.x. [DOI] [PubMed] [Google Scholar]
- Saibil H., Chabre M., Worcester D. Neutron diffraction studies of retinal rod outer segment membranes. Nature. 1976 Jul 22;262(5566):266–270. doi: 10.1038/262266a0. [DOI] [PubMed] [Google Scholar]
- Schwartz S., Cain J. E., Dratz E. A., Blasie J. K. An analysis of lamellar x-ray diffraction from disordered membrane multilayers with application to data from retinal rod outer segments. Biophys J. 1975 Dec;15(12):1201–1233. doi: 10.1016/S0006-3495(75)85895-4. [DOI] [PMC free article] [PubMed] [Google Scholar]
- Stryer L. Cyclic GMP cascade of vision. Annu Rev Neurosci. 1986;9:87–119. doi: 10.1146/annurev.ne.09.030186.000511. [DOI] [PubMed] [Google Scholar]
- Stryer L. Transducin and the cyclic GMP phosphodiesterase: amplifier proteins in vision. Cold Spring Harb Symp Quant Biol. 1983;48(Pt 2):841–852. doi: 10.1101/sqb.1983.048.01.087. [DOI] [PubMed] [Google Scholar]
- Vuong T. M., Chabre M., Stryer L. Millisecond activation of transducin in the cyclic nucleotide cascade of vision. Nature. 1984 Oct 18;311(5987):659–661. doi: 10.1038/311659a0. [DOI] [PubMed] [Google Scholar]
- Wilden U., Hall S. W., Kühn H. Phosphodiesterase activation by photoexcited rhodopsin is quenched when rhodopsin is phosphorylated and binds the intrinsic 48-kDa protein of rod outer segments. Proc Natl Acad Sci U S A. 1986 Mar;83(5):1174–1178. doi: 10.1073/pnas.83.5.1174. [DOI] [PMC free article] [PubMed] [Google Scholar]
- Woodruff M. L., Bownds M. D. Amplitude, kinetics, and reversibility of a light-induced decrease in guanosine 3',5'-cyclic monophosphate in frog photoreceptor membranes. J Gen Physiol. 1979 May;73(5):629–653. doi: 10.1085/jgp.73.5.629. [DOI] [PMC free article] [PubMed] [Google Scholar]
- Yeager M., Schoenborn B., Engelman D., Moore P., Stryer L. Neutron diffraction analysis of the structure of rod photoreceptor membranes in intact retinas. J Mol Biol. 1980 Mar 5;137(3):315–348. doi: 10.1016/0022-2836(80)90319-8. [DOI] [PubMed] [Google Scholar]