Abstract
A quantitative investigation into the mechanism of neutrophil phagocytosis of opsonized microspheres possessing well defined dimensions was undertaken. Three aspects were documented: membrane conservation, cell adhesion to the spheres, and active cell cytoplasmic projection around the microspheres. The physical act of internalizing a particle by a cell involves a reduction in its plasma membrane area and an increase in its volume. As a consequence, a cell can internalize only a finite number of particles. A store of membrane area exists on cytoplasmic granules and may be recruited during phagocytosis. Previous measurements of neutrophil membrane area and volume served as a basis for estimates of the maximum number of internalized microspheres. A comparison with experimental prediction based on membrane conservation and degranulation agrees within 10% for a range of microsphere diameters, from 0.5 to 8 microns. This suggests that the limitation for additional particle uptake in the population of engorged neutrophils is the lack of excess plasma membrane area. In a random population of neutrophils, there was a sub-group, approximately 40%, which could no longer phagocytose before depleting their membrane stores. Several aspects of the engulfment process were investigated to elucidate the cause of this phagocytosis deficiency. It could be shown by single cell observation that these cases were associated with a lack of pseudopod projection, although adhesion was still evident between the cell membrane and the microspheres.
Full text
PDF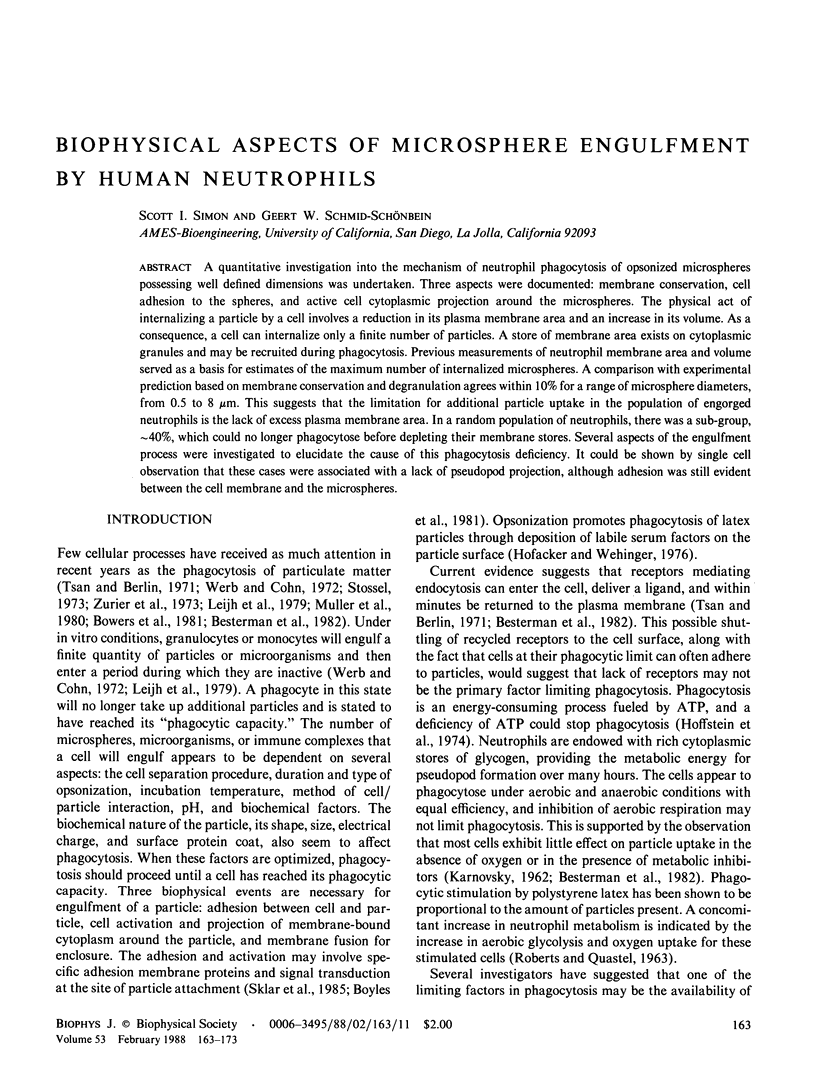
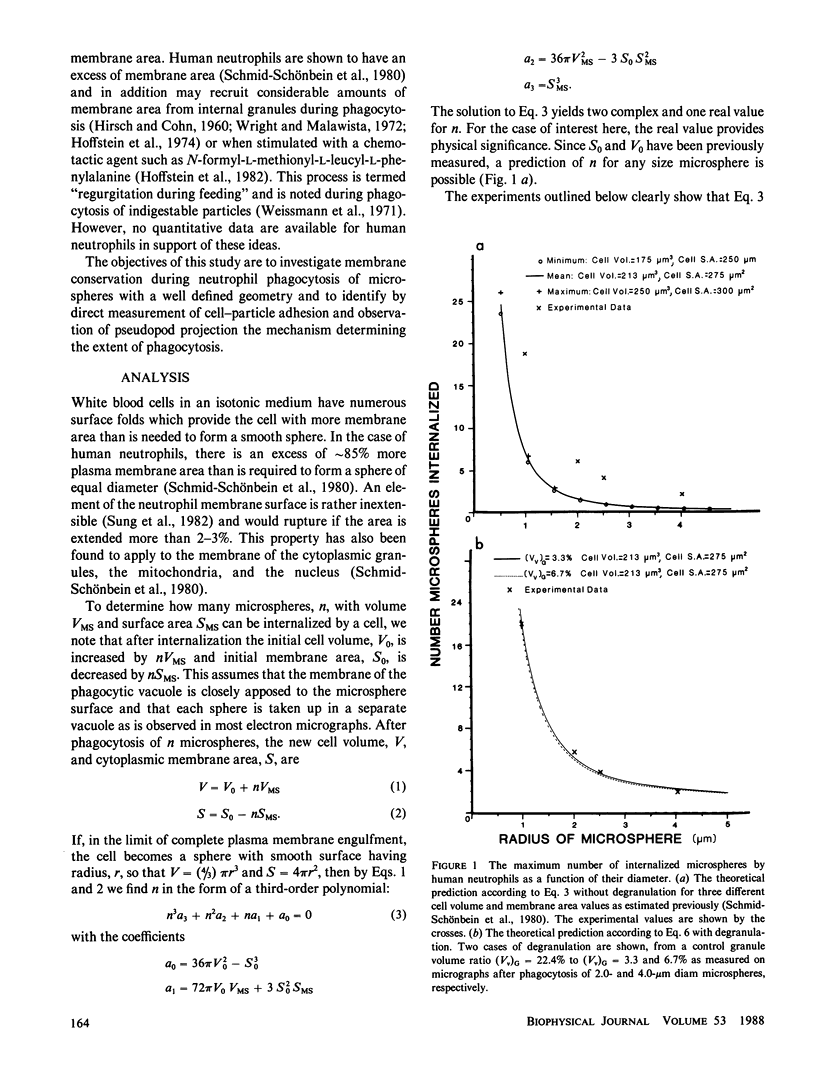
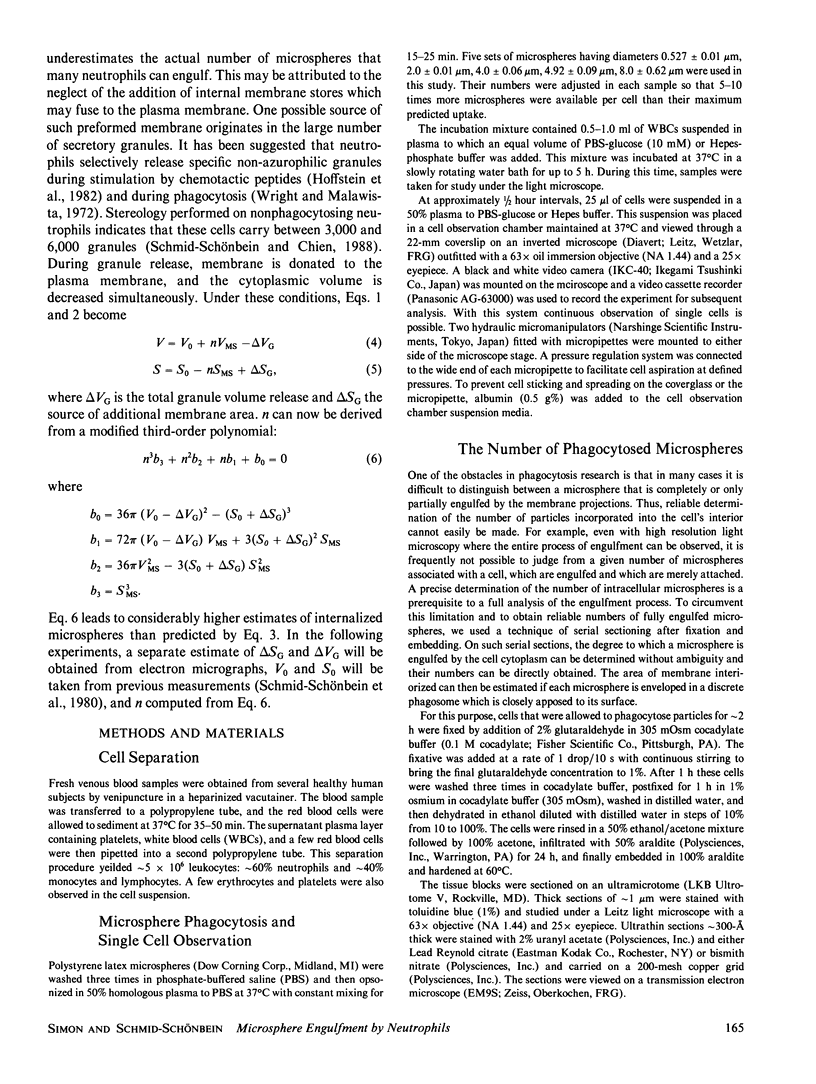
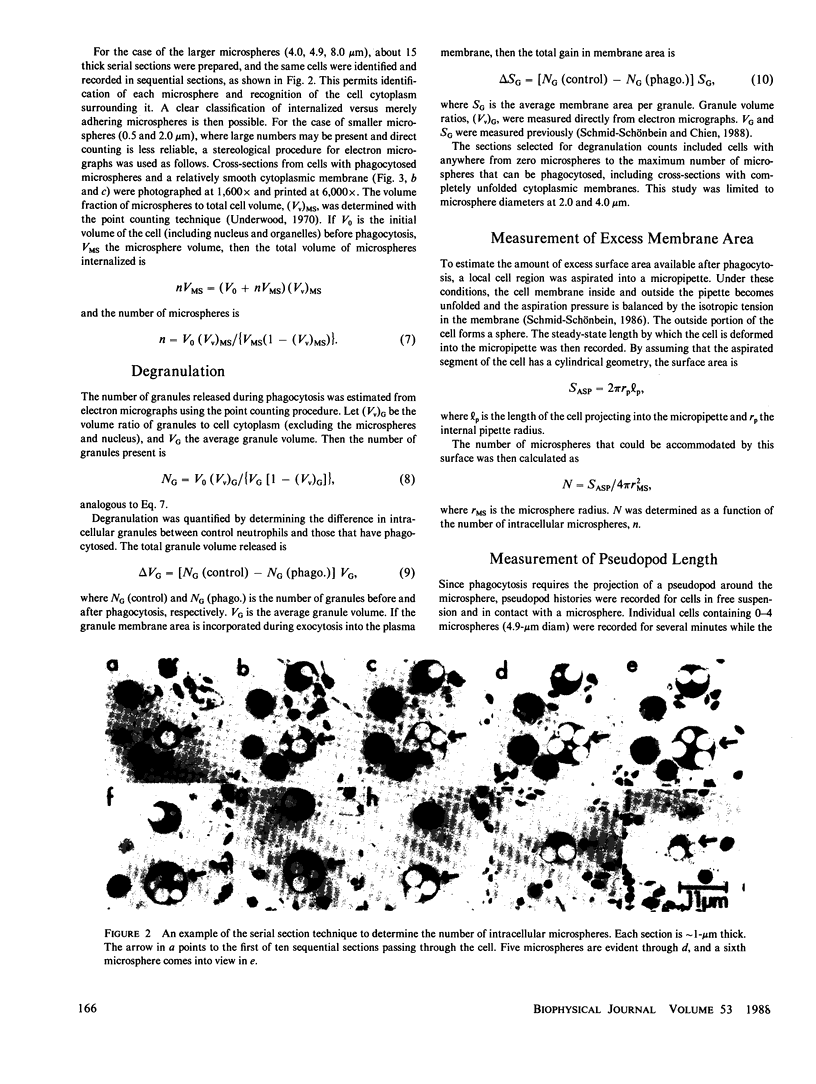
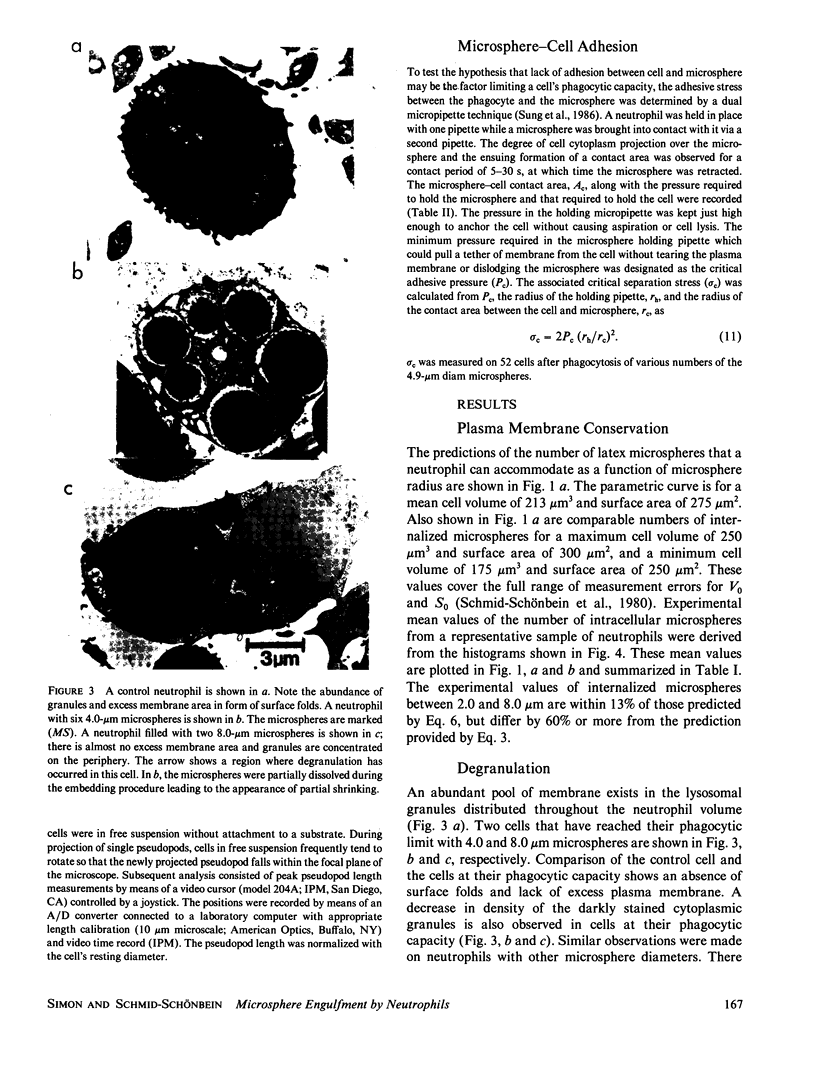
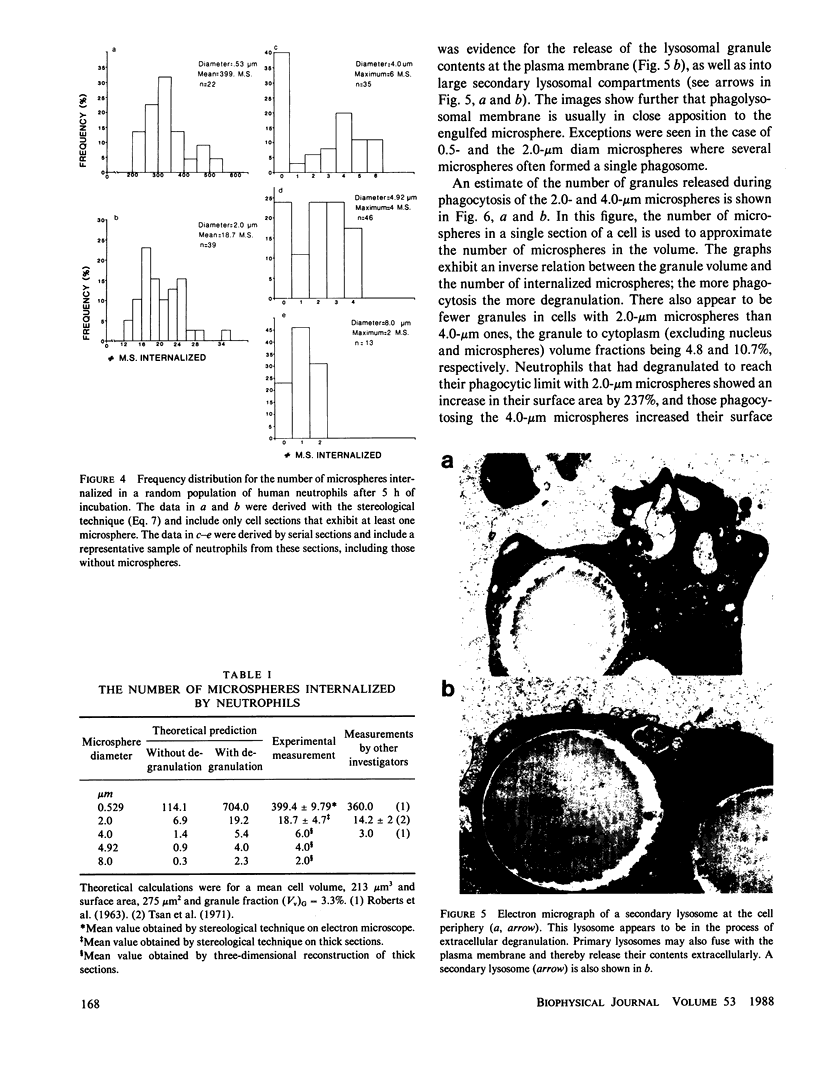
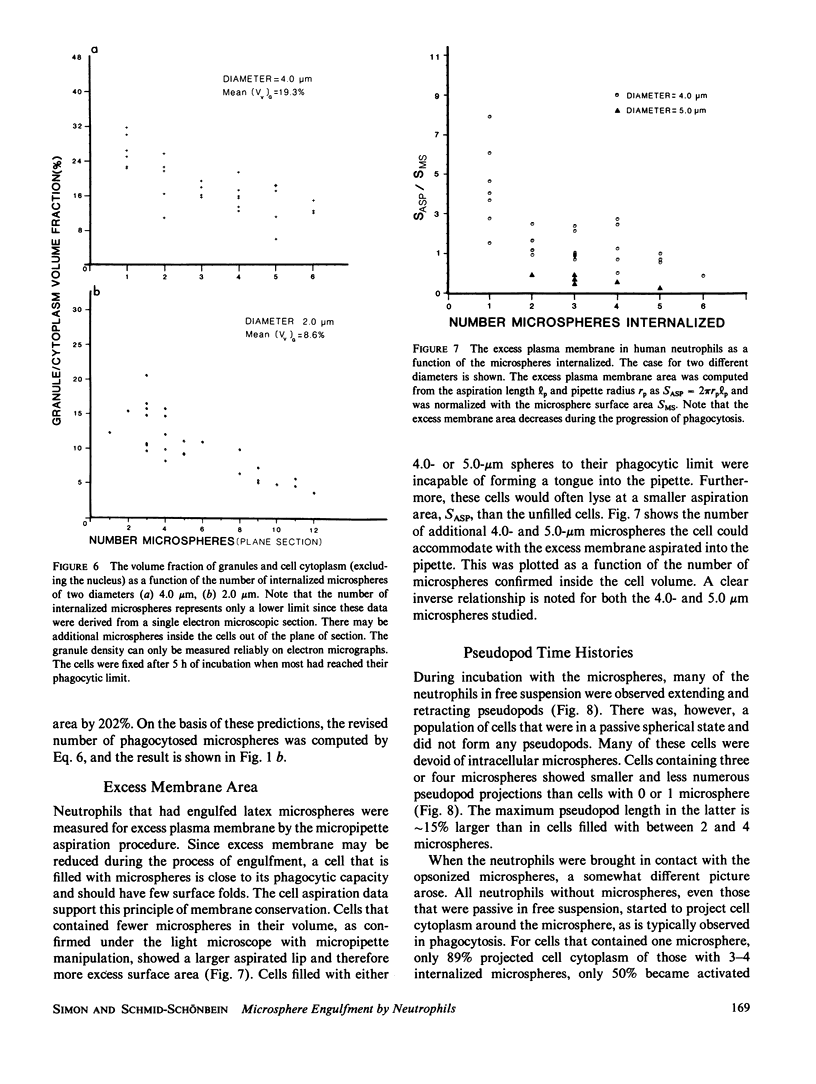
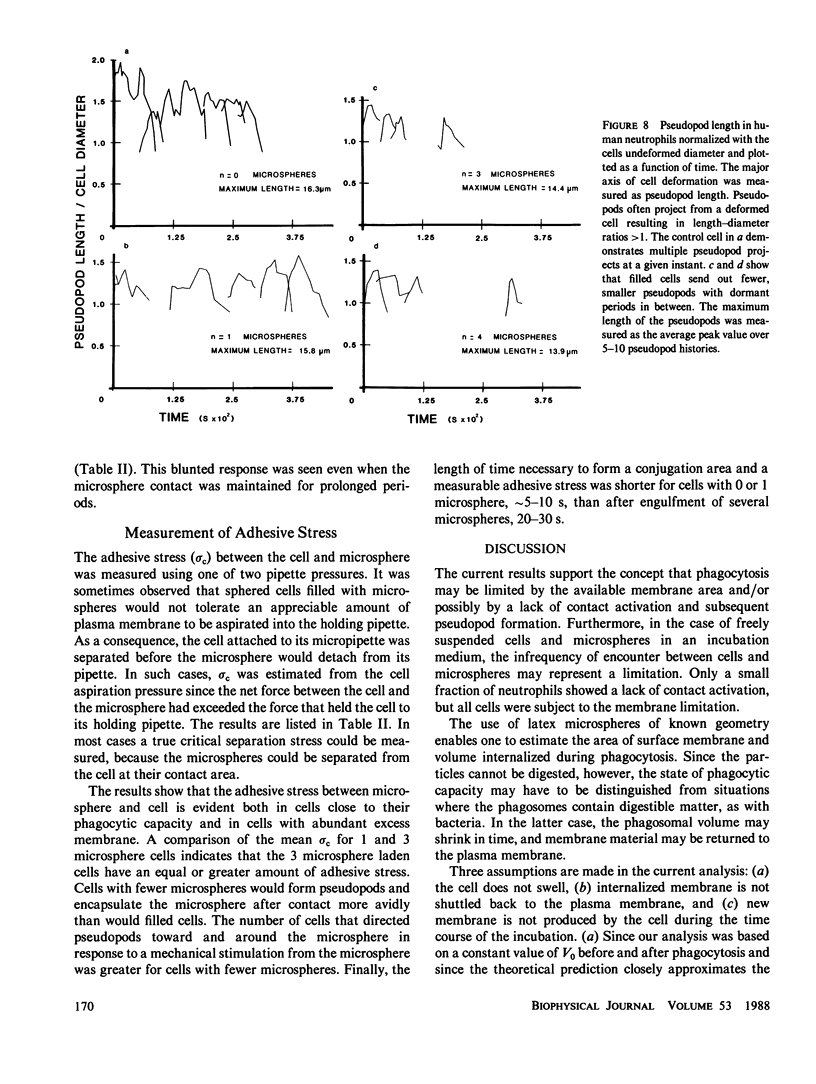
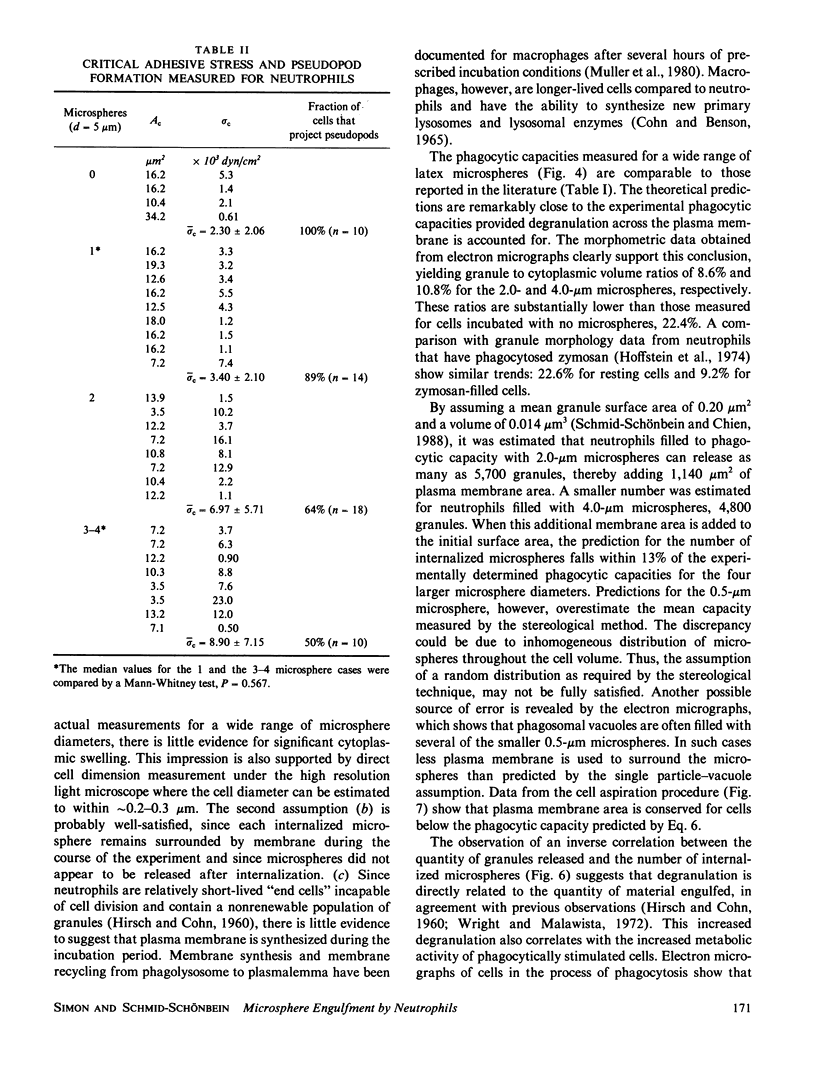
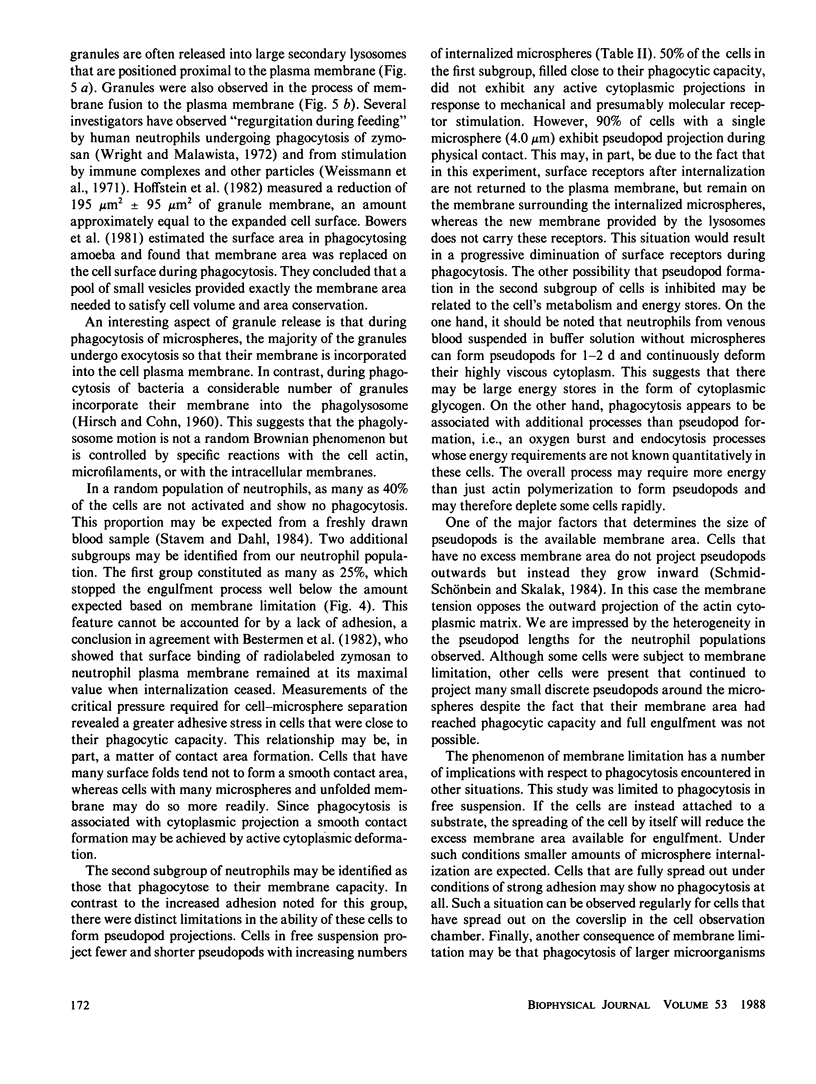
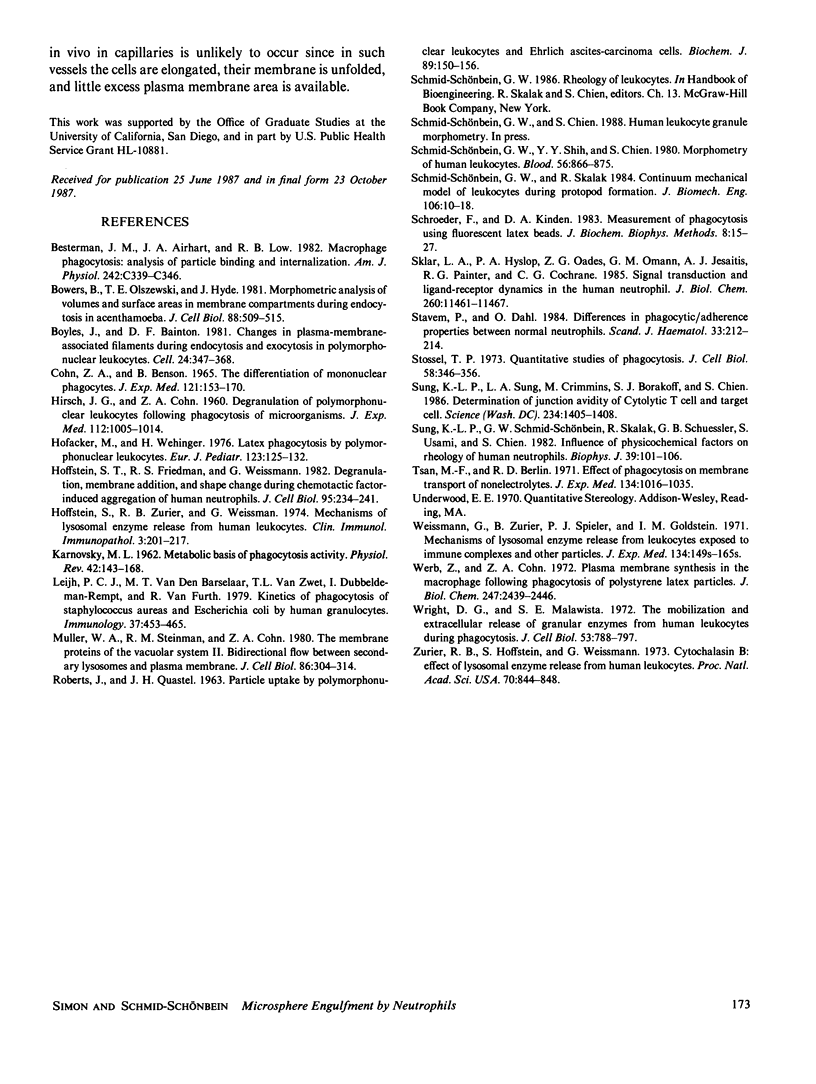
Images in this article
Selected References
These references are in PubMed. This may not be the complete list of references from this article.
- Besterman J. M., Airhart J. A., Low R. B. Macrophage phagocytosis: analysis of particle binding and internalization. Am J Physiol. 1982 May;242(5):C339–C346. doi: 10.1152/ajpcell.1982.242.5.C339. [DOI] [PubMed] [Google Scholar]
- Bowers B., Olszewski T. E., Hyde J. Morphometric analysis of volumes and surface areas in membrane compartments during endocytosis in Acanthamoeba. J Cell Biol. 1981 Mar;88(3):509–515. doi: 10.1083/jcb.88.3.509. [DOI] [PMC free article] [PubMed] [Google Scholar]
- COHN Z. A., BENSON B. THE DIFFERENTIATION OF MONONUCLEAR PHAGOCYTES. MORPHOLOGY, CYTOCHEMISTRY, AND BIOCHEMISTRY. J Exp Med. 1965 Jan 1;121:153–170. doi: 10.1084/jem.121.1.153. [DOI] [PMC free article] [PubMed] [Google Scholar]
- HIRSCH J. G., COHN Z. A. Degranulation of polymorphonuclear leucocytes following phagocytosis of microorganisms. J Exp Med. 1960 Dec 1;112:1005–1014. doi: 10.1084/jem.112.6.1005. [DOI] [PMC free article] [PubMed] [Google Scholar]
- Hoffstein S. T., Friedman R. S., Weissmann G. Degranulation, membrane addition, and shape change during chemotactic factor-induced aggregation of human neutrophils. J Cell Biol. 1982 Oct;95(1):234–241. doi: 10.1083/jcb.95.1.234. [DOI] [PMC free article] [PubMed] [Google Scholar]
- Hoffstein S., Zurier R. B., Weissmann G. Mechanisms of lysosomal enzyme release from human leucocytes. III. Quantitative morphologic evidence for an effect of cyclic nucleotides and colchicine on degranulation. Clin Immunol Immunopathol. 1974 Nov;3(2):201–217. doi: 10.1016/0090-1229(74)90006-3. [DOI] [PubMed] [Google Scholar]
- KARNOVSKY M. L. Metabolic basis of phagocytic activity. Physiol Rev. 1962 Jan;42:143–168. doi: 10.1152/physrev.1962.42.1.143. [DOI] [PubMed] [Google Scholar]
- Leijh P. C., van den Barselaar M. T., van Zwet T. L., Dubbeldeman-Rempt I., van Furth R. Kinetics of phagocytosis of Staphylococcus aureus and Escherichia coli by human granulocytes. Immunology. 1979 Jun;37(2):453–465. [PMC free article] [PubMed] [Google Scholar]
- Muller W. A., Steinman R. M., Cohn Z. A. The membrane proteins of the vacuolar system. II. Bidirectional flow between secondary lysosomes and plasma membrane. J Cell Biol. 1980 Jul;86(1):304–314. doi: 10.1083/jcb.86.1.304. [DOI] [PMC free article] [PubMed] [Google Scholar]
- ROBERTS J., QUASTEL J. H. PARTICLE UPTAKE BY POLYMORPHONUCLEAR LEUCOCYTES AND EHRLICH ASCITES-CARCINOMA CELLS. Biochem J. 1963 Oct;89:150–156. doi: 10.1042/bj0890150. [DOI] [PMC free article] [PubMed] [Google Scholar]
- Schmid-Schönbein G. W., Shih Y. Y., Chien S. Morphometry of human leukocytes. Blood. 1980 Nov;56(5):866–875. [PubMed] [Google Scholar]
- Schmid-Schönbein G. W., Skalak R. Continuum mechanical model of leukocytes during protopod formation. J Biomech Eng. 1984 Feb;106(1):10–18. doi: 10.1115/1.3138448. [DOI] [PubMed] [Google Scholar]
- Schroeder F., Kinden D. A. Measurement of phagocytosis using fluorescent latex beads. J Biochem Biophys Methods. 1983 Aug;8(1):15–27. doi: 10.1016/0165-022x(83)90017-9. [DOI] [PubMed] [Google Scholar]
- Sklar L. A., Hyslop P. A., Oades Z. G., Omann G. M., Jesaitis A. J., Painter R. G., Cochrane C. G. Signal transduction and ligand-receptor dynamics in the human neutrophil. Transient responses and occupancy-response relations at the formyl peptide receptor. J Biol Chem. 1985 Sep 25;260(21):11461–11467. [PubMed] [Google Scholar]
- Stavem P., Dahl O. Differences in phagocytic/adherence properties between normal neutrophils. Scand J Haematol. 1984 Aug;33(2):212–214. doi: 10.1111/j.1600-0609.1984.tb02398.x. [DOI] [PubMed] [Google Scholar]
- Stossel T. P. Quantitative studies of phagocytosis. Kinetic effects of cations and heat-labile opsonin. J Cell Biol. 1973 Aug;58(2):346–356. doi: 10.1083/jcb.58.2.346. [DOI] [PMC free article] [PubMed] [Google Scholar]
- Sung K. L., Schmid-Schönbein G. W., Skalak R., Schuessler G. B., Usami S., Chien S. Influence of physicochemical factors on rheology of human neutrophils. Biophys J. 1982 Jul;39(1):101–106. doi: 10.1016/S0006-3495(82)84495-0. [DOI] [PMC free article] [PubMed] [Google Scholar]
- Sung K. L., Sung L. A., Crimmins M., Burakoff S. J., Chien S. Determination of junction avidity of cytolytic T cell and target cell. Science. 1986 Dec 12;234(4782):1405–1408. doi: 10.1126/science.3491426. [DOI] [PubMed] [Google Scholar]
- Tsan M. F., Berlin R. D. Effect of phagocytosis on membrane transport of nonelectrolytes. J Exp Med. 1971 Oct 1;134(4):1016–1035. doi: 10.1084/jem.134.4.1016. [DOI] [PMC free article] [PubMed] [Google Scholar]
- Wehinger H., Hofacker M. Latex phagocytosis by polymorphonuclear leukocytes. In vitro and in vivo studies with a simple screening test. Eur J Pediatr. 1976 Sep 1;123(2):125–132. doi: 10.1007/BF00442642. [DOI] [PubMed] [Google Scholar]
- Weissmann G., Zurier R. B., Spieler P. J., Goldstein I. M. Mechanisms of lysosomal enzyme release from leukocytes exposed to immune complexes and other particles. J Exp Med. 1971 Sep 1;134(3 Pt 2):149s–165s. [PubMed] [Google Scholar]
- Werb Z., Cohn Z. A. Plasma membrane synthesis in the macrophage following phagocytosis of polystyrene latex particles. J Biol Chem. 1972 Apr 25;247(8):2439–2446. [PubMed] [Google Scholar]
- Wright D. G., Malawista S. E. The mobilization and extracellular release of granular enzymes from human leukocytes during phagocytosis. J Cell Biol. 1972 Jun;53(3):788–797. doi: 10.1083/jcb.53.3.788. [DOI] [PMC free article] [PubMed] [Google Scholar]
- Zurier R. B., Hoffstein S., Weissmann G. Cytochalasin B: effect on lysosomal enzyme release from human leukocytes. Proc Natl Acad Sci U S A. 1973 Mar;70(3):844–848. doi: 10.1073/pnas.70.3.844. [DOI] [PMC free article] [PubMed] [Google Scholar]