Abstract
The distribution of a selection of cationic fluorescent dyes can be used to measure the membrane potential of individual cells with a microfluorometer. The essential attributes of these dyes include membrane permeability, low membrane binding, spectral properties which are insensitive to environment, and, of course, strong fluorescence. A series of dyes were screened on HeLa cells for their ability to meet these criteria and several commercially available dyes were found to be satisfactory. In addition, two new dyes were synthesized for this work by esterification of tetramethyl rhodamine. The analysis of the measured fluorescent intensities requires correction for fluorescence collected from outside the plane of focus of the cell and for nonpotentiometric binding of the dye. The measurements and analysis were performed on three different cell types for which there exists a body of literature on membrane potential; the potentials determined in this work were always within the range of literature values. The rhodamine esters are nontoxic, highly fluorescent dyes which do not form aggregates or display binding-dependent changes in fluorescence efficiency. Thus, their reversible accumulation is quantitatively related to the contrast between intracellular and extracellular fluorescence and allows membrane potentials in individual cells to be continuously monitored.
Full text
PDF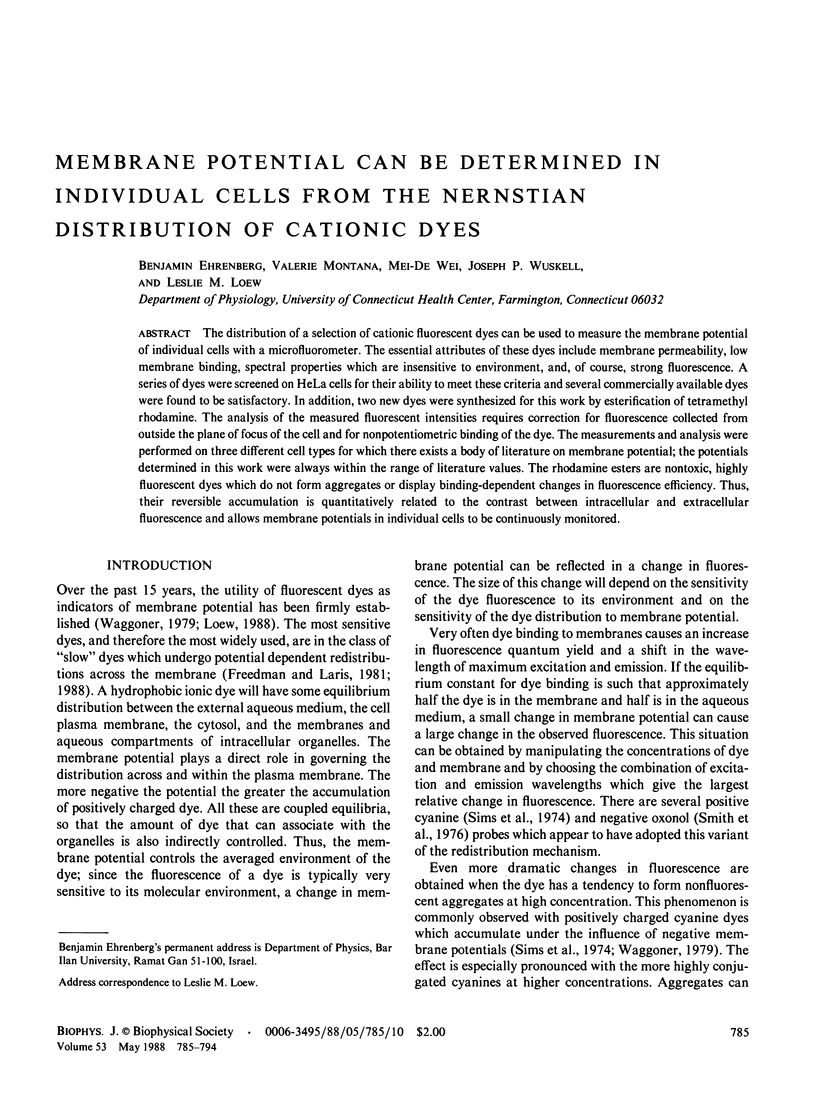
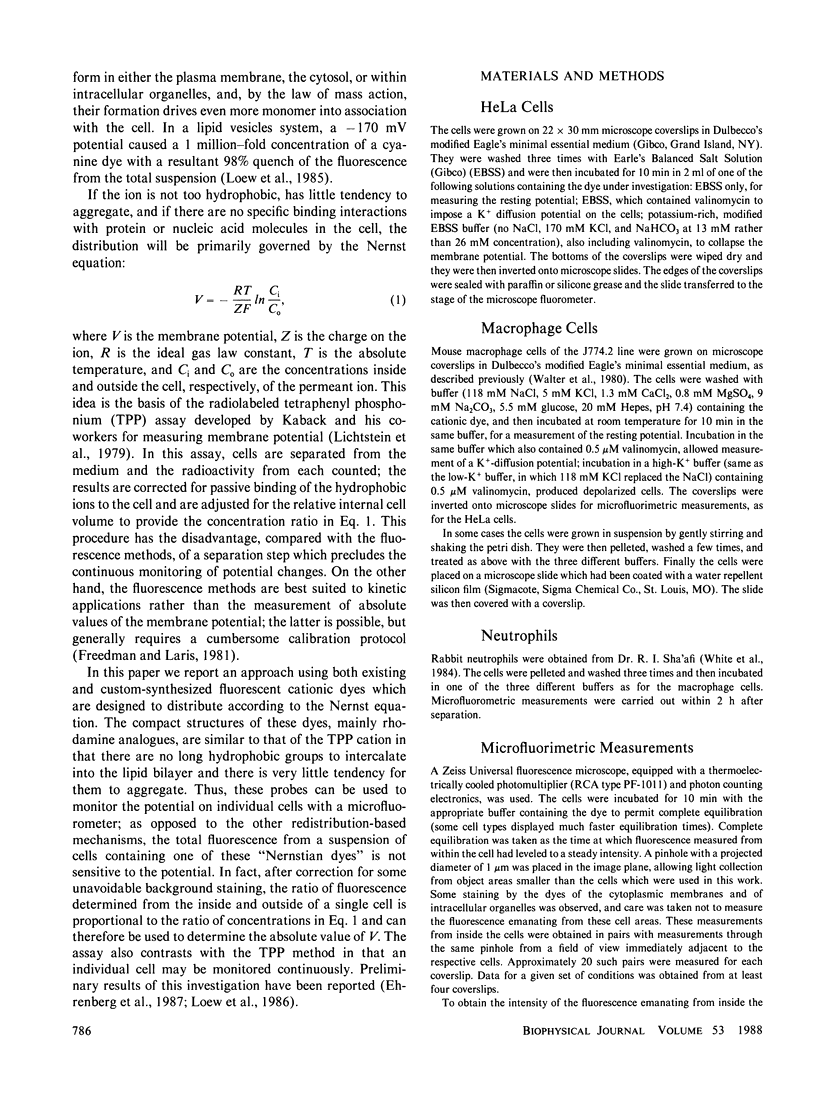
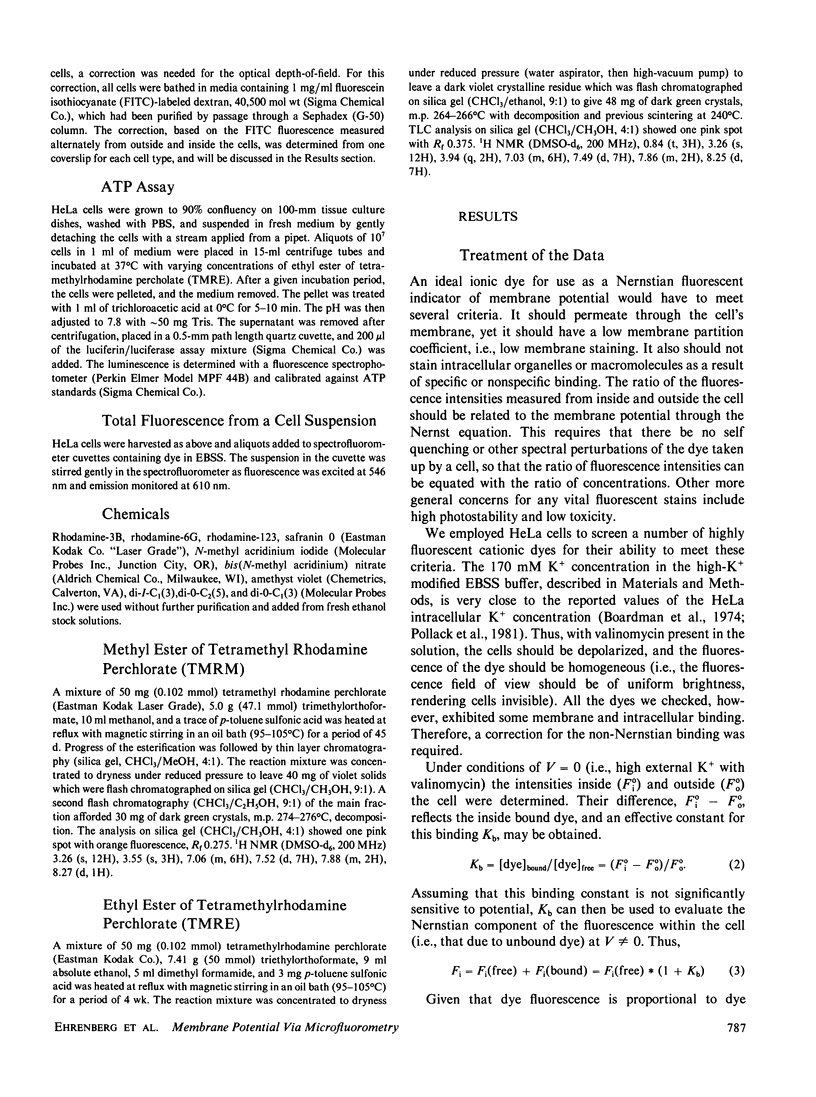
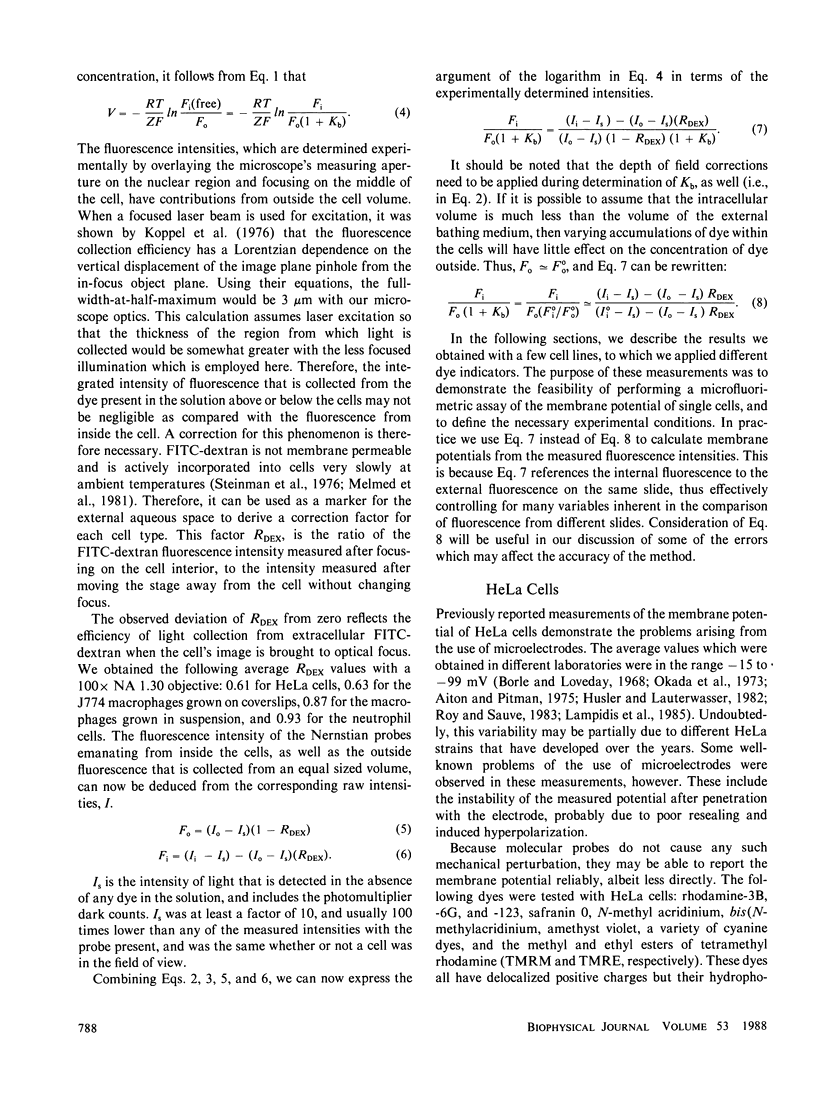
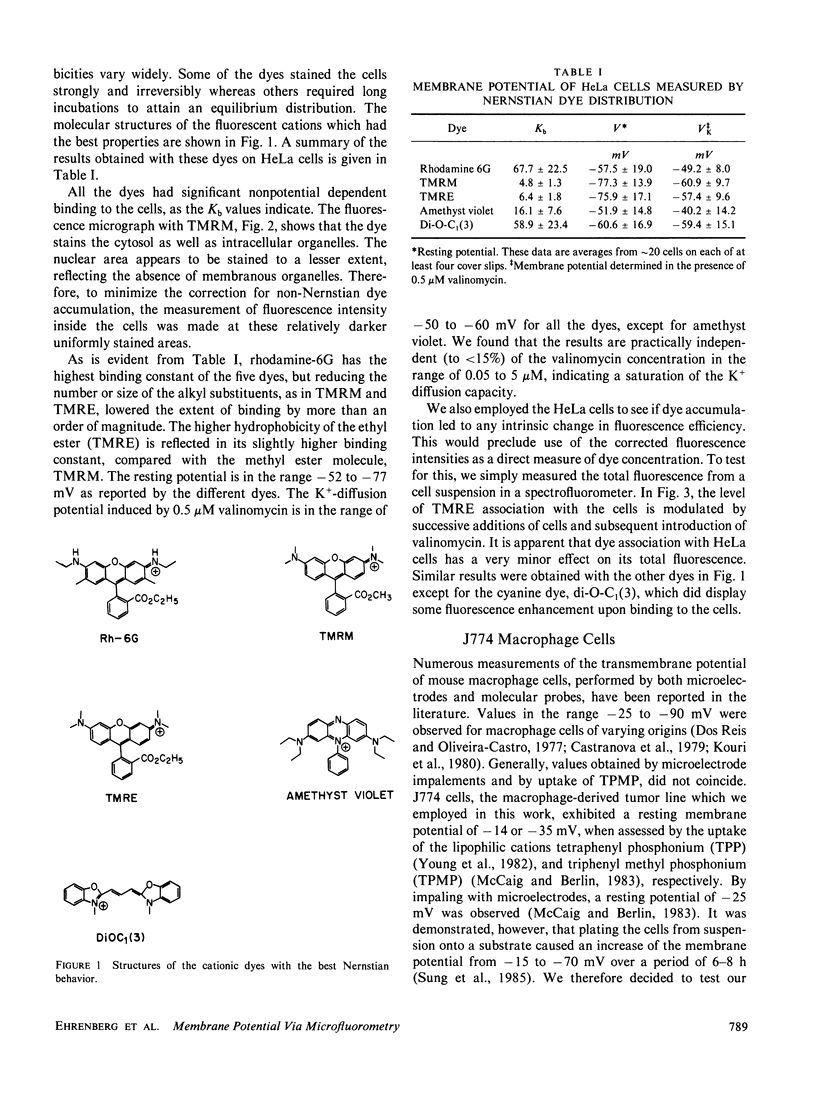
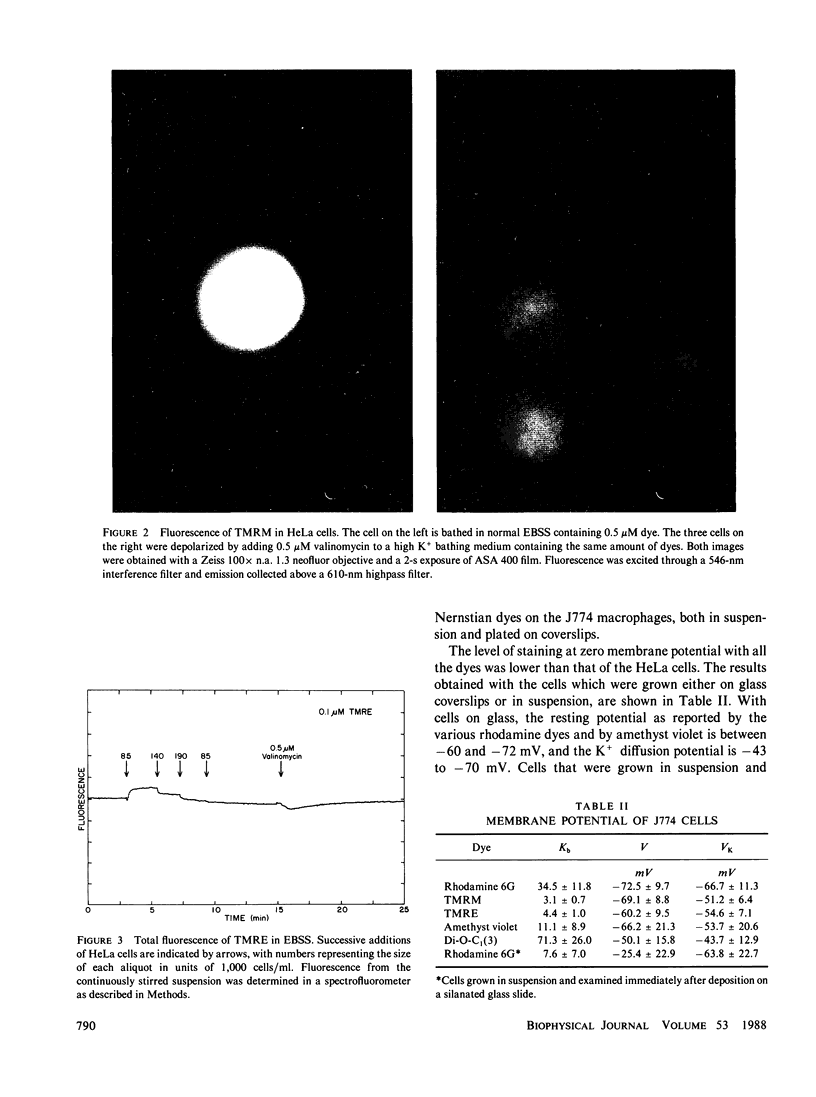
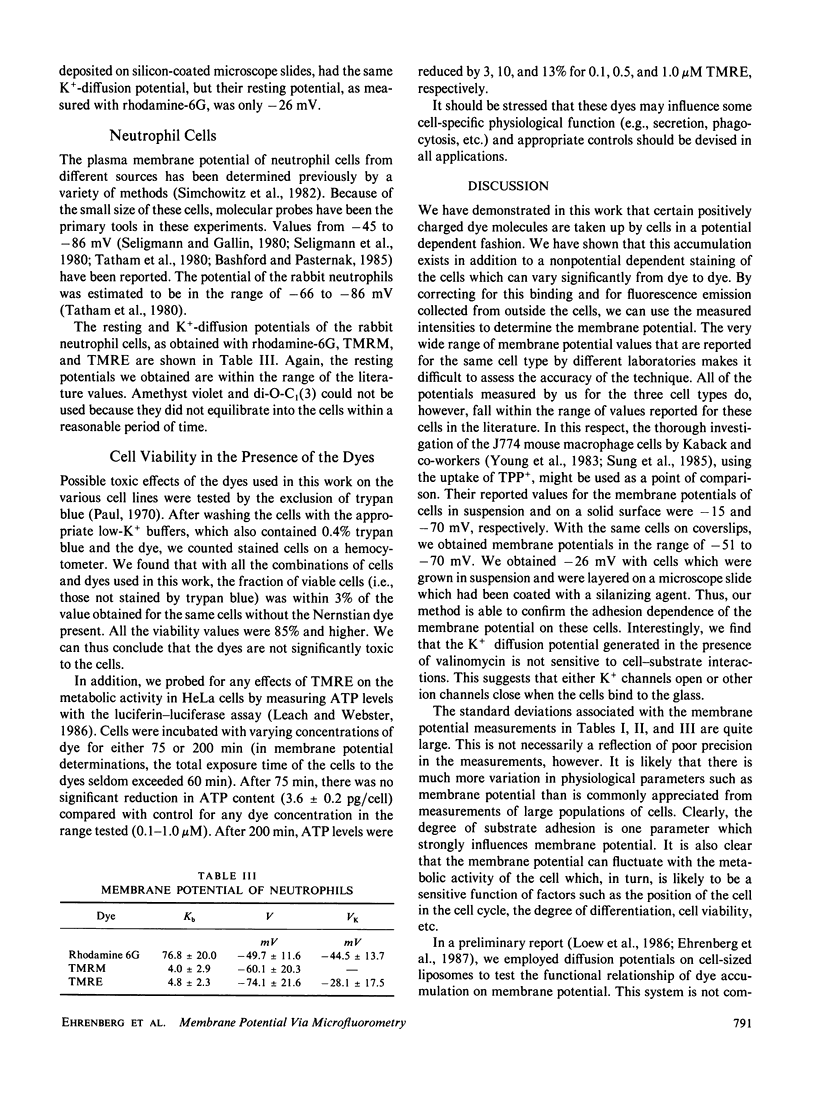
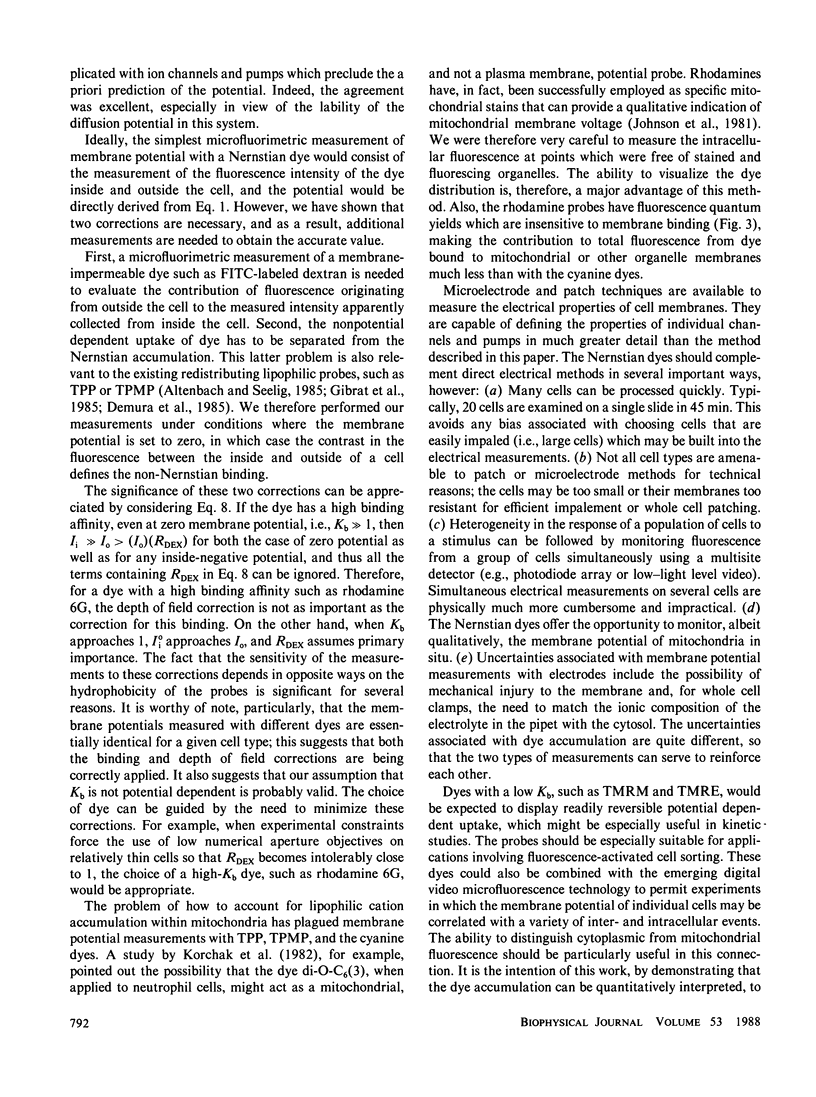
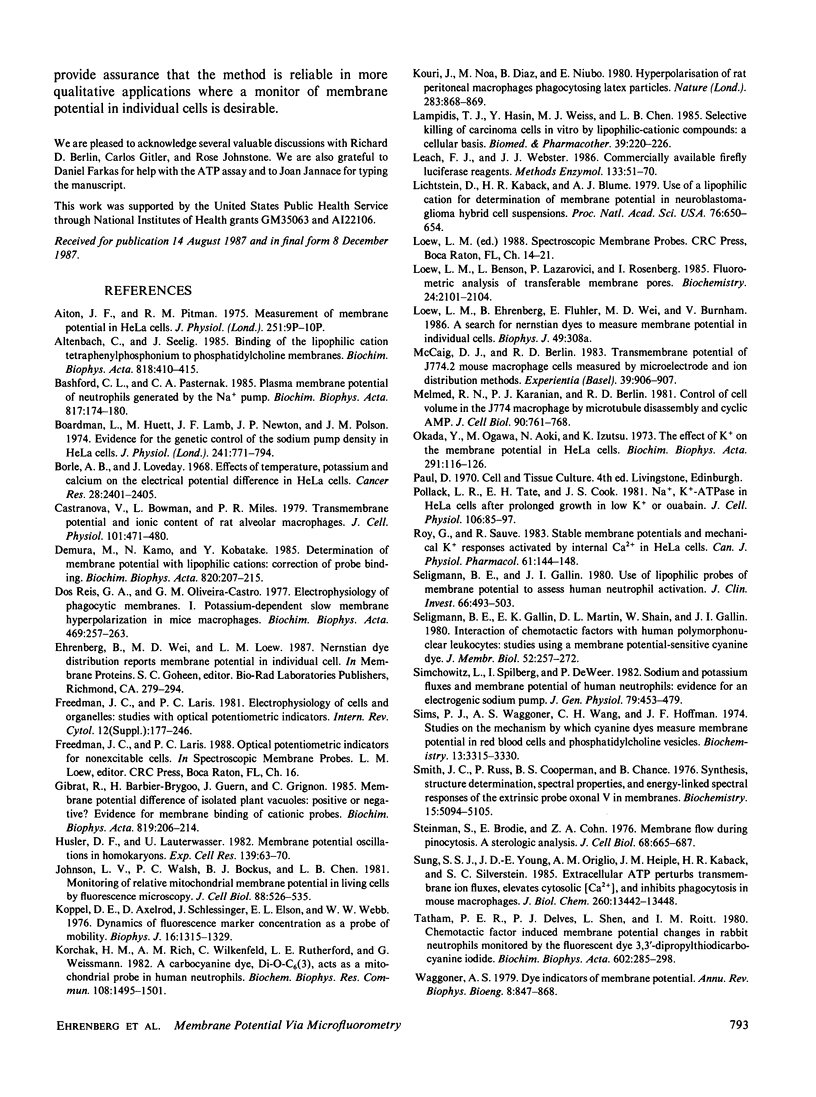
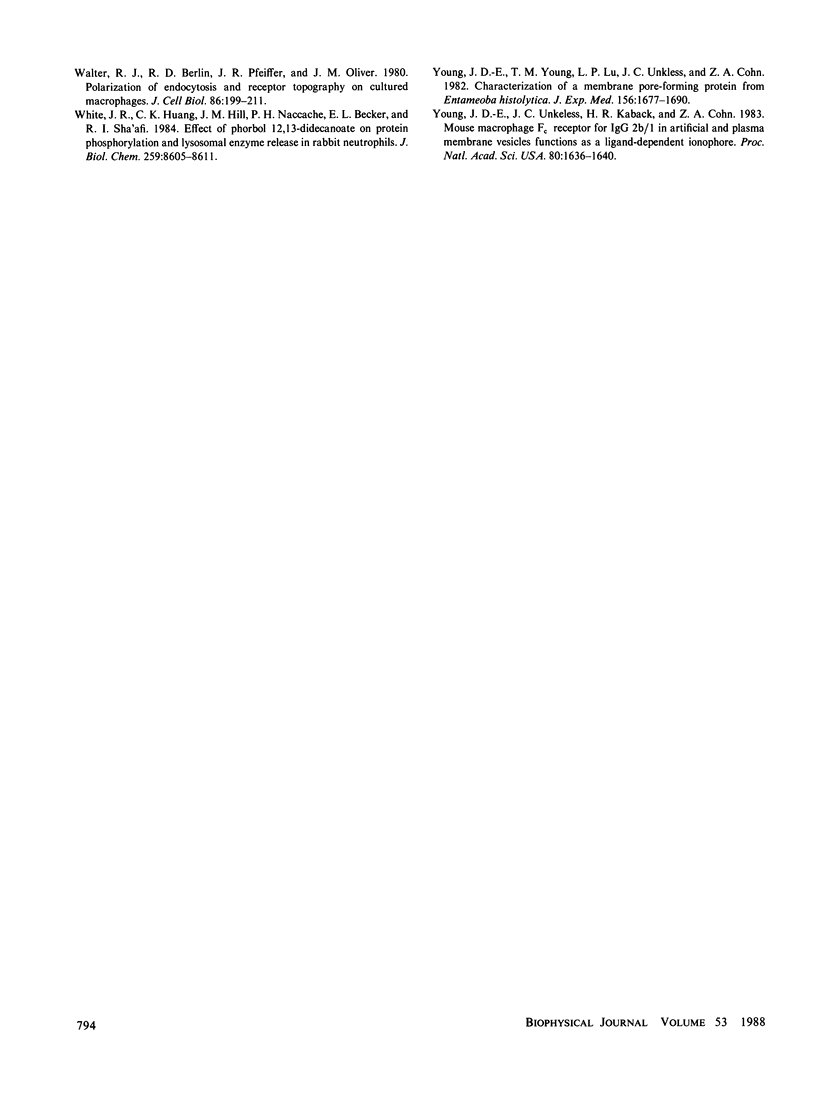
Images in this article
Selected References
These references are in PubMed. This may not be the complete list of references from this article.
- Bashford C. L., Pasternak C. A. Plasma membrane potential of neutrophils generated by the Na+ pump. Biochim Biophys Acta. 1985 Jul 11;817(1):174–180. doi: 10.1016/0005-2736(85)90080-x. [DOI] [PubMed] [Google Scholar]
- Boardman L., Huett M., Lamb J. F., Newton J. P., Polson J. M. Evidence for the genetic control of the sodium pump density in HeLa cells. J Physiol. 1974 Sep;241(3):771–794. doi: 10.1113/jphysiol.1974.sp010684. [DOI] [PMC free article] [PubMed] [Google Scholar]
- Borle A. B., Loveday J. Effects of temperature, potassium, and calcium on the electrical potential difference in HeLa cells. Cancer Res. 1968 Dec;28(12):2401–2405. [PubMed] [Google Scholar]
- Castranova V., Bowman L., Miles P. R. Transmembrane potential and ionic content of rat alveolar macrophages. J Cell Physiol. 1979 Dec;101(3):471–479. doi: 10.1002/jcp.1041010313. [DOI] [PubMed] [Google Scholar]
- Dos Reis G. A., Oliveira-Castro G. M. Electrophysiology of phagocytic membranes. I. Potassium-dependent slow membrane hyperpolarizations in mice macrophages. Biochim Biophys Acta. 1977 Sep 19;469(3):257–263. doi: 10.1016/0005-2736(77)90161-4. [DOI] [PubMed] [Google Scholar]
- Freedman J. C., Laris P. C. Electrophysiology of cells and organelles: studies with optical potentiometric indicators. Int Rev Cytol Suppl. 1981;12:177–246. doi: 10.1016/b978-0-12-364373-5.50015-9. [DOI] [PubMed] [Google Scholar]
- Hülser D. F., Lauterwasser U. Membrane potential oscillations in homokaryons. An endogenous signal for detecting intercellular communication. Exp Cell Res. 1982 May;139(1):63–70. doi: 10.1016/0014-4827(82)90318-4. [DOI] [PubMed] [Google Scholar]
- Johnson L. V., Walsh M. L., Bockus B. J., Chen L. B. Monitoring of relative mitochondrial membrane potential in living cells by fluorescence microscopy. J Cell Biol. 1981 Mar;88(3):526–535. doi: 10.1083/jcb.88.3.526. [DOI] [PMC free article] [PubMed] [Google Scholar]
- Koppel D. E., Axelrod D., Schlessinger J., Elson E. L., Webb W. W. Dynamics of fluorescence marker concentration as a probe of mobility. Biophys J. 1976 Nov;16(11):1315–1329. doi: 10.1016/S0006-3495(76)85776-1. [DOI] [PMC free article] [PubMed] [Google Scholar]
- Korchak H. M., Rich A. M., Wilkenfeld C., Rutherford L. E., Weissmann G. A carbocyanine dye, DiOC6(3), acts as a mitochondrial probe in human neutrophils. Biochem Biophys Res Commun. 1982 Oct 29;108(4):1495–1501. doi: 10.1016/s0006-291x(82)80076-4. [DOI] [PubMed] [Google Scholar]
- Kouri J., Noa M., Diaz B., Niubo E. Hyperpolarisation of rat peritoneal macrophages phagocytosing latex particles. Nature. 1980 Feb 28;283(5750):868–869. doi: 10.1038/283868a0. [DOI] [PubMed] [Google Scholar]
- Lampidis T. J., Hasin Y., Weiss M. J., Chen L. B. Selective killing of carcinoma cells "in vitro" by lipophilic-cationic compounds: a cellular basis. Biomed Pharmacother. 1985;39(5):220–226. [PubMed] [Google Scholar]
- Leach F. R., Webster J. J. Commercially available firefly luciferase reagents. Methods Enzymol. 1986;133:51–70. doi: 10.1016/0076-6879(86)33055-6. [DOI] [PubMed] [Google Scholar]
- Lichtshtein D., Kaback H. R., Blume A. J. Use of a lipophilic cation for determination of membrane potential in neuroblastoma-glioma hybrid cell suspensions. Proc Natl Acad Sci U S A. 1979 Feb;76(2):650–654. doi: 10.1073/pnas.76.2.650. [DOI] [PMC free article] [PubMed] [Google Scholar]
- Loew L. M., Benson L., Lazarovici P., Rosenberg I. Fluorometric analysis of transferable membrane pores. Biochemistry. 1985 Apr 23;24(9):2101–2104. doi: 10.1021/bi00330a001. [DOI] [PubMed] [Google Scholar]
- McCaig D. J., Berlin R. D. Transmembrane potential of J774.2 mouse macrophage cells measured by microelectrode and ion distribution methods. Experientia. 1983 Aug 15;39(8):906–907. doi: 10.1007/BF01990431. [DOI] [PubMed] [Google Scholar]
- Melmed R. N., Karanian P. J., Berlin R. D. Control of cell volume in the J774 macrophage by microtubule disassembly and cyclic AMP. J Cell Biol. 1981 Sep;90(3):761–768. doi: 10.1083/jcb.90.3.761. [DOI] [PMC free article] [PubMed] [Google Scholar]
- Okada Y., Ogawa M., Aoki N., Izutsu K. The effect of K + on the membrane potential in HeLa cells. Biochim Biophys Acta. 1973 Jan 2;291(1):116–126. doi: 10.1016/0005-2736(73)90066-7. [DOI] [PubMed] [Google Scholar]
- Pollack L. R., Tate E. H., Cook J. S. Na+, K+-ATPase in HeLa cells after prolonged growth in low K+ or ouabain. J Cell Physiol. 1981 Jan;106(1):85–97. doi: 10.1002/jcp.1041060110. [DOI] [PubMed] [Google Scholar]
- Roy G., Sauvé R. Stable membrane potentials and mechanical K+ responses activated by internal Ca2+ in HeLa cells. Can J Physiol Pharmacol. 1983 Feb;61(2):144–148. doi: 10.1139/y83-021. [DOI] [PubMed] [Google Scholar]
- Seligmann B. E., Gallin E. K., Martin D. L., Shain W., Gallin J. I. Interaction of chemotactic factors with human polymorphonuclear leukocytes: studies using a membrane potential-sensitive cyanine dye. J Membr Biol. 1980;52(3):257–272. doi: 10.1007/BF01869194. [DOI] [PubMed] [Google Scholar]
- Seligmann B. E., Gallin J. I. Use of lipophilic probes of membrane potential to assess human neutrophil activation. Abnormality in chronic granulomatous disease. J Clin Invest. 1980 Sep;66(3):493–503. doi: 10.1172/JCI109880. [DOI] [PMC free article] [PubMed] [Google Scholar]
- Simchowitz L., Spilberg I., De Weer P. Sodium and potassium fluxes and membrane potential of human neutrophils: evidence for an electrogenic sodium pump. J Gen Physiol. 1982 Mar;79(3):453–479. doi: 10.1085/jgp.79.3.453. [DOI] [PMC free article] [PubMed] [Google Scholar]
- Sims P. J., Waggoner A. S., Wang C. H., Hoffman J. F. Studies on the mechanism by which cyanine dyes measure membrane potential in red blood cells and phosphatidylcholine vesicles. Biochemistry. 1974 Jul 30;13(16):3315–3330. doi: 10.1021/bi00713a022. [DOI] [PubMed] [Google Scholar]
- Smith J. C., Russ P., Cooperman B. S., Chance B. Synthesis, structure determination, spectral properties, and energy-linked spectral responses of the extrinsic probe oxonol V in membranes. Biochemistry. 1976 Nov 16;15(23):5094–5105. doi: 10.1021/bi00668a023. [DOI] [PubMed] [Google Scholar]
- Steinman R. M., Brodie S. E., Cohn Z. A. Membrane flow during pinocytosis. A stereologic analysis. J Cell Biol. 1976 Mar;68(3):665–687. doi: 10.1083/jcb.68.3.665. [DOI] [PMC free article] [PubMed] [Google Scholar]
- Sung S. S., Young J. D., Origlio A. M., Heiple J. M., Kaback H. R., Silverstein S. C. Extracellular ATP perturbs transmembrane ion fluxes, elevates cytosolic [Ca2+], and inhibits phagocytosis in mouse macrophages. J Biol Chem. 1985 Nov 5;260(25):13442–13449. [PubMed] [Google Scholar]
- Tatham P. E., Delves P. J., Shen L., Roitt I. M. Chemotactic factor-induced membrane potential changes in rabbit neutrophils monitored by the fluorescent dye 3,3'-dipropylthiadicarbocyanine iodide. Biochim Biophys Acta. 1980 Nov 4;602(2):285–298. doi: 10.1016/0005-2736(80)90312-0. [DOI] [PubMed] [Google Scholar]
- Walter R. J., Berlin R. D., Pfeiffer J. R., Oliver J. M. Polarization of endocytosis and receptor topography on cultured macrophages. J Cell Biol. 1980 Jul;86(1):199–211. doi: 10.1083/jcb.86.1.199. [DOI] [PMC free article] [PubMed] [Google Scholar]
- White J. R., Huang C. K., Hill J. M., Jr, Naccache P. H., Becker E. L., Sha'afi R. I. Effect of phorbol 12-myristate 13-acetate and its analogue 4 alpha-phorbol 12,13-didecanoate on protein phosphorylation and lysosomal enzyme release in rabbit neutrophils. J Biol Chem. 1984 Jul 10;259(13):8605–8611. [PubMed] [Google Scholar]
- Young J. D., Unkeless J. C., Kaback H. R., Cohn Z. A. Mouse macrophage Fc receptor for IgG gamma 2b/gamma 1 in artificial and plasma membrane vesicles functions as a ligand-dependent ionophore. Proc Natl Acad Sci U S A. 1983 Mar;80(6):1636–1640. doi: 10.1073/pnas.80.6.1636. [DOI] [PMC free article] [PubMed] [Google Scholar]
- Young J. D., Young T. M., Lu L. P., Unkeless J. C., Cohn Z. A. Characterization of a membrane pore-forming protein from Entamoeba histolytica. J Exp Med. 1982 Dec 1;156(6):1677–1690. doi: 10.1084/jem.156.6.1677. [DOI] [PMC free article] [PubMed] [Google Scholar]