Abstract
When cells are frozen slowly in aqueous suspensions, the solutes in the suspending solution concentrate as the amount of ice increases; the cells undergo osmotic dehydration and are sequestered in ever-narrowing liquid-filled channels. Cryoprotective solutes, such as glycerol, reduce the amount of ice that forms at any specified subzero temperature, thereby controlling the buildup in concentration of those other solutes present, as well as increasing the volume of the channels that remain to accommodate the cells. It has generally been thought that freezing injury is mediated by the increase in electrolyte concentration in the milieu surrounding the cells, rather than reduction of temperature or any direct action of ice. In this study we have frozen human erythrocytes in isotonic solutions of sodium chloride and glycerol and have demonstrated a correlation between the extent of damage at specific subzero temperatures, and that caused by the action at 0 degrees C of solutions having the same composition as those produced by freezing. The cell lysis observed increased directly with glycerol concentration, both in the freezing experiments and when the cells were exposed to corresponding solutions at 0 degrees C, showing that the concentration of sodium chloride alone is not sufficient to account quantitatively for the damage observed. We then studied the effect of freezing in anisotonic solutions to break the fixed relationship between solute concentration and the volume of the unfrozen fraction, as described by Mazur, P., W. F. Rall, and N. Rigopoulos (1981. Biophys. J. 653-675). We confirmed their experimental findings, but we explain them differently. We ascribe the apparently dominant effect of the unfrozen fraction to the fact that the cells were frozen in, and returned to, anisotonic solutions in which their volume was either less than, or greater than, their physiological volume. When similar cell suspensions were subjected to a similar cycle of increase and then decrease in solution strength, but in the absence of ice (at 20 degrees C), a similar pattern of hemolysis was observed. We conclude that freezing injury to human erythrocytes is due solely to changes that occur in the composition of their surrounding milieu, and is most probably mediated by a temporary leak in the plasma membrane that occurs during the thawing (reexpansion) phase.
Full text
PDF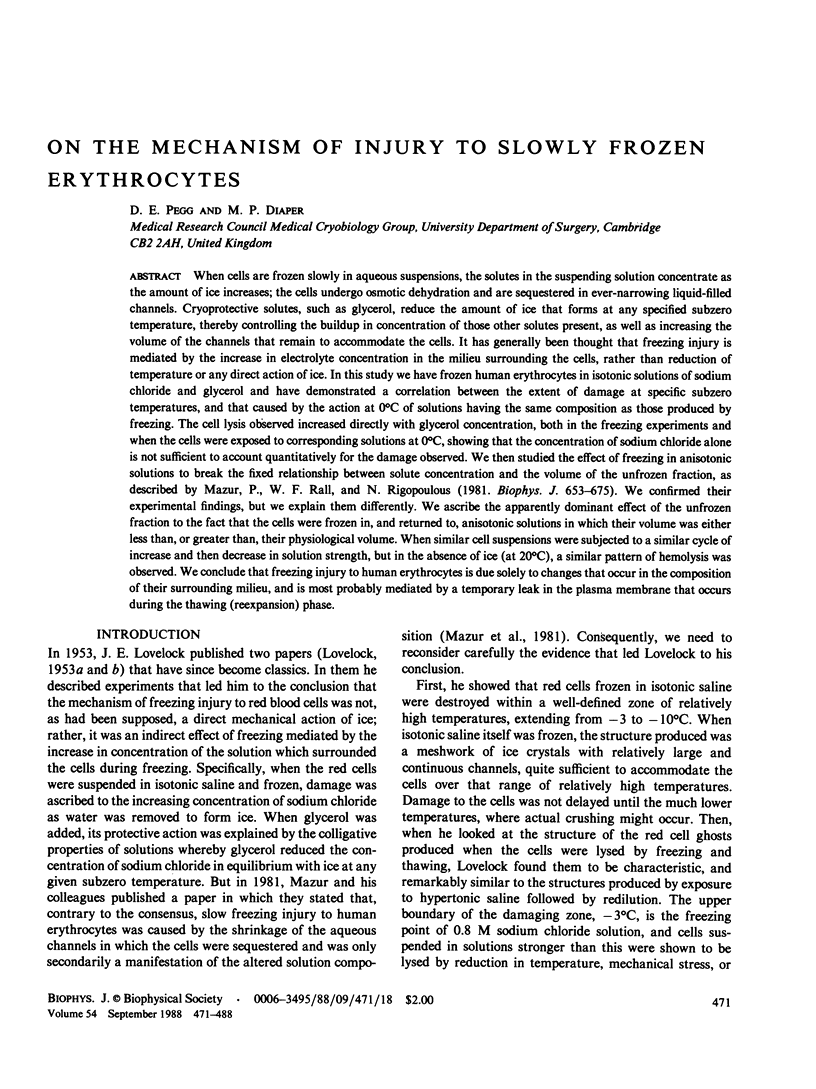
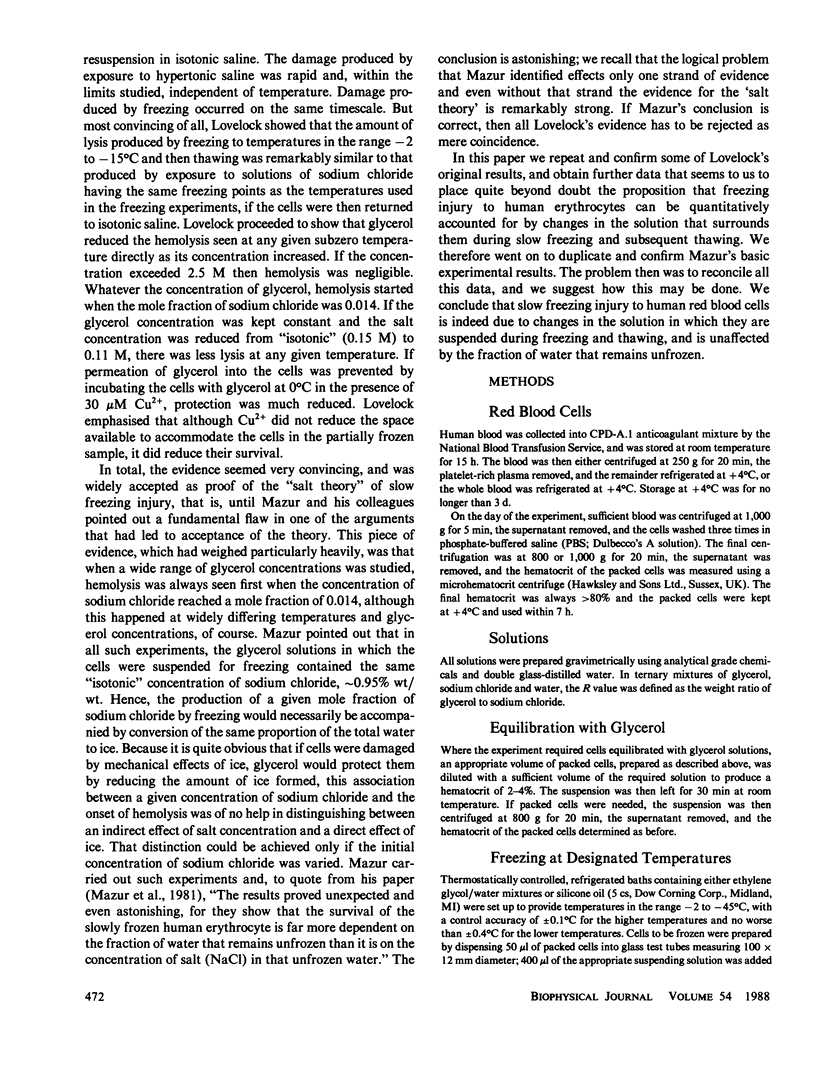
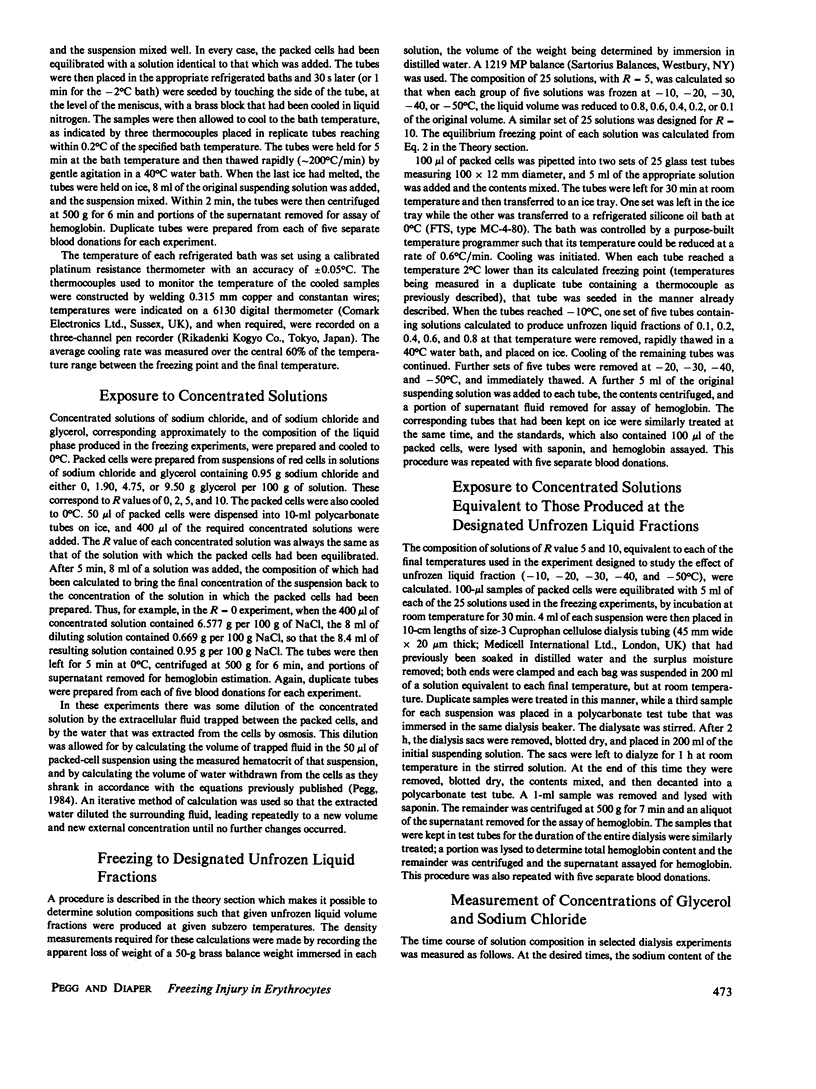
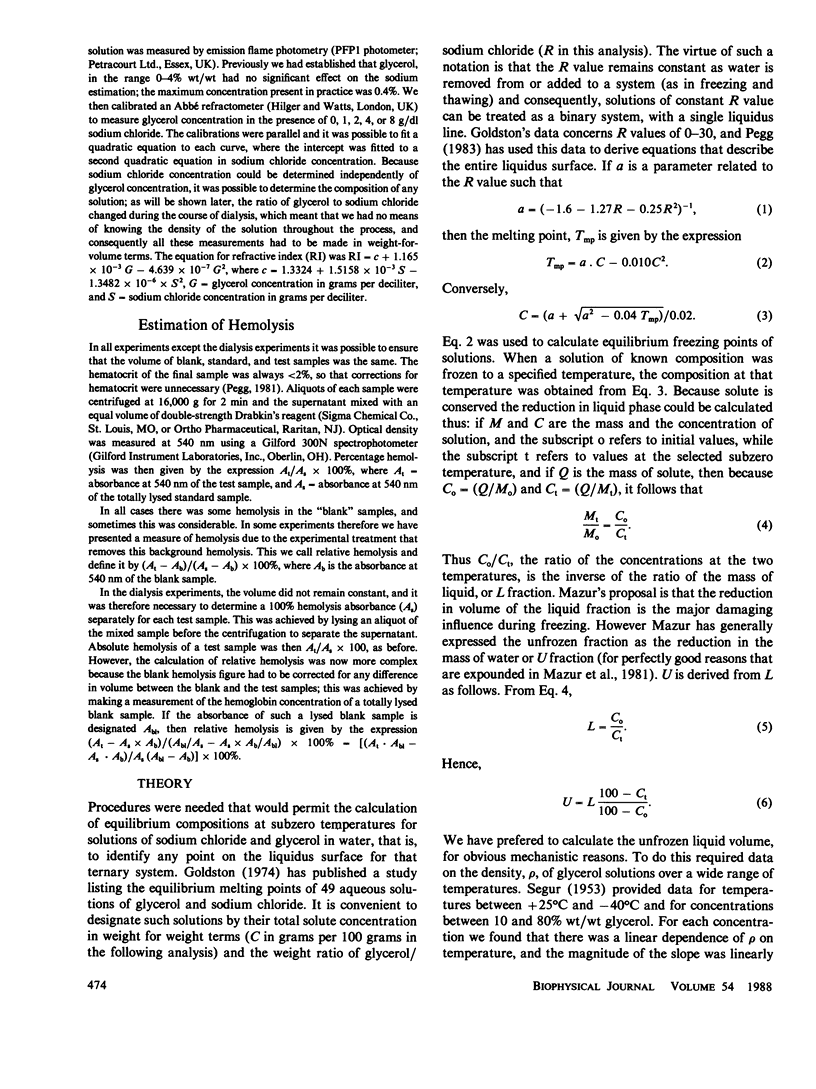
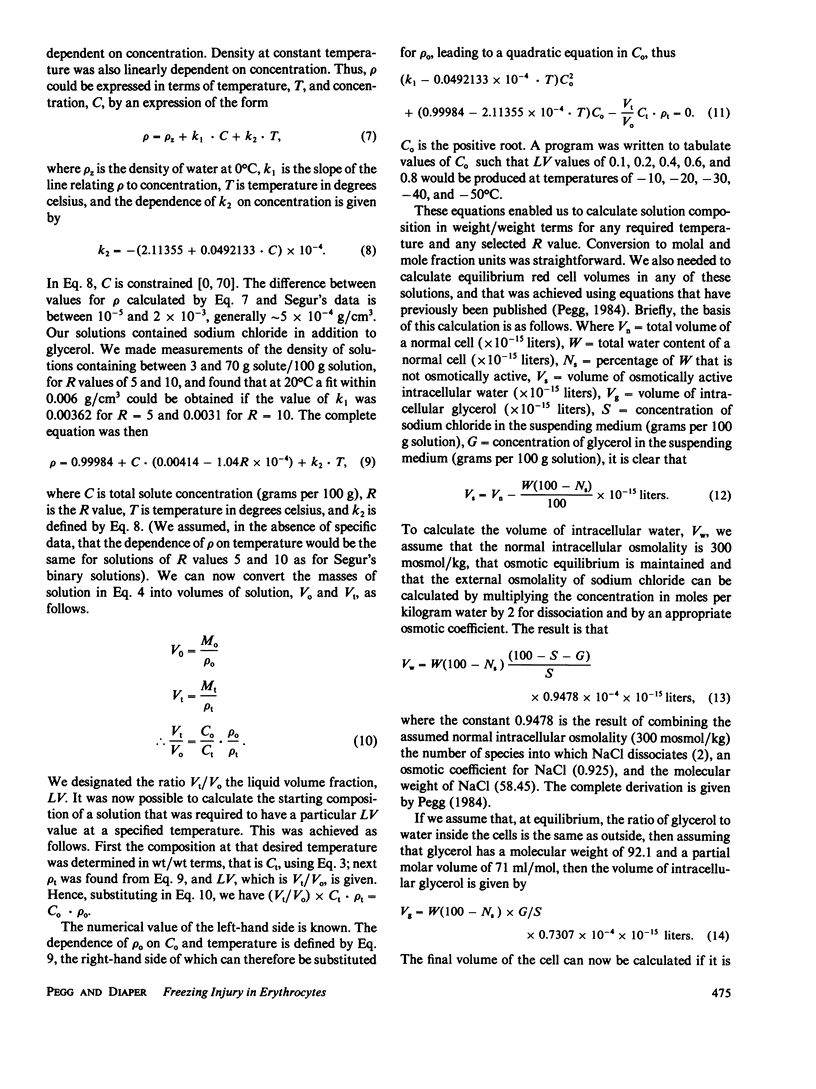
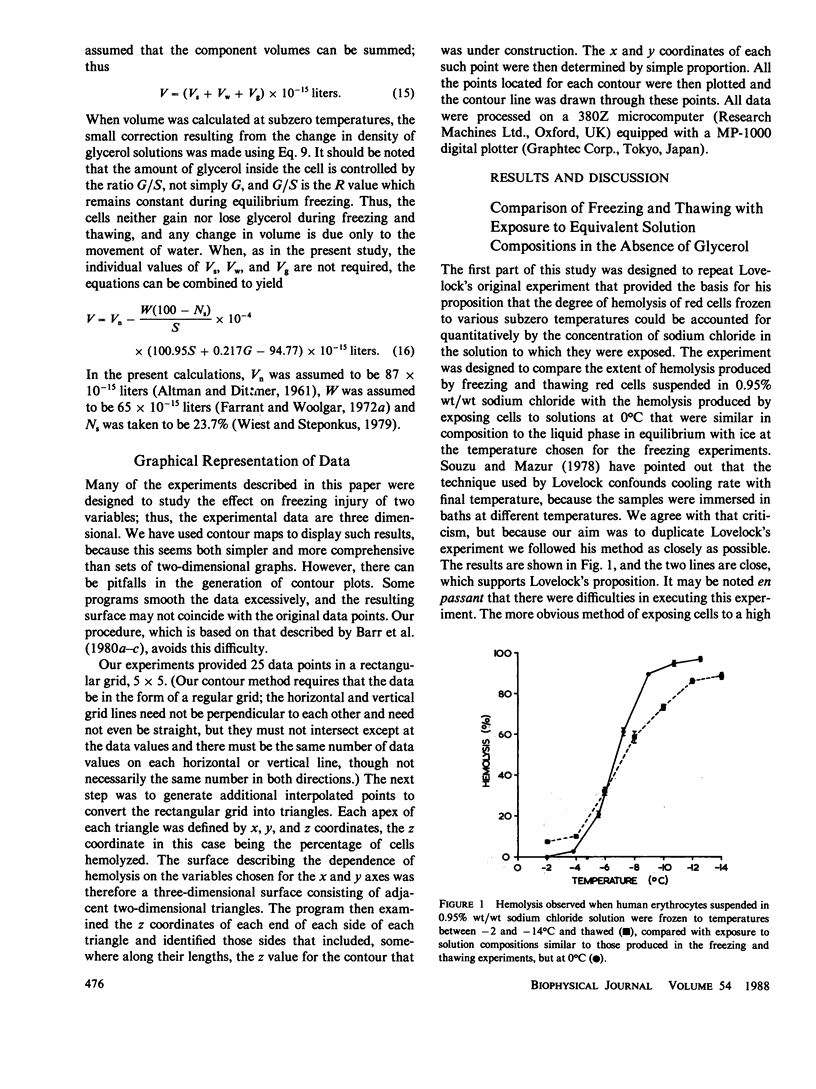
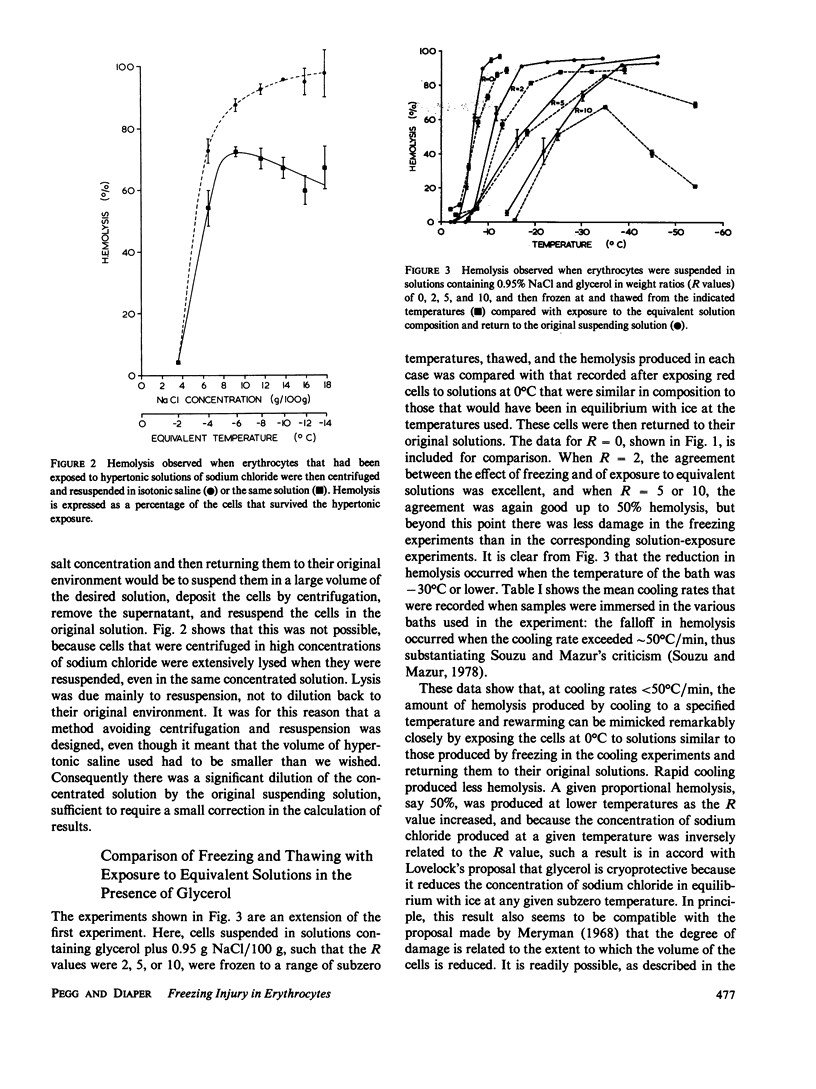
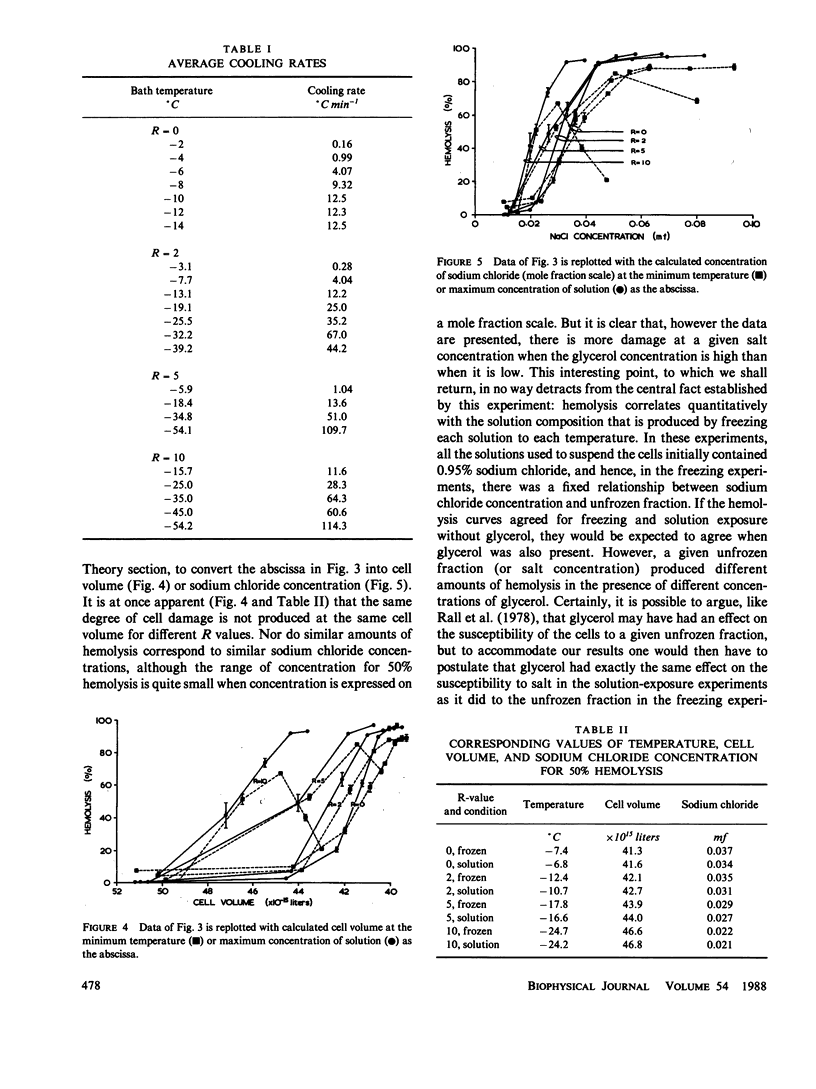
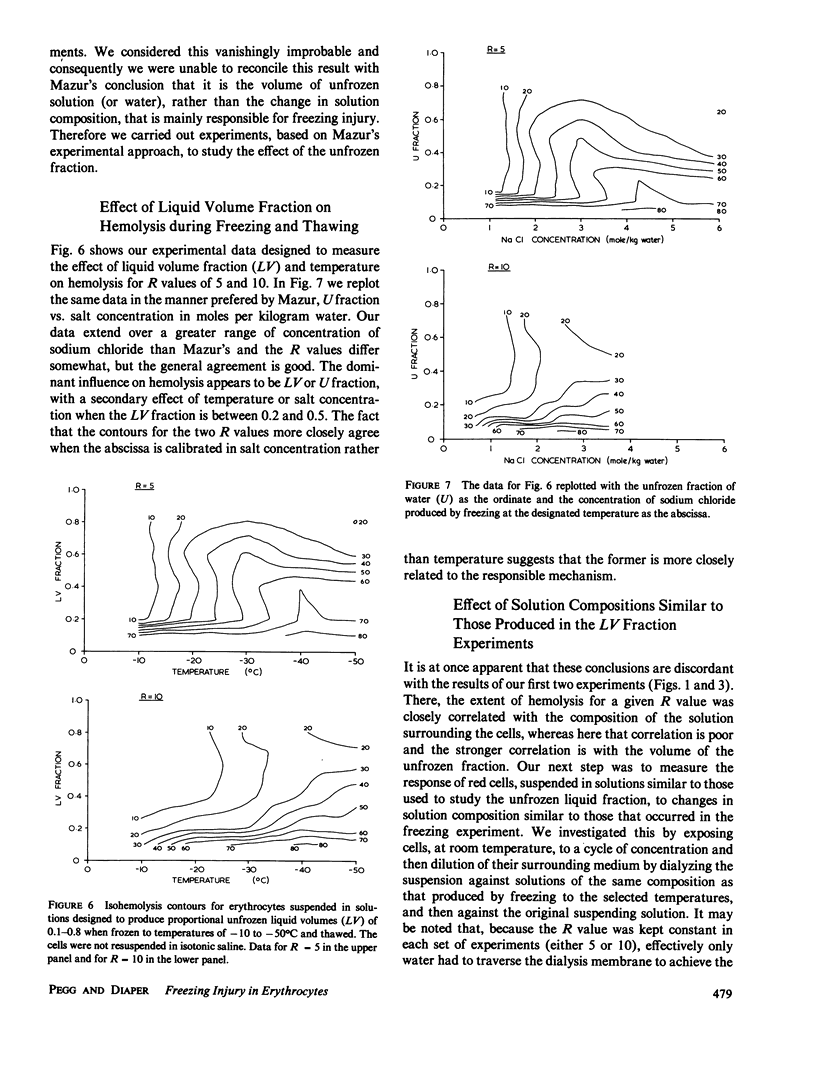
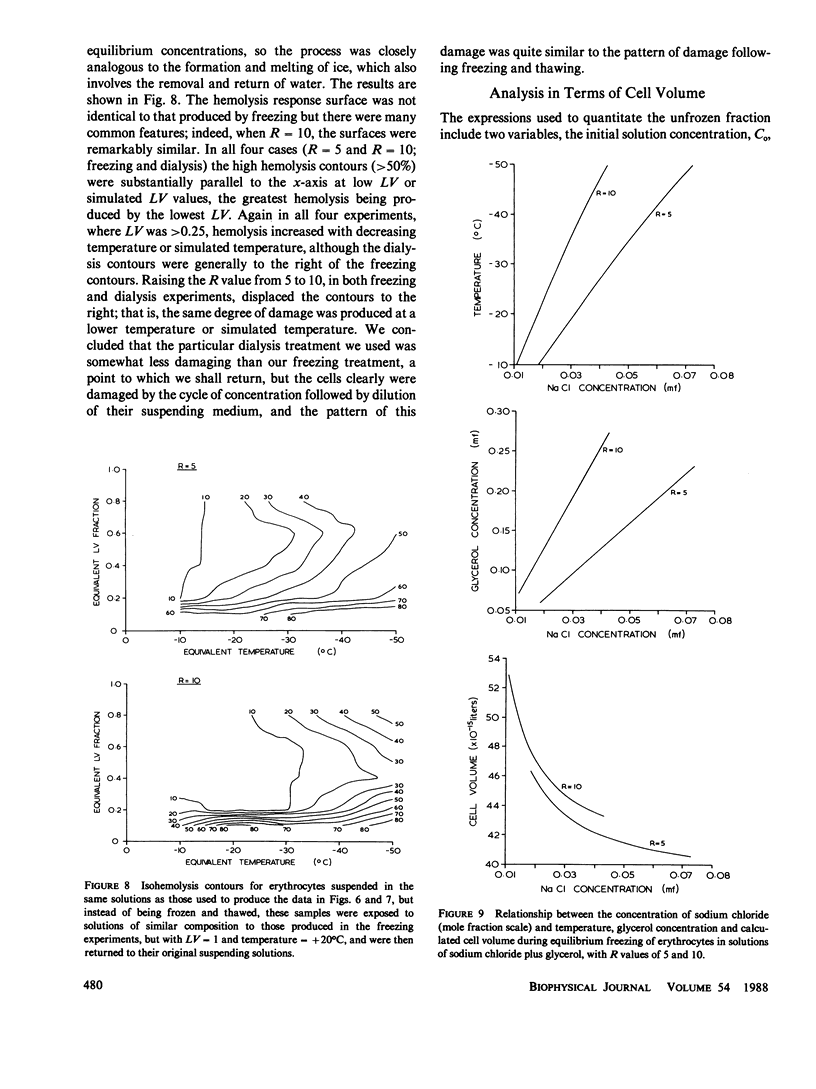
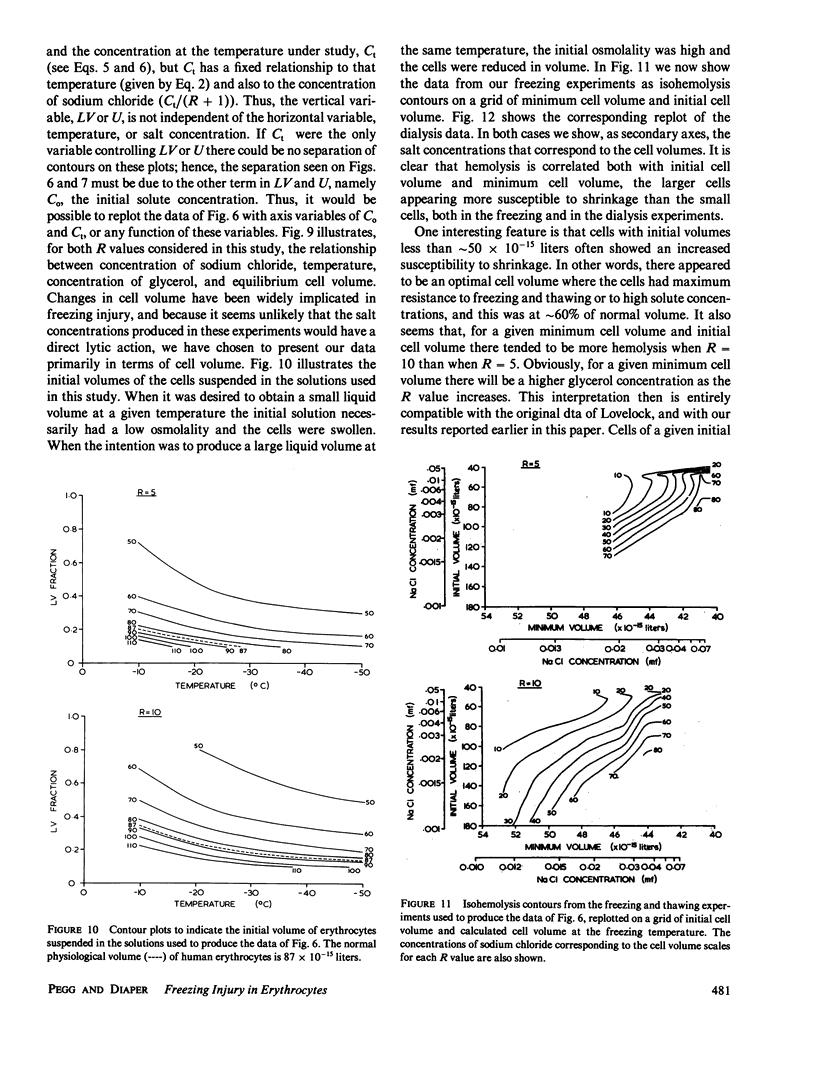
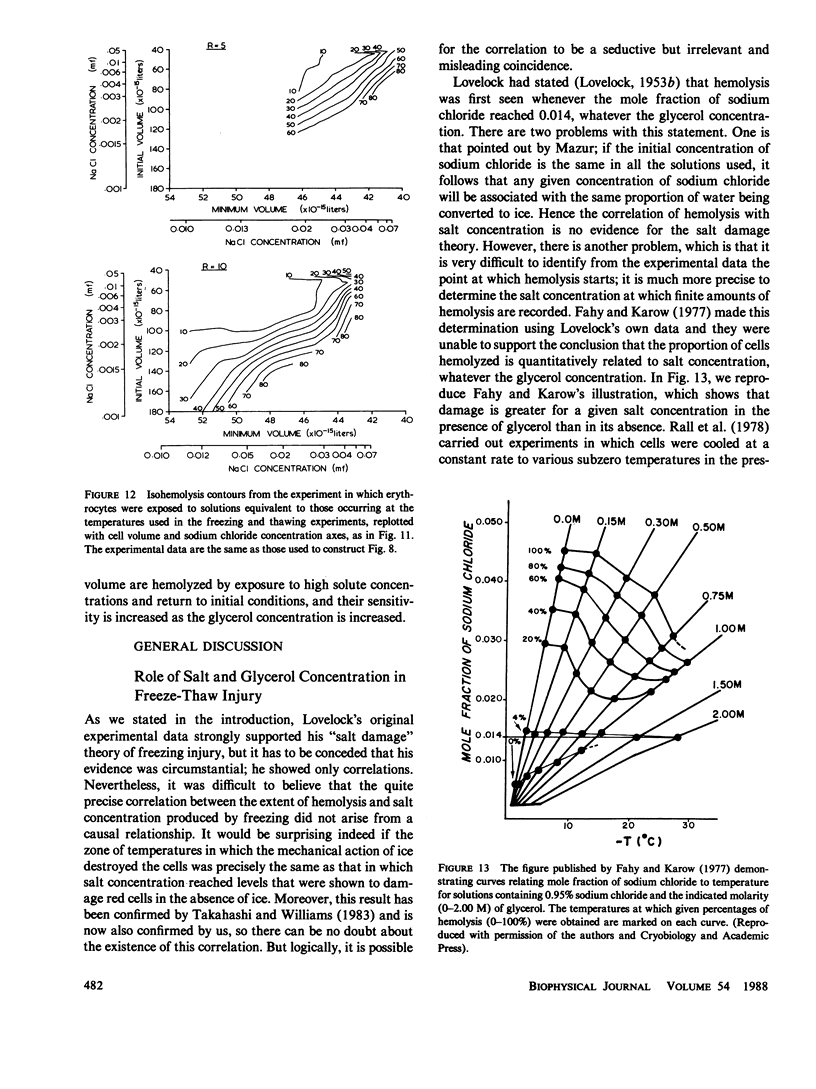
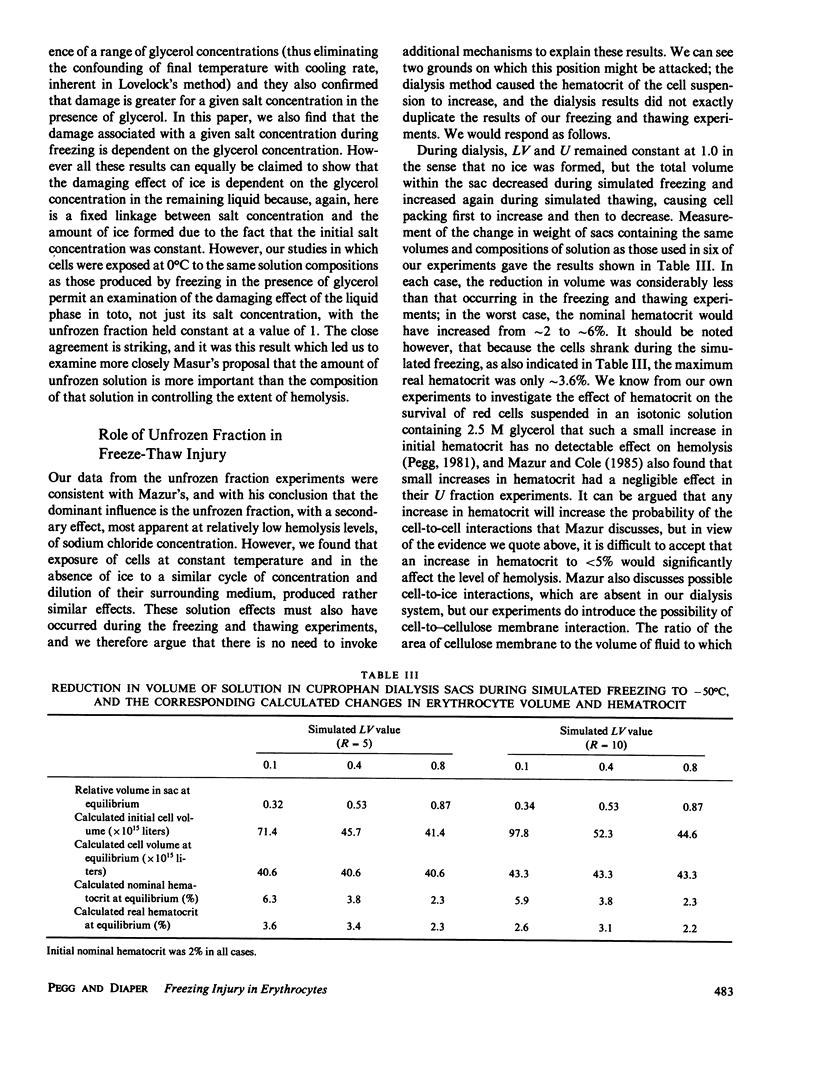
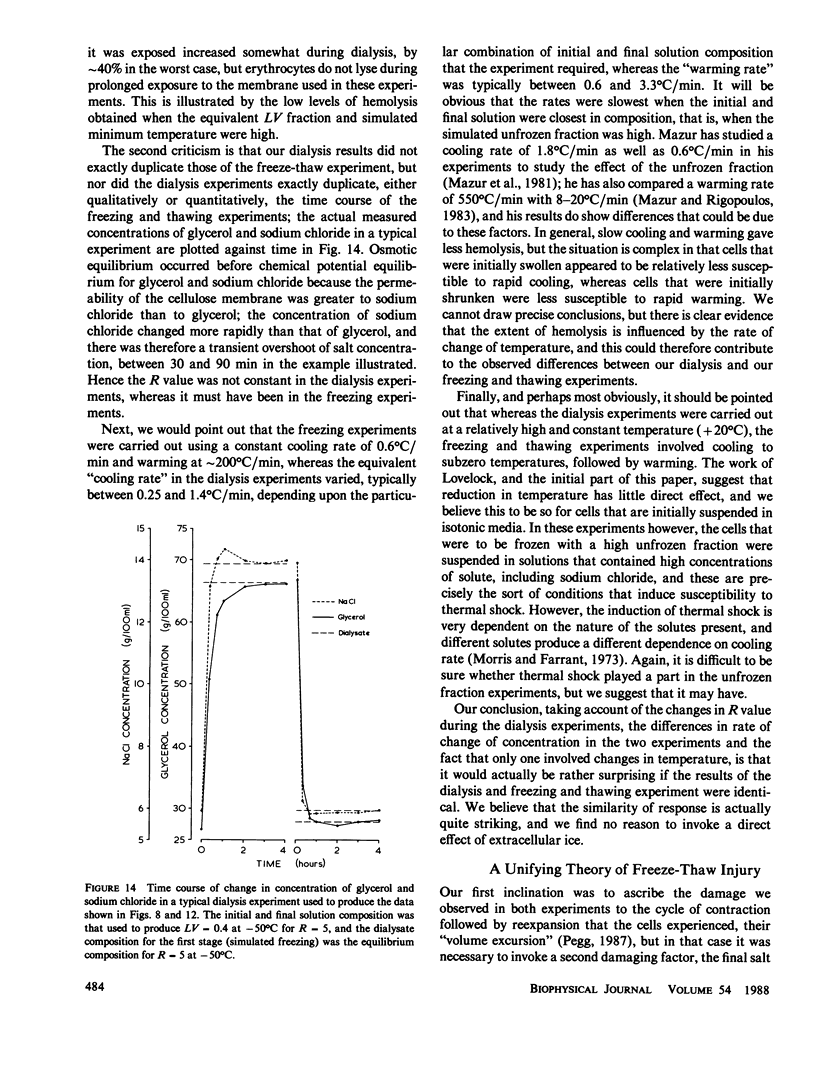
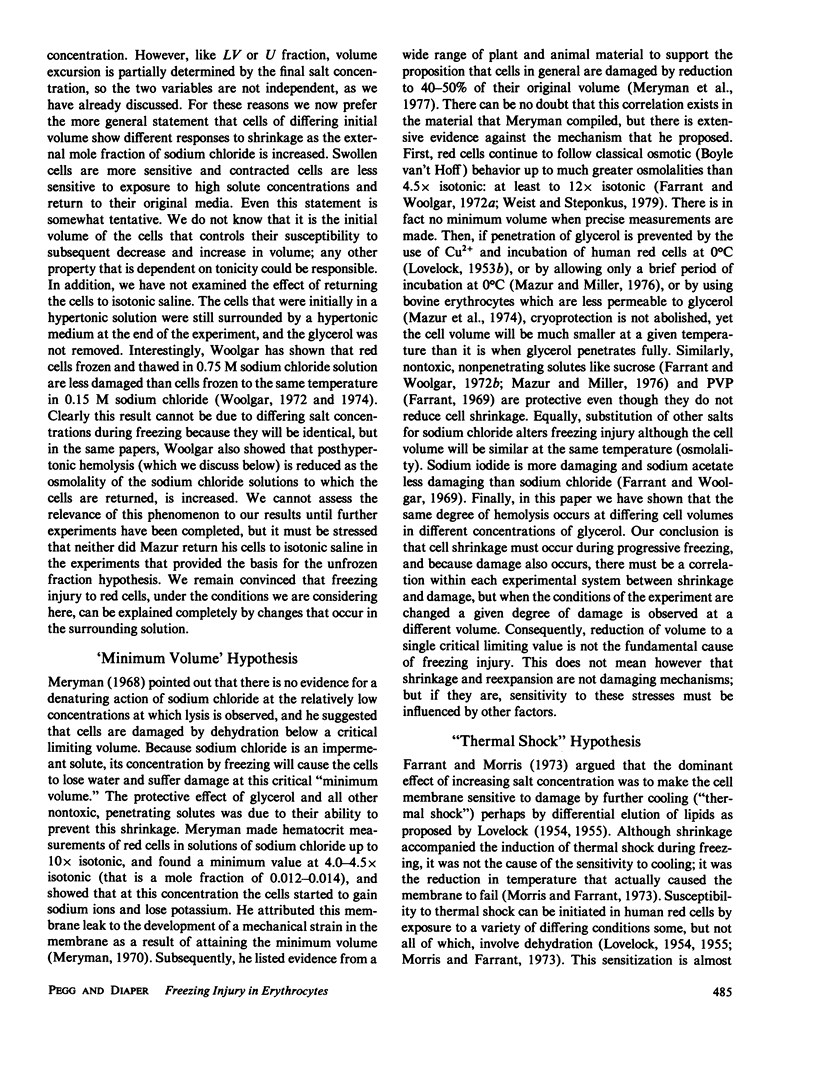
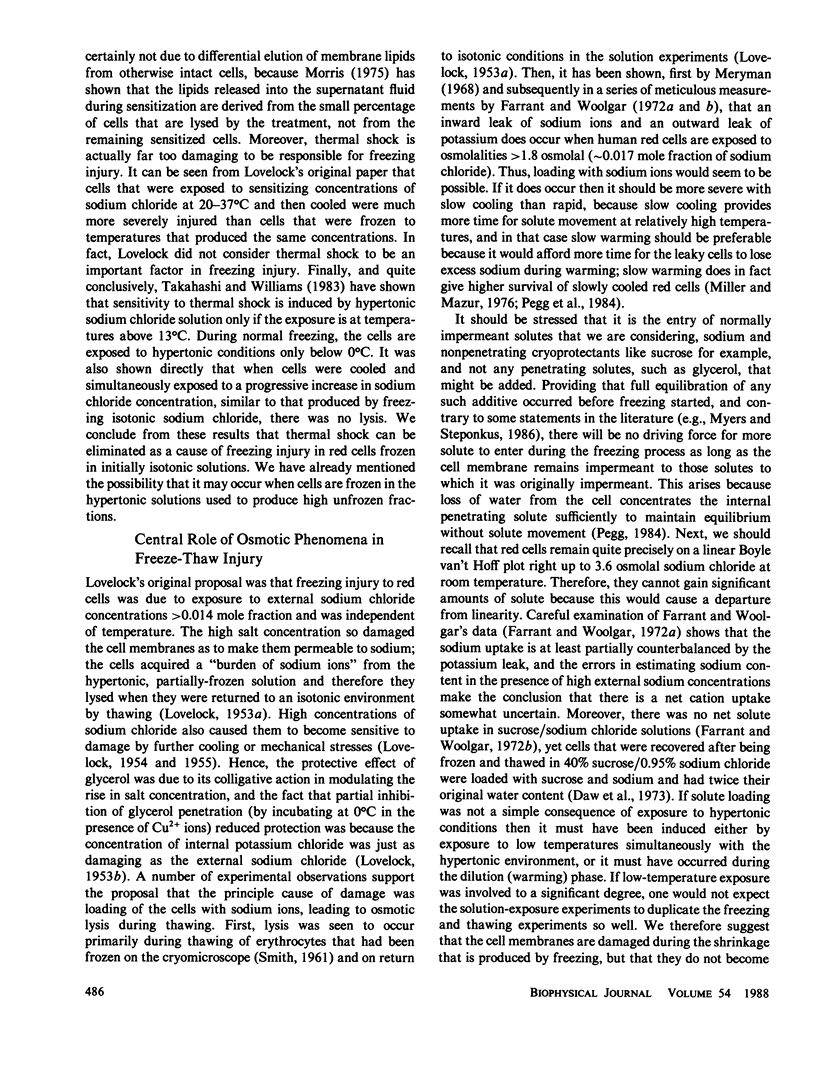
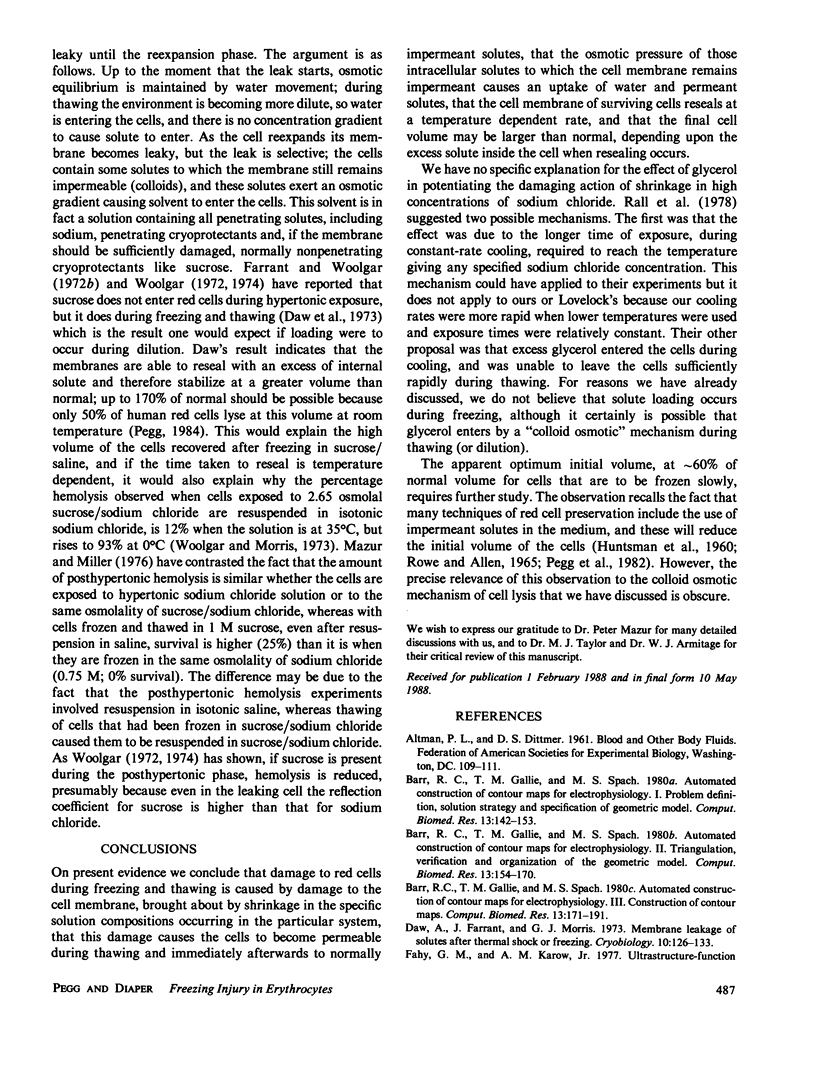
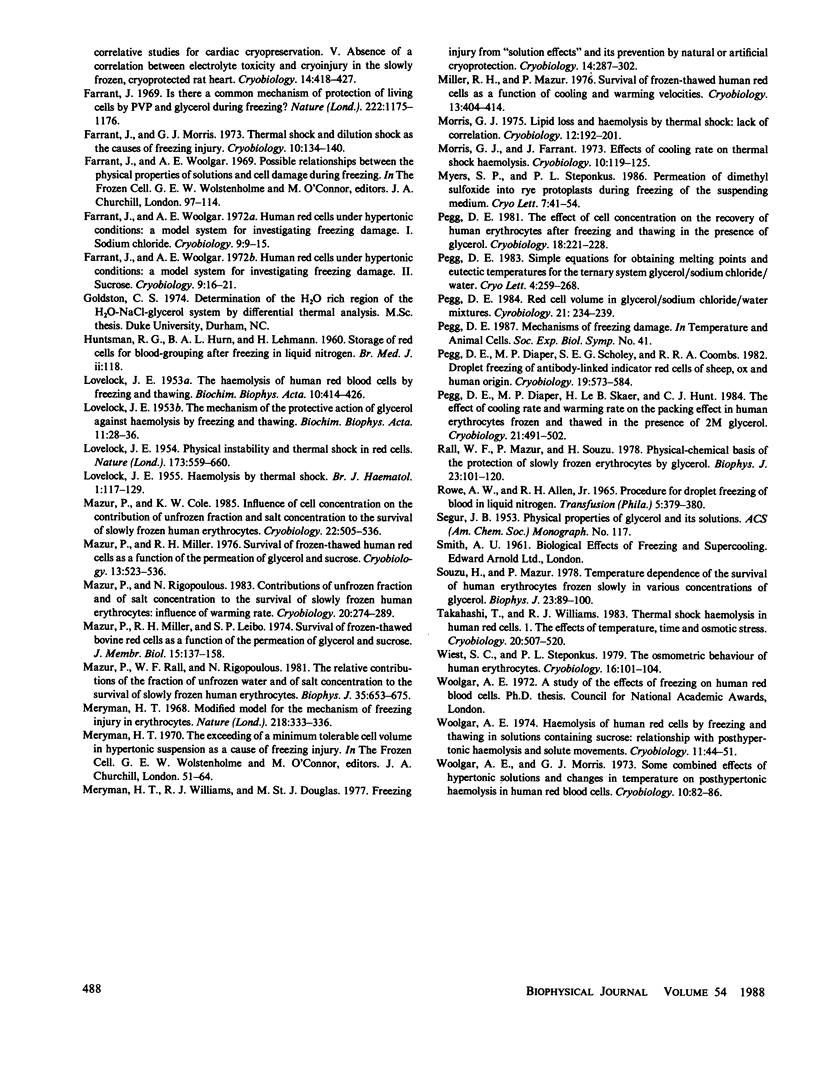
Selected References
These references are in PubMed. This may not be the complete list of references from this article.
- Barr R. C., Gallie T. M., Spach M. S. Automated production of contour maps for electrophysiology. I. Problem definition, solution strategy, and specification of geometric model. Comput Biomed Res. 1980 Apr;13(2):142–153. doi: 10.1016/0010-4809(80)90013-0. [DOI] [PubMed] [Google Scholar]
- Barr R. C., Gallie T. M., Spach M. S. Automated production of contour maps for electrophysiology. II. Triangulation, verification, and organization of the geometric model. Comput Biomed Res. 1980 Apr;13(2):154–170. doi: 10.1016/0010-4809(80)90014-2. [DOI] [PubMed] [Google Scholar]
- Barr R. C., Gallie T. M., Spach M. S. Automated production of contour maps for electrophysiology. III. Construction of contour maps. Comput Biomed Res. 1980 Apr;13(2):171–191. doi: 10.1016/0010-4809(80)90015-4. [DOI] [PubMed] [Google Scholar]
- Daw A., Farrant J., Morris G. J. Membrane leakage of solutes after thermal shock or freezing. Cryobiology. 1973 Jun;10(2):126–133. doi: 10.1016/0011-2240(73)90018-7. [DOI] [PubMed] [Google Scholar]
- Fahy G. M., Karow A. M., Jr Ultrastructure-function correlative studies for cardiac cryopreservation. V. Absence of a correlation between electrolyte toxicity and cryoinjury in the slowly frozen, cryoprotected rat heart. Cryobiology. 1977 Aug;14(4):418–427. doi: 10.1016/0011-2240(77)90003-7. [DOI] [PubMed] [Google Scholar]
- Farrant J. Is there a common mechanism of protection of living cells by polyvinylpyrrolidone and glycerol ding freezing? Nature. 1969 Jun 21;222(5199):1175–1176. doi: 10.1038/2221175a0. [DOI] [PubMed] [Google Scholar]
- Farrant J., Morris G. J. Thermal shock and dilution shock as the causes of freezing injury. Cryobiology. 1973 Jun;10(2):134–140. doi: 10.1016/0011-2240(73)90019-9. [DOI] [PubMed] [Google Scholar]
- Farrant J., Woolgar A. E. Human red cells under hypertonic conditions; a model system for investigating freezing damage. 2. Sucrose. Cryobiology. 1972 Feb;9(1):16–21. doi: 10.1016/0011-2240(72)90004-1. [DOI] [PubMed] [Google Scholar]
- Farrant J., Woolgar A. E. Human red cells under hypertonic conditions; a model system for investigating freezing damage. I. Sodium chloride. Cryobiology. 1972 Feb;9(1):9–15. doi: 10.1016/0011-2240(72)90003-x. [DOI] [PubMed] [Google Scholar]
- LOVELOCK J. E. Haemolysis by thermal shock. Br J Haematol. 1955 Jan;1(1):117–129. doi: 10.1111/j.1365-2141.1955.tb05493.x. [DOI] [PubMed] [Google Scholar]
- LOVELOCK J. E. The haemolysis of human red blood-cells by freezing and thawing. Biochim Biophys Acta. 1953 Mar;10(3):414–426. doi: 10.1016/0006-3002(53)90273-x. [DOI] [PubMed] [Google Scholar]
- LOVELOCK J. E. The mechanism of the protective action of glycerol against haemolysis by freezing and thawing. Biochim Biophys Acta. 1953 May;11(1):28–36. doi: 10.1016/0006-3002(53)90005-5. [DOI] [PubMed] [Google Scholar]
- Mazur P., Cole K. W. Influence of cell concentration on the contribution of unfrozen fraction and salt concentration to the survival of slowly frozen human erythrocytes. Cryobiology. 1985 Dec;22(6):509–536. doi: 10.1016/0011-2240(85)90029-x. [DOI] [PubMed] [Google Scholar]
- Mazur P., Miller R. H., Leibo S. P. Survival of frozen-thawed bovine red cells as a function of the permeation of glycerol and sucrose. J Membr Biol. 1974;15(2):137–158. doi: 10.1007/BF01870085. [DOI] [PubMed] [Google Scholar]
- Mazur P., Miller R. H. Survival of frozen-thawed human red cells as a function of the permeation of glycerol and sucrose. Cryobiology. 1976 Oct;13(5):523–536. doi: 10.1016/0011-2240(76)90145-0. [DOI] [PubMed] [Google Scholar]
- Mazur P., Rall W. F., Rigopoulos N. Relative contributions of the fraction of unfrozen water and of salt concentration to the survival of slowly frozen human erythrocytes. Biophys J. 1981 Dec;36(3):653–675. doi: 10.1016/S0006-3495(81)84757-1. [DOI] [PMC free article] [PubMed] [Google Scholar]
- Mazur P., Rigopoulos N. Contributions of unfrozen fraction and of salt concentration to the survival of slowly frozen human erythrocytes: influence of warming rate. Cryobiology. 1983 Jun;20(3):274–289. doi: 10.1016/0011-2240(83)90016-0. [DOI] [PubMed] [Google Scholar]
- Meryman H. T. Modified model for the mechanism of freezing injury in erythrocytes. Nature. 1968 Apr 27;218(5139):333–336. doi: 10.1038/218333a0. [DOI] [PubMed] [Google Scholar]
- Meryman H. T., Williams R. J., Douglas M. S. Freezing injury from "solution effects" and its prevention by natural or artificial cryoprotection. Cryobiology. 1977 Jun;14(3):287–302. doi: 10.1016/0011-2240(77)90177-8. [DOI] [PubMed] [Google Scholar]
- Miller R. H., Mazur P. Survival of frozen-thawed human red cells as a function of cooling and warming velocities. Cryobiology. 1976 Aug;13(4):404–414. doi: 10.1016/0011-2240(76)90096-1. [DOI] [PubMed] [Google Scholar]
- Morris G. J., Farrant J. Effects of cooling rate on thermal shock hemolysis. Cryobiology. 1973 Jun;10(2):119–125. doi: 10.1016/0011-2240(73)90017-5. [DOI] [PubMed] [Google Scholar]
- Morris G. J. Lipid loss and haemolysis by thermal shock: lack of correlation. Cryobiology. 1975 Jun;12(3):192–201. doi: 10.1016/0011-2240(75)90017-6. [DOI] [PubMed] [Google Scholar]
- Pegg D. E., Diaper M. P., Scholey S. E., Coombs R. R. Droplet freezing of antibody-linked indicator red cells of sheep, ox, and human origin. Cryobiology. 1982 Dec;19(6):573–584. doi: 10.1016/0011-2240(82)90187-0. [DOI] [PubMed] [Google Scholar]
- Pegg D. E., Diaper M. P., Skaer H. L., Hunt C. J. The effect of cooling rate and warming rate on the packing effect in human erythrocytes frozen and thawed in the presence of 2 M glycerol. Cryobiology. 1984 Oct;21(5):491–502. doi: 10.1016/0011-2240(84)90047-6. [DOI] [PubMed] [Google Scholar]
- Pegg D. E. Red cell volume in glycerol/sodium chloride/water mixtures. Cryobiology. 1984 Apr;21(2):234–239. doi: 10.1016/0011-2240(84)90214-1. [DOI] [PubMed] [Google Scholar]
- Pegg D. E. The effect of cell concentration on the recovery of human erythrocytes after freezing and thawing in the presence of glycerol. Cryobiology. 1981 Jun;18(3):221–228. doi: 10.1016/0011-2240(81)90092-4. [DOI] [PubMed] [Google Scholar]
- Rall W. F., Mazur P., Souzu H. Physical-chemical basis of the protection of slowly frozen human erythrocytes by glycerol. Biophys J. 1978 Jul;23(1):101–120. doi: 10.1016/S0006-3495(78)85436-8. [DOI] [PMC free article] [PubMed] [Google Scholar]
- Souzu H., Mazur P. Temperature dependence of the survival of human erythrocytes frozen slowly in various concentrations of glycerol. Biophys J. 1978 Jul;23(1):89–100. doi: 10.1016/S0006-3495(78)85435-6. [DOI] [PMC free article] [PubMed] [Google Scholar]
- Takahashi T., Williams R. J. Thermal shock hemolysis in human red cells. I. The effects of temperature, time, and osmotic stress. Cryobiology. 1983 Oct;20(5):507–520. doi: 10.1016/0011-2240(83)90039-1. [DOI] [PubMed] [Google Scholar]
- Wiest S. C., Steponkus P. L. The osmometric behavior of human erythrocytes. Cryobiology. 1979 Feb;16(1):101–104. doi: 10.1016/0011-2240(79)90018-x. [DOI] [PubMed] [Google Scholar]
- Woolgar A. E. Hemolysis of human red blood cells by freezing and thawing in solutions containing sucrose: relationship with posthypertonic hemolysis and solute movements. Cryobiology. 1974 Feb;11(1):44–51. doi: 10.1016/0011-2240(74)90037-6. [DOI] [PubMed] [Google Scholar]
- Woolgar A. E., Morris G. J. Some combined effects of hypertonic solutions and changes in temperature on posthypertonic hemolysis of human red blood cells. Cryobiology. 1973 Apr;10(1):82–86. doi: 10.1016/0011-2240(73)90011-4. [DOI] [PubMed] [Google Scholar]