Abstract
To elucidate the means by which polymer solutions protect cells from freezing injury, we cooled human monocytes to -80 degrees C or below in the presence of various polymers. Differential scanning calorimetric studies showed that those polymers which protect cells best have a limiting glass transition temperature (T'g) of approximately -20 degrees C; those with a T'g significantly higher or lower did not protect. Freeze-etch electron micrographs indicated that intracellular ice crystals had formed during this freezing procedure, but remained smaller than approximately 300 nm in the same proportion of cells as survived rapid thawing. We propose that cryoprotection of slowly frozen monocytes by polymers is a consequence of a T'g of -20 degrees C in the extracellular solution. In our hypothesis, the initial concentration and viscosity of protective polymer solutions reduce the extent and rate of cell water loss to extracellular ice and limit the injurious osmotic stress, which cells face during freezing at moderate rates to -20 degrees C. Below -20 degrees C, glass formation prevents further osmotic stress by isolating cells from extracellular ice crystals, virtually eliminating cell water loss at lower temperatures. On the other hand, the protective polymer solutions will allow some diffusion of water away from cells at temperatures above T'g. If conditions are correct, cells will concentrate the cytoplasm sufficiently during the initial cooling to T'g to avoid lethal intracellular freezing between T'g and the intracellular Tg, which has been depressed to low temperatures by that concentration. Thus, when polymers are used as cryoprotective agents, cell survival is contingent upon maintenance of osmotic stress within narrow limits.
Full text
PDF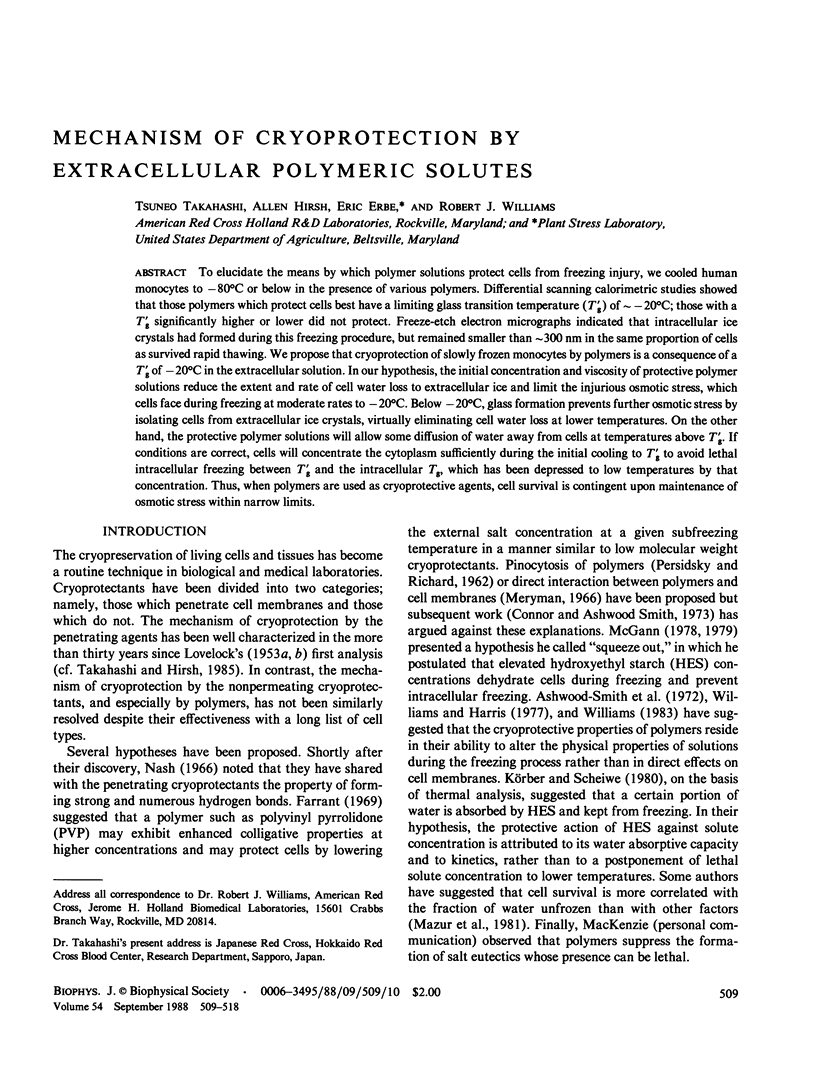
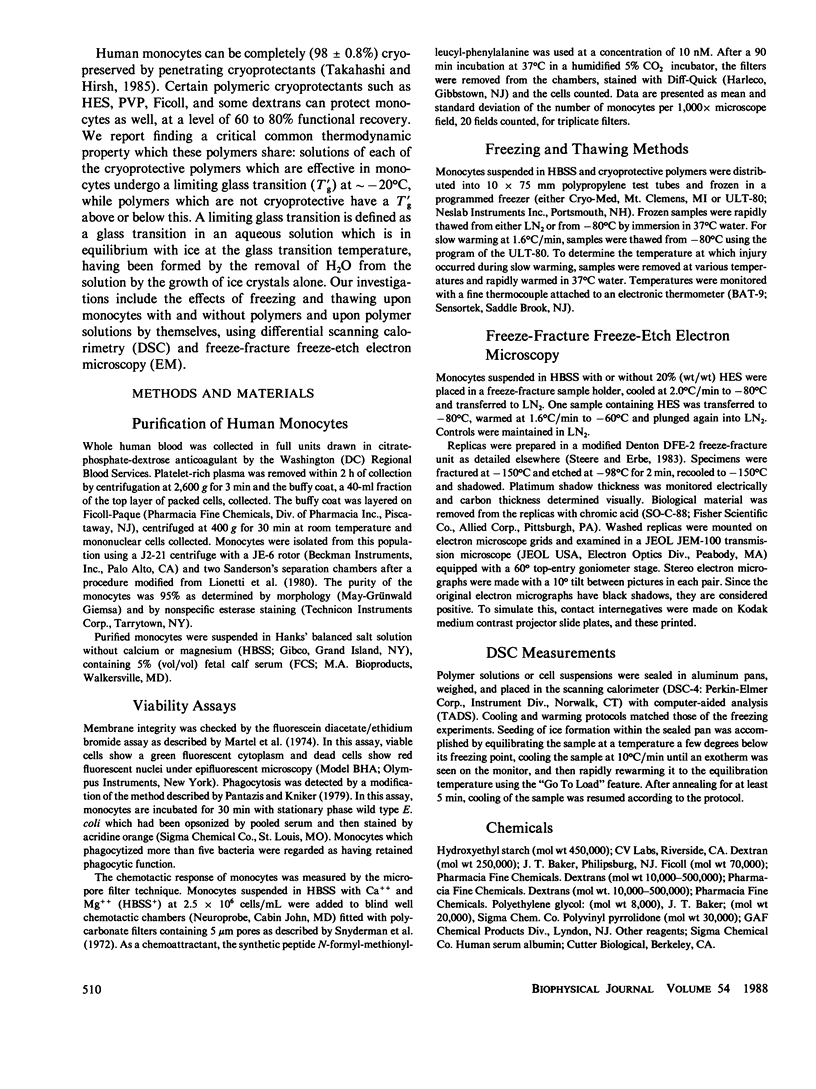
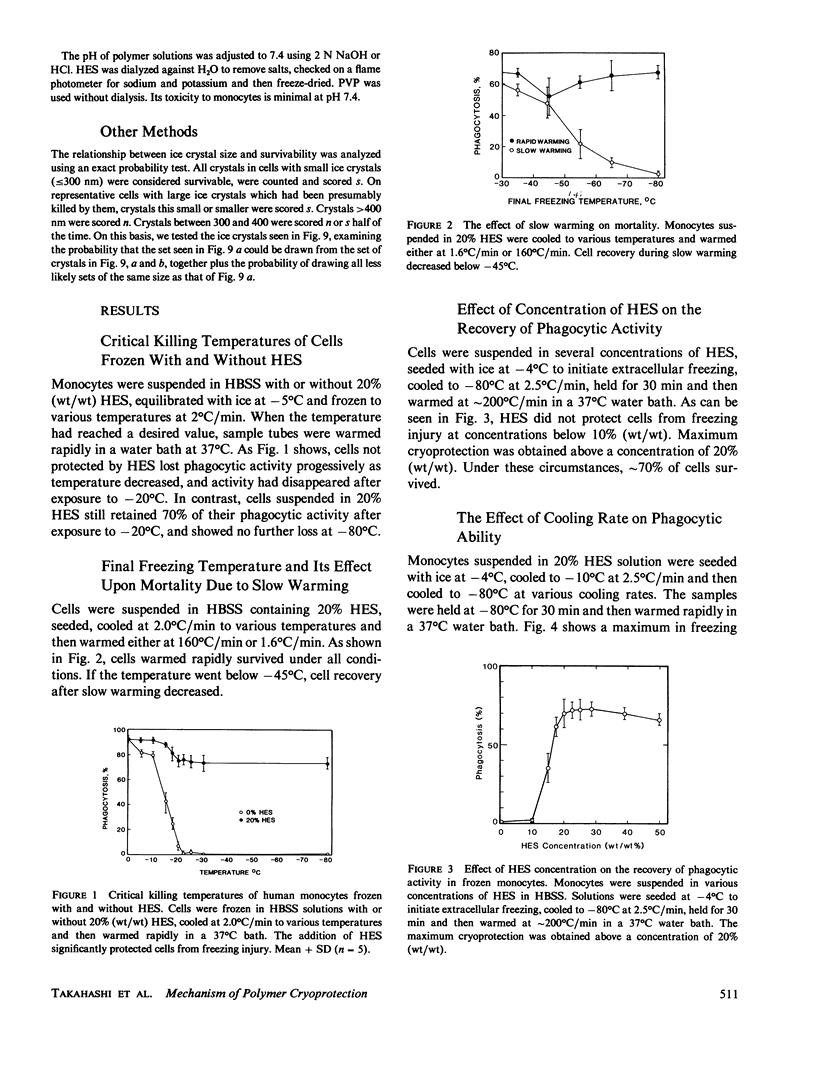
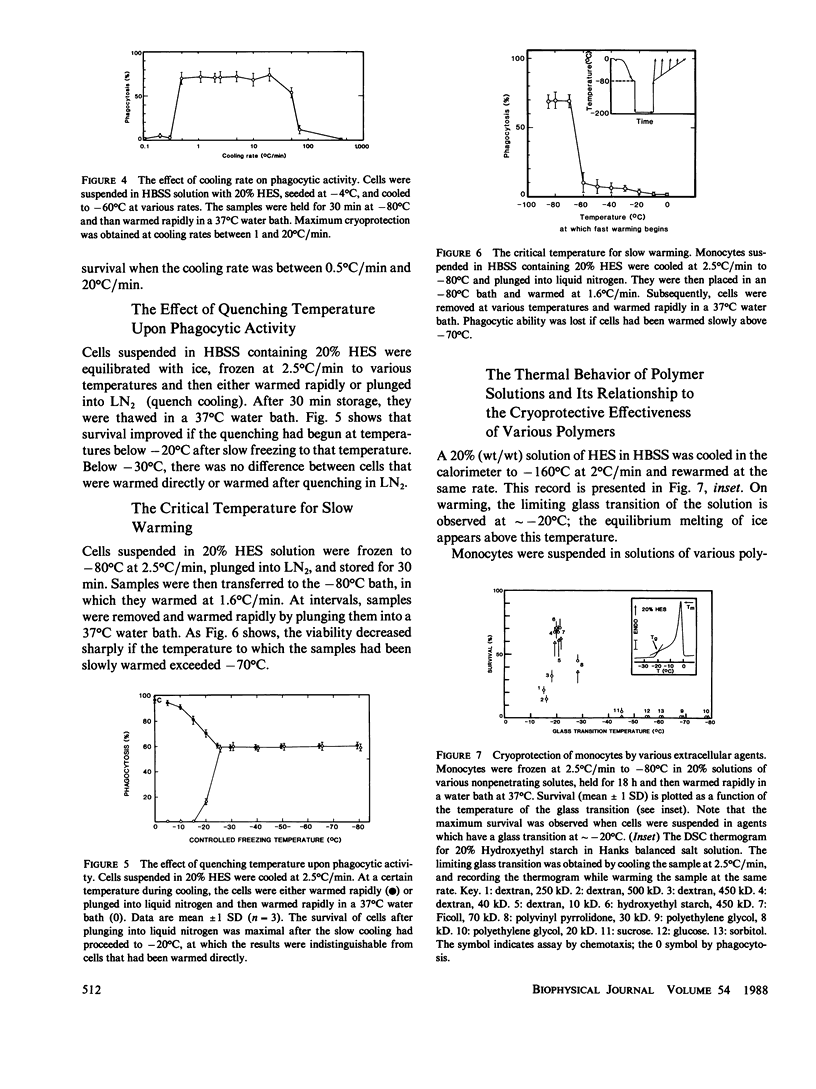
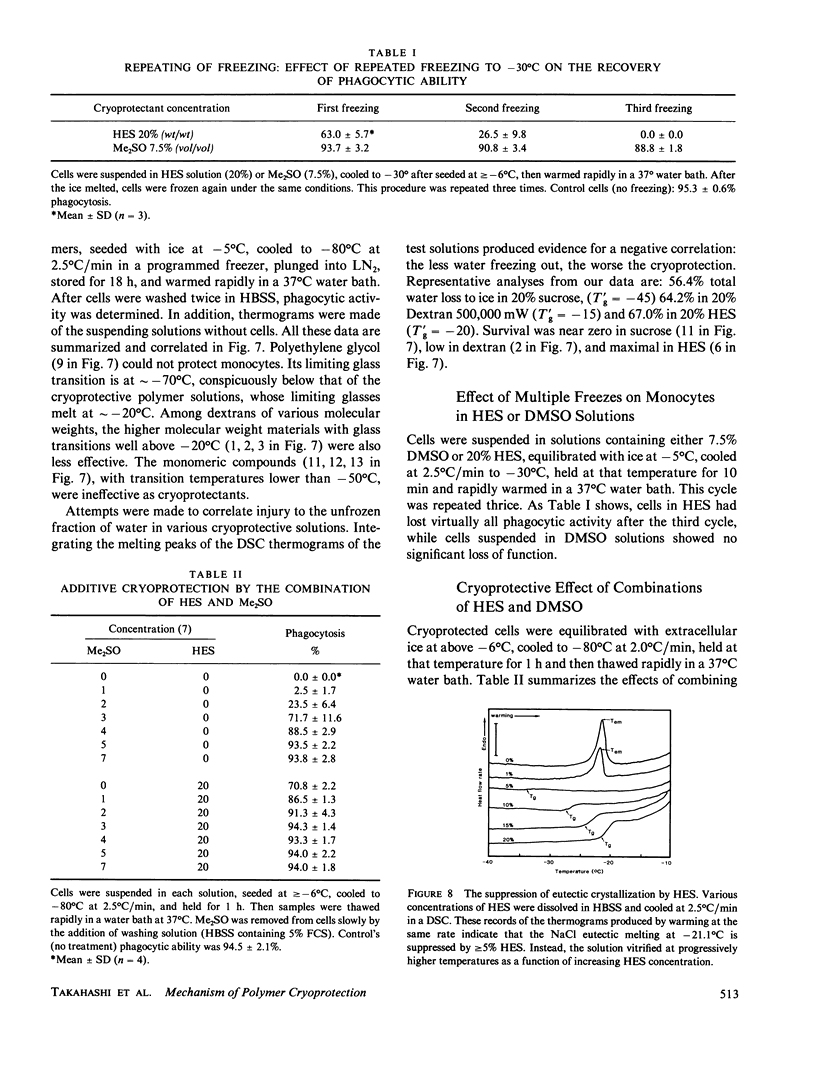
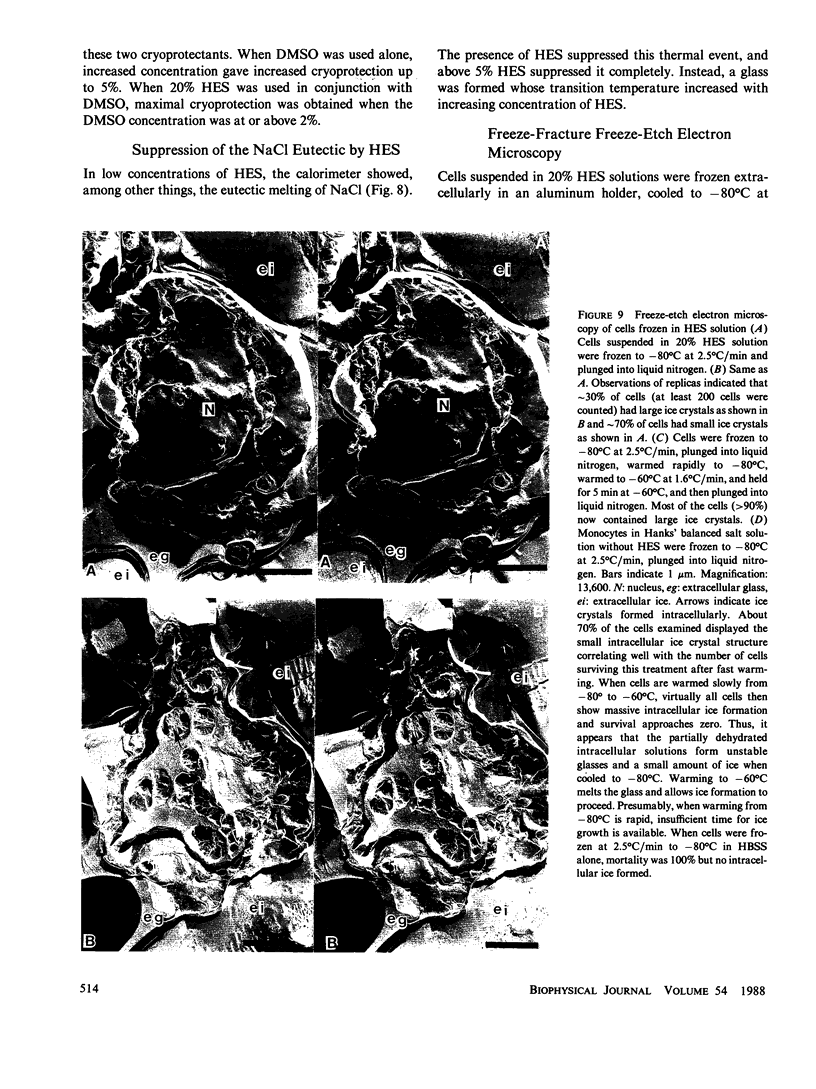
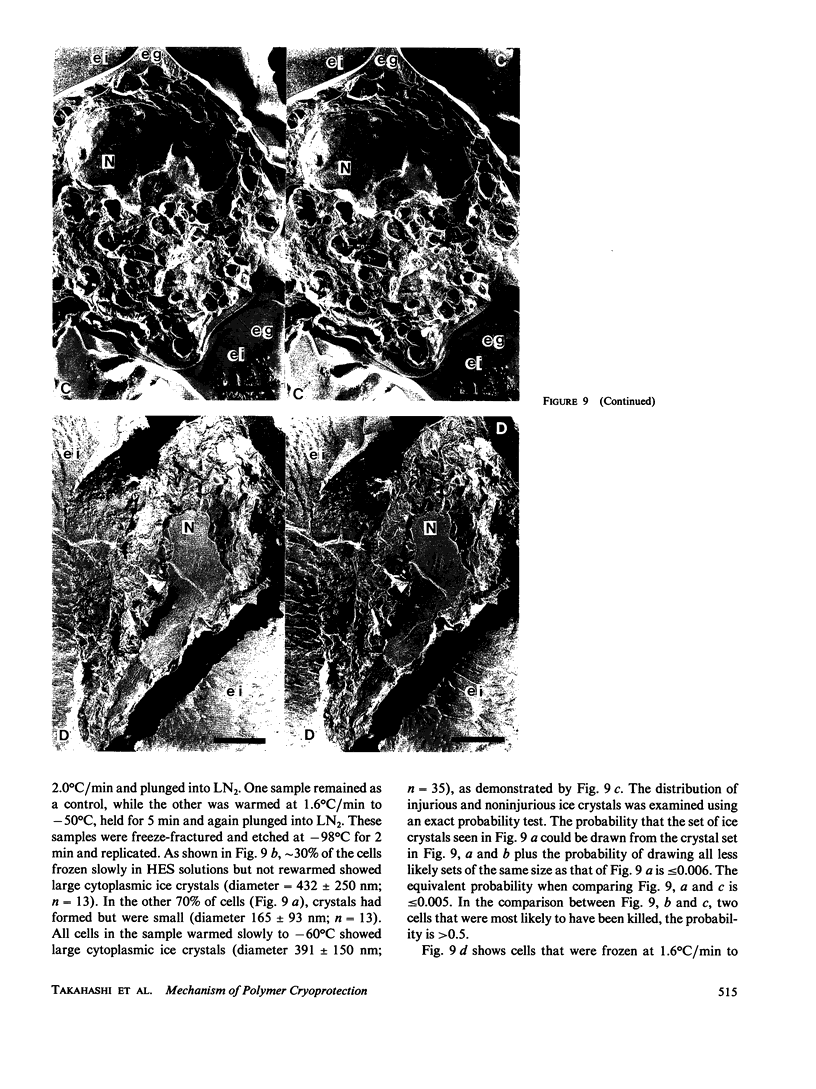
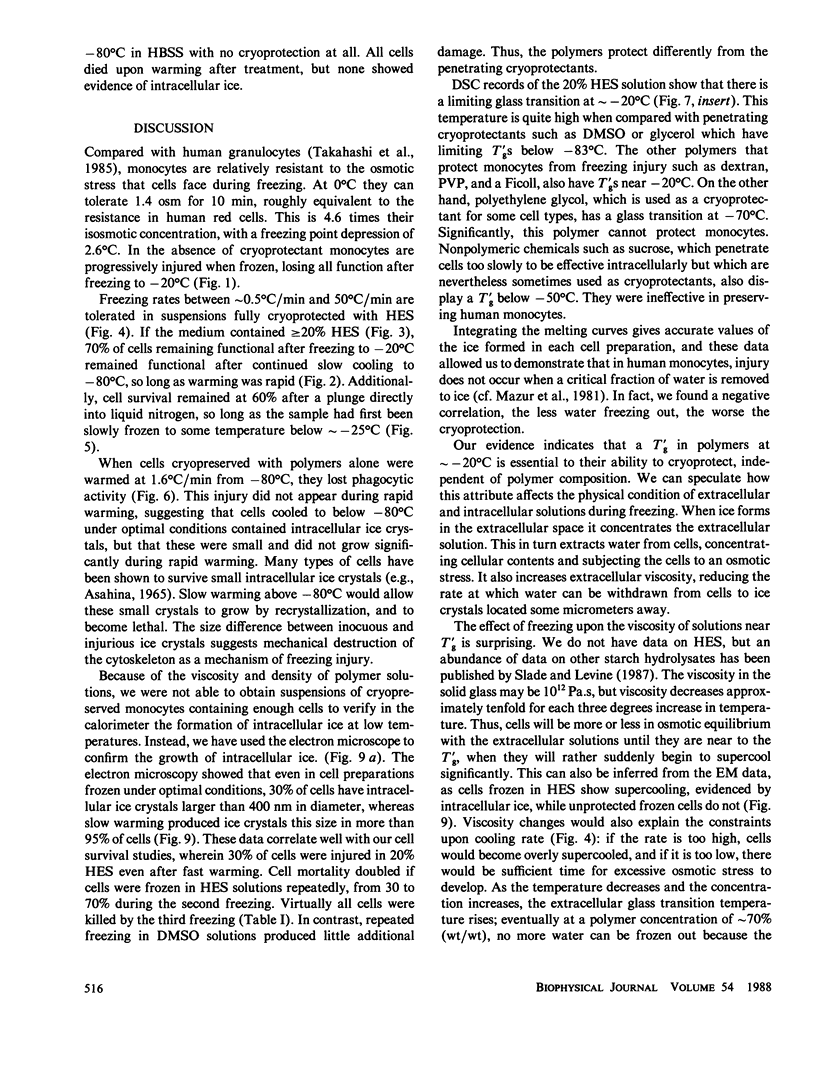
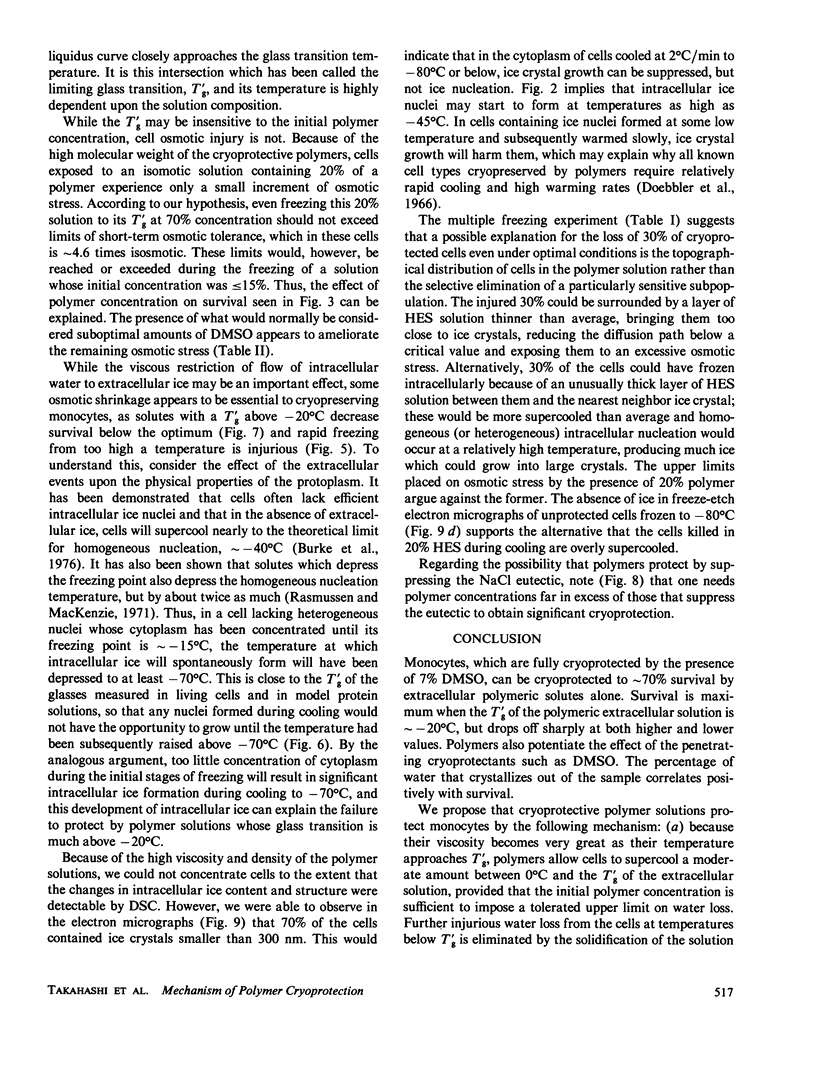
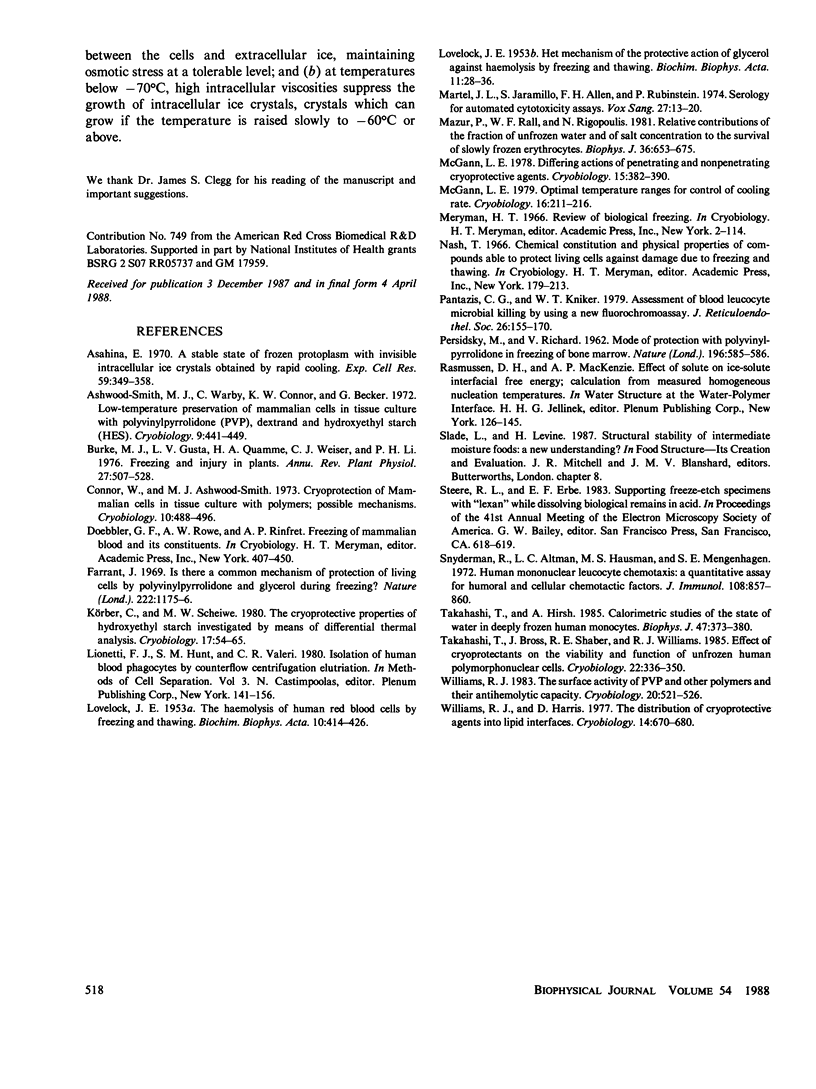
Images in this article
Selected References
These references are in PubMed. This may not be the complete list of references from this article.
- Asahina E., Shimada K., Hisada Y. A stable state of frozen protoplasm with invisible intracellular ice crystals obtained by rapid cooling. Exp Cell Res. 1970 Mar;59(3):349–358. doi: 10.1016/0014-4827(70)90641-5. [DOI] [PubMed] [Google Scholar]
- Ashwood-Smith M. J., Warby C., Connor K. W., Becker G. Low-temperature preservation of mammalian cells in tissue culture with polyvinylpyrrolidone (PVP), dextrans, and hydroxyethyl starch (HES). Cryobiology. 1972 Oct;9(5):441–449. doi: 10.1016/0011-2240(72)90161-7. [DOI] [PubMed] [Google Scholar]
- Connor W., Ashwood-Smith M. J. Cryoprotection of mammalian cells in tissue culture with polymers; possible mechanisms. Cryobiology. 1973 Dec;10(6):488–496. doi: 10.1016/s0011-2240(73)80002-1. [DOI] [PubMed] [Google Scholar]
- Farrant J. Is there a common mechanism of protection of living cells by polyvinylpyrrolidone and glycerol ding freezing? Nature. 1969 Jun 21;222(5199):1175–1176. doi: 10.1038/2221175a0. [DOI] [PubMed] [Google Scholar]
- Körber C., Scheiwe M. W. The cryoprotective properties of hydroxyethyl starch investigated by means of differential thermal analysis. Cryobiology. 1980 Feb;17(1):54–65. doi: 10.1016/0011-2240(80)90008-5. [DOI] [PubMed] [Google Scholar]
- LOVELOCK J. E. The haemolysis of human red blood-cells by freezing and thawing. Biochim Biophys Acta. 1953 Mar;10(3):414–426. doi: 10.1016/0006-3002(53)90273-x. [DOI] [PubMed] [Google Scholar]
- LOVELOCK J. E. The mechanism of the protective action of glycerol against haemolysis by freezing and thawing. Biochim Biophys Acta. 1953 May;11(1):28–36. doi: 10.1016/0006-3002(53)90005-5. [DOI] [PubMed] [Google Scholar]
- Martel J. L., Jaramillo S., Allen F. H., Jr, Rubinstein P. Serology for automated cytotoxicity assays. Contrast fluorescence test. Vox Sang. 1974;27(1):13–20. doi: 10.1111/j.1423-0410.1974.tb02384.x. [DOI] [PubMed] [Google Scholar]
- Mazur P., Rall W. F., Rigopoulos N. Relative contributions of the fraction of unfrozen water and of salt concentration to the survival of slowly frozen human erythrocytes. Biophys J. 1981 Dec;36(3):653–675. doi: 10.1016/S0006-3495(81)84757-1. [DOI] [PMC free article] [PubMed] [Google Scholar]
- McGann L. E. Differing actions of penetrating and nonpenetrating cryoprotective agents. Cryobiology. 1978 Aug;15(4):382–390. doi: 10.1016/0011-2240(78)90056-1. [DOI] [PubMed] [Google Scholar]
- McGann L. E. Optimal temperature ranges for control of cooling rate. Cryobiology. 1979 Jun;16(3):211–216. doi: 10.1016/0011-2240(79)90033-6. [DOI] [PubMed] [Google Scholar]
- Pantazis C. G., Kniker W. T. Assessment of blood leukocyte microbial killing by using a new fluorochrome microassay. J Reticuloendothel Soc. 1979 Aug;26(2):155–170. [PubMed] [Google Scholar]
- Snyderman R., Altman L. C., Hausman M. S., Mergenhagen S. E. Human mononuclear leukocyte chemotaxis: a quantitative assay for humoral and cellular chemotactic factors. J Immunol. 1972 Mar;108(3):857–860. [PubMed] [Google Scholar]
- Takahashi T., Bross J. B., Shaber R. E., Williams R. J. Effect of cryoprotectants on the viability and function of unfrozen human polymorphonuclear cells. Cryobiology. 1985 Aug;22(4):336–350. doi: 10.1016/0011-2240(85)90181-6. [DOI] [PubMed] [Google Scholar]
- Takahashi T., Hirsh A. Calorimetric studies of the state of water in deeply frozen human monocytes. Biophys J. 1985 Mar;47(3):373–380. doi: 10.1016/S0006-3495(85)83928-X. [DOI] [PMC free article] [PubMed] [Google Scholar]
- Williams R. J., Harris D. The distribution of cryoprotective agents into lipid interfaces. Cryobiology. 1977 Dec;14(6):670–680. doi: 10.1016/0011-2240(77)90022-0. [DOI] [PubMed] [Google Scholar]
- Williams R. J. The surface activity of PVP and other polymers and their antihemolytic capacity. Cryobiology. 1983 Oct;20(5):521–526. doi: 10.1016/0011-2240(83)90040-8. [DOI] [PubMed] [Google Scholar]