Abstract
We have obtained new insights into the behavior of a class of excitable systems when a stimulus, or parameter, is slowly tuned through a threshold value. Such systems do not accommodate no matter how slowly a stimulus ramp is applied, and the stimulus value at onset of repetitive activity shows a curious, nonmonotonic dependence on ramp speed. (Jakobsson, E. and R. Guttman. Biophys. J. 1980. 31:293-298.) demonstrated this for squid axon and for the Hodgkin-Huxley (HH) model. Furthermore, they showed theoretically that for moderately slow ramps the threshold increases as the ramp speed decreases, but for much slower ramp speeds threshold decreases as the ramp speed decreases. This latter feature was found surprising and it was suggested that the HH model, and squid axon in low calcium, exhibits reverse accommodation. We have found that reverse accommodation reflects the influence of persistent random fluctuations, and is a feature of all such excitable systems. We have derived an analytic condition which yields an approximation for threshold in the case of a slow ramp when the effect of fluctuations are negligible. This condition predicts, and numerical calculations confirm, that the onset of oscillations occurs beyond the critical stimulus value which is predicted by treating the stimulus intensity as a static parameter, i.e., the dynamic aspect of a ramp leads to a delay in the onset. The condition further demonstrates a memory effect, i.e., firing threshold is dependent on the initial state of the system. For very slow ramps then, fluctuations diminish both the delay and memory effects. We characterize the class of excitable systems for which these behaviors are expected, and we illustrate the phenomena for the HH model and for a model of cAMP-receptor dynamics in Dictyostelium discoideum.
Full text
PDF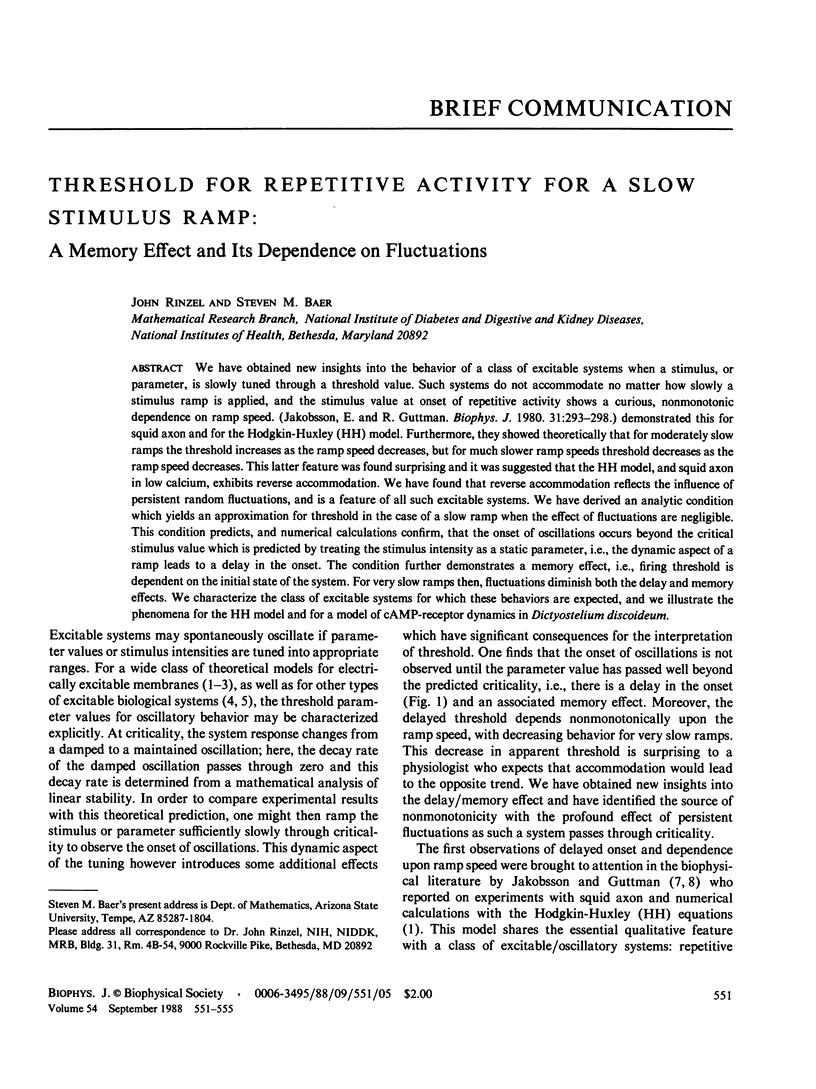
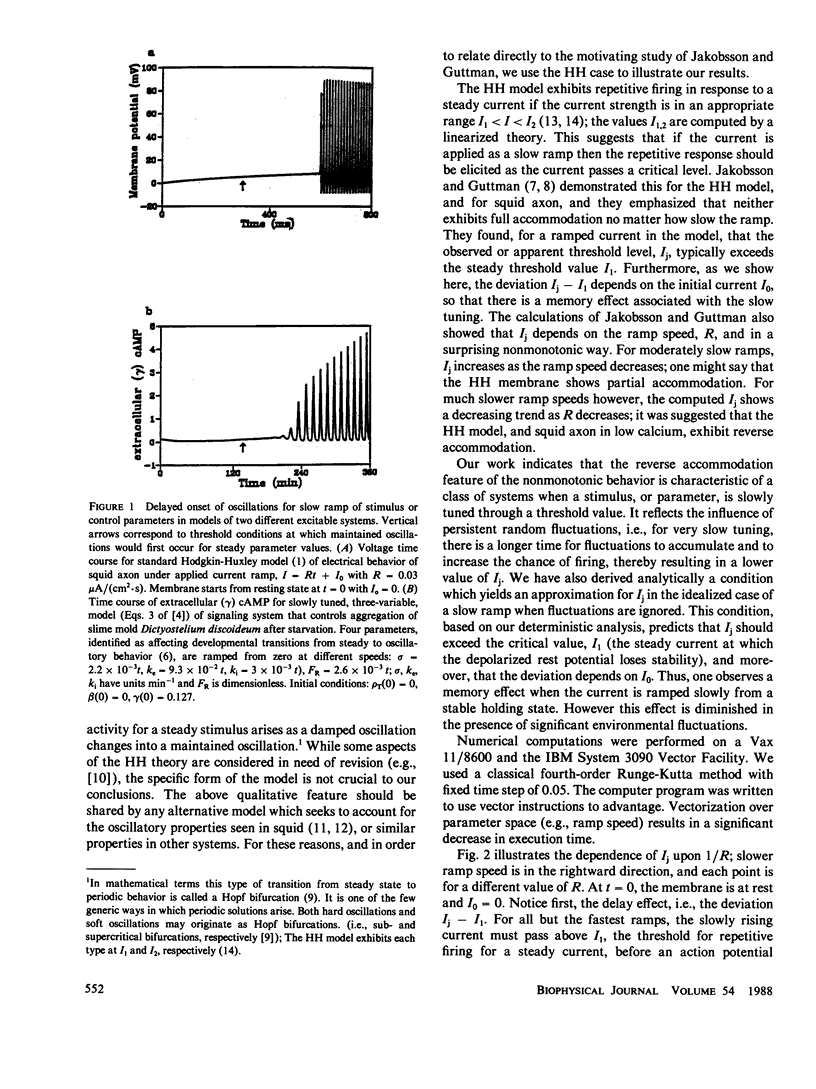
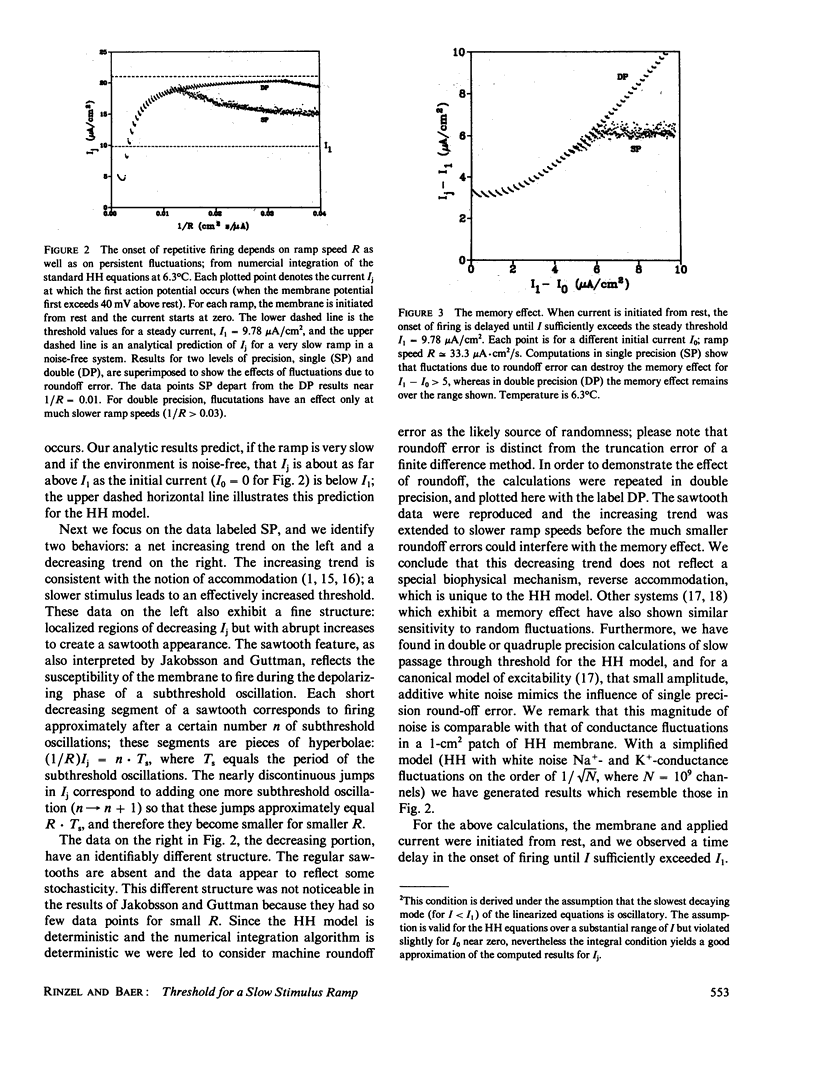
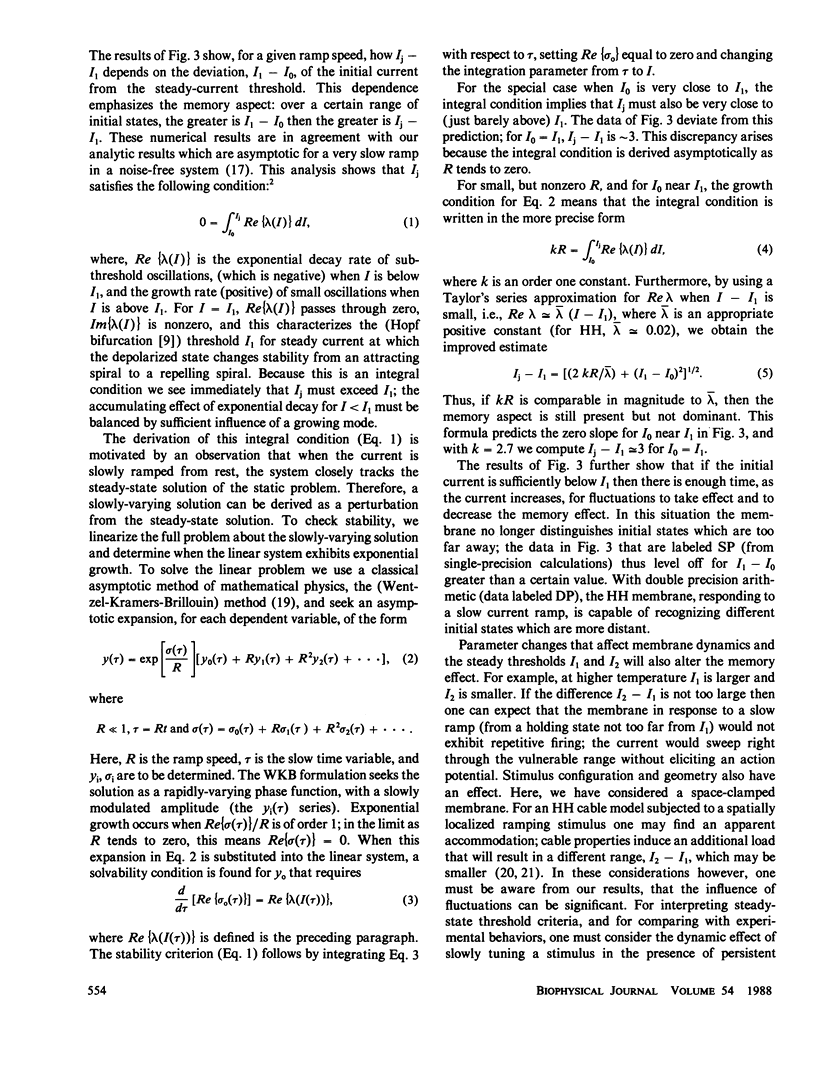
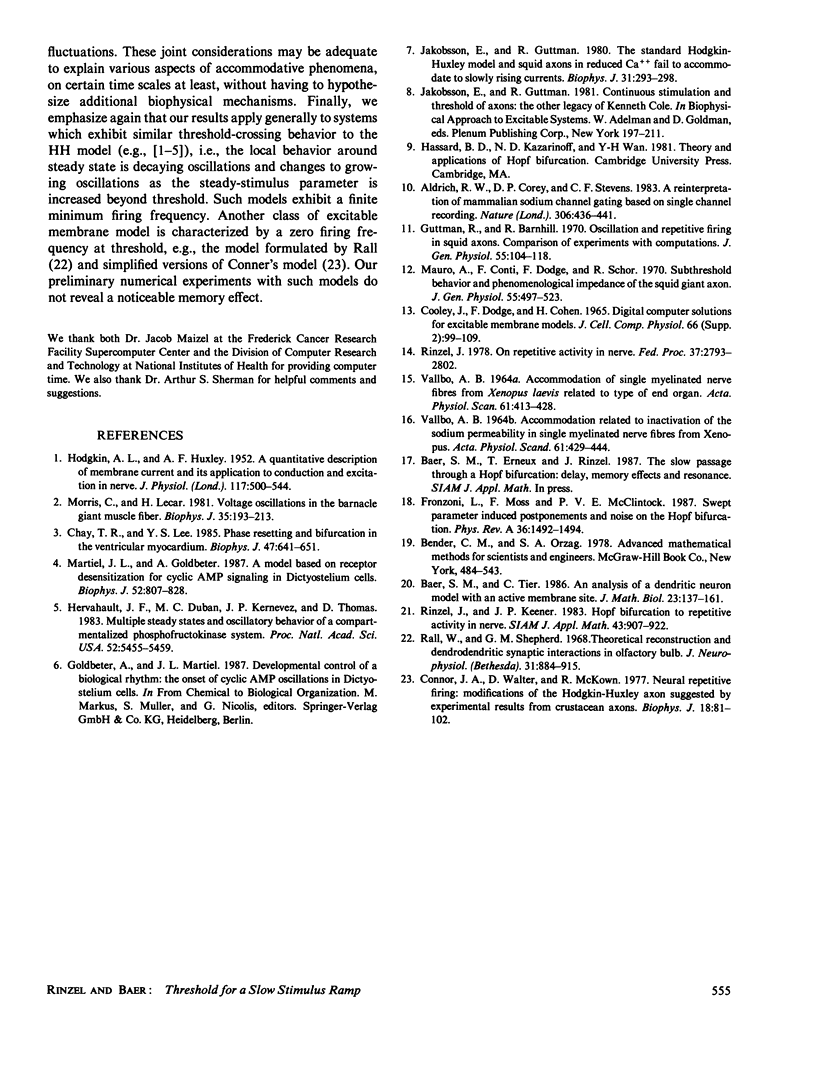
Selected References
These references are in PubMed. This may not be the complete list of references from this article.
- Aldrich R. W., Corey D. P., Stevens C. F. A reinterpretation of mammalian sodium channel gating based on single channel recording. Nature. 1983 Dec 1;306(5942):436–441. doi: 10.1038/306436a0. [DOI] [PubMed] [Google Scholar]
- Baer S. M., Tier C. An analysis of a dendritic neuron model with an active membrane site. J Math Biol. 1986;23(2):137–161. doi: 10.1007/BF00276954. [DOI] [PubMed] [Google Scholar]
- Chay T. R., Lee Y. S. Phase resetting and bifurcation in the ventricular myocardium. Biophys J. 1985 May;47(5):641–651. doi: 10.1016/S0006-3495(85)83960-6. [DOI] [PMC free article] [PubMed] [Google Scholar]
- Connor J. A., Walter D., McKown R. Neural repetitive firing: modifications of the Hodgkin-Huxley axon suggested by experimental results from crustacean axons. Biophys J. 1977 Apr;18(1):81–102. doi: 10.1016/S0006-3495(77)85598-7. [DOI] [PMC free article] [PubMed] [Google Scholar]
- Fronzoni L, Moss F, McClintock PV. Swept-parameter-induced postponements and noise on the Hopf bifurcation. Phys Rev A Gen Phys. 1987 Aug 1;36(3):1492–1494. doi: 10.1103/physreva.36.1492. [DOI] [PubMed] [Google Scholar]
- Guttman R., Barnhill R. Oscillation and repetitive firing in squid axons. Comparison of experiments with computations. J Gen Physiol. 1970 Jan;55(1):104–118. doi: 10.1085/jgp.55.1.104. [DOI] [PMC free article] [PubMed] [Google Scholar]
- HODGKIN A. L., HUXLEY A. F. A quantitative description of membrane current and its application to conduction and excitation in nerve. J Physiol. 1952 Aug;117(4):500–544. doi: 10.1113/jphysiol.1952.sp004764. [DOI] [PMC free article] [PubMed] [Google Scholar]
- Hervagault J. F., Duban M. C., Kernevez J. P., Thomas D. Multiple steady states and oscillatory behavior of a compartmentalized phosphofructokinase system. Proc Natl Acad Sci U S A. 1983 Sep;80(18):5455–5459. doi: 10.1073/pnas.80.18.5455. [DOI] [PMC free article] [PubMed] [Google Scholar]
- Jakobsson E., Guttman R. The standard Hodgkin-Huxley model and squid axons in reduced external Ca++ fail to accommodate to slowly rising currents. Biophys J. 1980 Aug;31(2):293–297. doi: 10.1016/S0006-3495(80)85059-4. [DOI] [PMC free article] [PubMed] [Google Scholar]
- Martiel J. L., Goldbeter A. A Model Based on Receptor Desensitization for Cyclic AMP Signaling in Dictyostelium Cells. Biophys J. 1987 Nov;52(5):807–828. doi: 10.1016/S0006-3495(87)83275-7. [DOI] [PMC free article] [PubMed] [Google Scholar]
- Mauro A., Conti F., Dodge F., Schor R. Subthreshold behavior and phenomenological impedance of the squid giant axon. J Gen Physiol. 1970 Apr;55(4):497–523. doi: 10.1085/jgp.55.4.497. [DOI] [PMC free article] [PubMed] [Google Scholar]
- Morris C., Lecar H. Voltage oscillations in the barnacle giant muscle fiber. Biophys J. 1981 Jul;35(1):193–213. doi: 10.1016/S0006-3495(81)84782-0. [DOI] [PMC free article] [PubMed] [Google Scholar]
- Rall W., Shepherd G. M. Theoretical reconstruction of field potentials and dendrodendritic synaptic interactions in olfactory bulb. J Neurophysiol. 1968 Nov;31(6):884–915. doi: 10.1152/jn.1968.31.6.884. [DOI] [PubMed] [Google Scholar]
- Rinzel J. On repetitive activity in nerve. Fed Proc. 1978 Dec;37(14):2793–2802. [PubMed] [Google Scholar]
- VALLBO A. B. ACCOMMODATION OF SINGLE MYELINATED NERVE FIBRES FROM XENOPUS LAEVIS RELATED TO TYPE OF END ORGAN. Acta Physiol Scand. 1964 Aug;61:413–428. [PubMed] [Google Scholar]
- VALLBO A. B. ACCOMMODATION RELATED TO INACTIVATION OF THE SODIUM PERMEABILITY IN SINGLE MYELINATED NERVE FIBRES FROM XENOPUS LAEVIS. Acta Physiol Scand. 1964 Aug;61:429–444. [PubMed] [Google Scholar]