Abstract
Single Na+ channels from rat skeletal muscle were inserted into planar lipid bilayers in the presence of either 200 nM batrachotoxin (BTX) or 50 microM veratridine (VT). These toxins, in addition to their ability to shift inactivation of voltage-gated Na+ channels, may be used as probes of ion conduction in these channels. Channels modified by either of the toxins have qualitatively similar selectivity for the alkali cations (Na+ approximately Li+ greater than K+ greater than Rb+ greater than Cs+). Biionic reversal potentials, for example, were concentration independent for all ions studied. Na+/K+ and Na+/Rb+ reversal potentials, however, were dependent on the orientation of the ionic species with respect to the intra- or extracellular face of the channel, whereas Na+/Li+ biionic reversal potentials were not orientation dependent. A simple, four-barrier, three-well, single-ion occupancy model was used to generate current-voltage relationships similar to those observed in symmetrical solutions of Na, K, or Li ions. The barrier profiles for Na and Li ions were symmetric, whereas that for K ions was asymmetric. This suggests the barrier to ion permeation for K ions may be different than that for Na and Li ions. With this model, these hypothetical energy barrier profiles could predict the orientation-dependent reversal potentials observed for Na+/K+ and Na+/Rb+. The energy barrier profiles, however, were not capable of describing biionic Na/Li ion permeation. Together these results support the hypothesis that Na ions have a different rate determining step for ion permeation than that of K and Rb ions.
Full text
PDF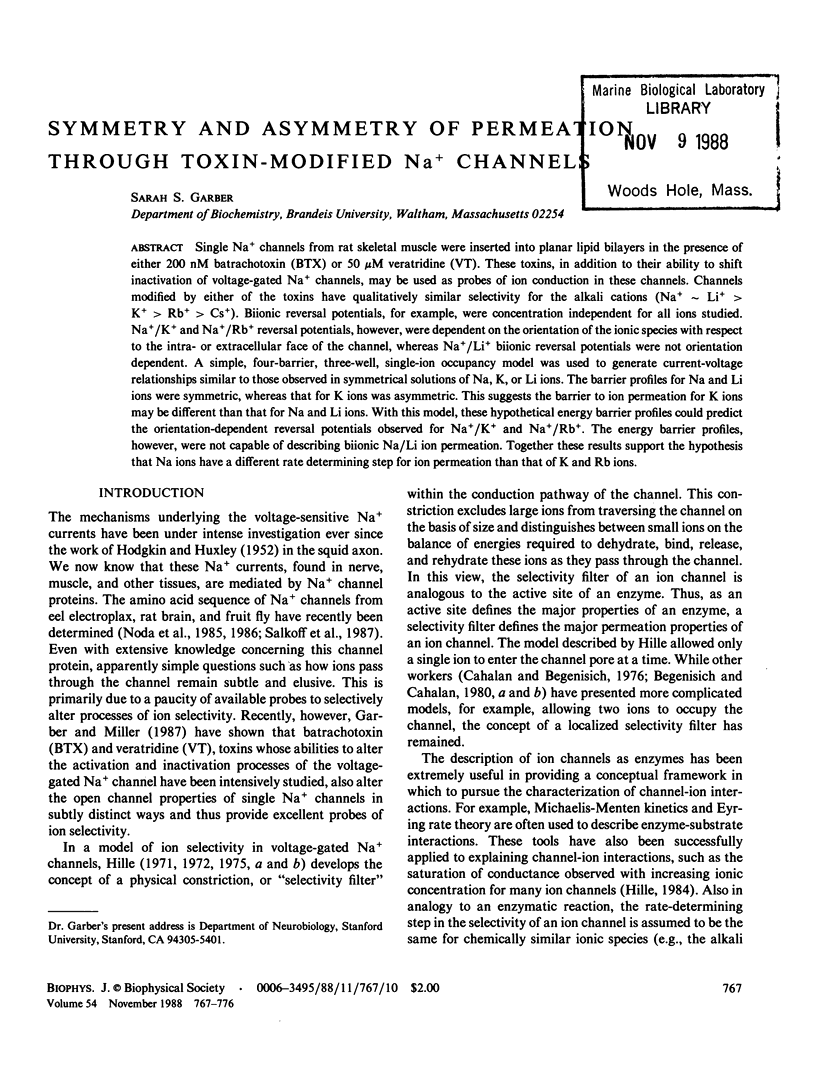
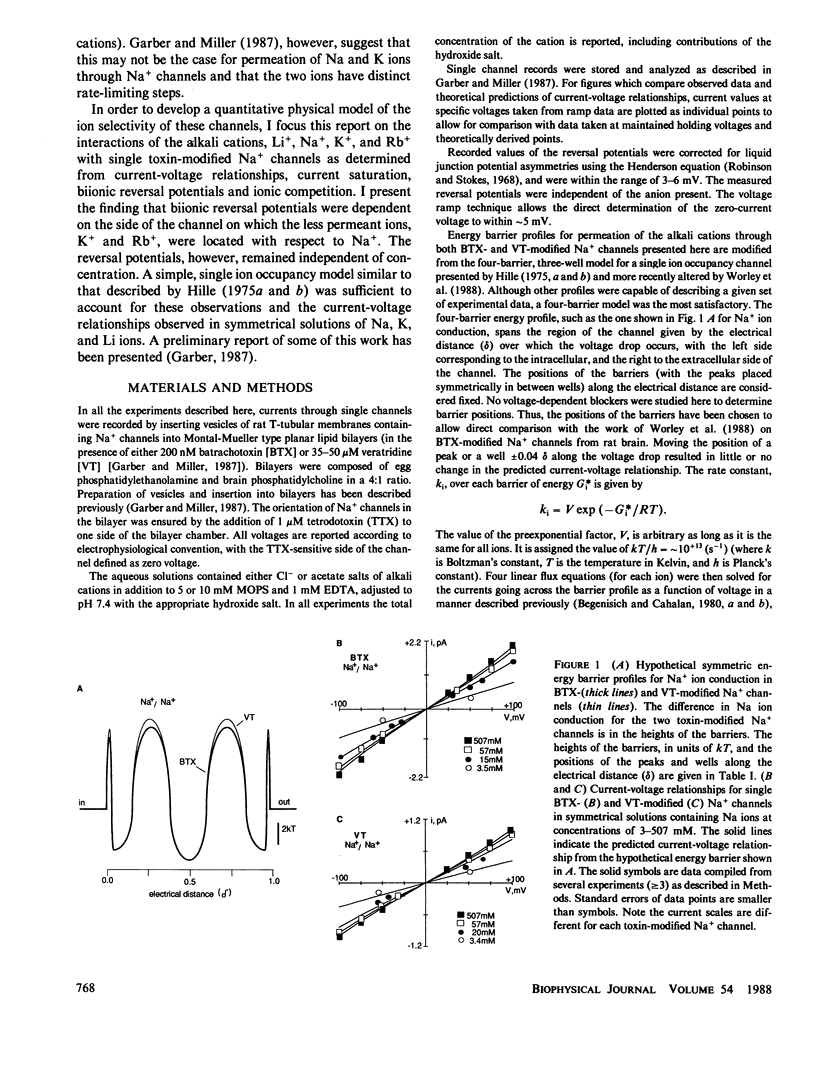
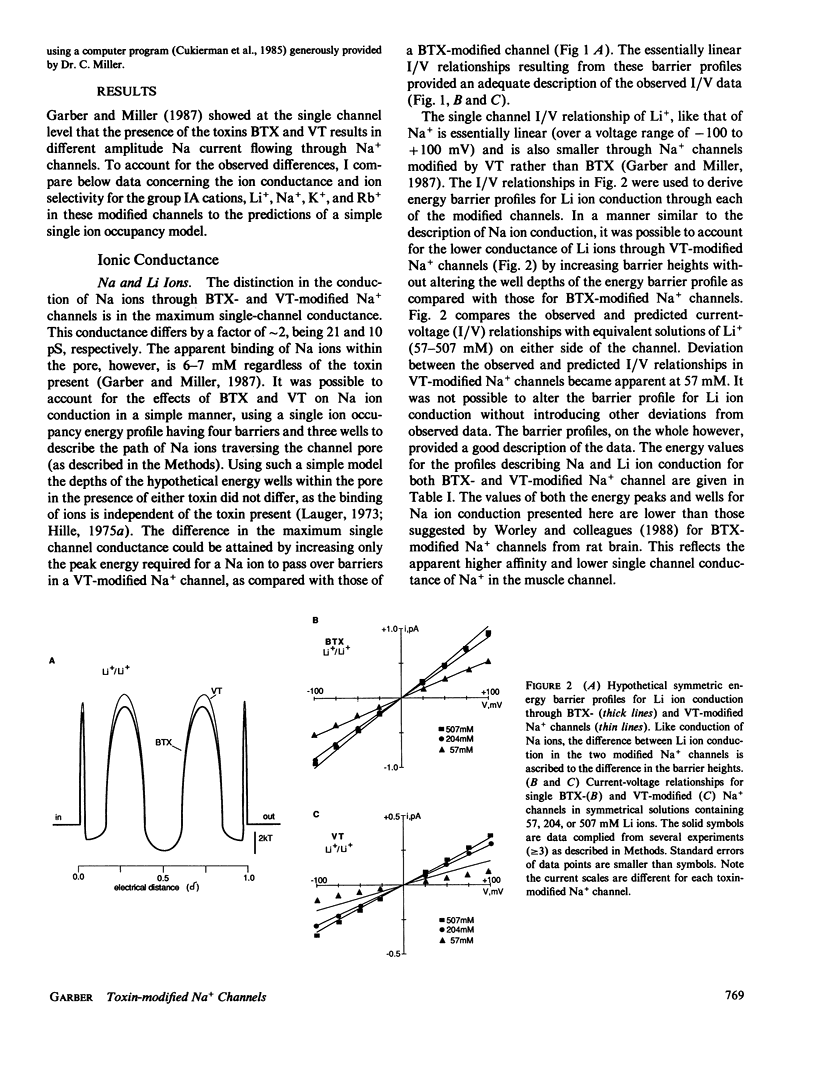
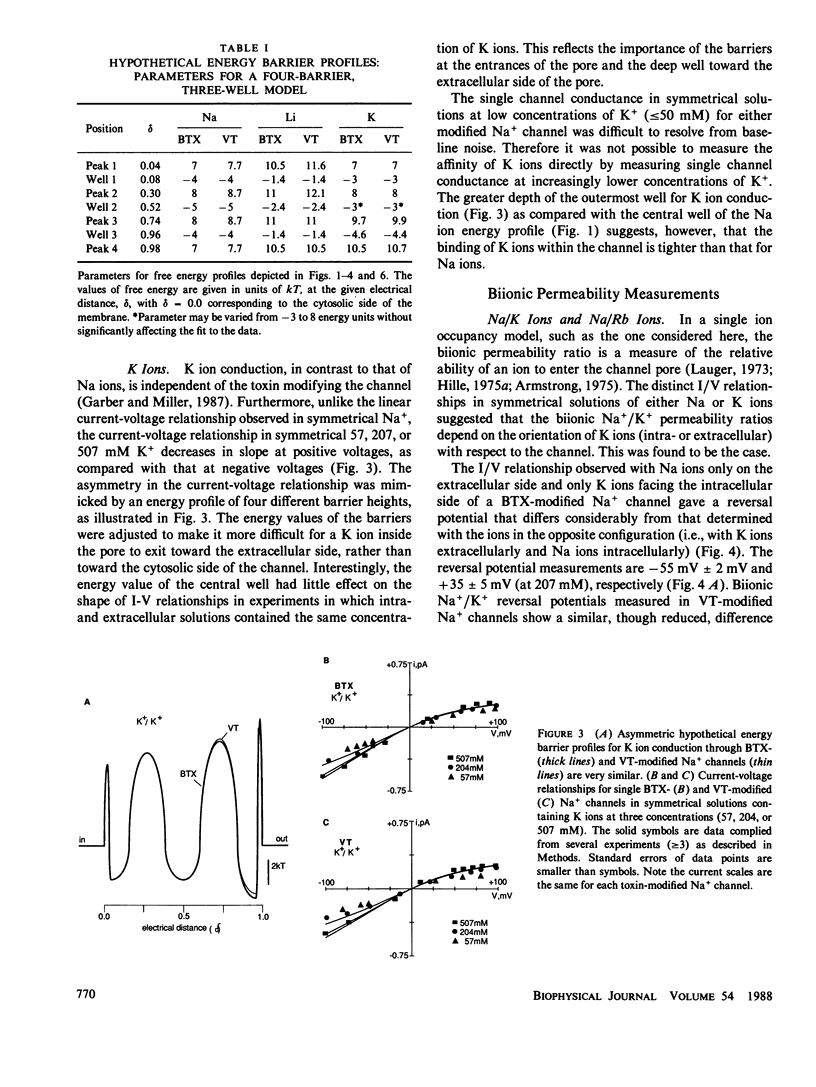
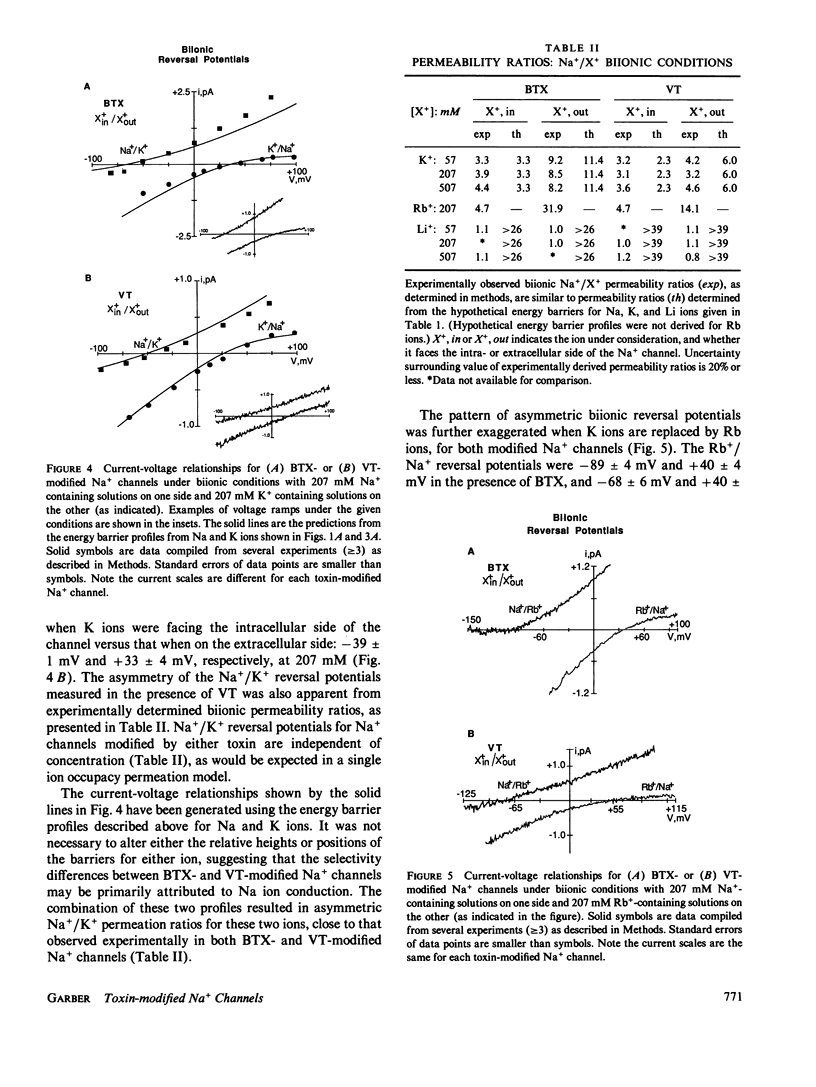
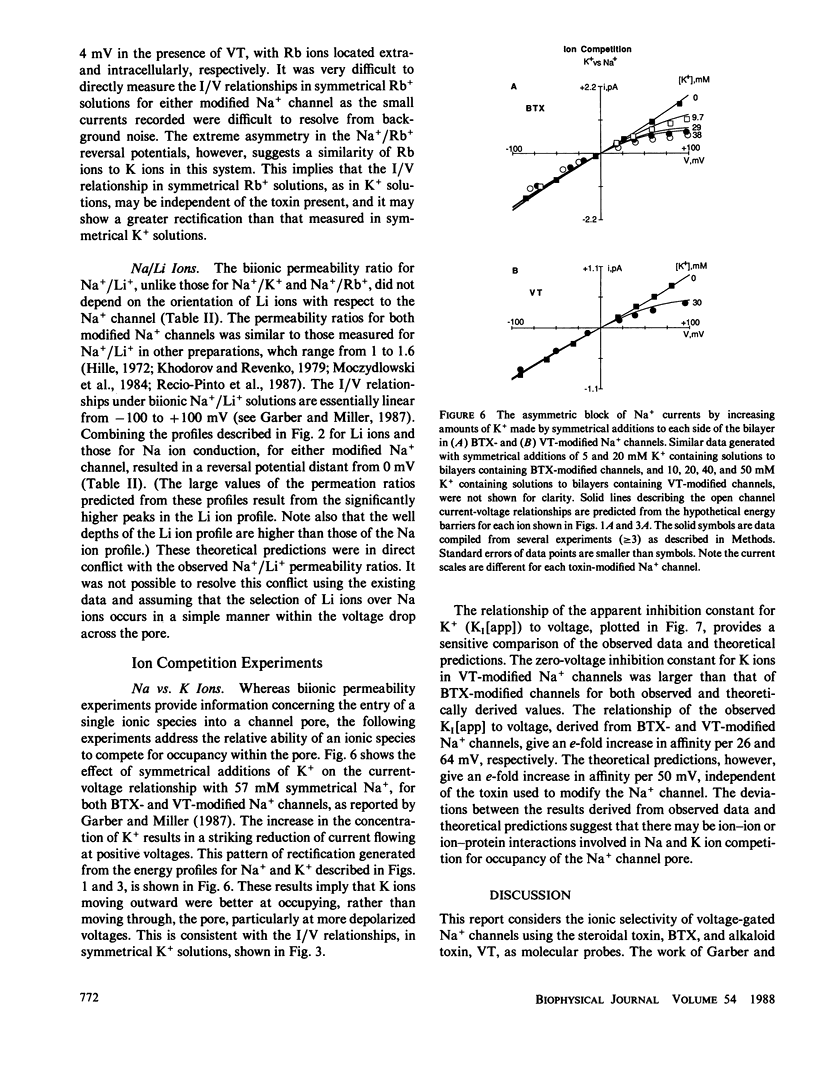
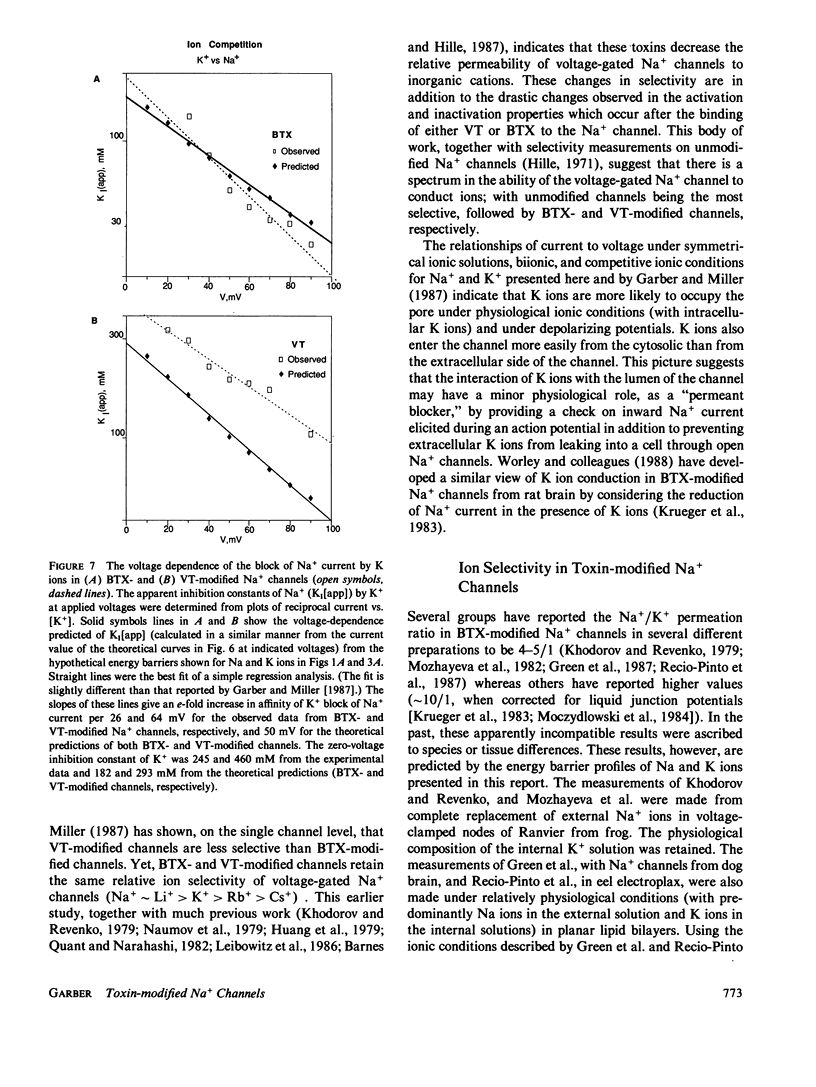
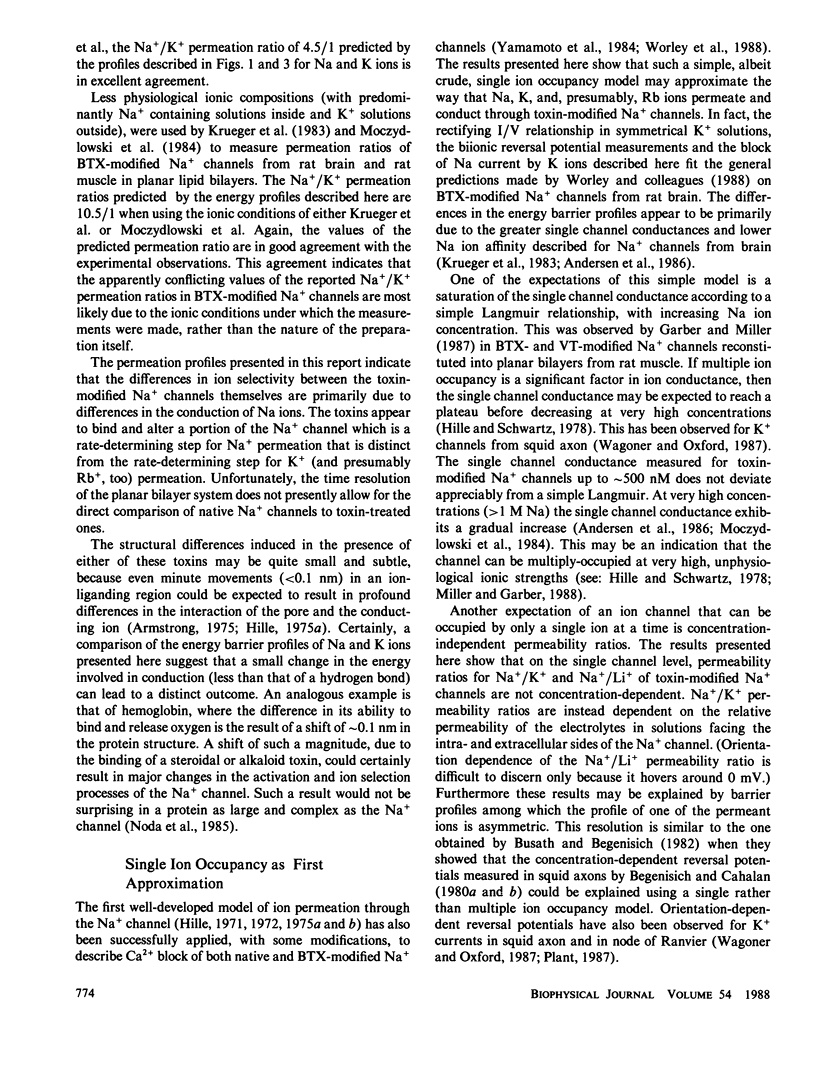
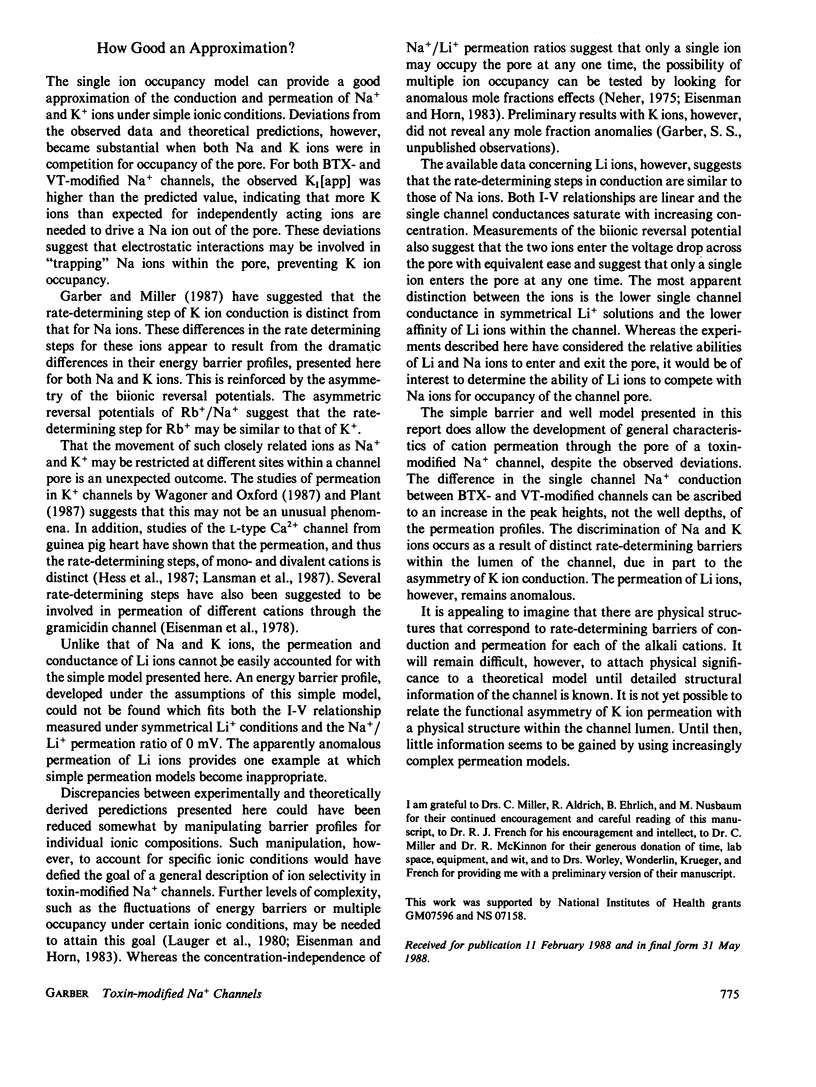
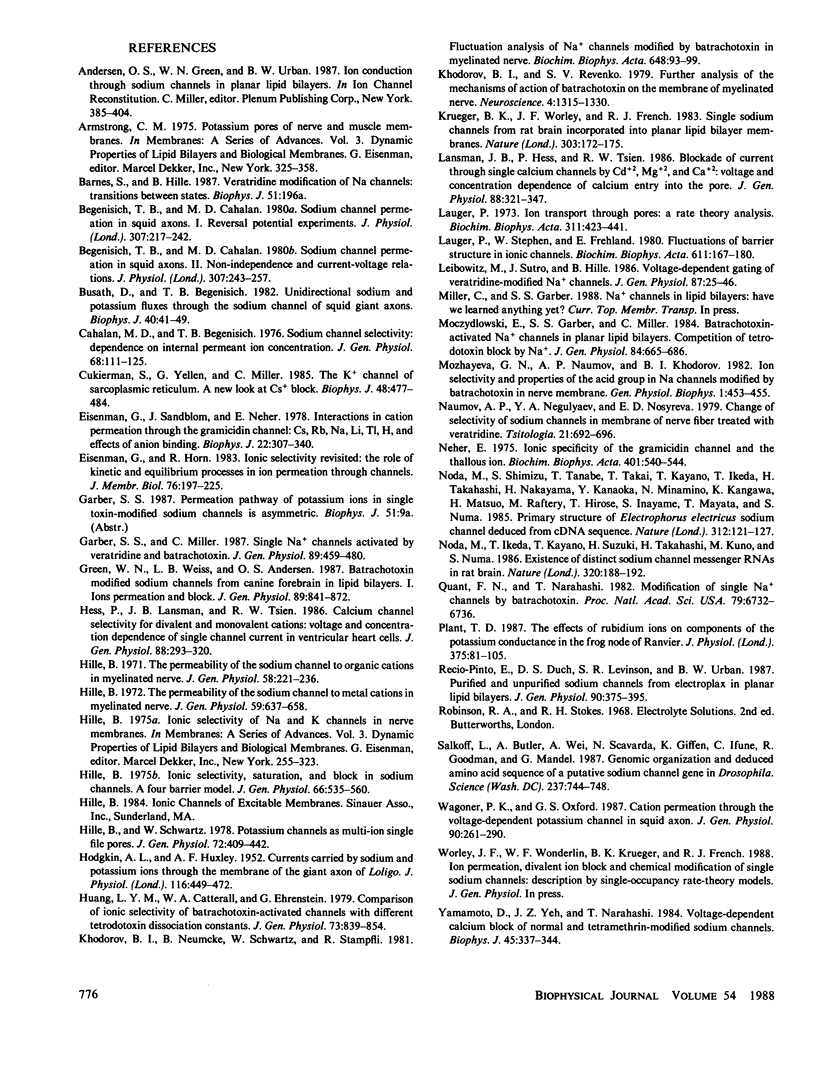
Selected References
These references are in PubMed. This may not be the complete list of references from this article.
- Begenisich T. B., Cahalan M. D. Sodium channel permeation in squid axons. I: Reversal potential experiments. J Physiol. 1980 Oct;307:217–242. doi: 10.1113/jphysiol.1980.sp013432. [DOI] [PMC free article] [PubMed] [Google Scholar]
- Begenisich T. B., Cahalan M. D. Sodium channel permeation in squid axons. II: Non-independence and current-voltage relations. J Physiol. 1980 Oct;307:243–257. doi: 10.1113/jphysiol.1980.sp013433. [DOI] [PMC free article] [PubMed] [Google Scholar]
- Busath D., Begenisich T. Unidirectional sodium and potassium fluxes through the sodium channel of squid giant axons. Biophys J. 1982 Oct;40(1):41–49. doi: 10.1016/S0006-3495(82)84456-1. [DOI] [PMC free article] [PubMed] [Google Scholar]
- Cahalan M., Begenisich T. Sodium channel selectivity. Dependence on internal permeant ion concentration. J Gen Physiol. 1976 Aug;68(2):111–125. doi: 10.1085/jgp.68.2.111. [DOI] [PMC free article] [PubMed] [Google Scholar]
- Cukierman S., Yellen G., Miller C. The K+ channel of sarcoplasmic reticulum. A new look at Cs+ block. Biophys J. 1985 Sep;48(3):477–484. doi: 10.1016/S0006-3495(85)83803-0. [DOI] [PMC free article] [PubMed] [Google Scholar]
- Eisenman G., Horn R. Ionic selectivity revisited: the role of kinetic and equilibrium processes in ion permeation through channels. J Membr Biol. 1983;76(3):197–225. doi: 10.1007/BF01870364. [DOI] [PubMed] [Google Scholar]
- Eisenman G., Sandblom J., Neher E. Interactions in cation permeation through the gramicidin channel. Cs, Rb, K, Na, Li, Tl, H, and effects of anion binding. Biophys J. 1978 May;22(2):307–340. doi: 10.1016/S0006-3495(78)85491-5. [DOI] [PMC free article] [PubMed] [Google Scholar]
- Garber S. S., Miller C. Single Na+ channels activated by veratridine and batrachotoxin. J Gen Physiol. 1987 Mar;89(3):459–480. doi: 10.1085/jgp.89.3.459. [DOI] [PMC free article] [PubMed] [Google Scholar]
- Green W. N., Weiss L. B., Andersen O. S. Batrachotoxin-modified sodium channels in planar lipid bilayers. Ion permeation and block. J Gen Physiol. 1987 Jun;89(6):841–872. doi: 10.1085/jgp.89.6.841. [DOI] [PMC free article] [PubMed] [Google Scholar]
- HODGKIN A. L., HUXLEY A. F. Currents carried by sodium and potassium ions through the membrane of the giant axon of Loligo. J Physiol. 1952 Apr;116(4):449–472. doi: 10.1113/jphysiol.1952.sp004717. [DOI] [PMC free article] [PubMed] [Google Scholar]
- Hess P., Lansman J. B., Tsien R. W. Calcium channel selectivity for divalent and monovalent cations. Voltage and concentration dependence of single channel current in ventricular heart cells. J Gen Physiol. 1986 Sep;88(3):293–319. doi: 10.1085/jgp.88.3.293. [DOI] [PMC free article] [PubMed] [Google Scholar]
- Hille B. Ionic selectivity of Na and K channels of nerve membranes. Membranes. 1975;3:255–323. [PubMed] [Google Scholar]
- Hille B. Ionic selectivity, saturation, and block in sodium channels. A four-barrier model. J Gen Physiol. 1975 Nov;66(5):535–560. doi: 10.1085/jgp.66.5.535. [DOI] [PMC free article] [PubMed] [Google Scholar]
- Hille B., Schwarz W. Potassium channels as multi-ion single-file pores. J Gen Physiol. 1978 Oct;72(4):409–442. doi: 10.1085/jgp.72.4.409. [DOI] [PMC free article] [PubMed] [Google Scholar]
- Hille B. The permeability of the sodium channel to metal cations in myelinated nerve. J Gen Physiol. 1972 Jun;59(6):637–658. doi: 10.1085/jgp.59.6.637. [DOI] [PMC free article] [PubMed] [Google Scholar]
- Huang L. Y., Catterall W. A., Ehrenstein G. Comparison of ionic selectivity of batrachotoxin-activated channels with different tetrodotoxin dissociation constants. J Gen Physiol. 1979 Jun;73(6):839–854. doi: 10.1085/jgp.73.6.839. [DOI] [PMC free article] [PubMed] [Google Scholar]
- Khodorov B. I., Neumcke B., Schwarz W., Stämpfli R. Fluctuation analysis of Na+ channels modified by batrachotoxin in myelinated nerve. Biochim Biophys Acta. 1981 Oct 20;648(1):93–99. doi: 10.1016/0005-2736(81)90128-0. [DOI] [PubMed] [Google Scholar]
- Khodorov B. I., Revenko S. V. Further analysis of the mechanisms of action of batrachotoxin on the membrane of myelinated nerve. Neuroscience. 1979;4(9):1315–1330. doi: 10.1016/0306-4522(79)90159-3. [DOI] [PubMed] [Google Scholar]
- Krueger B. K., Worley J. F., 3rd, French R. J. Single sodium channels from rat brain incorporated into planar lipid bilayer membranes. Nature. 1983 May 12;303(5913):172–175. doi: 10.1038/303172a0. [DOI] [PubMed] [Google Scholar]
- Lansman J. B., Hess P., Tsien R. W. Blockade of current through single calcium channels by Cd2+, Mg2+, and Ca2+. Voltage and concentration dependence of calcium entry into the pore. J Gen Physiol. 1986 Sep;88(3):321–347. doi: 10.1085/jgp.88.3.321. [DOI] [PMC free article] [PubMed] [Google Scholar]
- Leibowitz M. D., Sutro J. B., Hille B. Voltage-dependent gating of veratridine-modified Na channels. J Gen Physiol. 1986 Jan;87(1):25–46. doi: 10.1085/jgp.87.1.25. [DOI] [PMC free article] [PubMed] [Google Scholar]
- Läuger P. Ion transport through pores: a rate-theory analysis. Biochim Biophys Acta. 1973 Jul 6;311(3):423–441. doi: 10.1016/0005-2736(73)90323-4. [DOI] [PubMed] [Google Scholar]
- Läuger P., Stephan W., Frehland E. Fluctuations of barrier structure in ionic channels. Biochim Biophys Acta. 1980 Oct 16;602(1):167–180. doi: 10.1016/0005-2736(80)90299-0. [DOI] [PubMed] [Google Scholar]
- Moczydlowski E., Garber S. S., Miller C. Batrachotoxin-activated Na+ channels in planar lipid bilayers. Competition of tetrodotoxin block by Na+. J Gen Physiol. 1984 Nov;84(5):665–686. doi: 10.1085/jgp.84.5.665. [DOI] [PMC free article] [PubMed] [Google Scholar]
- Naumov A. P., Neguliaev Iu A., Nosyreva E. D. Izmenenie selektivnosti natrievykh kanalov membrany nervnogo volokna pri deistvii veratrina. Tsitologiia. 1979 Jun;21(6):692–696. [PubMed] [Google Scholar]
- Noda M., Ikeda T., Kayano T., Suzuki H., Takeshima H., Kurasaki M., Takahashi H., Numa S. Existence of distinct sodium channel messenger RNAs in rat brain. Nature. 1986 Mar 13;320(6058):188–192. doi: 10.1038/320188a0. [DOI] [PubMed] [Google Scholar]
- Noda M., Shimizu S., Tanabe T., Takai T., Kayano T., Ikeda T., Takahashi H., Nakayama H., Kanaoka Y., Minamino N. Primary structure of Electrophorus electricus sodium channel deduced from cDNA sequence. Nature. 1984 Nov 8;312(5990):121–127. doi: 10.1038/312121a0. [DOI] [PubMed] [Google Scholar]
- Plant T. D. The effects of rubidium ions on components of the potassium conductance in the frog node of Ranvier. J Physiol. 1986 Jun;375:81–105. doi: 10.1113/jphysiol.1986.sp016107. [DOI] [PMC free article] [PubMed] [Google Scholar]
- Quandt F. N., Narahashi T. Modification of single Na+ channels by batrachotoxin. Proc Natl Acad Sci U S A. 1982 Nov;79(21):6732–6736. doi: 10.1073/pnas.79.21.6732. [DOI] [PMC free article] [PubMed] [Google Scholar]
- Recio-Pinto E., Duch D. S., Levinson S. R., Urban B. W. Purified and unpurified sodium channels from eel electroplax in planar lipid bilayers. J Gen Physiol. 1987 Sep;90(3):375–395. doi: 10.1085/jgp.90.3.375. [DOI] [PMC free article] [PubMed] [Google Scholar]
- Salkoff L., Butler A., Wei A., Scavarda N., Giffen K., Ifune C., Goodman R., Mandel G. Genomic organization and deduced amino acid sequence of a putative sodium channel gene in Drosophila. Science. 1987 Aug 14;237(4816):744–749. doi: 10.1126/science.2441469. [DOI] [PubMed] [Google Scholar]
- Wagoner P. K., Oxford G. S. Cation permeation through the voltage-dependent potassium channel in the squid axon. Characteristics and mechanisms. J Gen Physiol. 1987 Aug;90(2):261–290. doi: 10.1085/jgp.90.2.261. [DOI] [PMC free article] [PubMed] [Google Scholar]
- Yamamoto D., Yeh J. Z., Narahashi T. Voltage-dependent calcium block of normal and tetramethrin-modified single sodium channels. Biophys J. 1984 Jan;45(1):337–344. doi: 10.1016/S0006-3495(84)84159-4. [DOI] [PMC free article] [PubMed] [Google Scholar]