Abstract
We have monitored the growth of domains of sickle hemoglobin polymers by using temporally and spatially resolved light scattering and birefringence measured pseudosimultaneously on a 50-microns square area. Polymerization was induced and indefinitely maintained by photolysis of the carbonmonoxy derivative using an argon ion laser. Intensity of scattering and birefringence (measured as intensity transmitted through crossed polarizers) were measured using a silicon-intensified target vidicon interfaced to a computer. Polymer concentration, as inferred by light scattering, grew with primarily circular symmetry, with approximately 20% of the signal initially in a twofold symmetric pattern. In time the circular symmetry increased. A distinct decrease in the scattering signal developed which spread outward from the center of the domain. Birefringence lagged the scattering and initially grew in a twofold pattern, with the formation of a characteristic Maltese cross only appearing much later, and well after the scattering signal had peaked. Radial profiles of the domain scattering and birefringence were both approximately gaussian. We successfully modeled the decrease in scattering by fitting the profiles to a large gaussian from which a second smaller gaussian was subtracted. This second gaussian had the width of the birefringence gaussian. The width of the birefringence gaussian grew linearly in time, while the width of the scattering gaussian showed a notable acceleration. We conclude that domains form primarily as disordered arrays which align at later times. We explain the above observations, including the shape of the birefringence progress curves, as the result of an alignment transition which is solely due to a redistribution of monomers from short to long, and from entangled to radial, polymers. We present a theoretical justification for this process in an appendix. In a separate paper (Zhou, H. X., and F. A. Ferrone, manuscript submitted for publication) we show that the gaussian shapes and acceleration of the width naturally arise from a generalization of the double nucleation mechanism for sickle hemoglobin gelation (Ferrone, F. A., J. Hofrichter, H. Sunshine, and W. A. Eaton 1980. Biophys. J. 32:361-377; Ferrone, F. A., J. Hofrichter, and W. A. Eaton. 1985. J. Mol. Biol. 183:611-631).
Full text
PDF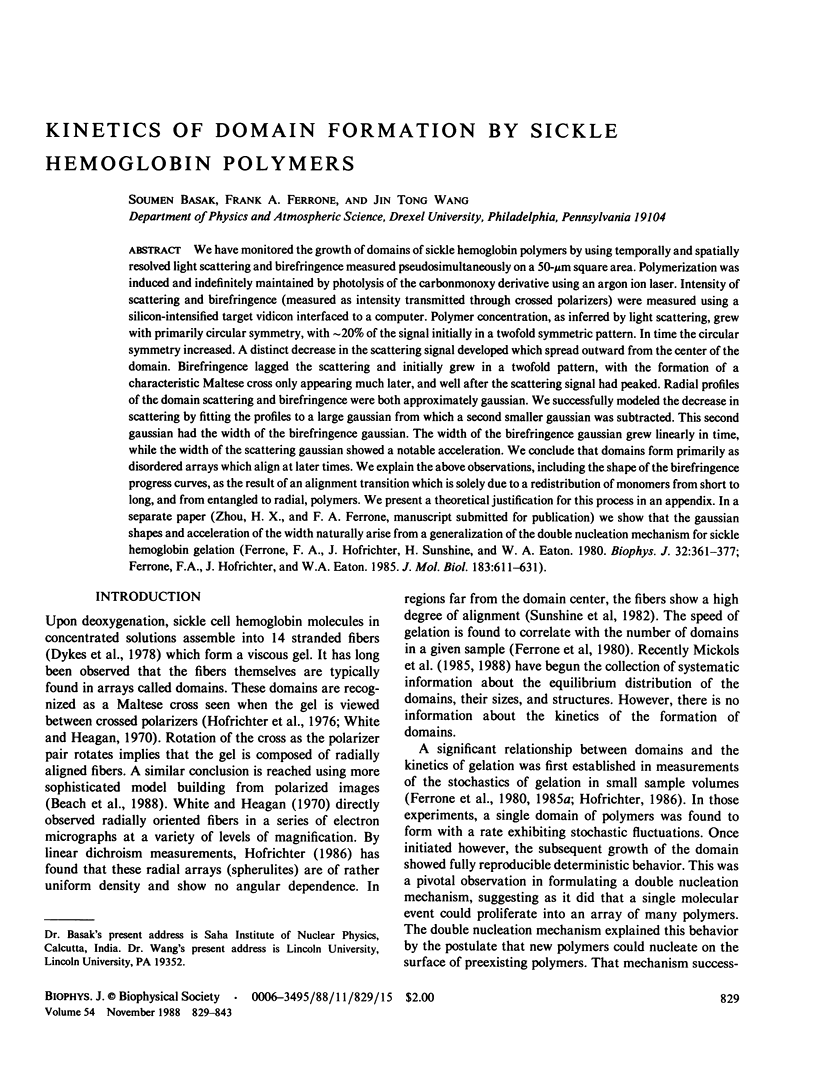
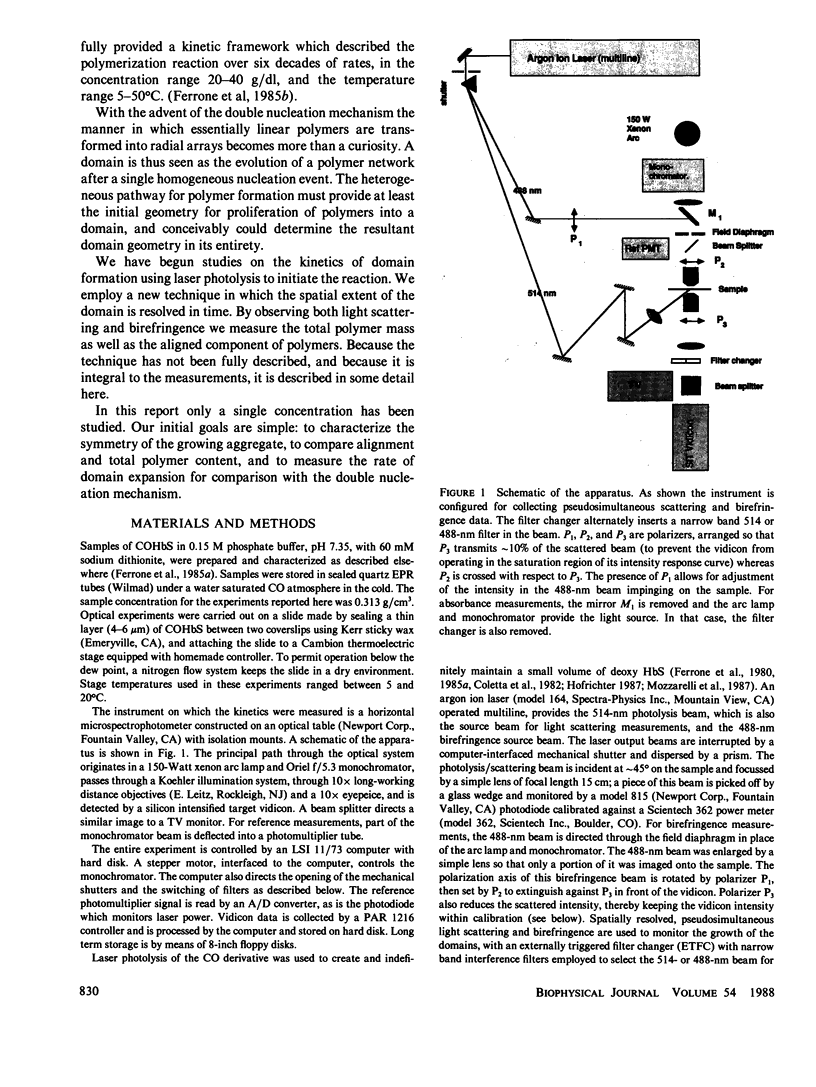
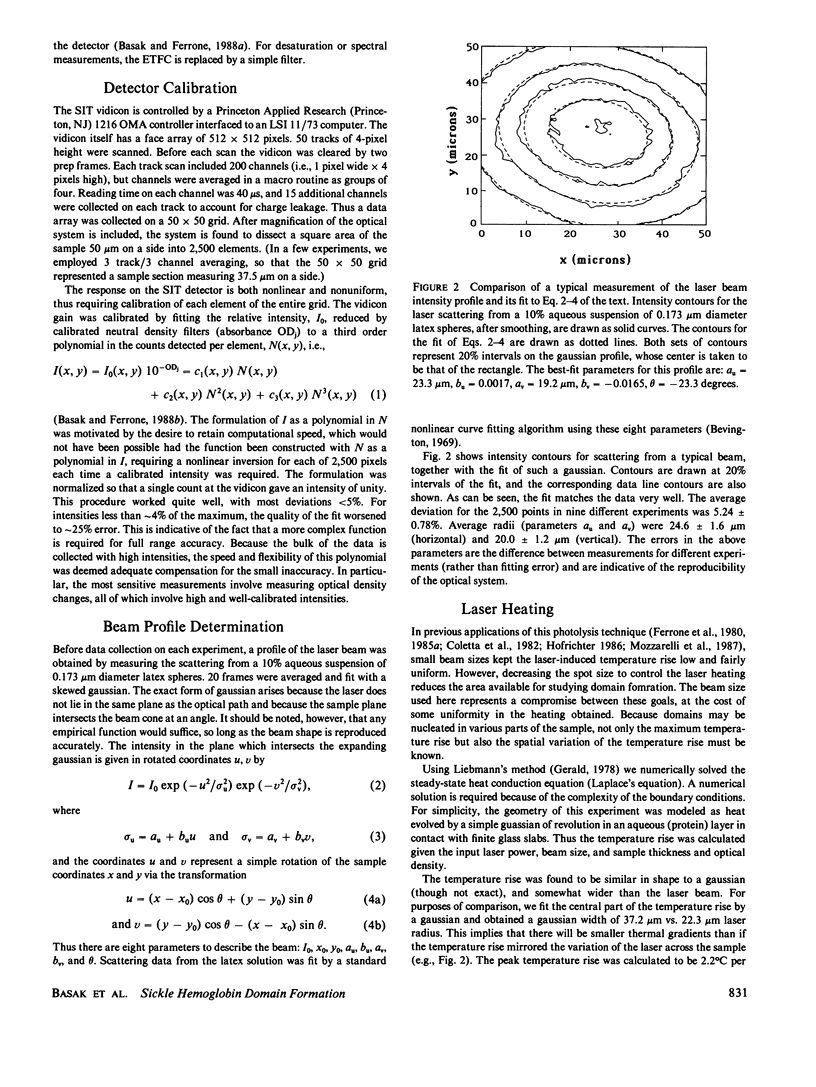
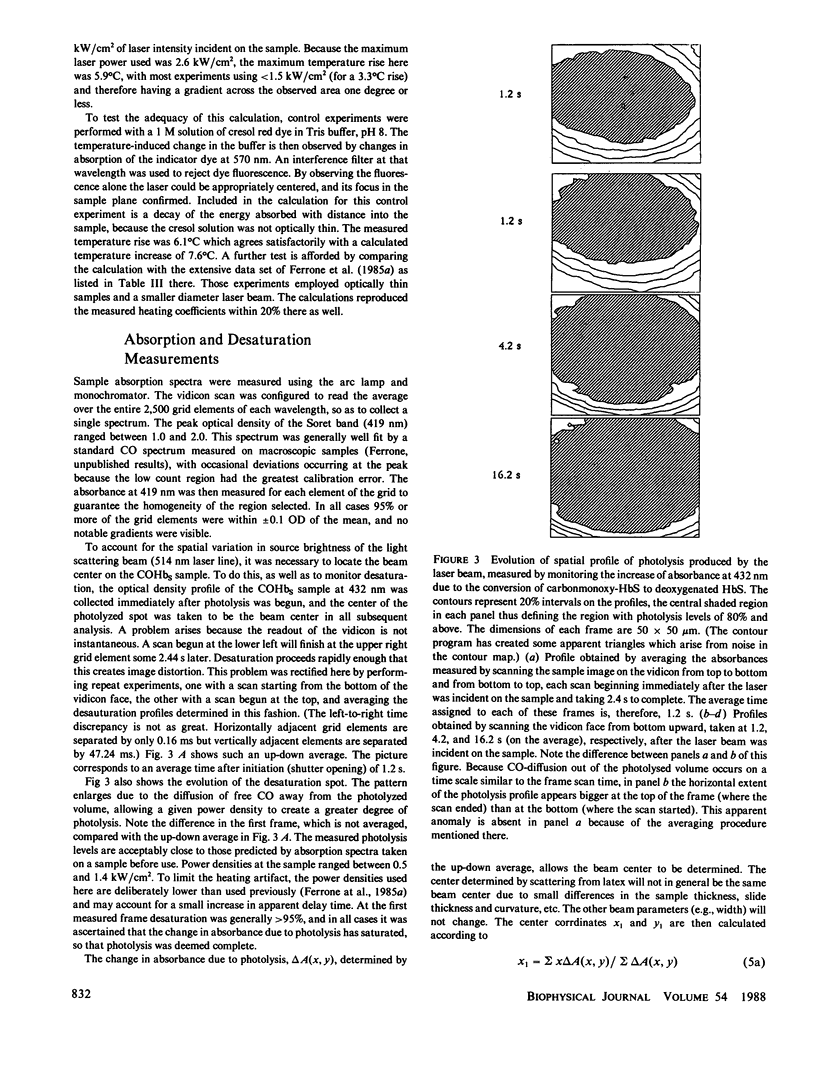
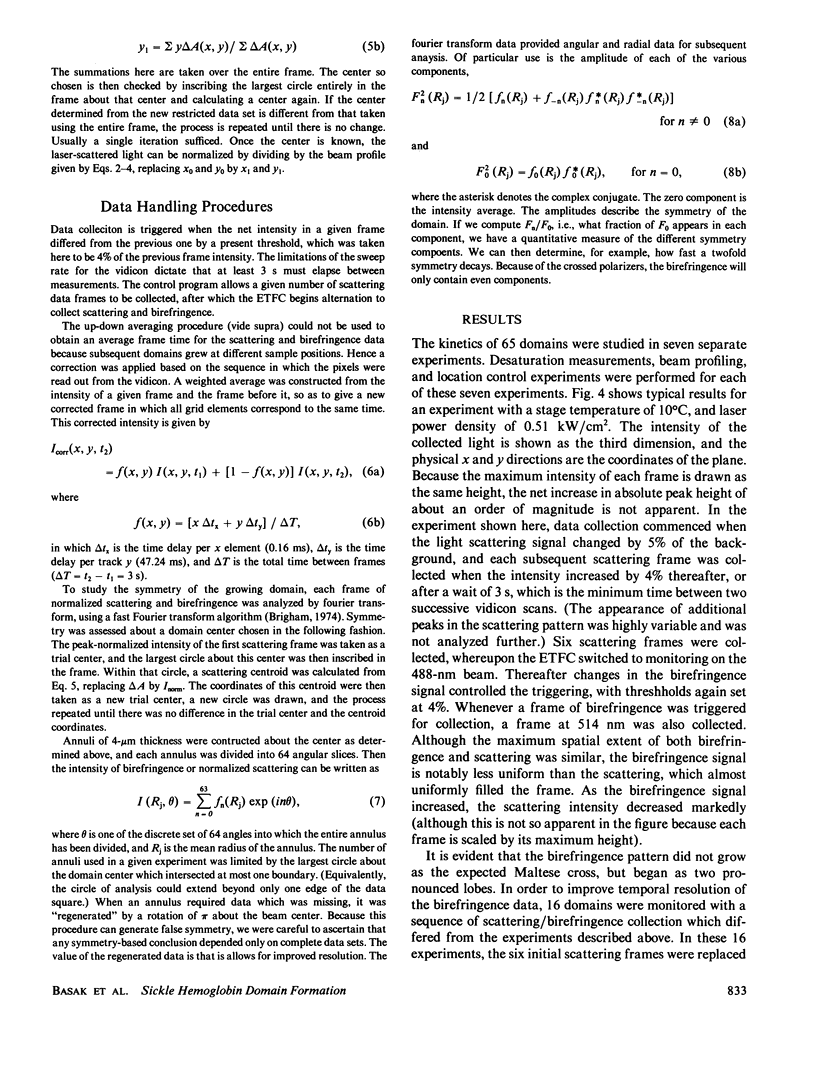
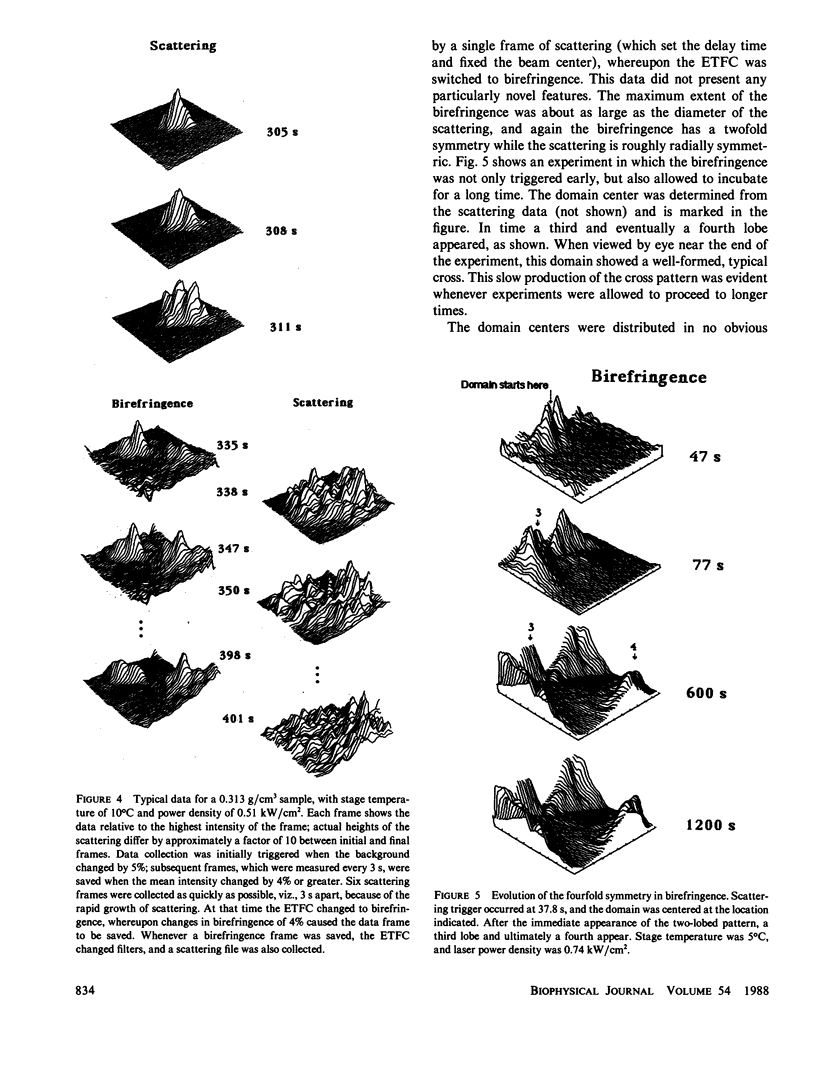
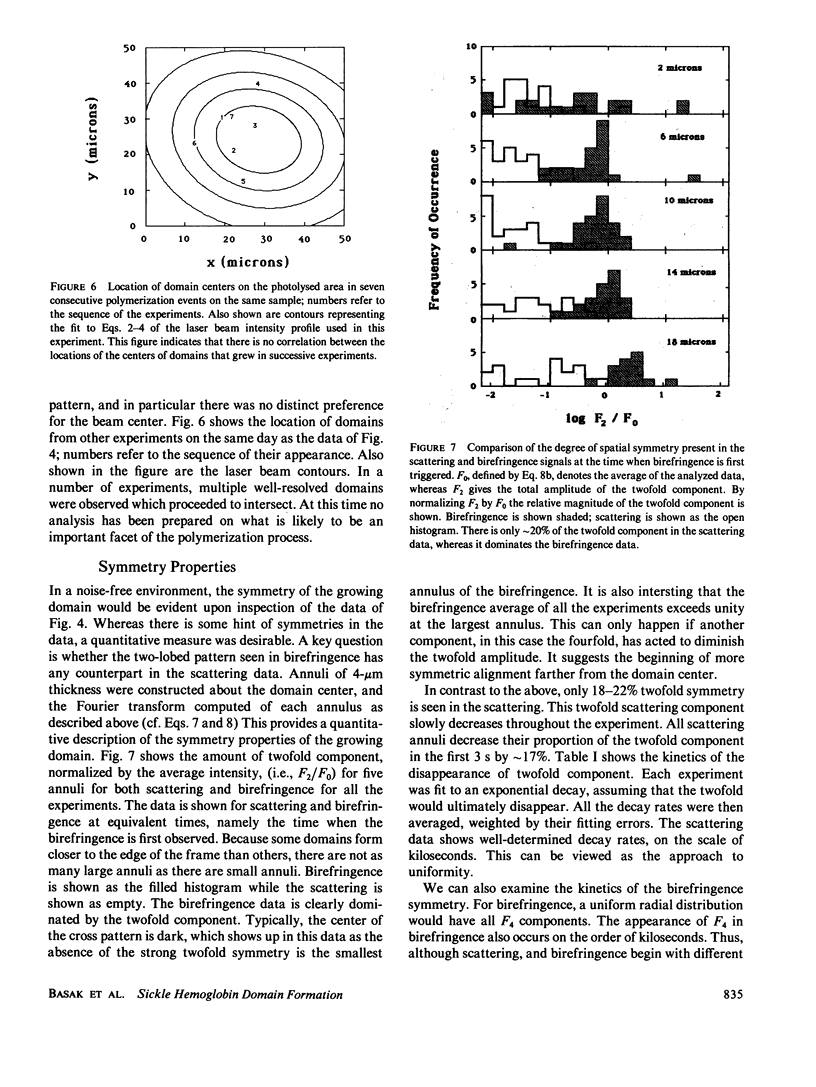
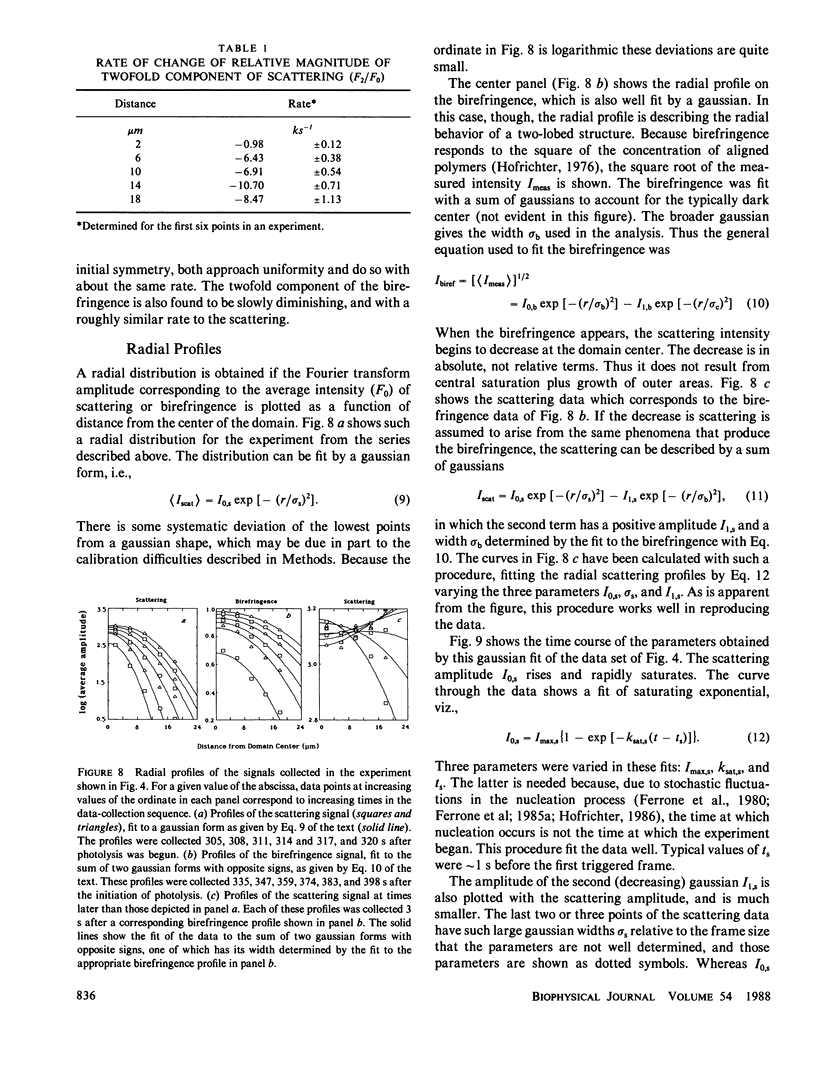
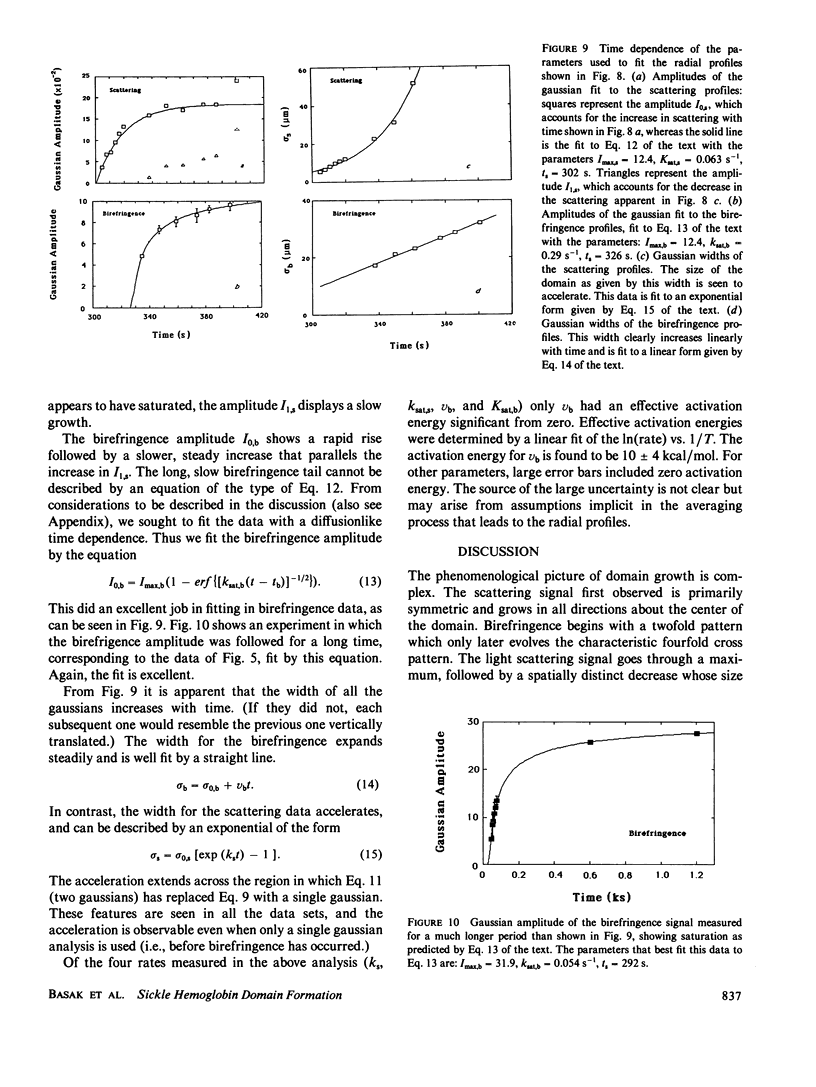
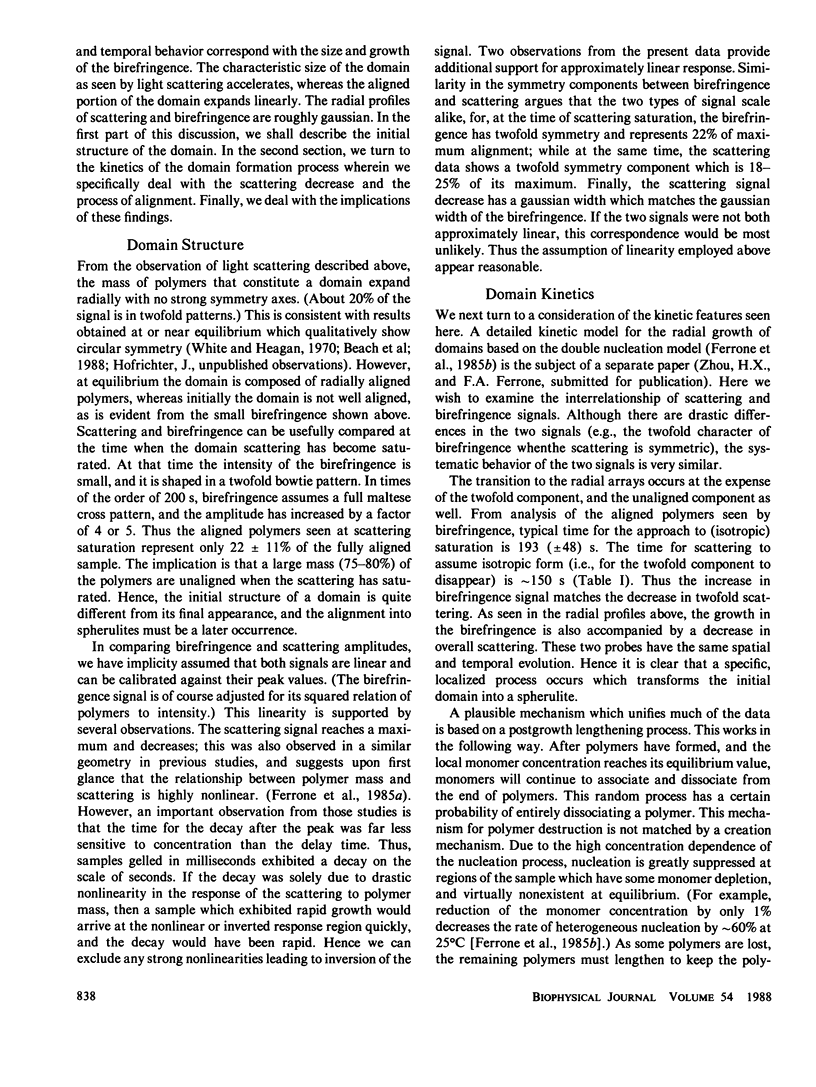
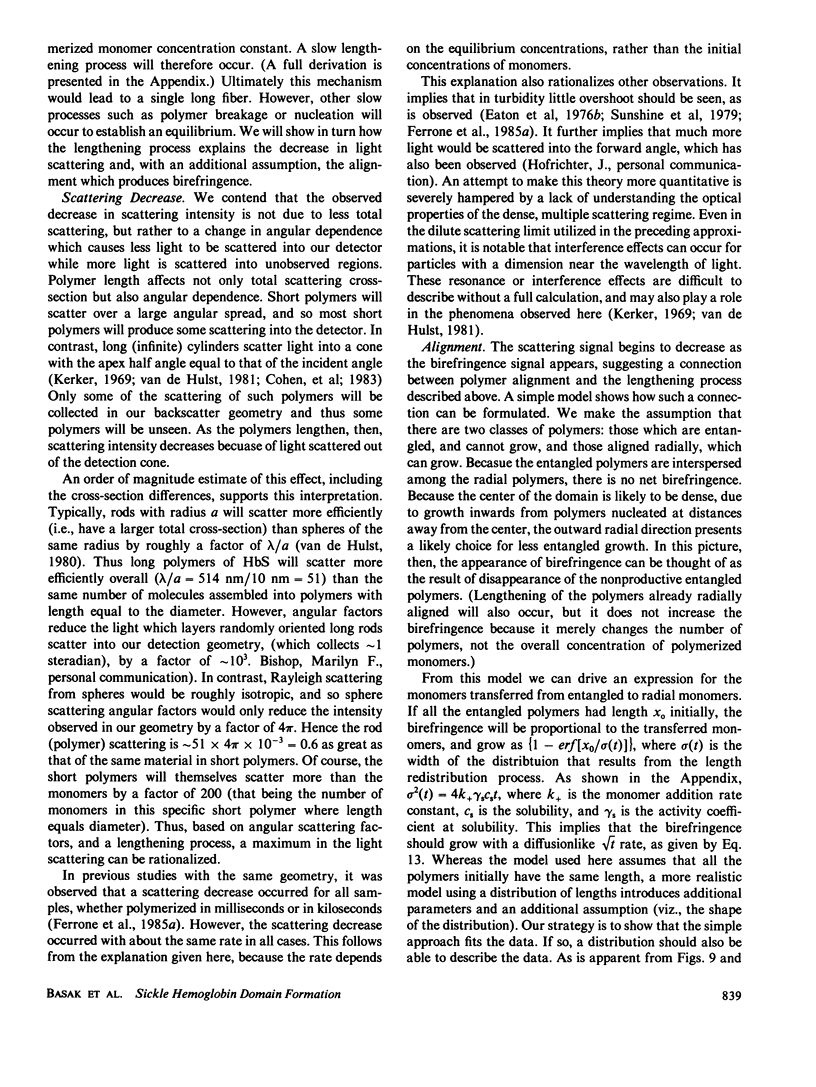
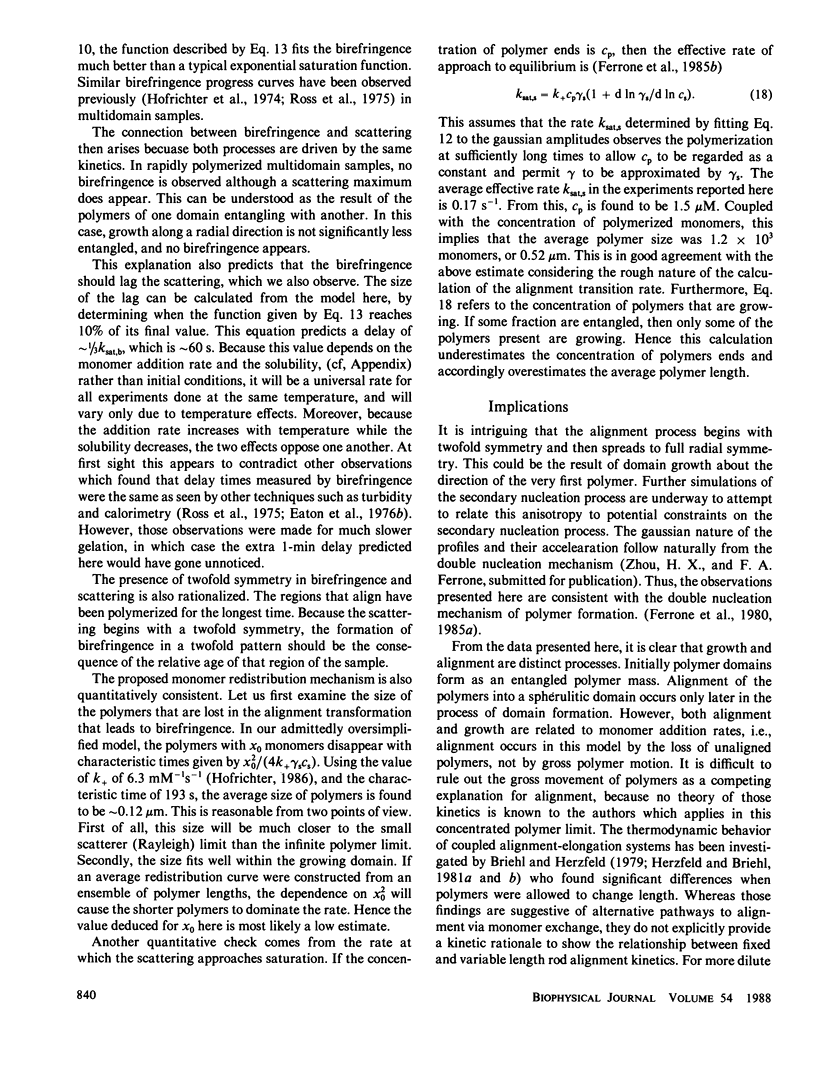
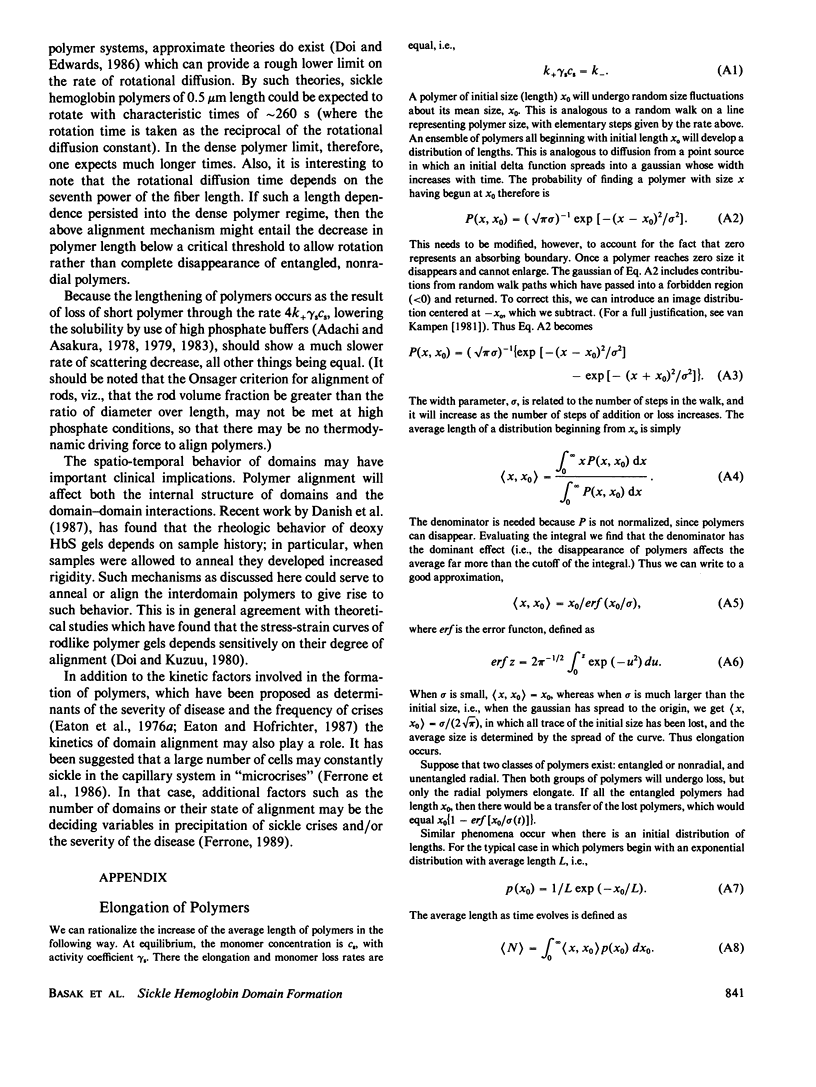
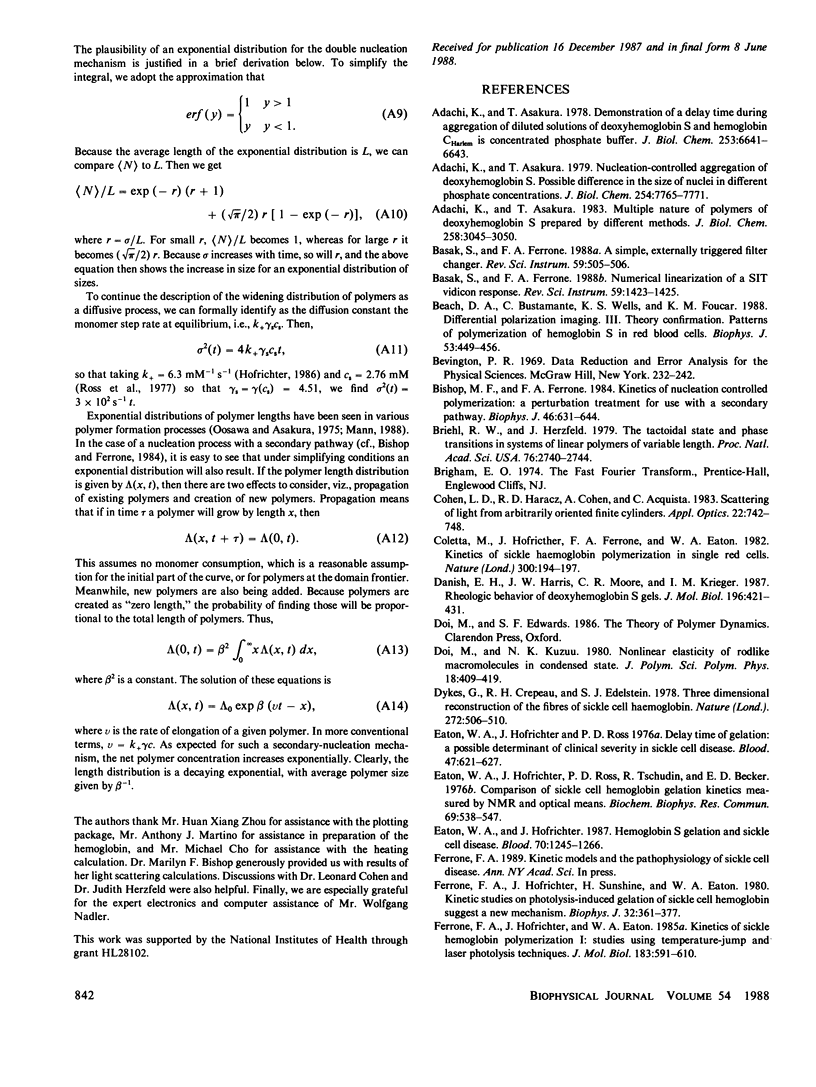
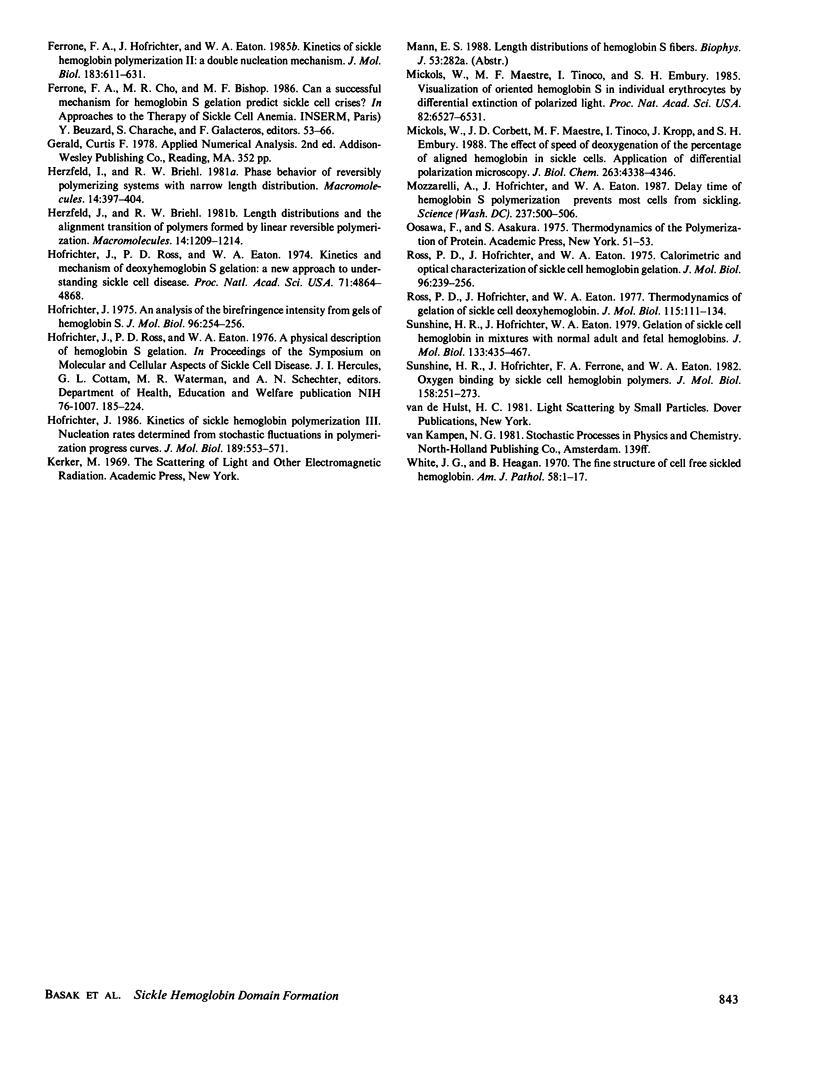
Images in this article
Selected References
These references are in PubMed. This may not be the complete list of references from this article.
- Adachi K., Asakura T. Demonstration of a delay time during aggregation of diluted solutions of deoxyhemoglobin S and hemoglobin CHarlem in concentrated phosphate buffer. J Biol Chem. 1978 Oct 10;253(19):6641–6643. [PubMed] [Google Scholar]
- Adachi K., Asakura T. Multiple nature of polymers of deoxyhemoglobin S prepared by different methods. J Biol Chem. 1983 Mar 10;258(5):3045–3050. [PubMed] [Google Scholar]
- Adachi K., Asakura T. Nucleation-controlled aggregation of deoxyhemoglobin S. Possible difference in the size of nuclei in different phosphate concentrations. J Biol Chem. 1979 Aug 25;254(16):7765–7771. [PubMed] [Google Scholar]
- Beach D. A., Bustamante C., Wells K. S., Foucar K. M. Differential polarization imaging. III. Theory confirmation. Patterns of polymerization of hemoglobin S in red blood sickle cells. Biophys J. 1988 Mar;53(3):449–456. doi: 10.1016/S0006-3495(88)83121-7. [DOI] [PMC free article] [PubMed] [Google Scholar]
- Bishop M. F., Ferrone F. A. Kinetics of nucleation-controlled polymerization. A perturbation treatment for use with a secondary pathway. Biophys J. 1984 Nov;46(5):631–644. doi: 10.1016/S0006-3495(84)84062-X. [DOI] [PMC free article] [PubMed] [Google Scholar]
- Briehl R. W., Herzfeld J. Tactoidal state and phase transitions in systems of linear polymers of variable length. Proc Natl Acad Sci U S A. 1979 Jun;76(6):2740–2744. doi: 10.1073/pnas.76.6.2740. [DOI] [PMC free article] [PubMed] [Google Scholar]
- Coletta M., Hofrichter J., Ferrone F. A., Eaton W. A. Kinetics of sickle haemoglobin polymerization in single red cells. Nature. 1982 Nov 11;300(5888):194–197. doi: 10.1038/300194a0. [DOI] [PubMed] [Google Scholar]
- Danish E. H., Harris J. W., Moore C. R., Krieger I. M. Rheologic behavior of deoxyhemoglobin S gels. J Mol Biol. 1987 Jul 20;196(2):421–431. doi: 10.1016/0022-2836(87)90702-9. [DOI] [PubMed] [Google Scholar]
- Dykes G., Crepeau R. H., Edelstein S. J. Three-dimensional reconstruction of the fibres of sickle cell haemoglobin. Nature. 1978 Apr 6;272(5653):506–510. doi: 10.1038/272506a0. [DOI] [PubMed] [Google Scholar]
- Eaton W. A., Hofrichter J. Hemoglobin S gelation and sickle cell disease. Blood. 1987 Nov;70(5):1245–1266. [PubMed] [Google Scholar]
- Eaton W. A., Hofrichter J., Ross P. D. Editorial: Delay time of gelation: a possible determinant of clinical severity in sickle cell disease. Blood. 1976 Apr;47(4):621–627. [PubMed] [Google Scholar]
- Eaton W. A., Hofrichter J., Ross P. D., Tschudin R. G., Becker E. D. Comparison of sickle cell hemoglobin gelation kinetics measured by NMR and optical methods. Biochem Biophys Res Commun. 1976 Mar 22;69(2):538–547. doi: 10.1016/0006-291x(76)90554-4. [DOI] [PubMed] [Google Scholar]
- Ferrone F. A., Hofrichter J., Eaton W. A. Kinetics of sickle hemoglobin polymerization. I. Studies using temperature-jump and laser photolysis techniques. J Mol Biol. 1985 Jun 25;183(4):591–610. doi: 10.1016/0022-2836(85)90174-3. [DOI] [PubMed] [Google Scholar]
- Ferrone F. A., Hofrichter J., Eaton W. A. Kinetics of sickle hemoglobin polymerization. II. A double nucleation mechanism. J Mol Biol. 1985 Jun 25;183(4):611–631. doi: 10.1016/0022-2836(85)90175-5. [DOI] [PubMed] [Google Scholar]
- Ferrone F. A., Hofrichter J., Sunshine H. R., Eaton W. A. Kinetic studies on photolysis-induced gelation of sickle cell hemoglobin suggest a new mechanism. Biophys J. 1980 Oct;32(1):361–380. doi: 10.1016/S0006-3495(80)84962-9. [DOI] [PMC free article] [PubMed] [Google Scholar]
- Hofrichter J. An analysis of the birefringence intensity from gels of hemoglobin S. J Mol Biol. 1975 Aug 5;96(2):254–256. doi: 10.1016/0022-2836(75)90346-0. [DOI] [PubMed] [Google Scholar]
- Hofrichter J. Kinetics of sickle hemoglobin polymerization. III. Nucleation rates determined from stochastic fluctuations in polymerization progress curves. J Mol Biol. 1986 Jun 5;189(3):553–571. doi: 10.1016/0022-2836(86)90324-4. [DOI] [PubMed] [Google Scholar]
- Hofrichter J., Ross P. D., Eaton W. A. Kinetics and mechanism of deoxyhemoglobin S gelation: a new approach to understanding sickle cell disease. Proc Natl Acad Sci U S A. 1974 Dec;71(12):4864–4868. doi: 10.1073/pnas.71.12.4864. [DOI] [PMC free article] [PubMed] [Google Scholar]
- Mickols W. E., Corbett J. D., Maestre M. F., Tinoco I., Jr, Kropp J., Embury S. H. The effect of speed of deoxygenation on the percentage of aligned hemoglobin in sickle cells. Application of differential polarization microscopy. J Biol Chem. 1988 Mar 25;263(9):4338–4346. [PubMed] [Google Scholar]
- Mickols W., Maestre M. F., Tinoco I., Jr, Embury S. H. Visualization of oriented hemoglobin S in individual erythrocytes by differential extinction of polarized light. Proc Natl Acad Sci U S A. 1985 Oct;82(19):6527–6531. doi: 10.1073/pnas.82.19.6527. [DOI] [PMC free article] [PubMed] [Google Scholar]
- Mozzarelli A., Hofrichter J., Eaton W. A. Delay time of hemoglobin S polymerization prevents most cells from sickling in vivo. Science. 1987 Jul 31;237(4814):500–506. doi: 10.1126/science.3603036. [DOI] [PubMed] [Google Scholar]
- Ross P. D., Hofrichter J., Eaton W. A. Calorimetric and optical characterization of sickle cell hemoglobin gelation. J Mol Biol. 1975 Aug 5;96(2):239–253. doi: 10.1016/0022-2836(75)90345-9. [DOI] [PubMed] [Google Scholar]
- Ross P. D., Hofrichter J., Eaton W. A. Thermodynamics of gelation of sickle cell deoxyhemoglobin. J Mol Biol. 1977 Sep 15;115(2):111–134. doi: 10.1016/0022-2836(77)90093-6. [DOI] [PubMed] [Google Scholar]
- Sunshine H. R., Hofrichter J., Eaton W. A. Gelation of sickle cell hemoglobin in mixtures with normal adult and fetal hemoglobins. J Mol Biol. 1979 Oct 9;133(4):435–467. doi: 10.1016/0022-2836(79)90402-9. [DOI] [PubMed] [Google Scholar]
- Sunshine H. R., Hofrichter J., Ferrone F. A., Eaton W. A. Oxygen binding by sickle cell hemoglobin polymers. J Mol Biol. 1982 Jun 25;158(2):251–273. doi: 10.1016/0022-2836(82)90432-6. [DOI] [PubMed] [Google Scholar]
- White J. G., Heagan B. The fine structure of cell-free sickled hemoglobin. Am J Pathol. 1970 Jan;58(1):1–17. [PMC free article] [PubMed] [Google Scholar]