Abstract
Cyclic patterns of motor neuron activity are involved in the production of many rhythmic movements, such as walking, swimming, and scratching. These movements are controlled by neural circuits referred to as central pattern generators (CPGs). Some of these circuits function in the absence of both internal pacemakers and external feedback. We describe an associative neural network model whose dynamic behavior is similar to that of CPGs. The theory predicts the strength of all possible connections between pairs of neurons on the basis of the outputs of the CPG. It also allows the mean operating levels of the neurons to be deduced from the measured synaptic strengths between the pairs of neurons. We apply our theory to the CPG controlling escape swimming in the mollusk Tritonia diomedea. The basic rhythmic behavior is shown to be consistent with a simplified model that approximates neurons as threshold units and slow synaptic responses as elementary time delays. The model we describe may have relevance to other fixed action behaviors, as well as to the learning, recall, and recognition of temporally ordered information.
Full text
PDF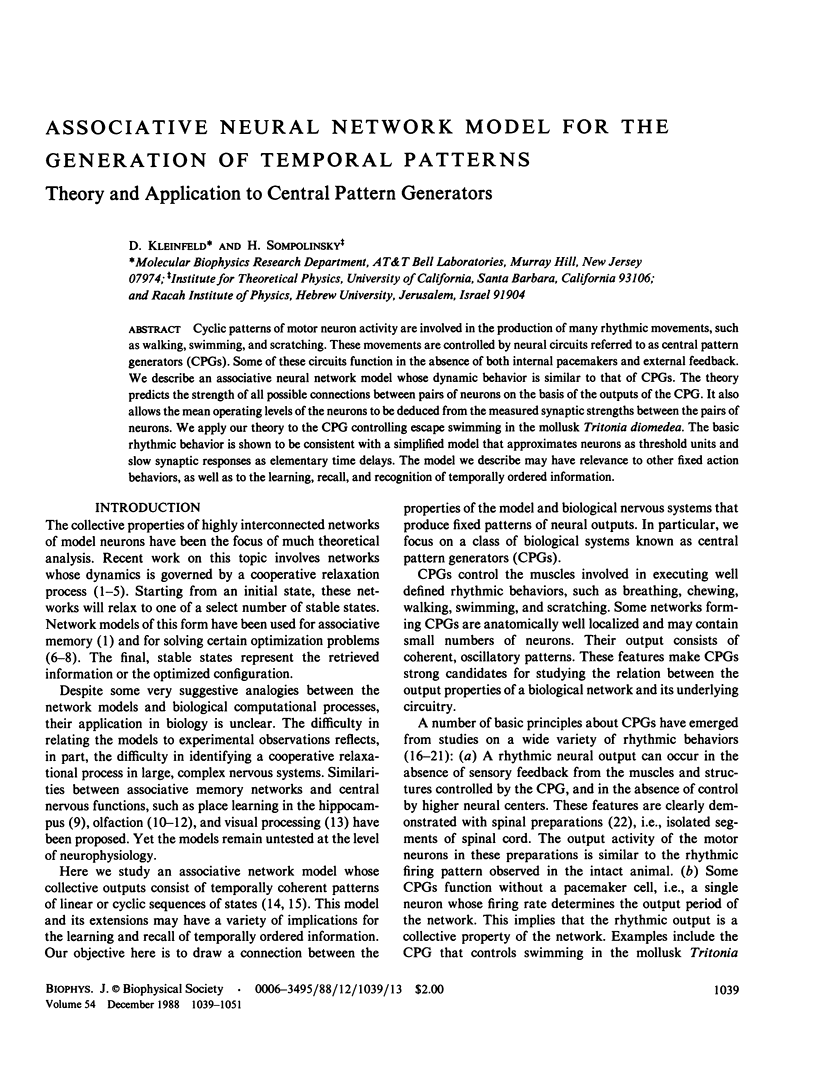
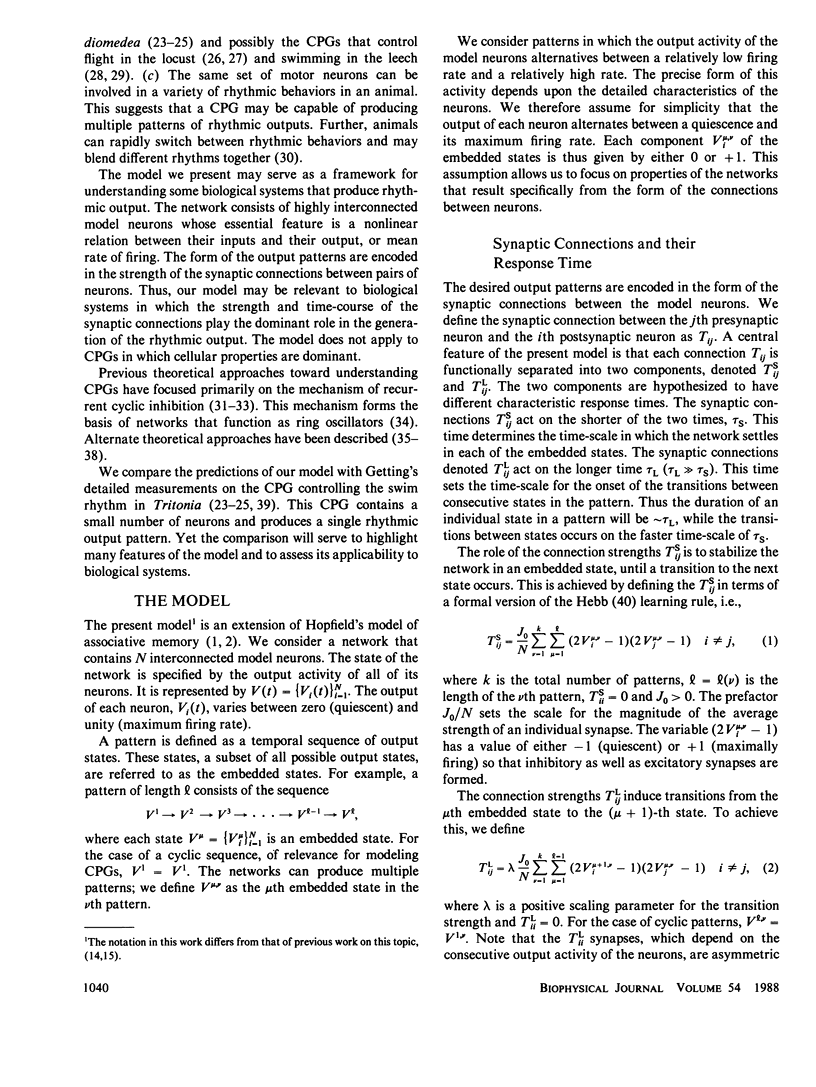
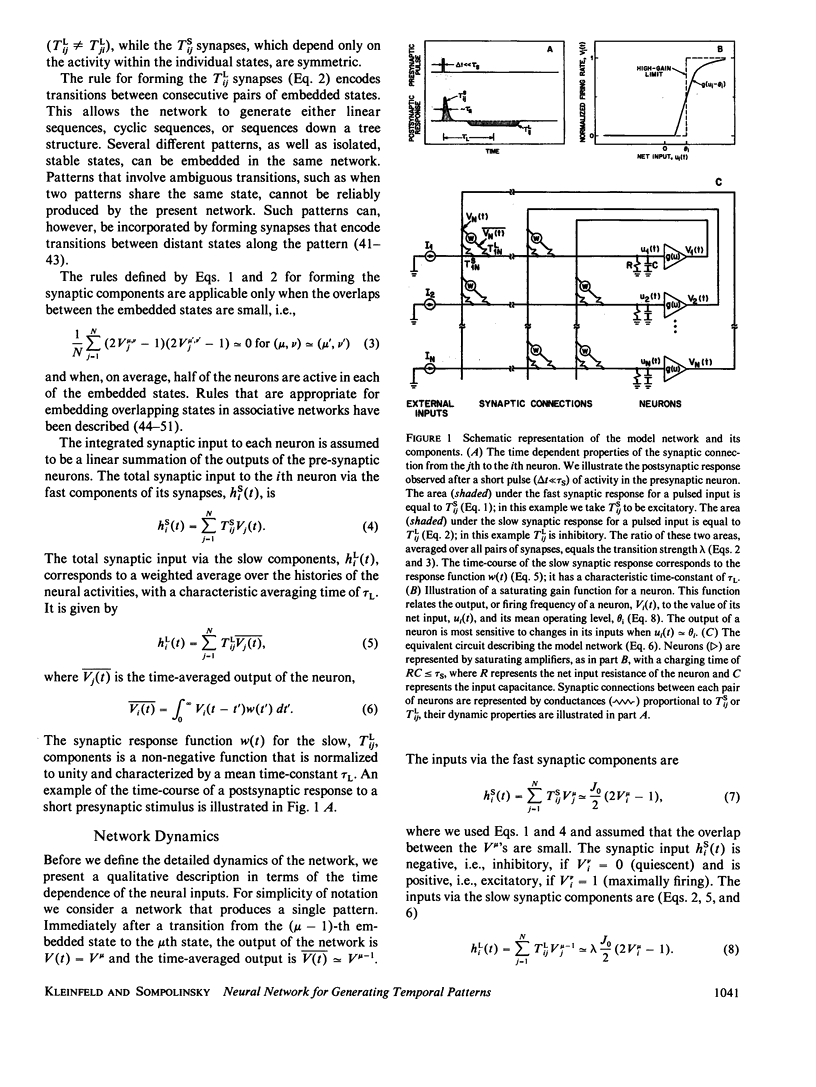
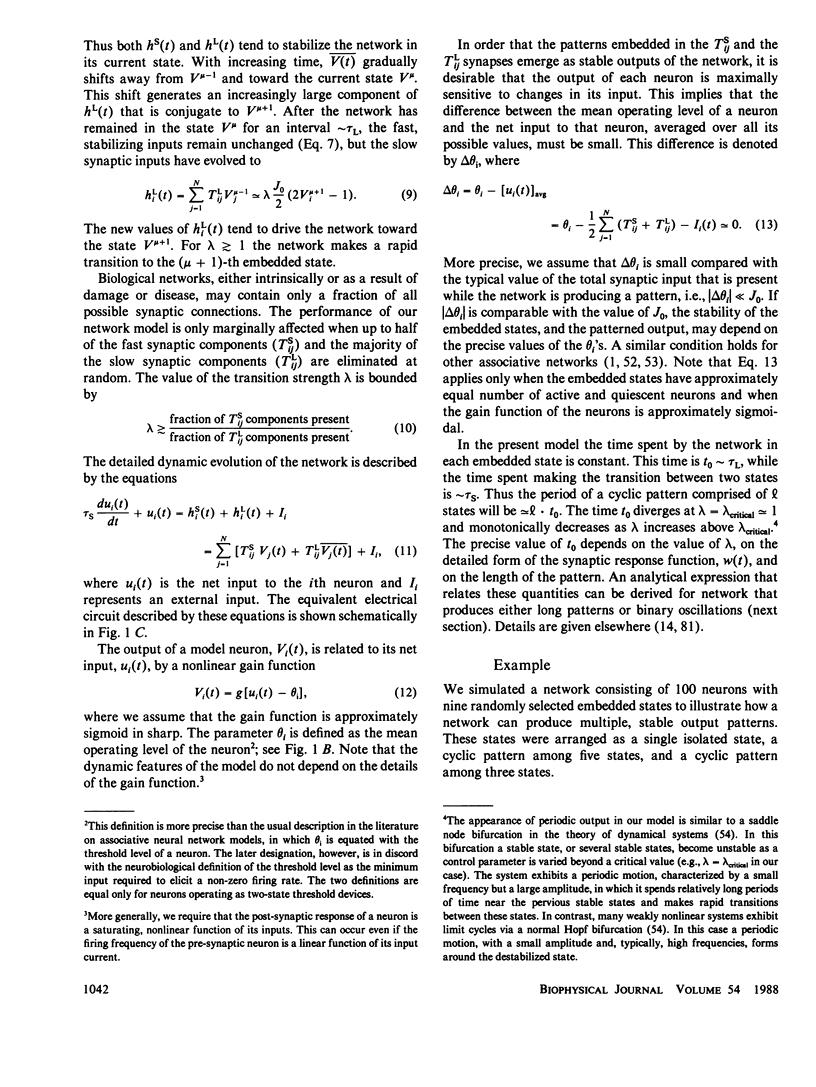
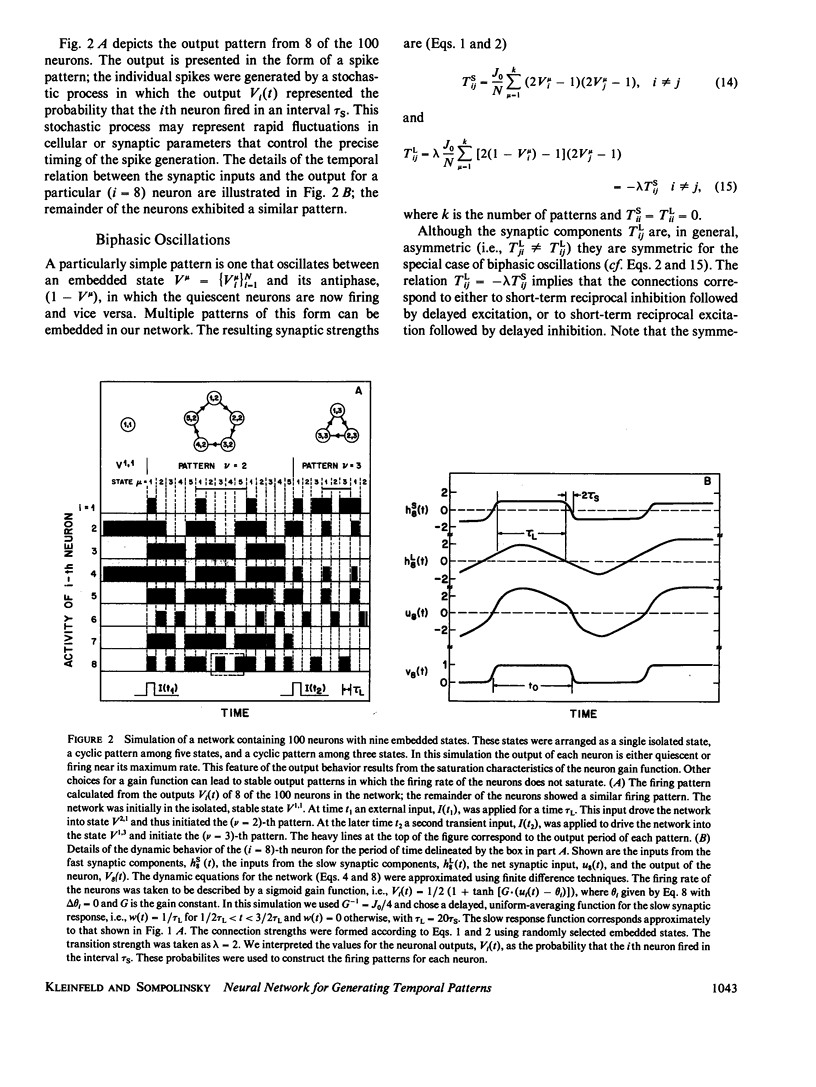
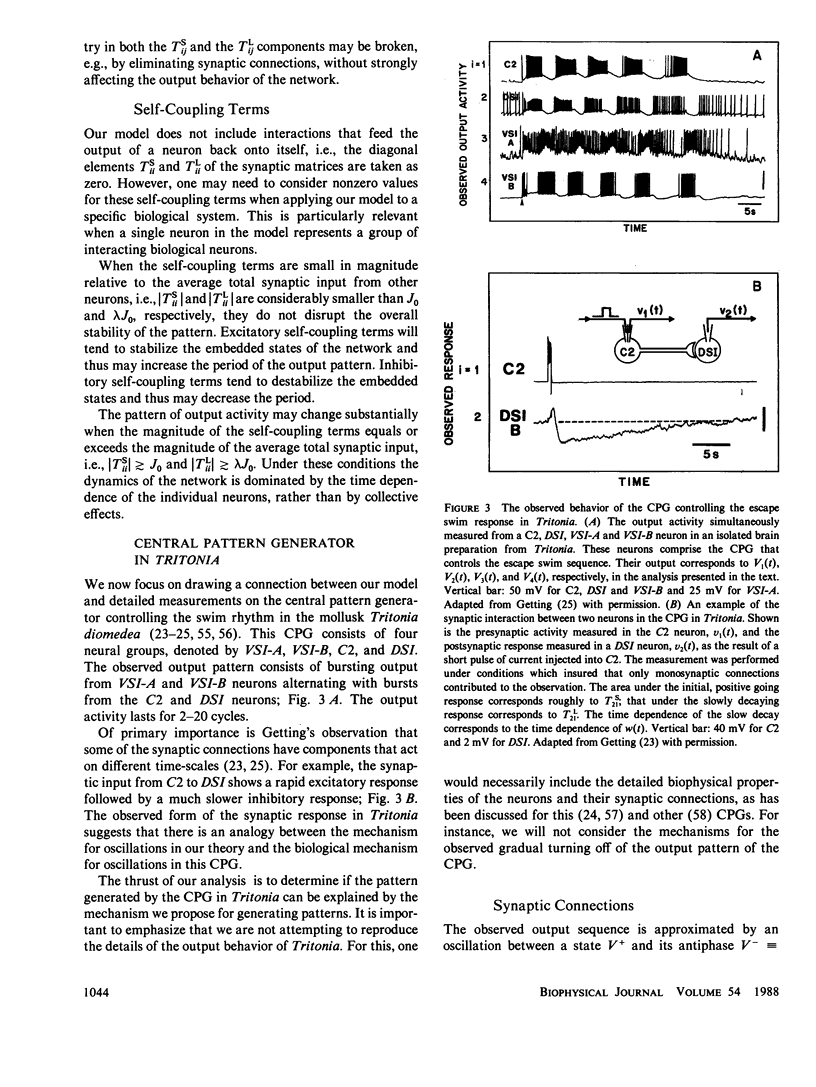
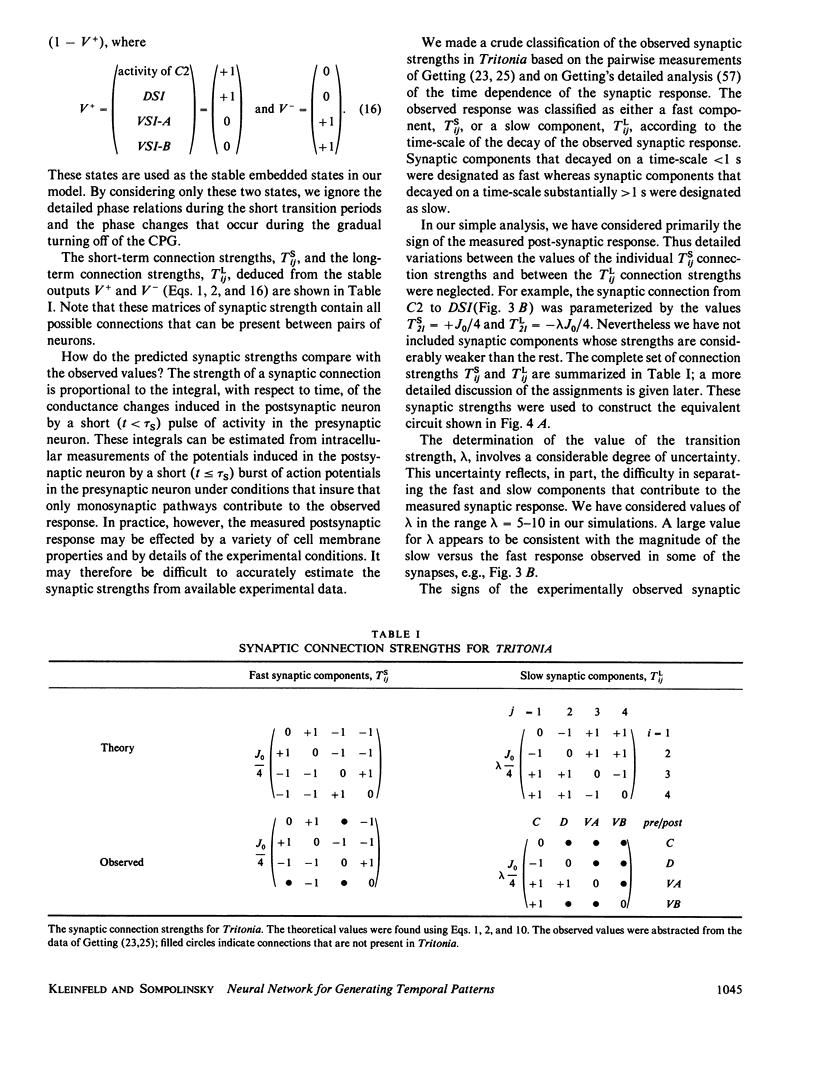
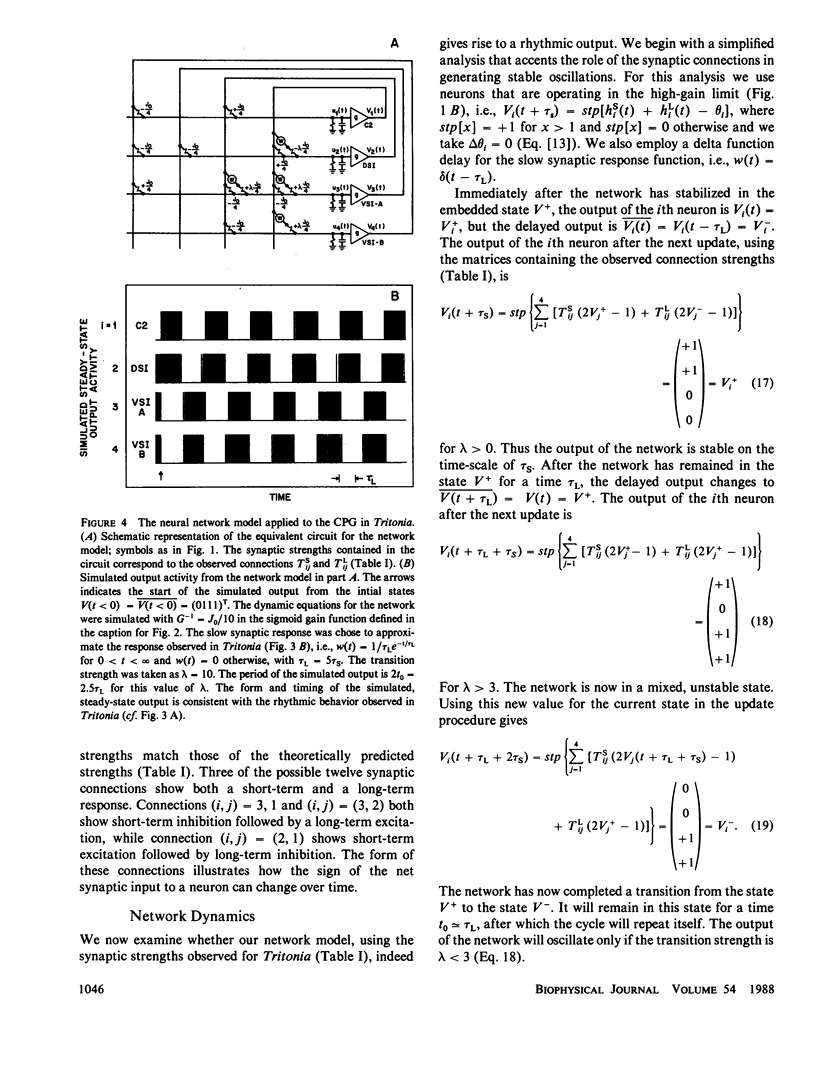
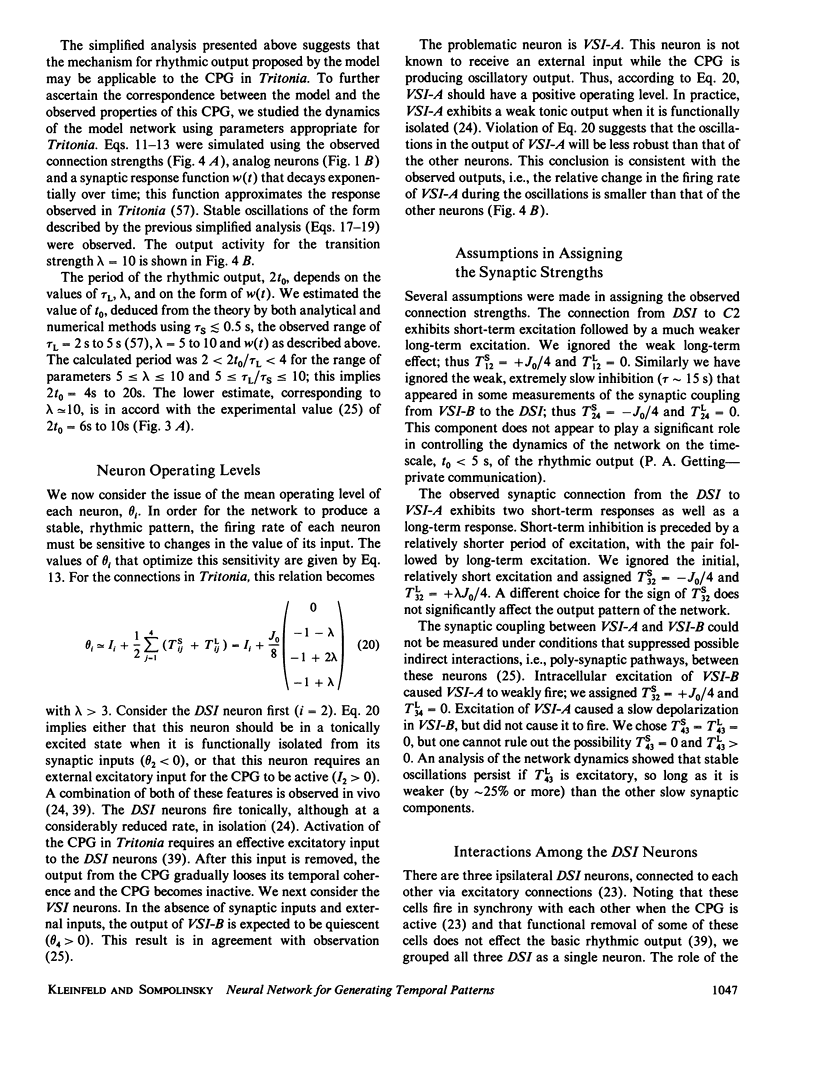
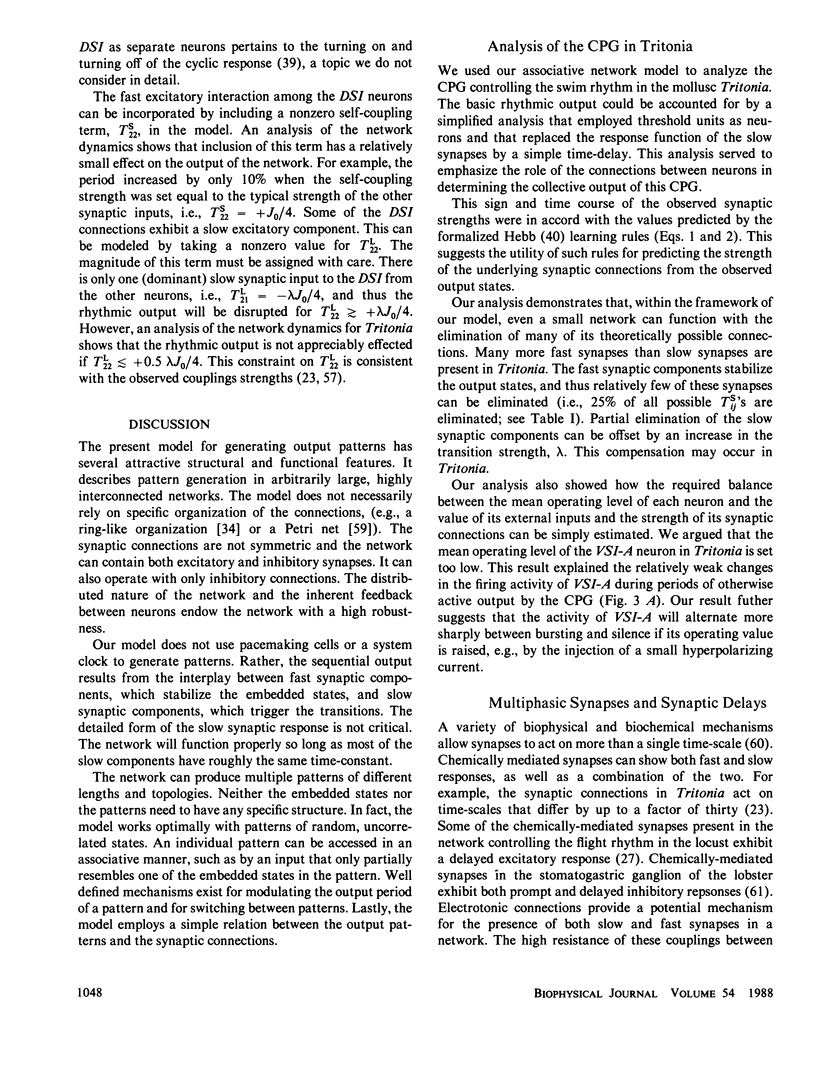
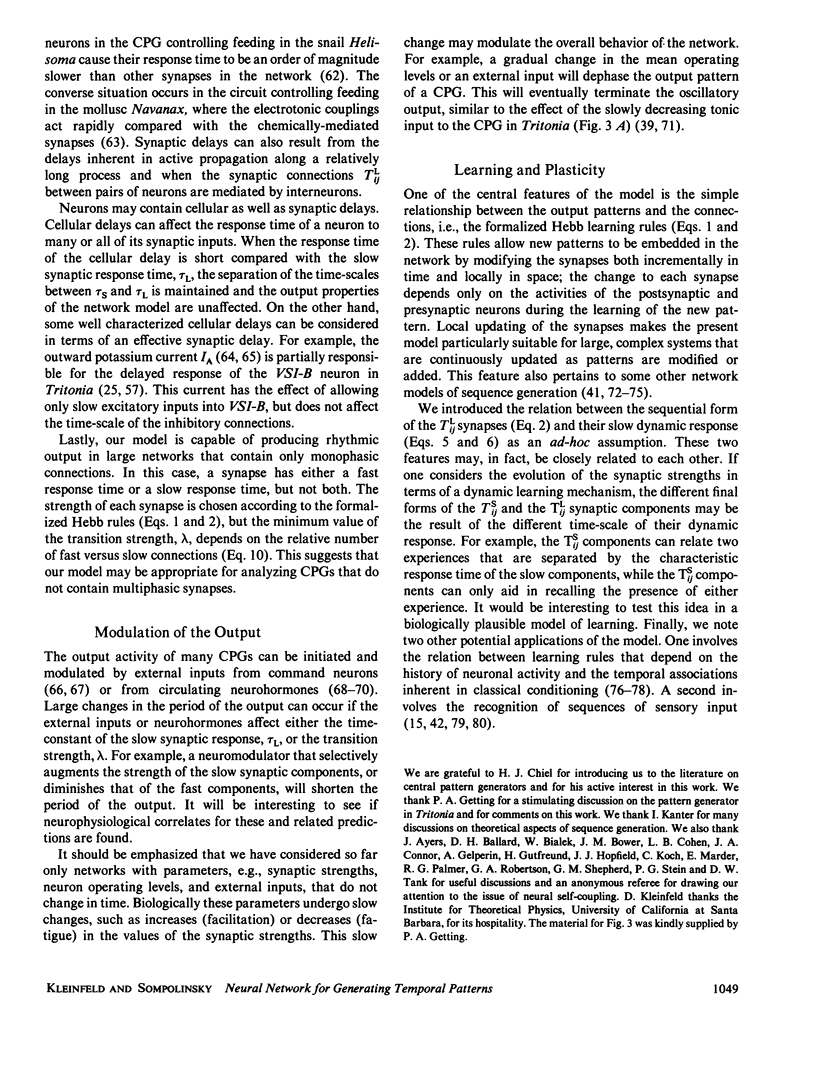
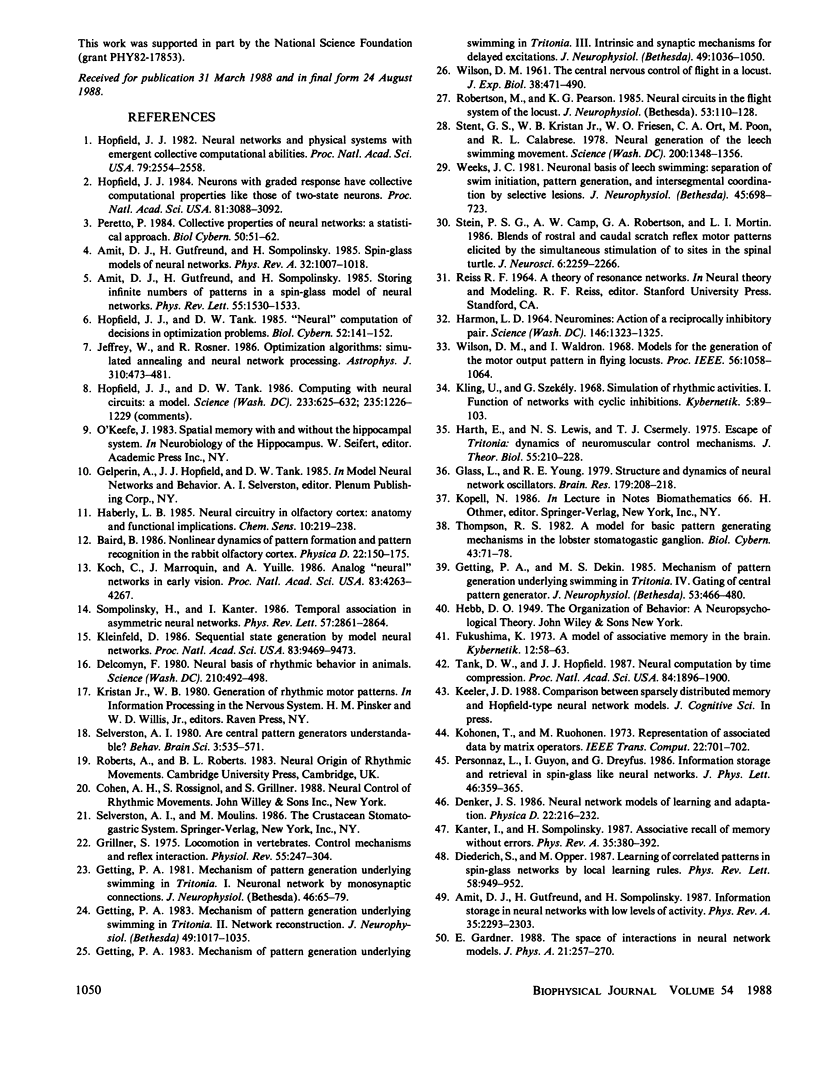
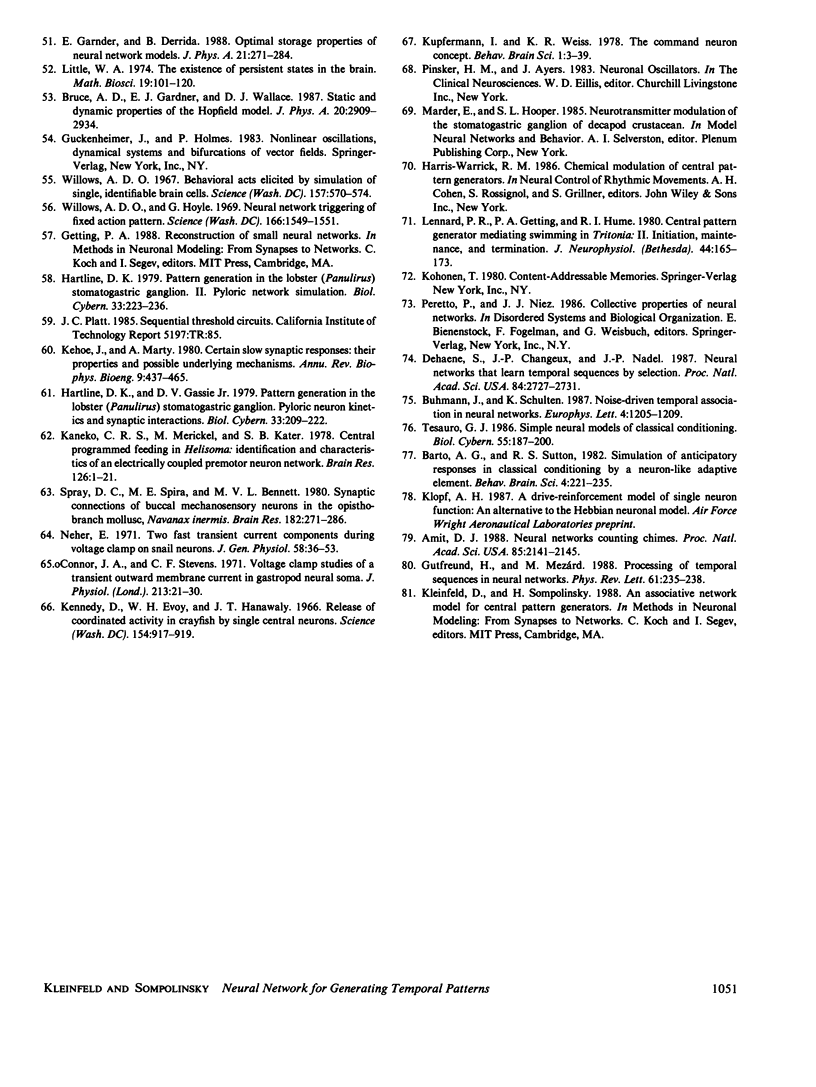
Images in this article
Selected References
These references are in PubMed. This may not be the complete list of references from this article.
- Amit D. J. Neural networks counting chimes. Proc Natl Acad Sci U S A. 1988 Apr;85(7):2141–2145. doi: 10.1073/pnas.85.7.2141. [DOI] [PMC free article] [PubMed] [Google Scholar]
- Amit DJ, Gutfreund H, Sompolinsky H. Information storage in neural networks with low levels of activity. Phys Rev A Gen Phys. 1987 Mar 1;35(5):2293–2303. doi: 10.1103/physreva.35.2293. [DOI] [PubMed] [Google Scholar]
- Amit DJ, Gutfreund H, Sompolinsky H. Spin-glass models of neural networks. Phys Rev A Gen Phys. 1985 Aug;32(2):1007–1018. doi: 10.1103/physreva.32.1007. [DOI] [PubMed] [Google Scholar]
- Amit DJ, Gutfreund H, Sompolinsky H. Storing infinite numbers of patterns in a spin-glass model of neural networks. Phys Rev Lett. 1985 Sep 30;55(14):1530–1533. doi: 10.1103/PhysRevLett.55.1530. [DOI] [PubMed] [Google Scholar]
- Barto A. G., Sutton R. S. Simulation of anticipatory responses in classical conditioning by a neuron-like adaptive element. Behav Brain Res. 1982 Mar;4(3):221–235. doi: 10.1016/0166-4328(82)90001-8. [DOI] [PubMed] [Google Scholar]
- Connor J. A., Stevens C. F. Voltage clamp studies of a transient outward membrane current in gastropod neural somata. J Physiol. 1971 Feb;213(1):21–30. doi: 10.1113/jphysiol.1971.sp009365. [DOI] [PMC free article] [PubMed] [Google Scholar]
- Dehaene S., Changeux J. P., Nadal J. P. Neural networks that learn temporal sequences by selection. Proc Natl Acad Sci U S A. 1987 May;84(9):2727–2731. doi: 10.1073/pnas.84.9.2727. [DOI] [PMC free article] [PubMed] [Google Scholar]
- Delcomyn F. Neural basis of rhythmic behavior in animals. Science. 1980 Oct 31;210(4469):492–498. doi: 10.1126/science.7423199. [DOI] [PubMed] [Google Scholar]
- Diederich S, Opper M. Learning of correlated patterns in spin-glass networks by local learning rules. Phys Rev Lett. 1987 Mar 2;58(9):949–952. doi: 10.1103/PhysRevLett.58.949. [DOI] [PubMed] [Google Scholar]
- Fukushima K. A model of associative memory in the brain. Kybernetik. 1973 Feb;12(2):58–63. doi: 10.1007/BF00272461. [DOI] [PubMed] [Google Scholar]
- Getting P. A., Dekin M. S. Mechanisms of pattern generation underlying swimming in Tritonia. IV. Gating of central pattern generator. J Neurophysiol. 1985 Feb;53(2):466–480. doi: 10.1152/jn.1985.53.2.466. [DOI] [PubMed] [Google Scholar]
- Getting P. A. Mechanisms of pattern generation underlying swimming in Tritonia. I. Neuronal network formed by monosynaptic connections. J Neurophysiol. 1981 Jul;46(1):65–79. doi: 10.1152/jn.1981.46.1.65. [DOI] [PubMed] [Google Scholar]
- Getting P. A. Mechanisms of pattern generation underlying swimming in Tritonia. II. Network reconstruction. J Neurophysiol. 1983 Apr;49(4):1017–1035. doi: 10.1152/jn.1983.49.4.1017. [DOI] [PubMed] [Google Scholar]
- Getting P. A. Mechanisms of pattern generation underlying swimming in Tritonia. III. Intrinsic and synaptic mechanisms for delayed excitation. J Neurophysiol. 1983 Apr;49(4):1036–1050. doi: 10.1152/jn.1983.49.4.1036. [DOI] [PubMed] [Google Scholar]
- Glass L., Young R. E. Structure and dynamics of neural network oscillators. Brain Res. 1979 Dec 28;179(2):207–218. doi: 10.1016/0006-8993(79)90439-6. [DOI] [PubMed] [Google Scholar]
- Grillner S. Locomotion in vertebrates: central mechanisms and reflex interaction. Physiol Rev. 1975 Apr;55(2):247–304. doi: 10.1152/physrev.1975.55.2.247. [DOI] [PubMed] [Google Scholar]
- Gutfreund H, Mezard M. Processing of temporal sequences in neural networks. Phys Rev Lett. 1988 Jul 11;61(2):235–238. doi: 10.1103/PhysRevLett.61.235. [DOI] [PubMed] [Google Scholar]
- HARMON L. D. NEUROMIMES: ACTION OF A RECIPROCALLY INHIBITORY PAIR. Science. 1964 Dec 4;146(3649):1323–1325. doi: 10.1126/science.146.3649.1323. [DOI] [PubMed] [Google Scholar]
- Harth E., Lewis N. S. The escape of Tritonia: dynamics of a neuromuscular control mechanism. J Theor Biol. 1975 Nov;55(1):201–228. doi: 10.1016/s0022-5193(75)80116-0. [DOI] [PubMed] [Google Scholar]
- Hartline D. K., Gassie D. V., Jr Pattern generation in the lobster (Panulirus) stomatogastric ganglion. I. Pyloric neuron kinetics and synaptic interactions. Biol Cybern. 1979 Aug;33(4):209–222. doi: 10.1007/BF00337410. [DOI] [PubMed] [Google Scholar]
- Hartline D. K. Pattern generation in the lobster (Panulirus) stomatogastric ganglion. II. Pyloric network simulation. Biol Cybern. 1979 Aug;33(4):223–236. doi: 10.1007/BF00337411. [DOI] [PubMed] [Google Scholar]
- Hopfield J. J. Neural networks and physical systems with emergent collective computational abilities. Proc Natl Acad Sci U S A. 1982 Apr;79(8):2554–2558. doi: 10.1073/pnas.79.8.2554. [DOI] [PMC free article] [PubMed] [Google Scholar]
- Hopfield J. J. Neurons with graded response have collective computational properties like those of two-state neurons. Proc Natl Acad Sci U S A. 1984 May;81(10):3088–3092. doi: 10.1073/pnas.81.10.3088. [DOI] [PMC free article] [PubMed] [Google Scholar]
- Hopfield J. J., Tank D. W. "Neural" computation of decisions in optimization problems. Biol Cybern. 1985;52(3):141–152. doi: 10.1007/BF00339943. [DOI] [PubMed] [Google Scholar]
- Hopfield J. J., Tank D. W. Computing with neural circuits: a model. Science. 1986 Aug 8;233(4764):625–633. doi: 10.1126/science.3755256. [DOI] [PubMed] [Google Scholar]
- Kaneko C. R., Merickel M., Kater S. B. Centrally programmed feeding in Helisoma: identification and chracteristics of an electrically coupled premotor neuron network. Brain Res. 1978 May 5;146(1):1–21. doi: 10.1016/0006-8993(78)90214-7. [DOI] [PubMed] [Google Scholar]
- Kanter I, I, Sompolinsky H. Associative recall of memory without errors. Phys Rev A Gen Phys. 1987 Jan 1;35(1):380–392. doi: 10.1103/physreva.35.380. [DOI] [PubMed] [Google Scholar]
- Kehoe J. S., Marty A. Certain slow synaptic responses: their properties and possible underlying mechanisms. Annu Rev Biophys Bioeng. 1980;9:437–465. doi: 10.1146/annurev.bb.09.060180.002253. [DOI] [PubMed] [Google Scholar]
- Kennedy D., Evoy W. H., Hanawalt J. T. Release of coordinated behavior in crayfish by single central neurons. Science. 1966 Nov 18;154(3751):917–919. doi: 10.1126/science.154.3751.917. [DOI] [PubMed] [Google Scholar]
- Kleinfeld D. Sequential state generation by model neural networks. Proc Natl Acad Sci U S A. 1986 Dec;83(24):9469–9473. doi: 10.1073/pnas.83.24.9469. [DOI] [PMC free article] [PubMed] [Google Scholar]
- Kling U., Székely G. Simulation of rhythmic nervous activities. I. Function of networks with cyclic inhibitions. Kybernetik. 1968 Sep;5(3):89–103. doi: 10.1007/BF00288899. [DOI] [PubMed] [Google Scholar]
- Koch C., Marroquin J., Yuille A. Analog "neuronal" networks in early vision. Proc Natl Acad Sci U S A. 1986 Jun;83(12):4263–4267. doi: 10.1073/pnas.83.12.4263. [DOI] [PMC free article] [PubMed] [Google Scholar]
- Lennard P. R., Getting P. A., Hume R. I. Central pattern generator mediating swimming in Tritonia. II. Initiation, maintenance, and termination. J Neurophysiol. 1980 Jul;44(1):165–173. doi: 10.1152/jn.1980.44.1.165. [DOI] [PubMed] [Google Scholar]
- Neher E. Two fast transient current components during voltage clamp on snail neurons. J Gen Physiol. 1971 Jul;58(1):36–53. doi: 10.1085/jgp.58.1.36. [DOI] [PMC free article] [PubMed] [Google Scholar]
- Peretto P. Collective properties of neural networks: a statistical physics approach. Biol Cybern. 1984;50(1):51–62. doi: 10.1007/BF00317939. [DOI] [PubMed] [Google Scholar]
- Robertson R. M., Pearson K. G. Neural circuits in the flight system of the locust. J Neurophysiol. 1985 Jan;53(1):110–128. doi: 10.1152/jn.1985.53.1.110. [DOI] [PubMed] [Google Scholar]
- Sompolinsky H, Kanter I., I Temporal association in asymmetric neural networks. Phys Rev Lett. 1986 Dec 1;57(22):2861–2864. doi: 10.1103/PhysRevLett.57.2861. [DOI] [PubMed] [Google Scholar]
- Spray D. C., Spira M. E., Bennett M. V. Synaptic connections of buccal mechanosensory neurons in the opisthobranch mollusc, Navanax inermis. Brain Res. 1980 Jan 27;182(2):271–286. doi: 10.1016/0006-8993(80)91188-9. [DOI] [PubMed] [Google Scholar]
- Stein P. S., Camp A. W., Robertson G. A., Mortin L. I. Blends of rostral and caudal scratch reflex motor patterns elicited by simultaneous stimulation of two sites in the spinal turtle. J Neurosci. 1986 Aug;6(8):2259–2266. doi: 10.1523/JNEUROSCI.06-08-02259.1986. [DOI] [PMC free article] [PubMed] [Google Scholar]
- Stent G. S., Kristan W. B., Jr, Friesen W. O., Ort C. A., Poon M., Calabrese R. L. Neuronal generation of the leech swimming movement. Science. 1978 Jun 23;200(4348):1348–1357. doi: 10.1126/science.663615. [DOI] [PubMed] [Google Scholar]
- Tank D. W., Hopfield J. J. Neural computation by concentrating information in time. Proc Natl Acad Sci U S A. 1987 Apr;84(7):1896–1900. doi: 10.1073/pnas.84.7.1896. [DOI] [PMC free article] [PubMed] [Google Scholar]
- Tesauro G. Simple neural models of classical conditioning. Biol Cybern. 1986;55(2-3):187–200. doi: 10.1007/BF00341933. [DOI] [PubMed] [Google Scholar]
- Weeks J. C. Neuronal basis of leech swimming: separation of swim initiation, pattern generation, and intersegmental coordination by selective lesions. J Neurophysiol. 1981 Apr;45(4):698–723. doi: 10.1152/jn.1981.45.4.698. [DOI] [PubMed] [Google Scholar]
- Willows A. O. Behavioral acts elicited by stimulation of single, identifiable brain cells. Science. 1967 Aug 4;157(3788):570–574. doi: 10.1126/science.157.3788.570. [DOI] [PubMed] [Google Scholar]
- Willows A. O., Hoyle G. Neuronal network triggering a fixed action pattern. Science. 1969 Dec 19;166(3912):1549–1551. doi: 10.1126/science.166.3912.1549. [DOI] [PubMed] [Google Scholar]