Abstract
Fluorescence microscopy combined with electrical conductance measurements were used to assess fusion of phospholipid vesicles with a planar bilayer. Large unilamellar vesicles (0.5-3 microns diam.) filled with the fluorescent dye, calcein, were made both with or without porin channels. Vesicle-bilayer fusion was induced by increasing the osmolarity of the solution on the side of the bilayer to which the vesicles were added. Fusion was detected optically by the fluorescent flash due to release of vesicular contents. Although both porin-containing and porin-free vesicles give the same kind of flash upon content release, the conditions necessary to induce release are very different. Only 4% of the porin-free vesicles fuse (release their contents) when subjected to 3 M urea. However, the same conditions induce 53% of the porin-containing vesicles to fuse and most of these fusions occur at a lower osmolarity ([urea] less than 400 mM). Thus channels greatly enhance fusion in this model system. A physical model based on the postulate that fusion is induced by an increase in surface tension, predicts that three conditions are necessary for fusion in this system: (a) an open channel in the vesicle membrane, (b) an osmotic gradient across the bilayer, and (c) the vesicle in contact with the planar membrane. These are the conditions that experimentally produce fusion in the model system.
Full text
PDF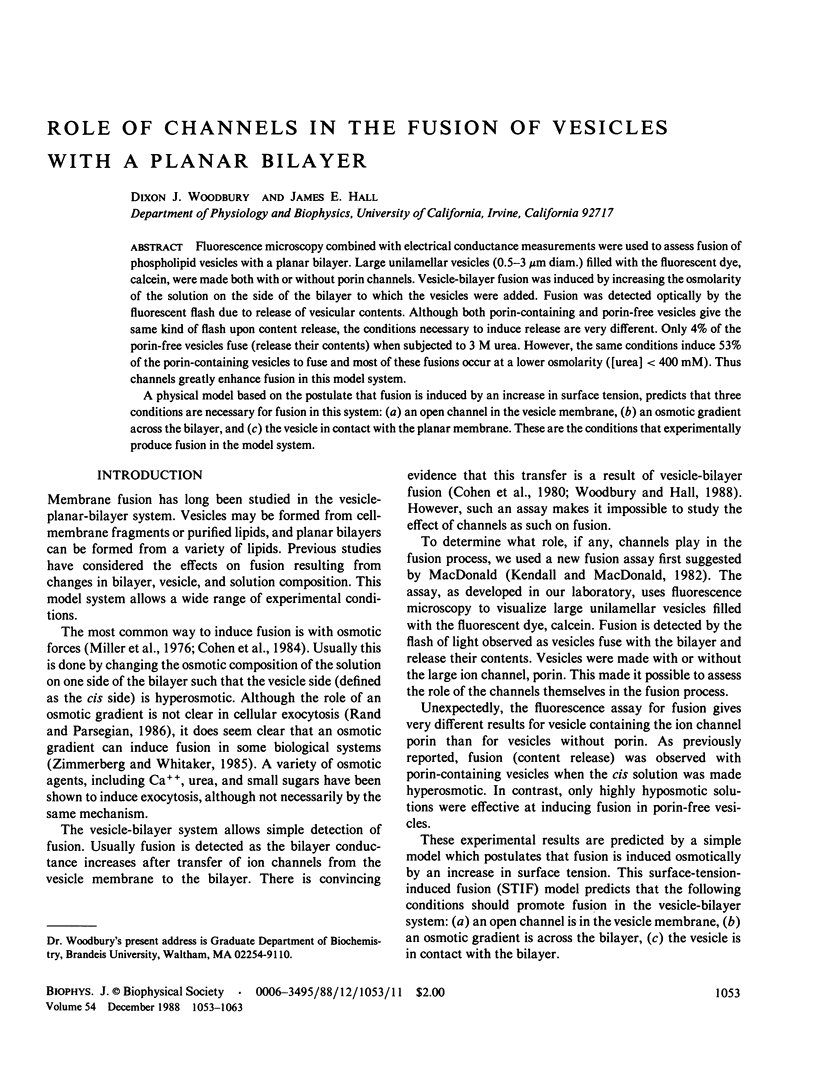
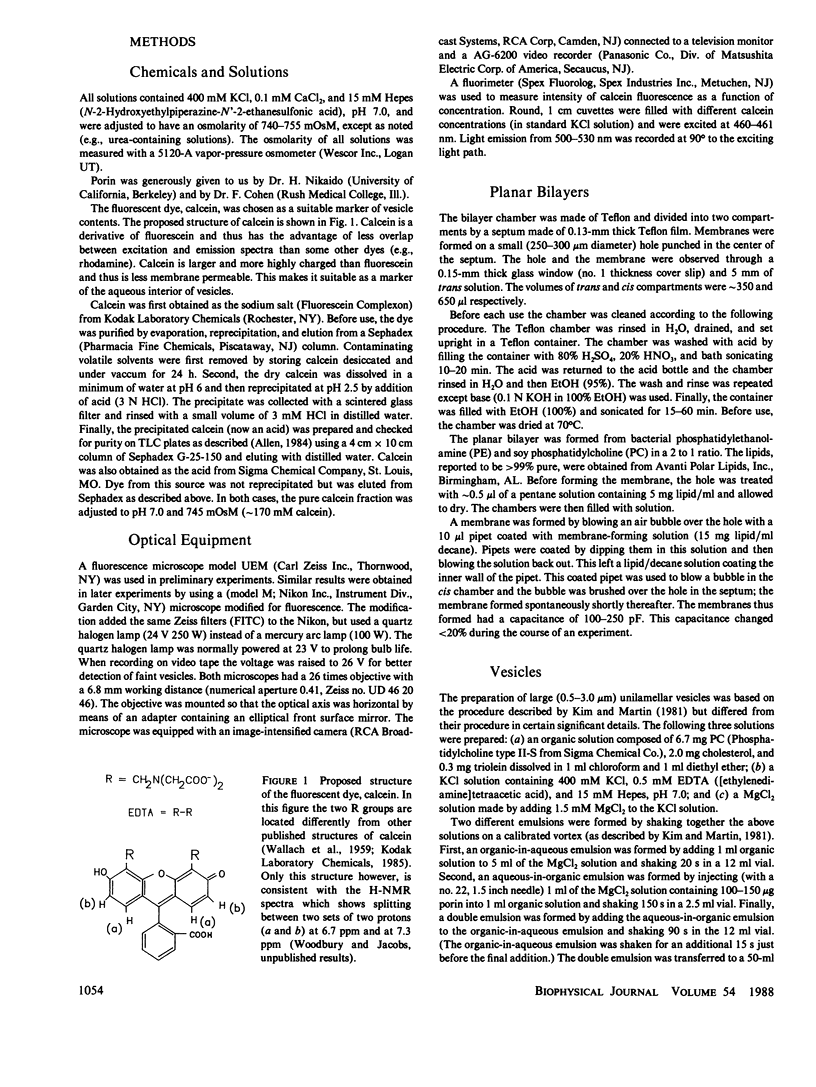
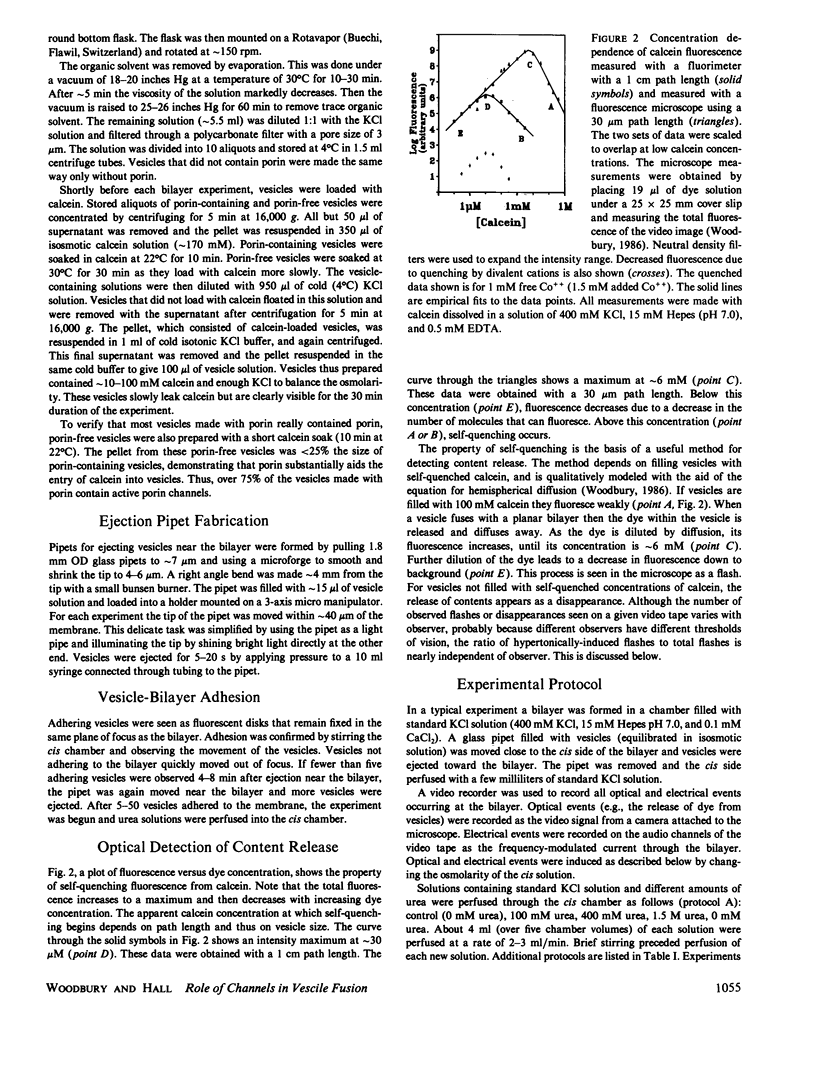
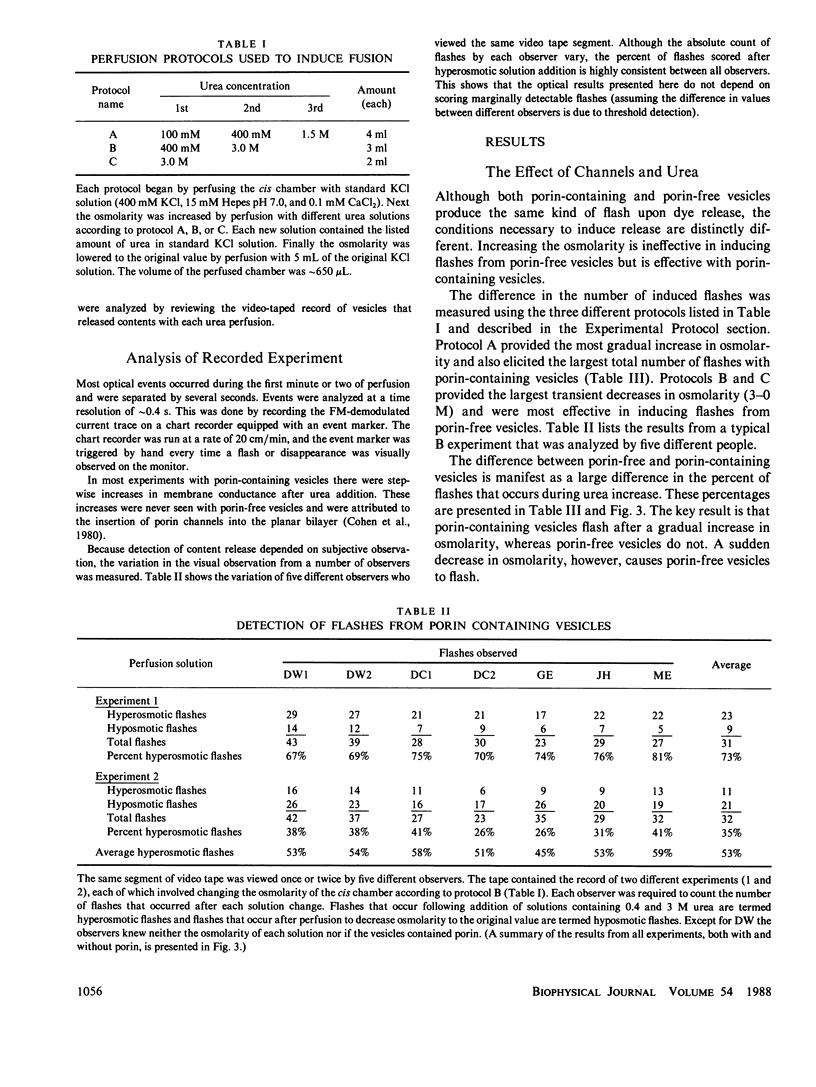
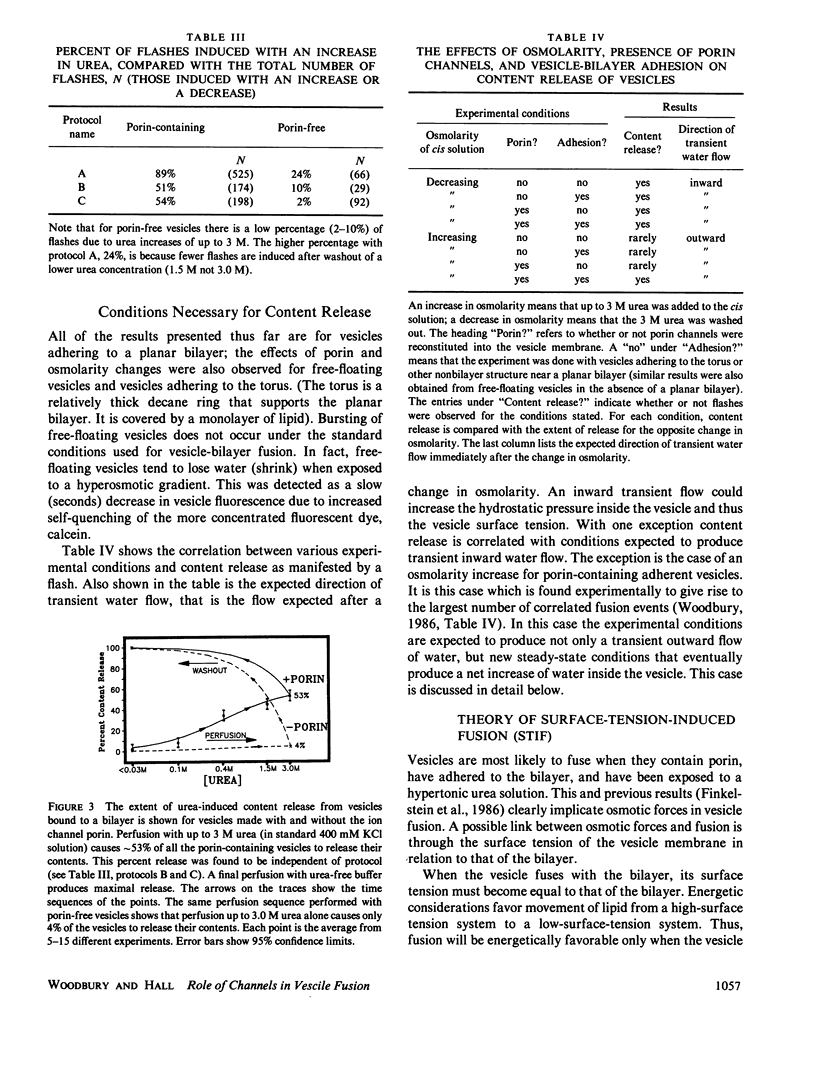
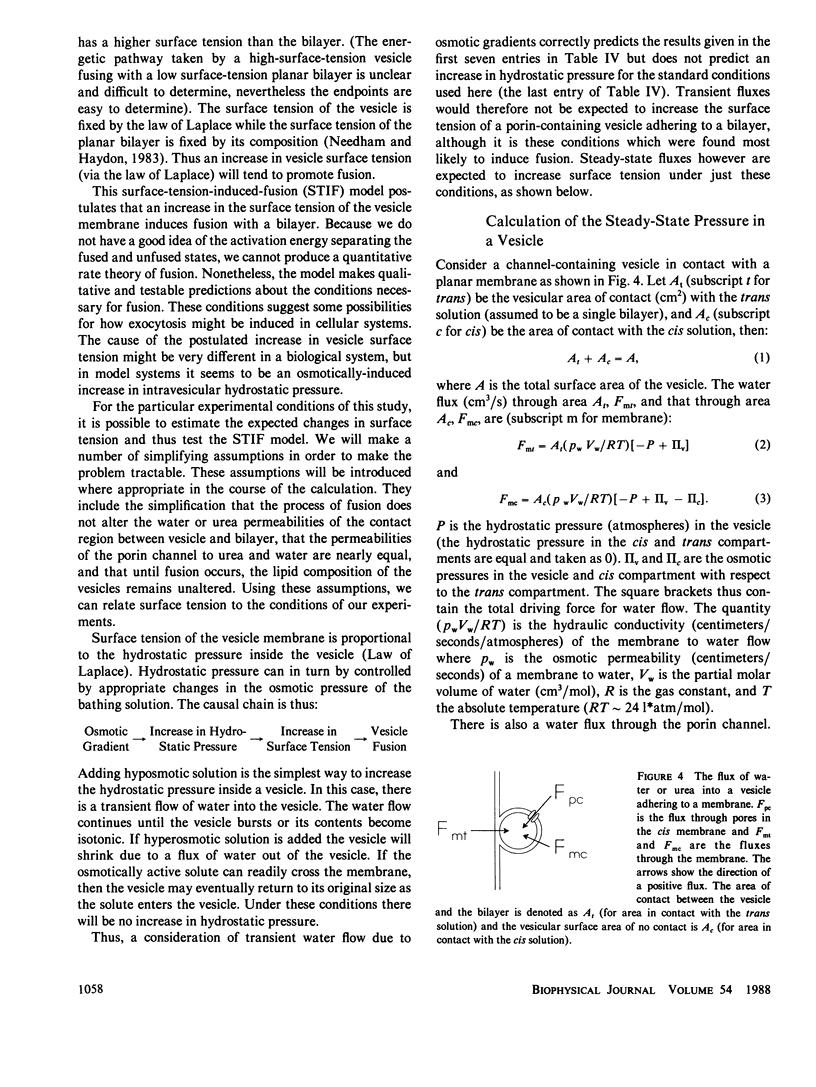
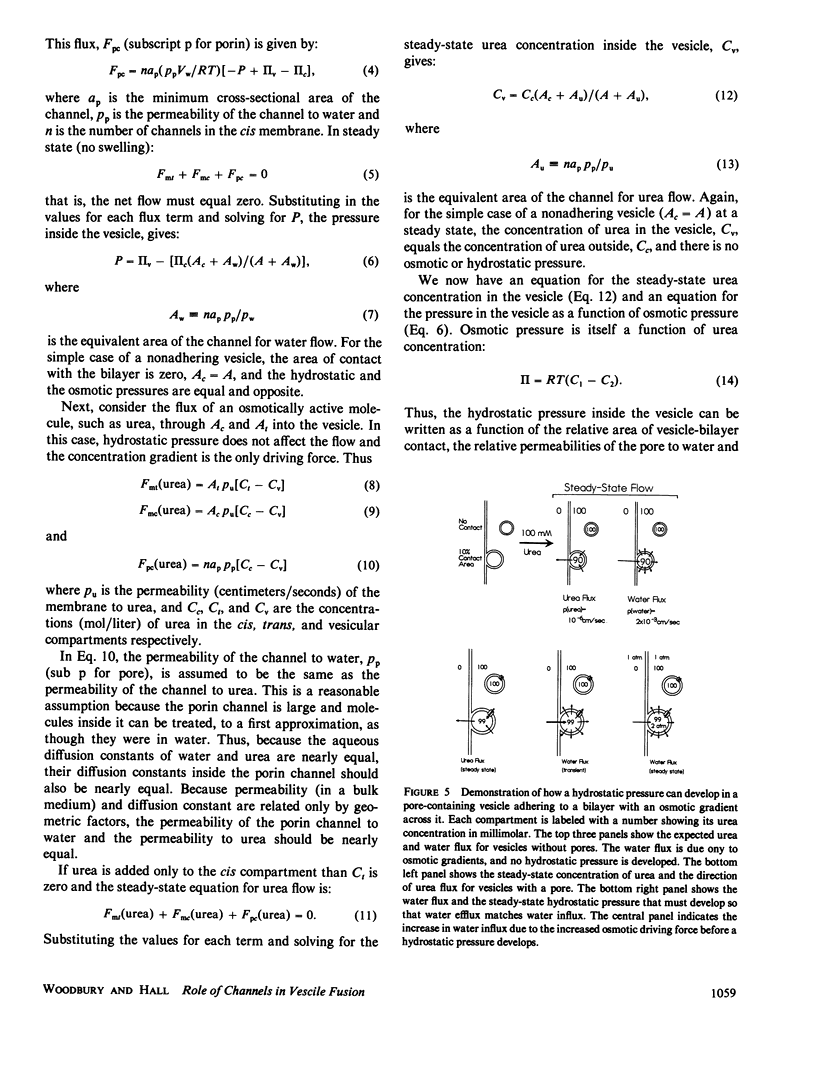
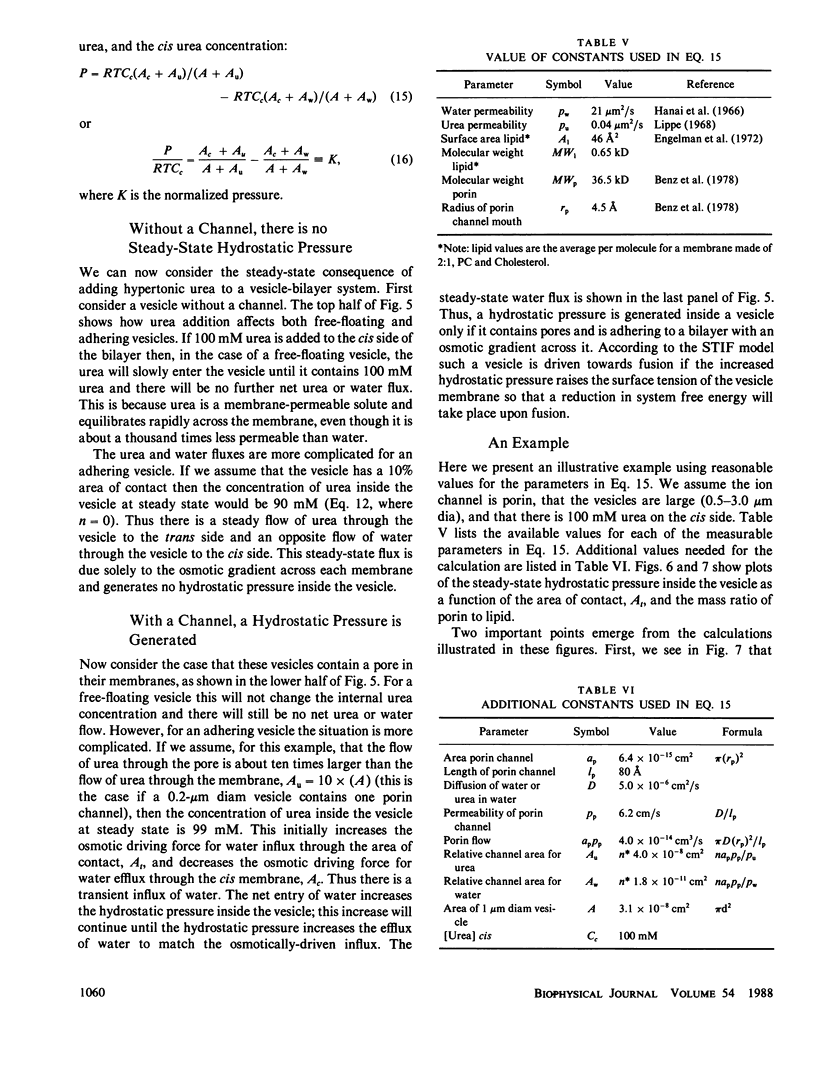
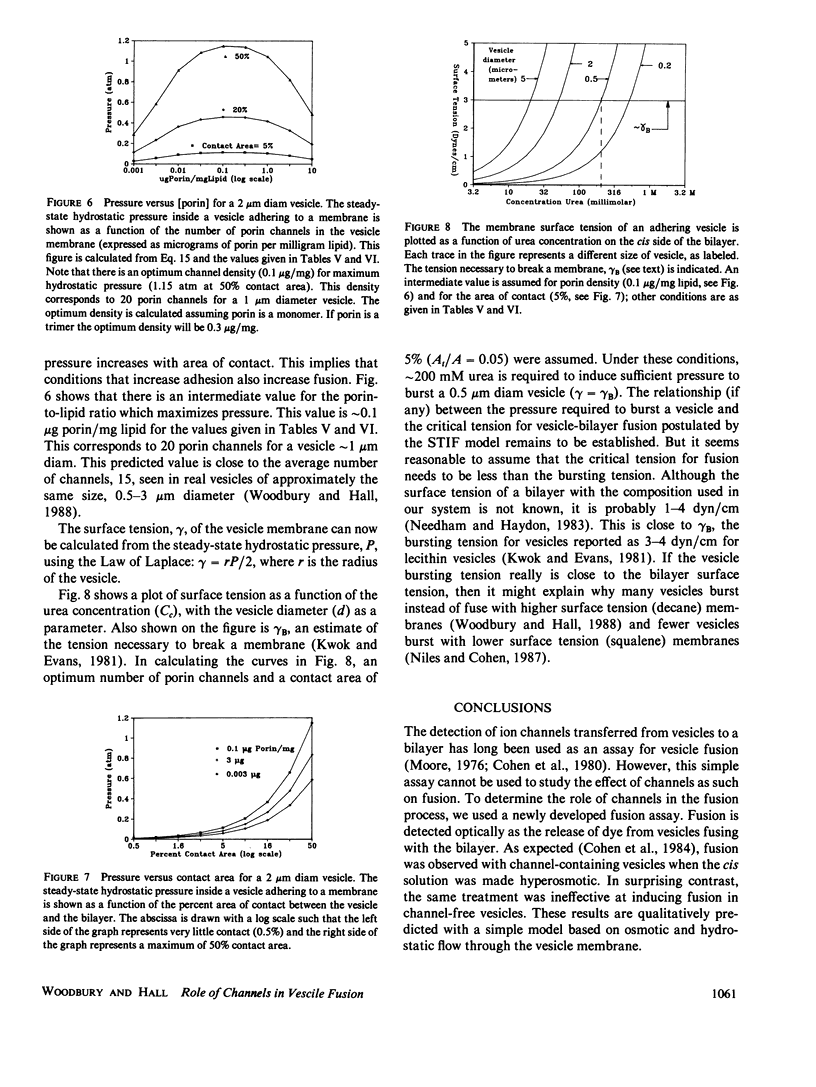
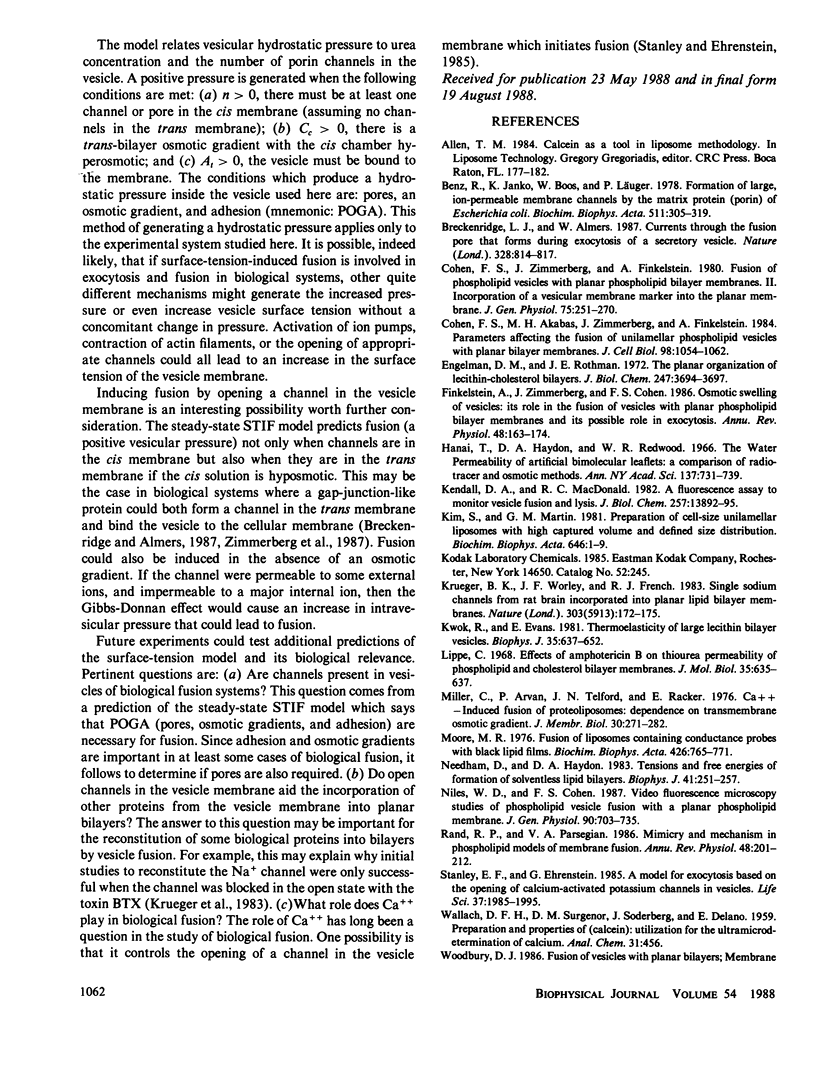
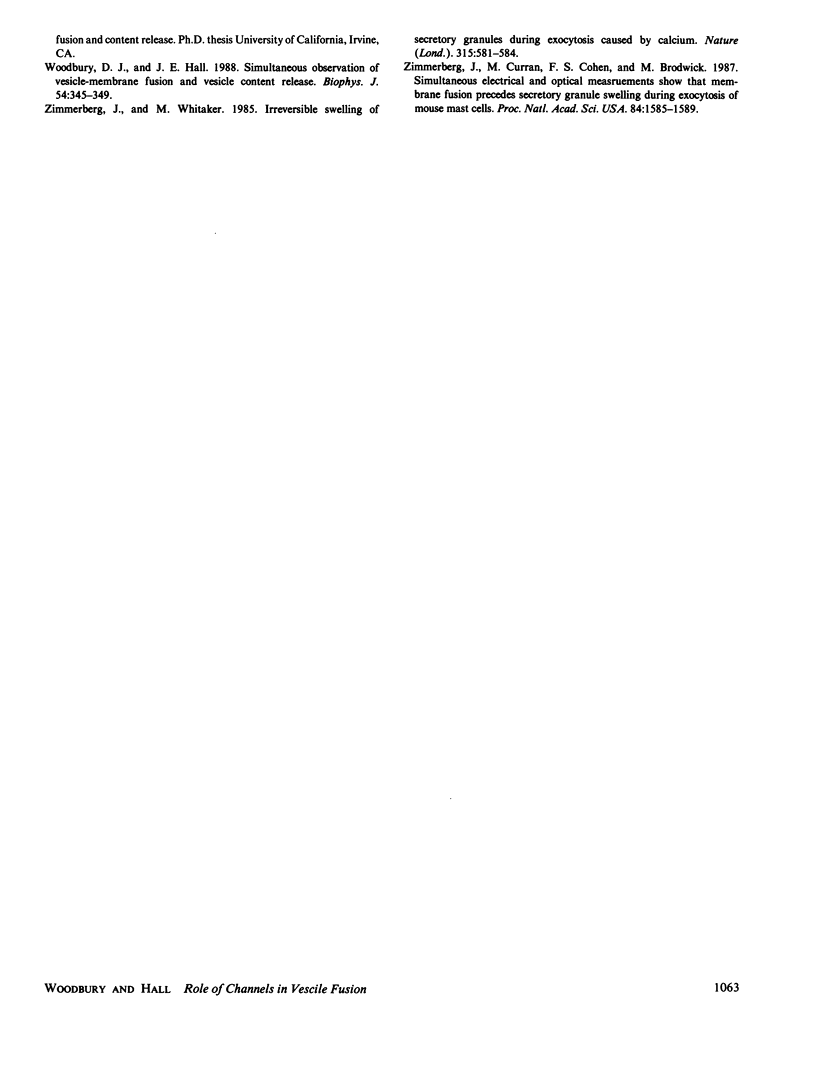
Selected References
These references are in PubMed. This may not be the complete list of references from this article.
- Benz R., Janko K., Boos W., Läuger P. Formation of large, ion-permeable membrane channels by the matrix protein (porin) of Escherichia coli. Biochim Biophys Acta. 1978 Aug 17;511(3):305–319. doi: 10.1016/0005-2736(78)90269-9. [DOI] [PubMed] [Google Scholar]
- Breckenridge L. J., Almers W. Currents through the fusion pore that forms during exocytosis of a secretory vesicle. 1987 Aug 27-Sep 2Nature. 328(6133):814–817. doi: 10.1038/328814a0. [DOI] [PubMed] [Google Scholar]
- Cohen F. S., Akabas M. H., Zimmerberg J., Finkelstein A. Parameters affecting the fusion of unilamellar phospholipid vesicles with planar bilayer membranes. J Cell Biol. 1984 Mar;98(3):1054–1062. doi: 10.1083/jcb.98.3.1054. [DOI] [PMC free article] [PubMed] [Google Scholar]
- Cohen F. S., Zimmerberg J., Finkelstein A. Fusion of phospholipid vesicles with planar phospholipid bilayer membranes. II. Incorporation of a vesicular membrane marker into the planar membrane. J Gen Physiol. 1980 Mar;75(3):251–270. doi: 10.1085/jgp.75.3.251. [DOI] [PMC free article] [PubMed] [Google Scholar]
- Engelman D. M., Rothman J. E. The planar organization of lecithin-cholesterol bilayers. J Biol Chem. 1972 Jun 10;247(11):3694–3697. [PubMed] [Google Scholar]
- Finkelstein A., Zimmerberg J., Cohen F. S. Osmotic swelling of vesicles: its role in the fusion of vesicles with planar phospholipid bilayer membranes and its possible role in exocytosis. Annu Rev Physiol. 1986;48:163–174. doi: 10.1146/annurev.ph.48.030186.001115. [DOI] [PubMed] [Google Scholar]
- Hanai T., Haydon D. A., Redwood W. R. The water permeability of artificial bimolecular leaflets: a comparison of radio-tracer and osmotic methods. Ann N Y Acad Sci. 1966 Jul 14;137(2):731–739. doi: 10.1111/j.1749-6632.1966.tb50194.x. [DOI] [PubMed] [Google Scholar]
- Kendall D. A., MacDonald R. C. A fluorescence assay to monitor vesicle fusion and lysis. J Biol Chem. 1982 Dec 10;257(23):13892–13895. [PubMed] [Google Scholar]
- Kim S., Martin G. M. Preparation of cell-size unilamellar liposomes with high captured volume and defined size distribution. Biochim Biophys Acta. 1981 Aug 6;646(1):1–9. doi: 10.1016/0005-2736(81)90264-9. [DOI] [PubMed] [Google Scholar]
- Krueger B. K., Worley J. F., 3rd, French R. J. Single sodium channels from rat brain incorporated into planar lipid bilayer membranes. Nature. 1983 May 12;303(5913):172–175. doi: 10.1038/303172a0. [DOI] [PubMed] [Google Scholar]
- Kwok R., Evans E. Thermoelasticity of large lecithin bilayer vesicles. Biophys J. 1981 Sep;35(3):637–652. doi: 10.1016/S0006-3495(81)84817-5. [DOI] [PMC free article] [PubMed] [Google Scholar]
- Lippe C. Effects of Amphotericin B on thiourea permeability of phospholipid and cholesterol bilayer membranes. J Mol Biol. 1968 Aug 14;35(3):635–637. doi: 10.1016/s0022-2836(68)80019-1. [DOI] [PubMed] [Google Scholar]
- Miller C., Arvan P., Telford J. N., Racker E. Ca++-induced fusion of proteoliposomes: dependence on transmembrane osmotic gradient. J Membr Biol. 1976;30(3):271–282. doi: 10.1007/BF01869672. [DOI] [PubMed] [Google Scholar]
- Moore M. R. Fusion of liposomes containing conductance probes with black lipid films. Biochim Biophys Acta. 1976 Apr 5;426(4):765–771. doi: 10.1016/0005-2736(76)90144-9. [DOI] [PubMed] [Google Scholar]
- Needham D., Haydon D. A. Tensions and free energies of formation of "solventless" lipid bilayers. Measurement of high contact angles. Biophys J. 1983 Mar;41(3):251–257. doi: 10.1016/S0006-3495(83)84435-X. [DOI] [PMC free article] [PubMed] [Google Scholar]
- Niles W. D., Cohen F. S. Video fluorescence microscopy studies of phospholipid vesicle fusion with a planar phospholipid membrane. Nature of membrane-membrane interactions and detection of release of contents. J Gen Physiol. 1987 Nov;90(5):703–735. doi: 10.1085/jgp.90.5.703. [DOI] [PMC free article] [PubMed] [Google Scholar]
- Rand R. P., Parsegian V. A. Mimicry and mechanism in phospholipid models of membrane fusion. Annu Rev Physiol. 1986;48:201–212. doi: 10.1146/annurev.ph.48.030186.001221. [DOI] [PubMed] [Google Scholar]
- Stanley E. F., Ehrenstein G. A model for exocytosis based on the opening of calcium-activated potassium channels in vesicles. Life Sci. 1985 Nov 25;37(21):1985–1995. doi: 10.1016/0024-3205(85)90029-3. [DOI] [PubMed] [Google Scholar]
- Woodbury D. J., Hall J. E. Vesicle-membrane fusion. Observation of simultaneous membrane incorporation and content release. Biophys J. 1988 Aug;54(2):345–349. doi: 10.1016/S0006-3495(88)82965-5. [DOI] [PMC free article] [PubMed] [Google Scholar]
- Zimmerberg J., Curran M., Cohen F. S., Brodwick M. Simultaneous electrical and optical measurements show that membrane fusion precedes secretory granule swelling during exocytosis of beige mouse mast cells. Proc Natl Acad Sci U S A. 1987 Mar;84(6):1585–1589. doi: 10.1073/pnas.84.6.1585. [DOI] [PMC free article] [PubMed] [Google Scholar]
- Zimmerberg J., Whitaker M. Irreversible swelling of secretory granules during exocytosis caused by calcium. Nature. 1985 Jun 13;315(6020):581–584. doi: 10.1038/315581a0. [DOI] [PubMed] [Google Scholar]