Abstract
Many biological and biotechnological systems involve the diffusion of macromolecules through complicated macroporous (pore size on the order of 10-100 microns) environments. In this report, we present and evaluate an experimental system for measuring the rate of protein transport in an inert, macroporous membrane. For this particular membrane system, the microgeometry was characterized in terms of distribution of pore size, position, and orientation. Although the rate of protein desorption was much less than expected based on continuum diffusion models, we demonstrate that the measured transport rates are consistent with diffusion of protein in a complex, interconnected network of water-filled pores. The porous systems exhibit transitional behavior in quantitative agreement with the behavior of percolation lattices (mean square error 7%, n = 29). Predictive mathematical models of the diffusion process were developed: these models used percolation concepts to describe pore topology, continuum models of diffusion/dissolution to describe protein movement at each single pore, and measured pore size distributions. Effective diffusion coefficients for protein transport in aqueous, constricted macropores were predicted by this technique. Predicted diffusion coefficients, based on measured and derived microstructural parameters, agree with experimentally measured diffusion coefficients within a factor of 2. This approach may be useful in the design of porous polymer systems for biological applications and for evaluating other biological systems where conduction of mass, heat, momentum, or charge occurs in a heterogeneous environment.
Full text
PDF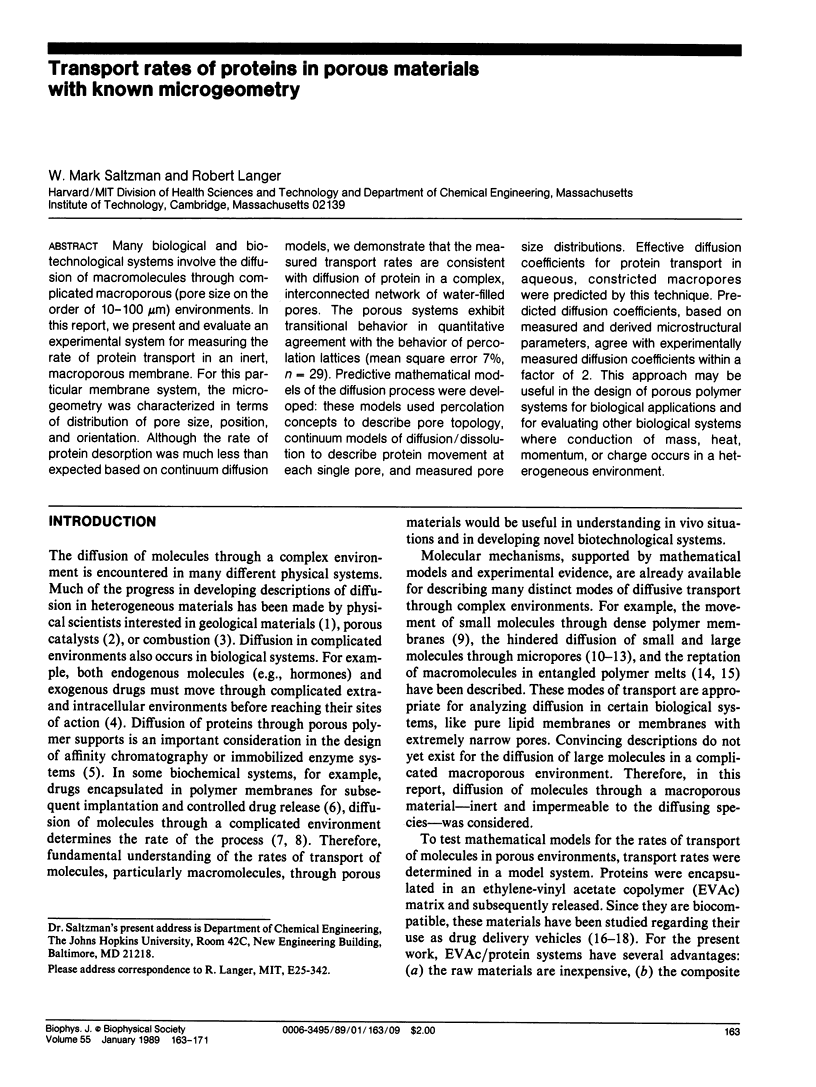
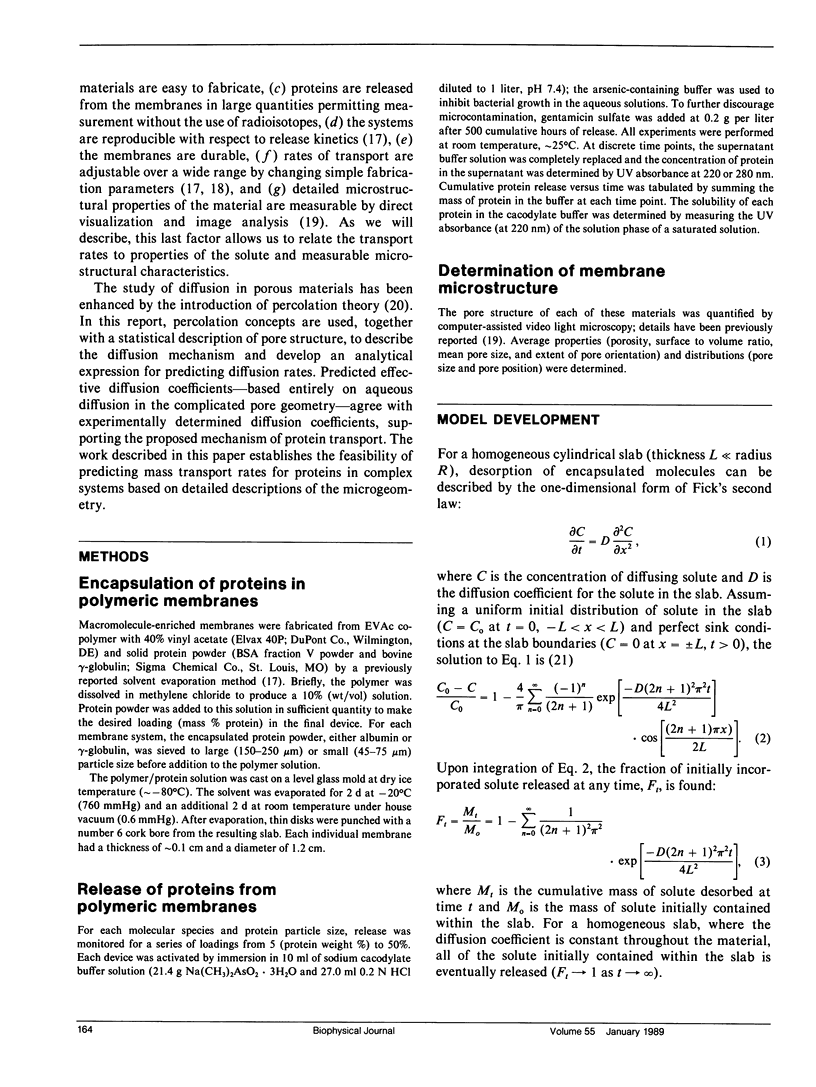
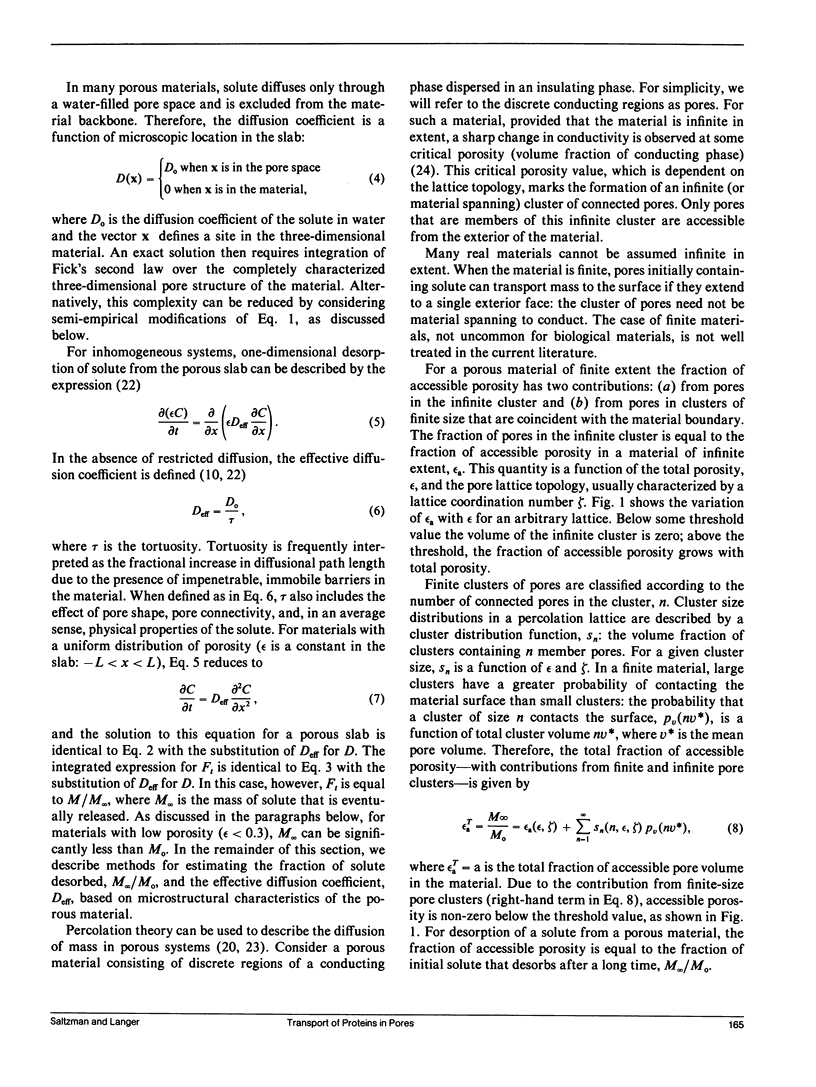
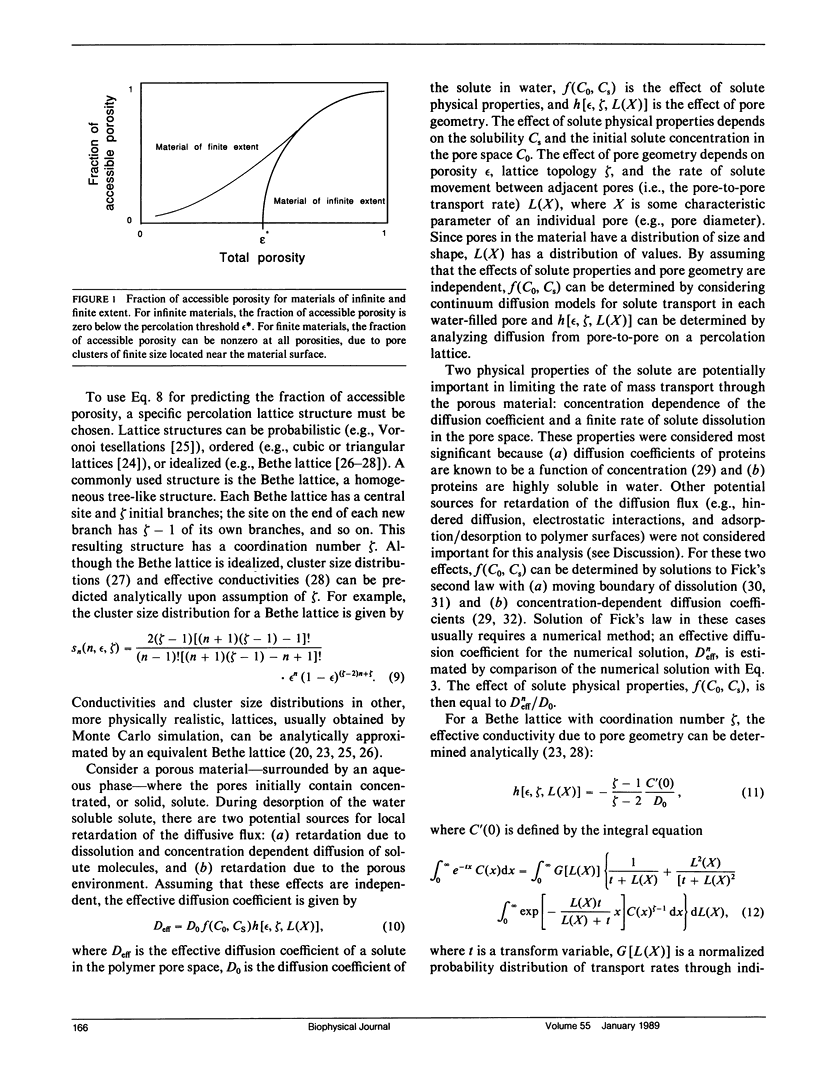
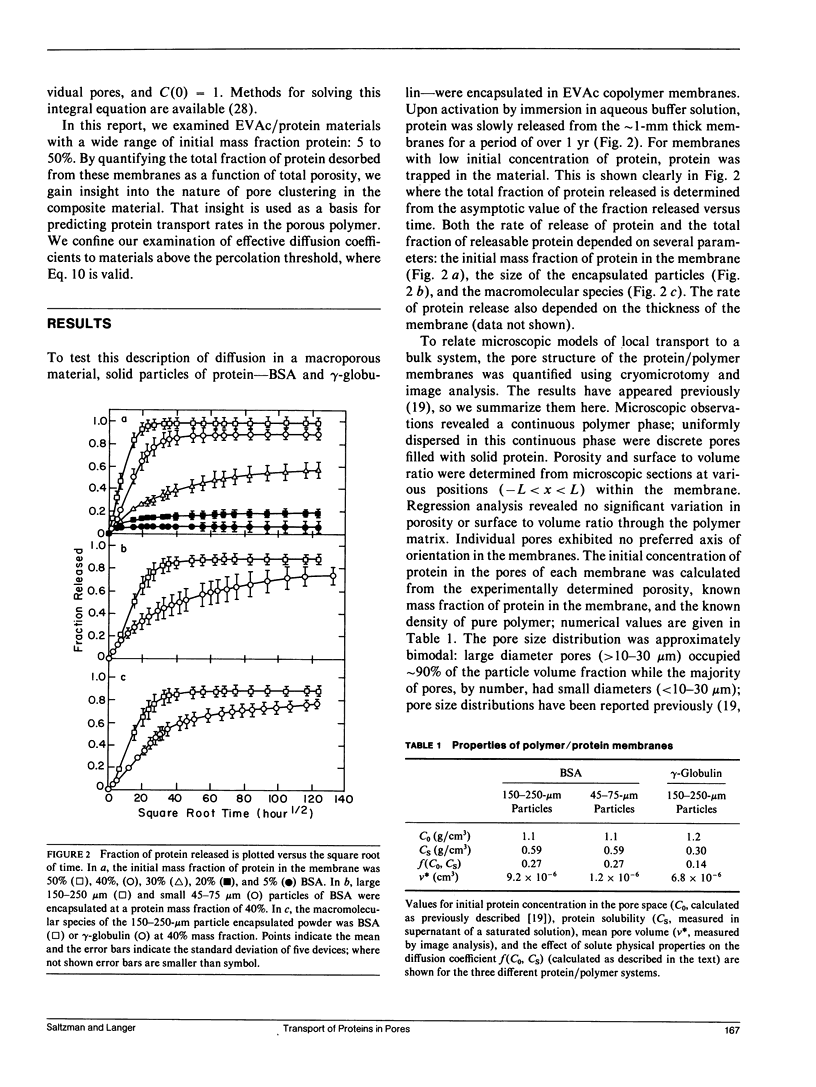
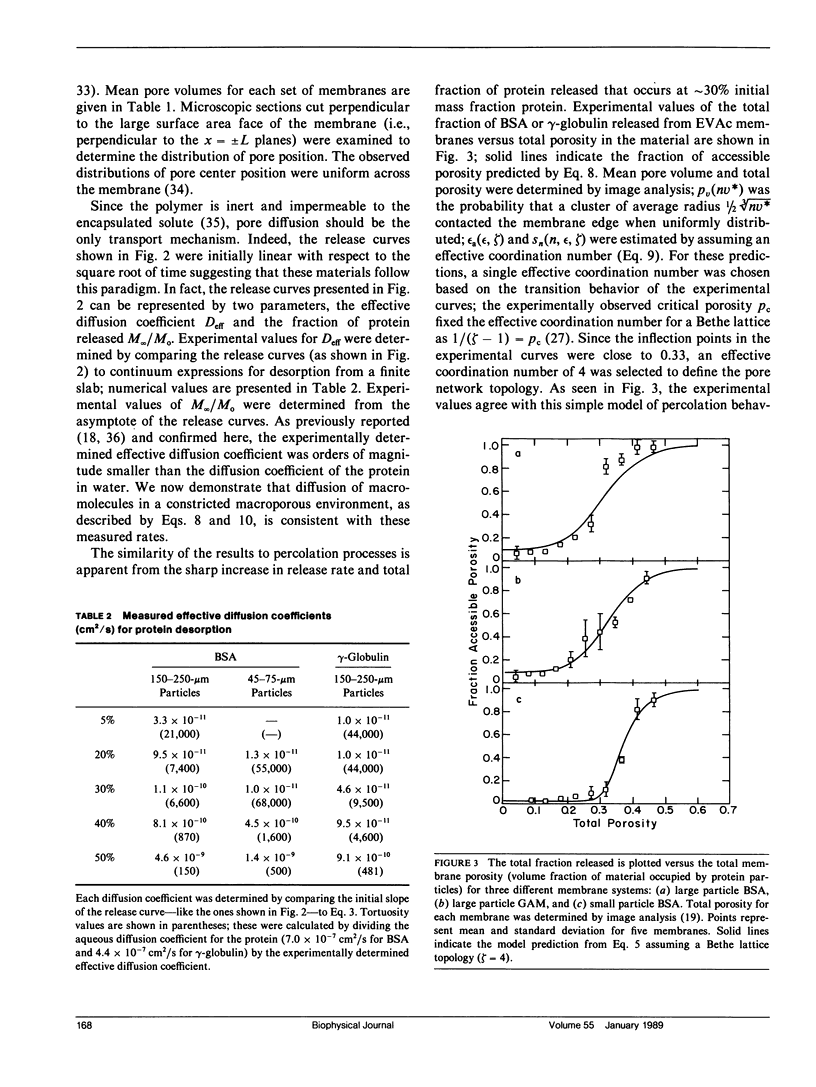
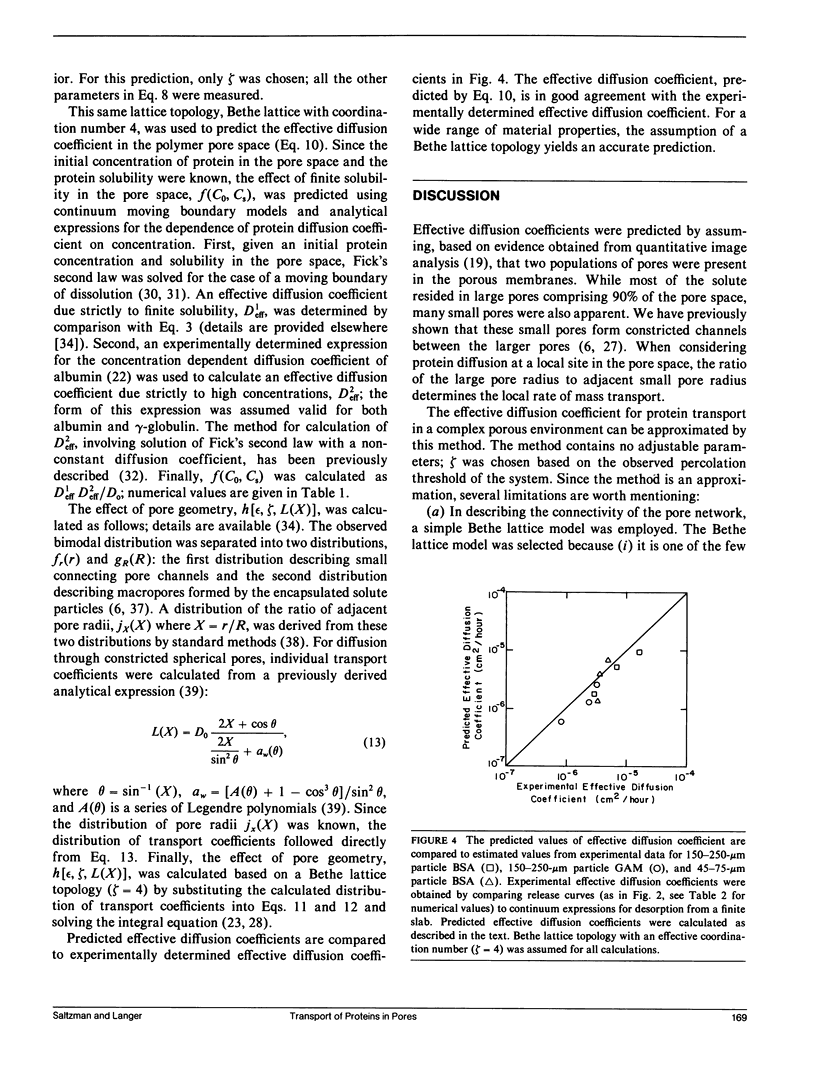
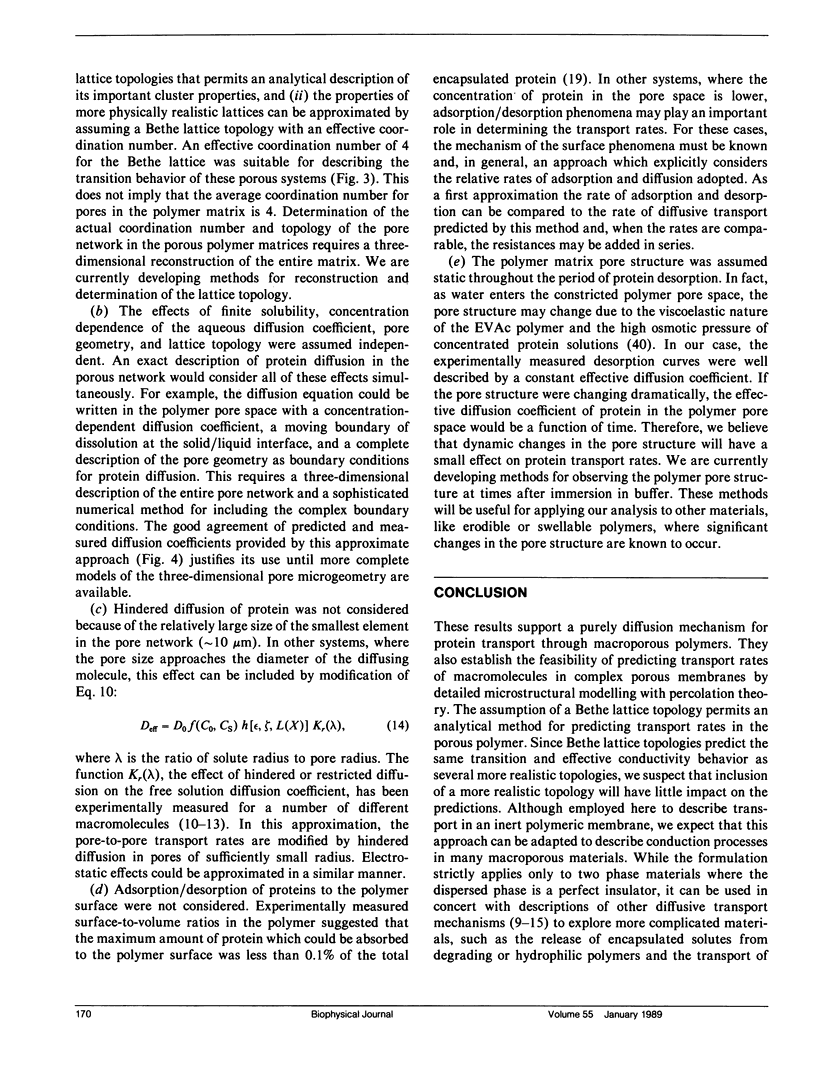
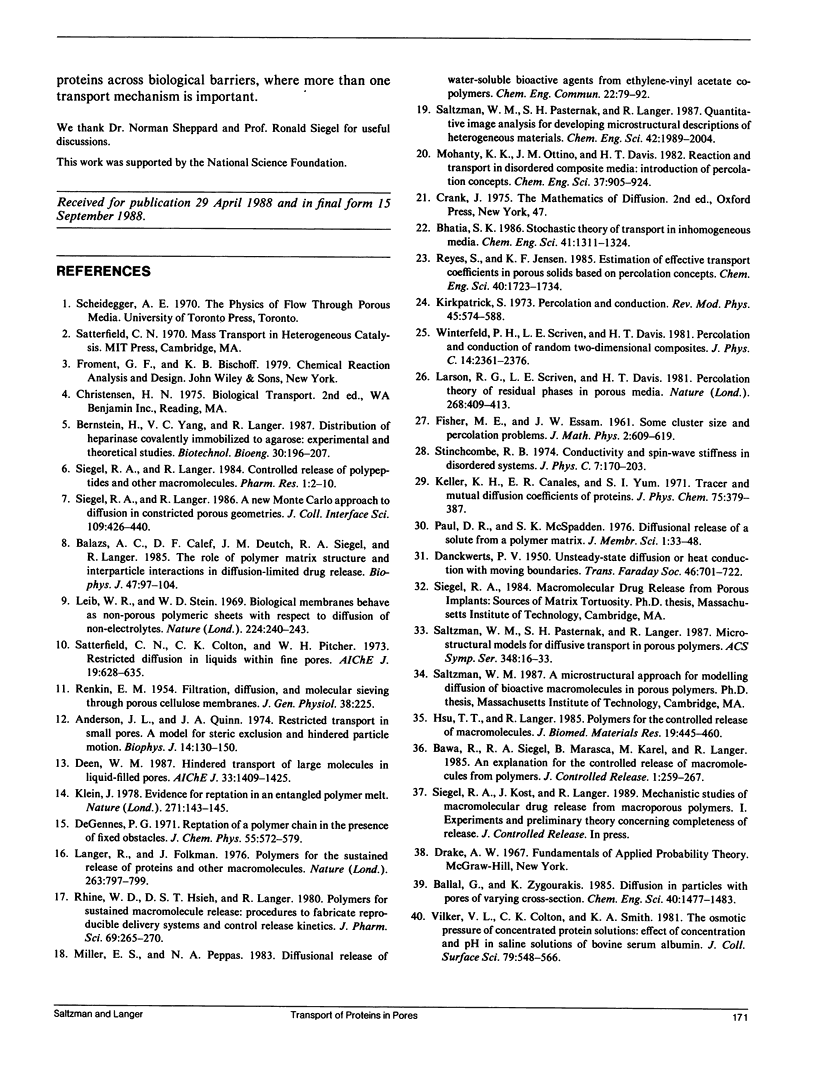
Selected References
These references are in PubMed. This may not be the complete list of references from this article.
- Anderson J. L., Quinn J. A. Restricted transport in small pores. A model for steric exclusion and hindered particle motion. Biophys J. 1974 Feb;14(2):130–150. doi: 10.1016/S0006-3495(74)70005-4. [DOI] [PMC free article] [PubMed] [Google Scholar]
- Balazs A. C., Calef D. F., Deutch J. M., Siegel R. A., Langer R. The role of polymer matrix structure and interparticle interactions in diffusion-limited drug release. Biophys J. 1985 Jan;47(1):97–104. doi: 10.1016/S0006-3495(85)83881-9. [DOI] [PMC free article] [PubMed] [Google Scholar]
- Hsu T. T., Langer R. Polymers for the controlled release of macromolecules: effect of molecular weight of ethylene-vinyl acetate copolymer. J Biomed Mater Res. 1985 Apr;19(4):445–460. doi: 10.1002/jbm.820190409. [DOI] [PubMed] [Google Scholar]
- Langer R., Folkman J. Polymers for the sustained release of proteins and other macromolecules. Nature. 1976 Oct 28;263(5580):797–800. doi: 10.1038/263797a0. [DOI] [PubMed] [Google Scholar]
- Lieb W. R., Stein W. D. Biological membranes behave as non-porous polymeric sheets with respect to the diffusion of non-electrolytes. Nature. 1969 Oct 18;224(5216):240–243. doi: 10.1038/224240a0. [DOI] [PubMed] [Google Scholar]
- RENKIN E. M. Filtration, diffusion, and molecular sieving through porous cellulose membranes. J Gen Physiol. 1954 Nov 20;38(2):225–243. [PMC free article] [PubMed] [Google Scholar]
- Rhine W. D., Hsieh D. S., Langer R. Polymers for sustained macromolecule release: procedures to fabricate reproducible delivery systems and control release kinetics. J Pharm Sci. 1980 May;69(3):265–270. doi: 10.1002/jps.2600690305. [DOI] [PubMed] [Google Scholar]