Abstract
Streaming potentials arising across large-conductance Ca2+-activated K+ channels incorporated into planar lipid bilayers were measured. Ca2+-activated channels obtained either from skeletal muscle or from smooth muscle membranes were used. Streaming potentials were extracted from the current-voltage relationship for the open channel obtained in the presence of an osmotic gradient. The osmotic gradient was established by adding glucose to one side of the membrane. At 300 mM KCl, the average streaming potential was 0.72 mV/osmol per kg for t-tubule channels and 0.83 mV/osmol per kg for smooth muscle channels. Streaming potential values depend on KCl concentration, they decrease as KCl concentration increases, and the value obtained by extrapolation to zero KCl concentration is 0.85 mV/osmol per kg. Assuming that water and ions cannot pass each other, at least in a region of the channel, the streaming potential values obtained indicate that this region contains a minimum of two and a maximum of four water molecules. It is concluded that the channel has a narrow region with a length of 0.6-1.2 nm.
Full text
PDF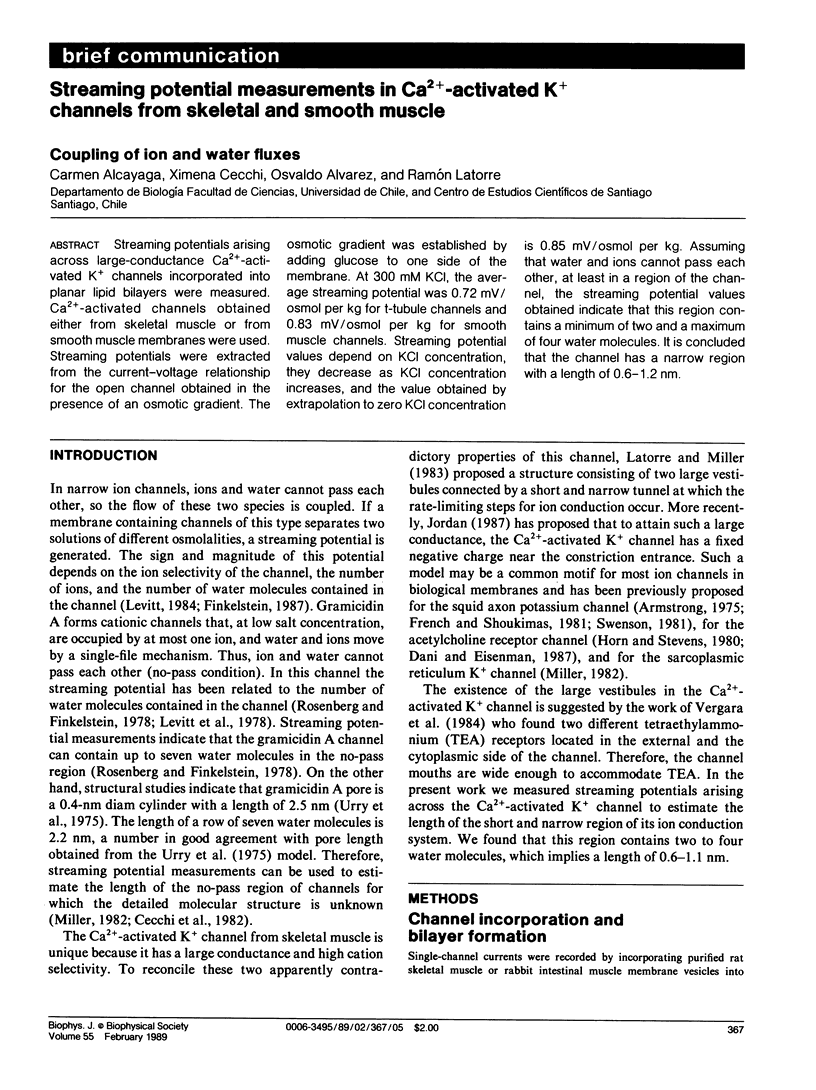
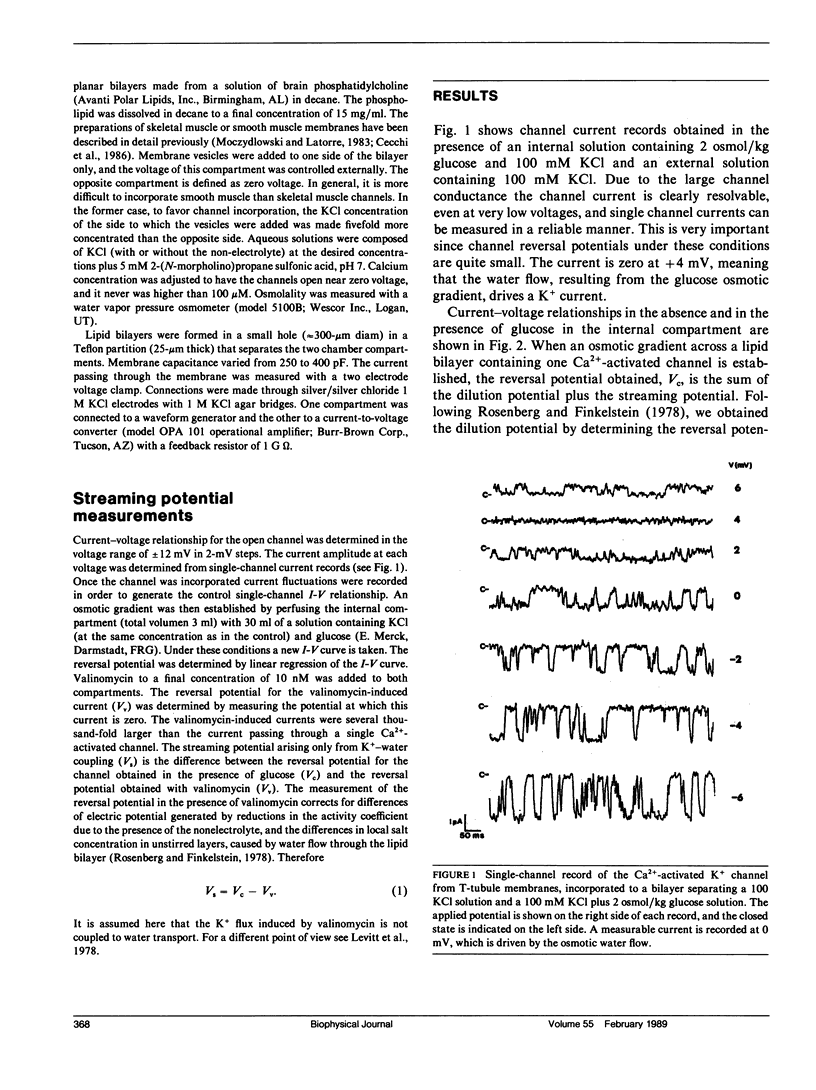
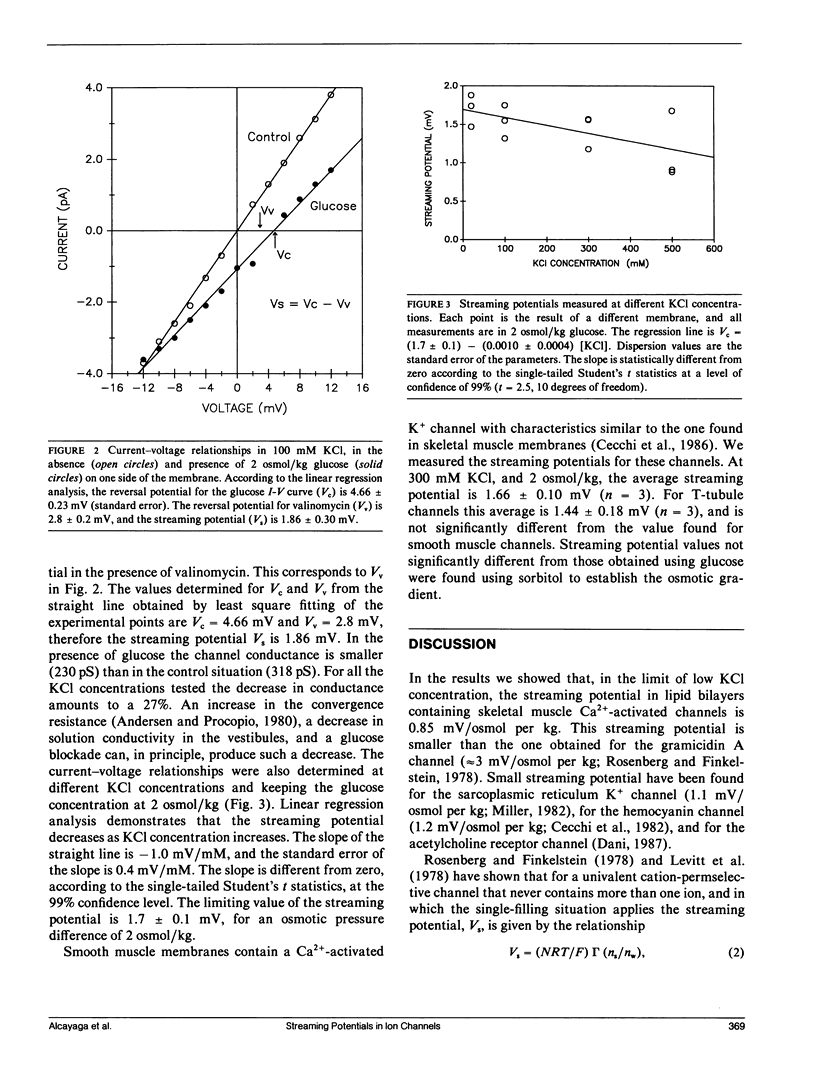
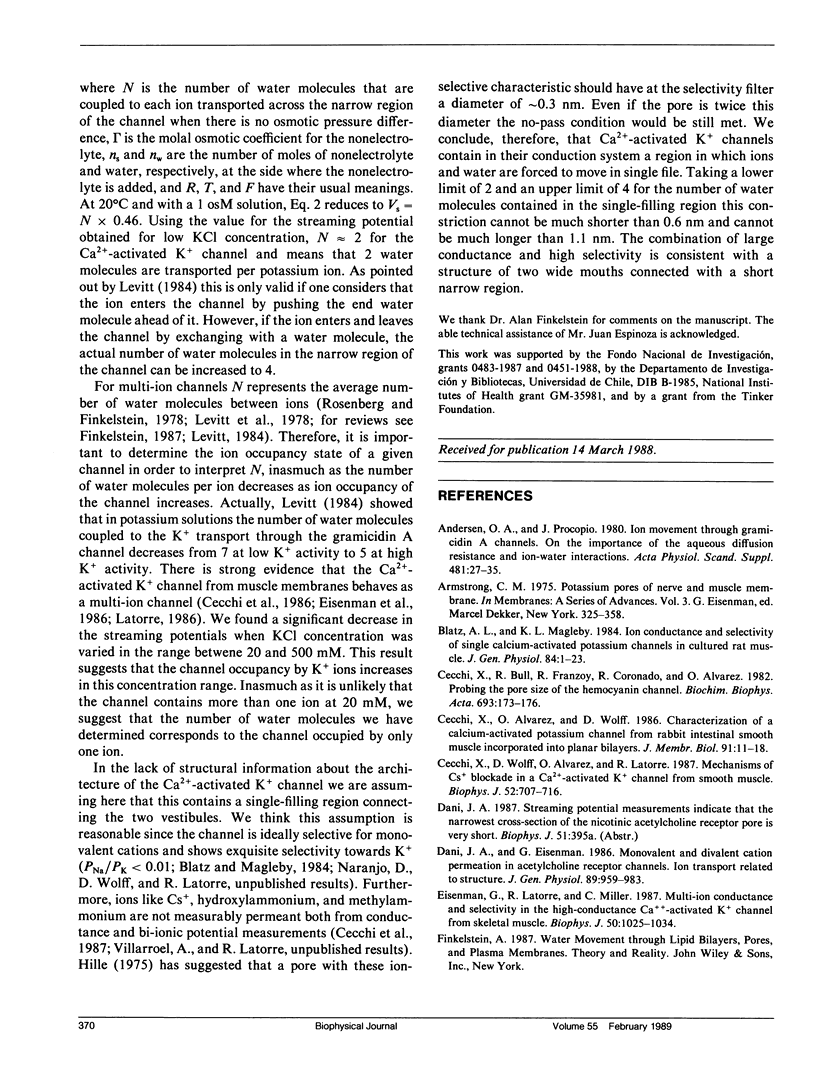
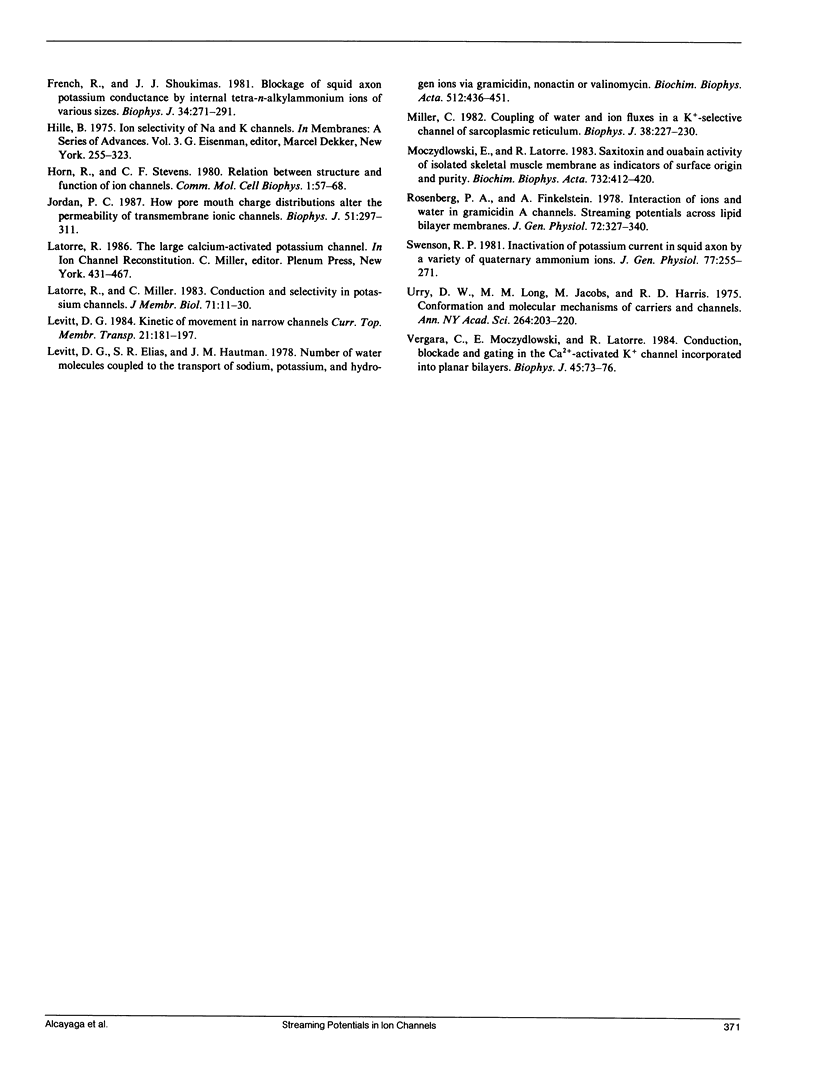
Selected References
These references are in PubMed. This may not be the complete list of references from this article.
- Andersen O. S., Procopio J. Ion movement through gramicidin A channels. On the importance of the aqueous diffusion resistance and ion-water interactions. Acta Physiol Scand Suppl. 1980;481:27–35. [PubMed] [Google Scholar]
- Armstrong C. M. Potassium pores of nerve and muscle membranes. Membranes. 1975;3:325–358. [PubMed] [Google Scholar]
- Blatz A. L., Magleby K. L. Ion conductance and selectivity of single calcium-activated potassium channels in cultured rat muscle. J Gen Physiol. 1984 Jul;84(1):1–23. doi: 10.1085/jgp.84.1.1. [DOI] [PMC free article] [PubMed] [Google Scholar]
- Cecchi X., Alvarez O., Wolff D. Characterization of a calcium-activated potassium channel from rabbit intestinal smooth muscle incorporated into planar bilayers. J Membr Biol. 1986;91(1):11–18. doi: 10.1007/BF01870210. [DOI] [PubMed] [Google Scholar]
- Cecchi X., Bull R., Franzoy R., Coronado R., Alvarez O. Probing the pore size of the hemocyanin channel. Biochim Biophys Acta. 1982 Dec 8;693(1):173–176. doi: 10.1016/0005-2736(82)90484-9. [DOI] [PubMed] [Google Scholar]
- Cecchi X., Wolff D., Alvarez O., Latorre R. Mechanisms of Cs+ blockade in a Ca2+-activated K+ channel from smooth muscle. Biophys J. 1987 Nov;52(5):707–716. doi: 10.1016/S0006-3495(87)83265-4. [DOI] [PMC free article] [PubMed] [Google Scholar]
- Dani J. A., Eisenman G. Monovalent and divalent cation permeation in acetylcholine receptor channels. Ion transport related to structure. J Gen Physiol. 1987 Jun;89(6):959–983. doi: 10.1085/jgp.89.6.959. [DOI] [PMC free article] [PubMed] [Google Scholar]
- Eisenman G., Latorre R., Miller C. Multi-ion conduction and selectivity in the high-conductance Ca++-activated K+ channel from skeletal muscle. Biophys J. 1986 Dec;50(6):1025–1034. doi: 10.1016/S0006-3495(86)83546-9. [DOI] [PMC free article] [PubMed] [Google Scholar]
- French R. J., Shoukimas J. J. Blockage of squid axon potassium conductance by internal tetra-N-alkylammonium ions of various sizes. Biophys J. 1981 May;34(2):271–291. doi: 10.1016/S0006-3495(81)84849-7. [DOI] [PMC free article] [PubMed] [Google Scholar]
- Jordan P. C. How pore mouth charge distributions alter the permeability of transmembrane ionic channels. Biophys J. 1987 Feb;51(2):297–311. doi: 10.1016/S0006-3495(87)83336-2. [DOI] [PMC free article] [PubMed] [Google Scholar]
- Latorre R., Miller C. Conduction and selectivity in potassium channels. J Membr Biol. 1983;71(1-2):11–30. doi: 10.1007/BF01870671. [DOI] [PubMed] [Google Scholar]
- Levitt D. G., Elias S. R., Hautman J. M. Number of water molecules coupled to the transport of sodium, potassium and hydrogen ions via gramicidin, nonactin or valinomycin. Biochim Biophys Acta. 1978 Sep 22;512(2):436–451. doi: 10.1016/0005-2736(78)90266-3. [DOI] [PubMed] [Google Scholar]
- Miller C. Coupling of water and ion fluxes in a K+-selective channel of sarcoplasmic reticulum. Biophys J. 1982 Jun;38(3):227–230. doi: 10.1016/S0006-3495(82)84552-9. [DOI] [PMC free article] [PubMed] [Google Scholar]
- Moczydlowski E. G., Latorre R. Saxitoxin and ouabain binding activity of isolated skeletal muscle membrane as indicators of surface origin and purity. Biochim Biophys Acta. 1983 Jul 27;732(2):412–420. doi: 10.1016/0005-2736(83)90058-5. [DOI] [PubMed] [Google Scholar]
- Rosenberg P. A., Finkelstein A. Interaction of ions and water in gramicidin A channels: streaming potentials across lipid bilayer membranes. J Gen Physiol. 1978 Sep;72(3):327–340. doi: 10.1085/jgp.72.3.327. [DOI] [PMC free article] [PubMed] [Google Scholar]
- Swenson R. P., Jr Inactivation of potassium current in squid axon by a variety of quaternary ammonium ions. J Gen Physiol. 1981 Mar;77(3):255–271. doi: 10.1085/jgp.77.3.255. [DOI] [PMC free article] [PubMed] [Google Scholar]
- Urry D. W., Long M. M., Jacobs M., Harris R. D. Conformation and molecular mechanisms of carriers and channels. Ann N Y Acad Sci. 1975 Dec 30;264:203–220. doi: 10.1111/j.1749-6632.1975.tb31484.x. [DOI] [PubMed] [Google Scholar]
- Vergara C., Moczydlowski E., Latorre R. Conduction, Blockade and Gating in a Ca -activated K Channel Incorporated into Planar Lipid Bilayers. Biophys J. 1984 Jan;45(1):73–76. doi: 10.1016/S0006-3495(84)84114-4. [DOI] [PMC free article] [PubMed] [Google Scholar]