Abstract
A micromanipulation method is used to determine the adhesive energy density (gamma) between pairs of cytotoxic T cells (F1) and their target cells (JY: HLA-A2-B7-DR4,W6). gamma is defined as the energy per unit area that must be supplied to reduce the region of contact between a conjugated cell pair. Our analysis of the data indicates that the force applied by the micropipette on the cell is not uniformly distributed throughout the contact region as we had previously assumed (Sung, K. L. P., L. A. Sung, M. Crimmins, S. J. Burakoff, and S. Chien. 1986. Science (Wash. DC). 234: 1405-1408), but acts only at the edges of the contact region. We show that gamma is not constant during peeling but increases with decreasing contact area of the conjugated cell pairs F1-JY, F1-F1, and JY-JY in contrast to the constancy of gamma for typical engineering adhesives. This finding supports the notion that the cross-linking protein molecules slide towards the conjugated area across the leading edge of the separation while remaining attached to both cells. Our mathematical analysis shows that the elastic energy stored in the cross-links by the membrane tensions balances the diffusive forces that act against cross-bridge migration. The binding affinity between F1-JY is found to be approximately 15-20 times larger than the corresponding affinity for F1-F1. The number of binding sites of F1 for attachment to JY is approximately the same for binding F1 to another F1 and vary between 10(5) and 10(6).
Full text
PDF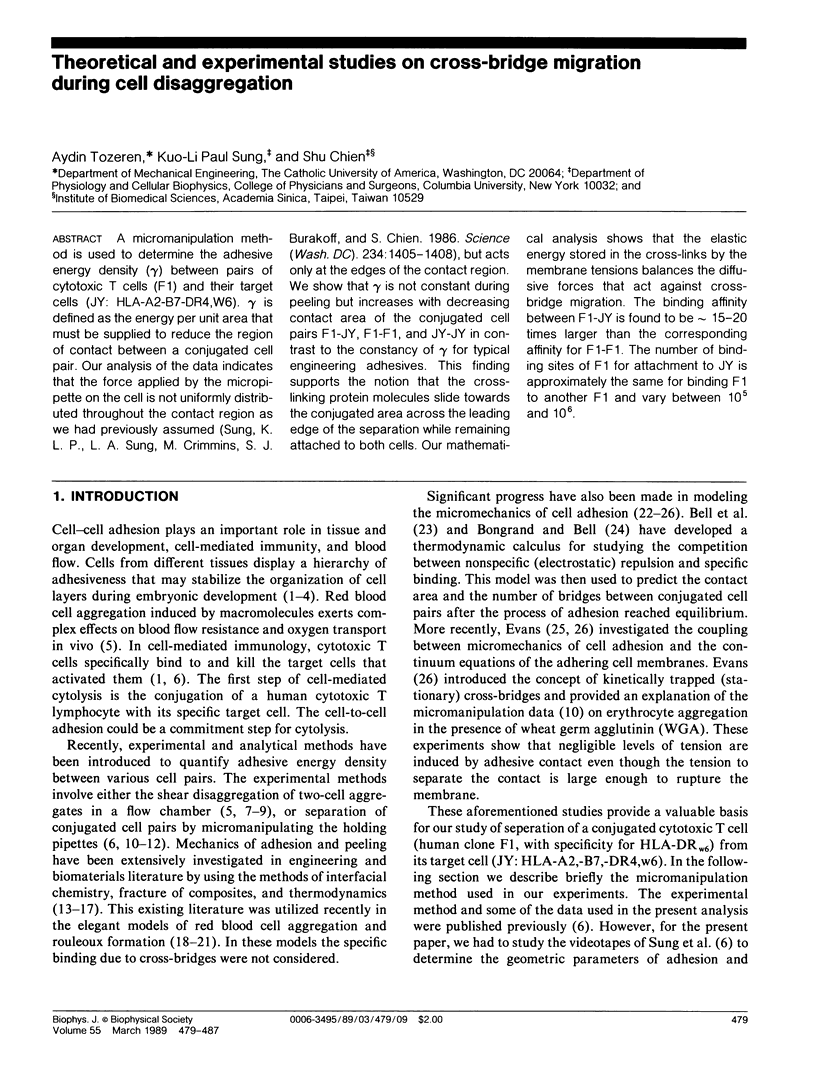
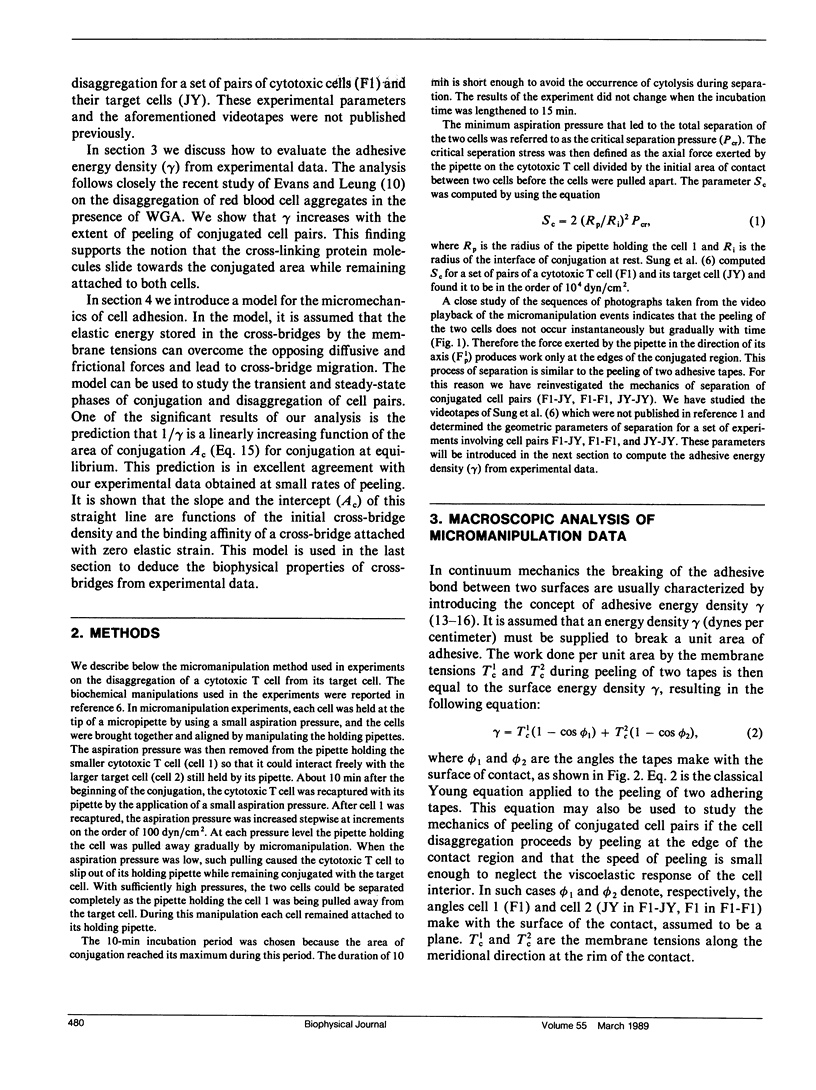
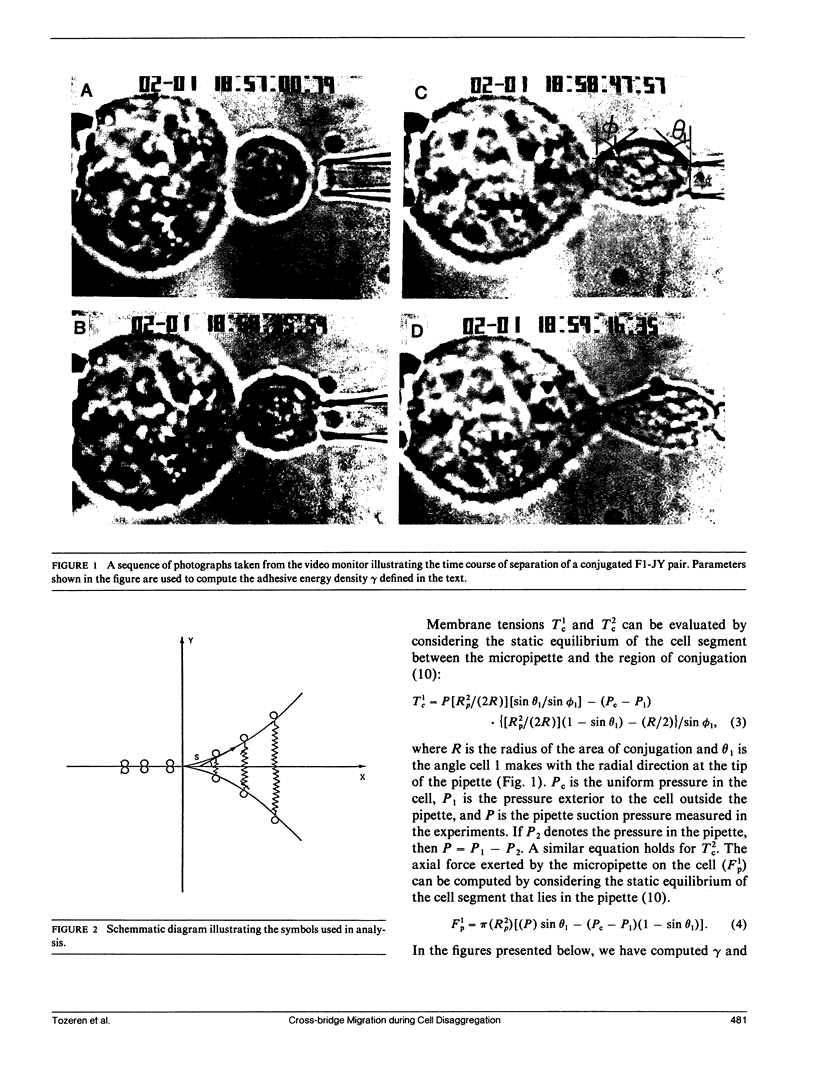
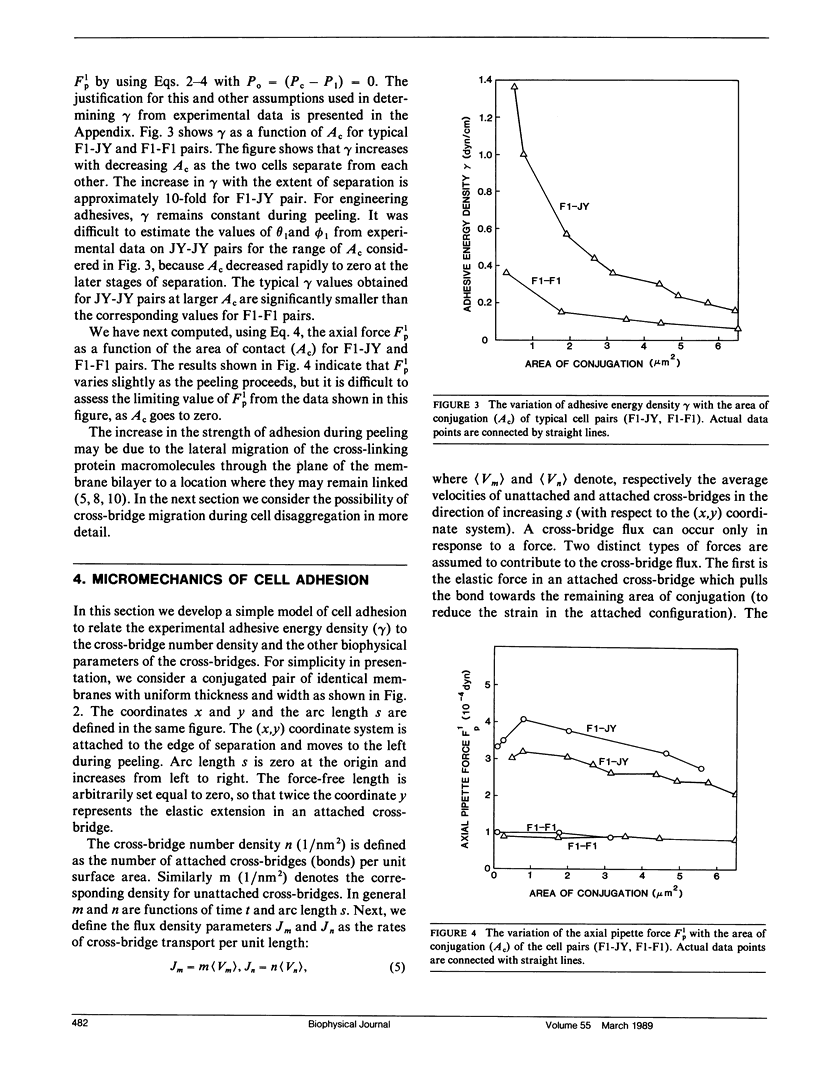
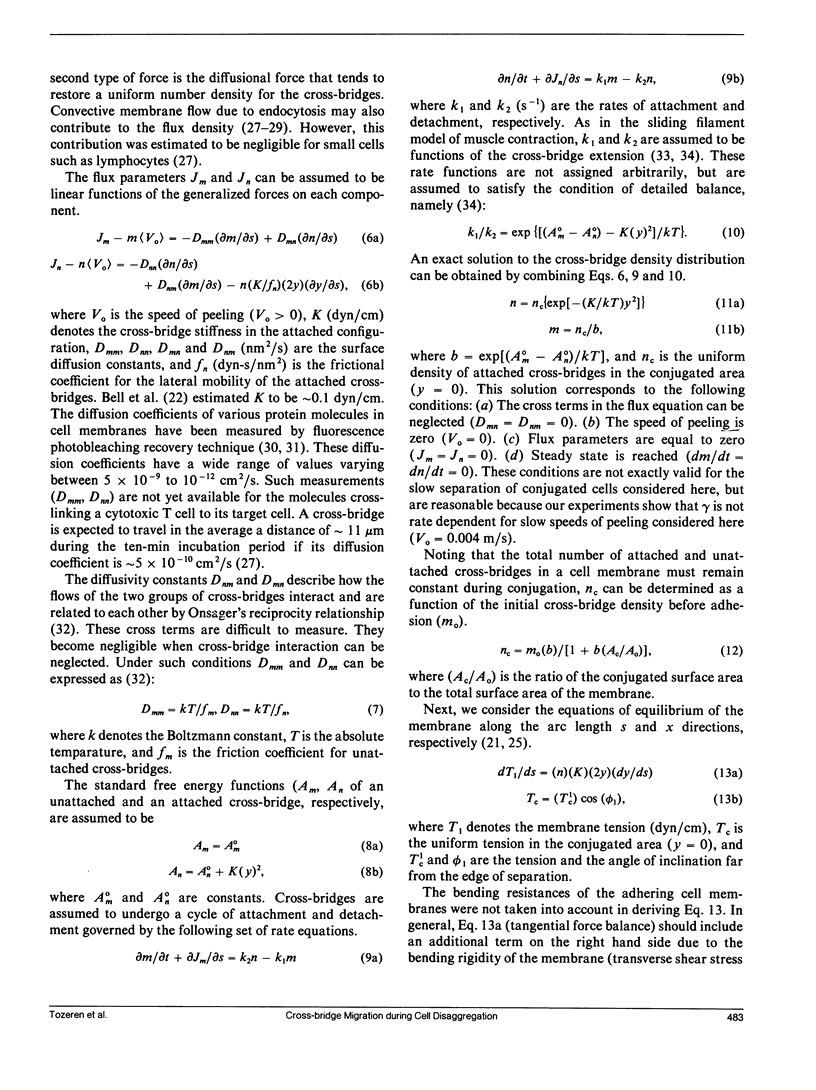
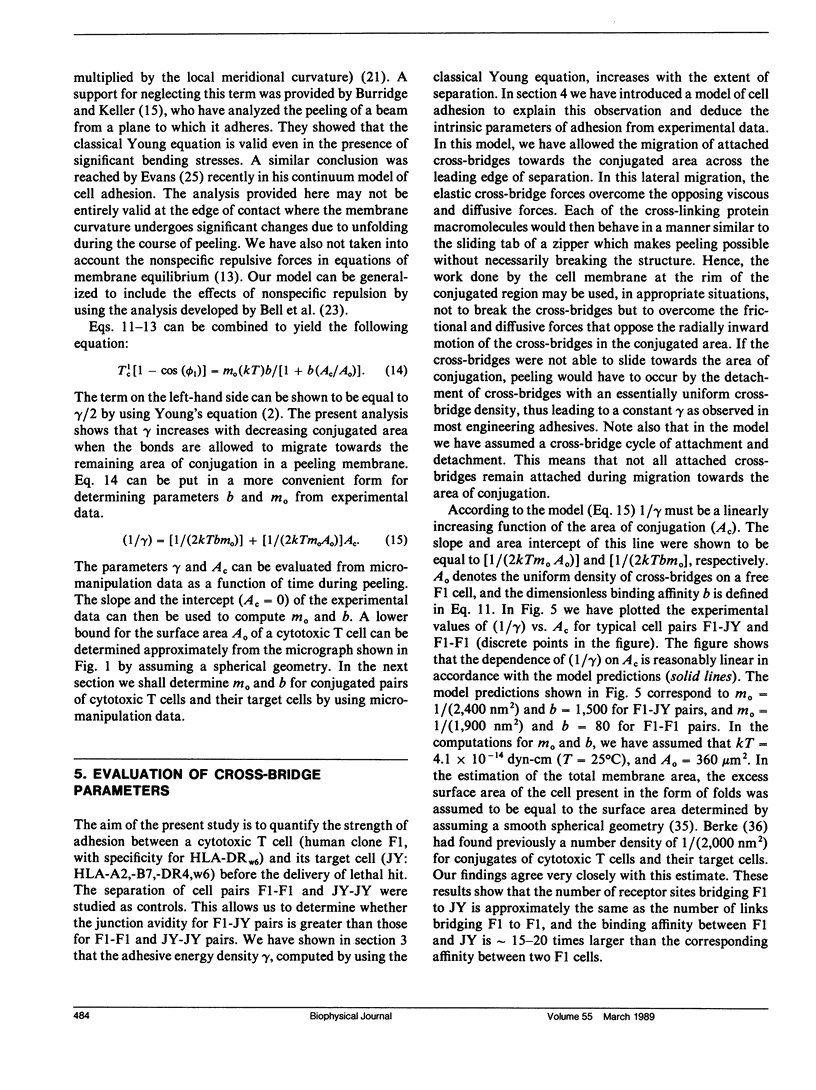
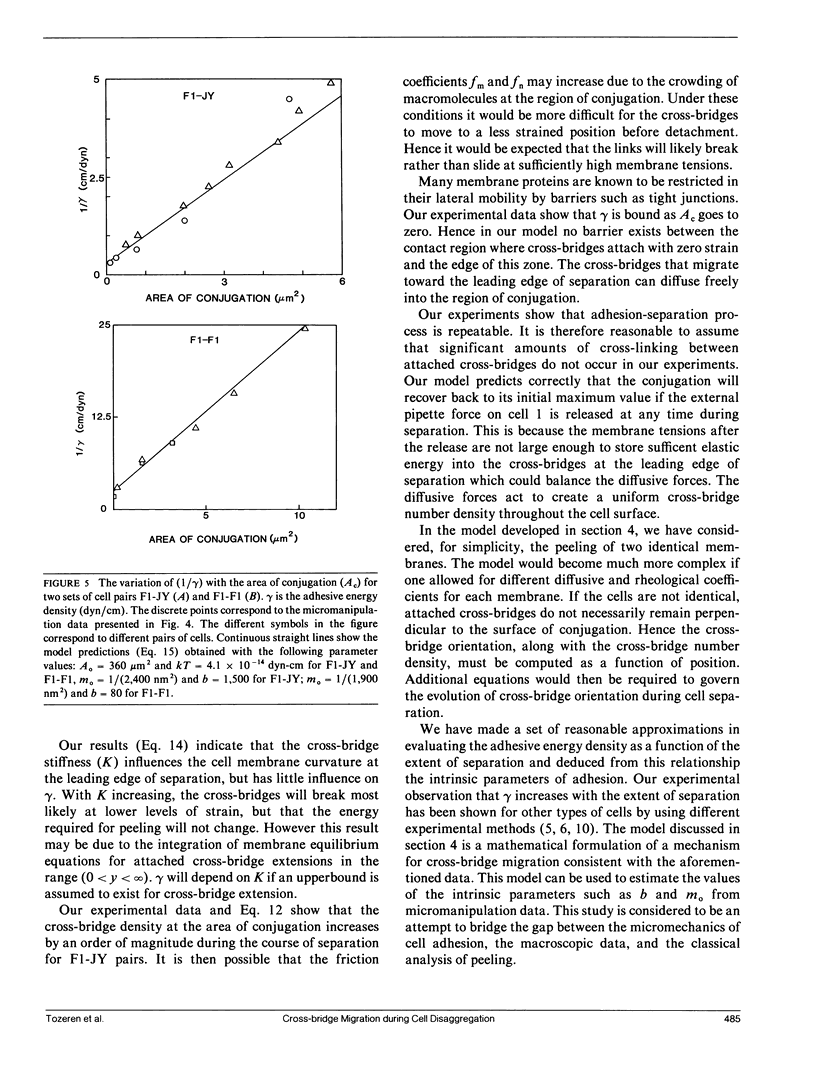
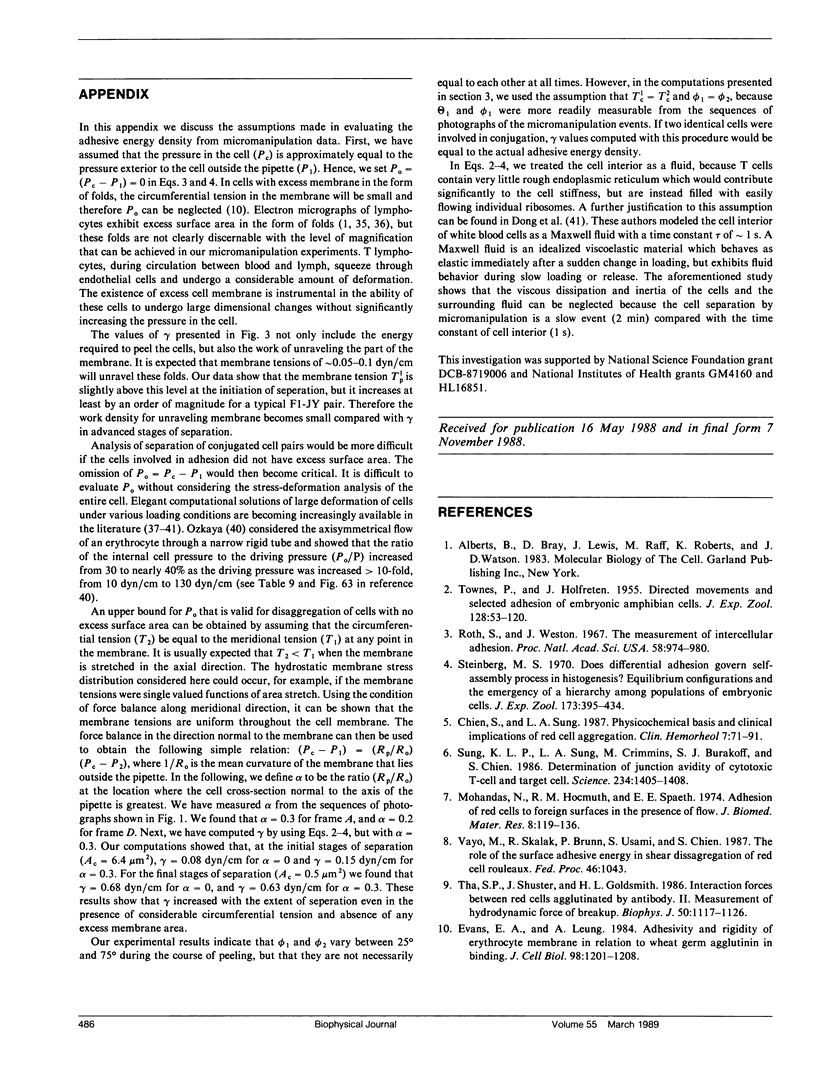
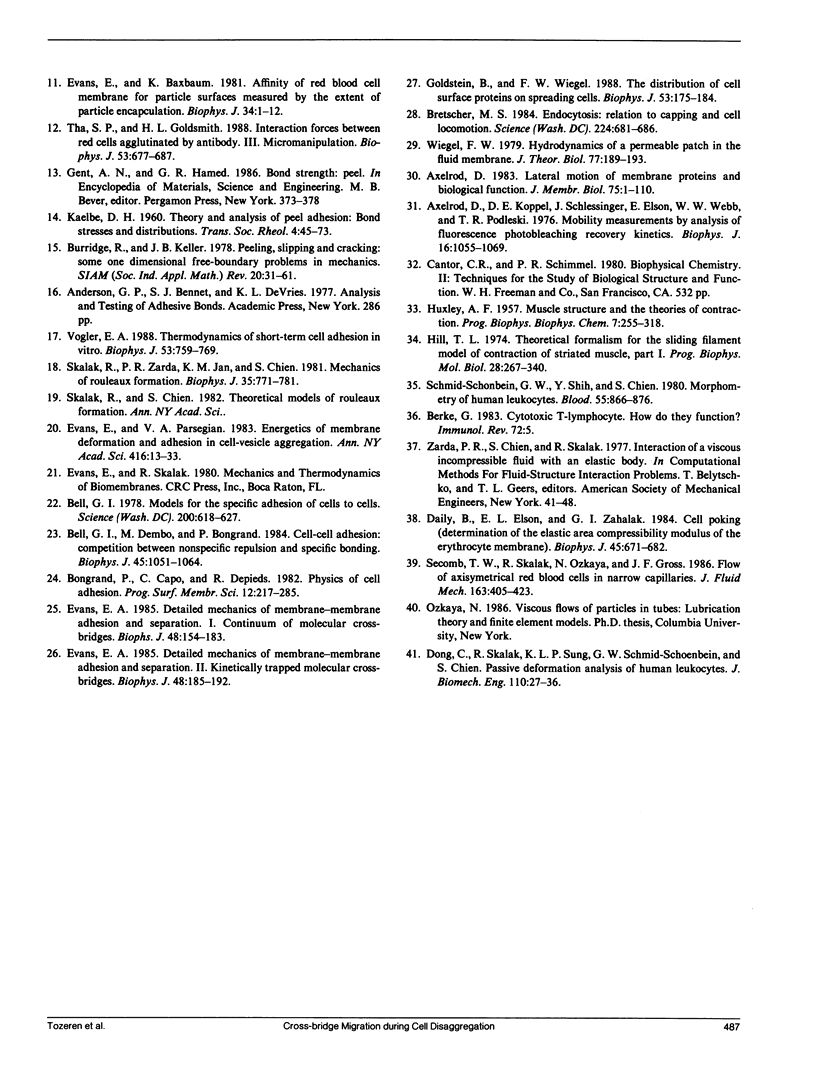
Images in this article
Selected References
These references are in PubMed. This may not be the complete list of references from this article.
- Axelrod D., Koppel D. E., Schlessinger J., Elson E., Webb W. W. Mobility measurement by analysis of fluorescence photobleaching recovery kinetics. Biophys J. 1976 Sep;16(9):1055–1069. doi: 10.1016/S0006-3495(76)85755-4. [DOI] [PMC free article] [PubMed] [Google Scholar]
- Axelrod D. Lateral motion of membrane proteins and biological function. J Membr Biol. 1983;75(1):1–10. doi: 10.1007/BF01870794. [DOI] [PubMed] [Google Scholar]
- Bell G. I., Dembo M., Bongrand P. Cell adhesion. Competition between nonspecific repulsion and specific bonding. Biophys J. 1984 Jun;45(6):1051–1064. doi: 10.1016/S0006-3495(84)84252-6. [DOI] [PMC free article] [PubMed] [Google Scholar]
- Bell G. I. Models for the specific adhesion of cells to cells. Science. 1978 May 12;200(4342):618–627. doi: 10.1126/science.347575. [DOI] [PubMed] [Google Scholar]
- Berke G. Cytotoxic T-lymphocytes. How do they function? Immunol Rev. 1983;72:5–42. doi: 10.1111/j.1600-065x.1983.tb01071.x. [DOI] [PubMed] [Google Scholar]
- Bretscher M. S. Endocytosis: relation to capping and cell locomotion. Science. 1984 May 18;224(4650):681–686. doi: 10.1126/science.6719108. [DOI] [PubMed] [Google Scholar]
- Daily B., Elson E. L., Zahalak G. I. Cell poking. Determination of the elastic area compressibility modulus of the erythrocyte membrane. Biophys J. 1984 Apr;45(4):671–682. doi: 10.1016/S0006-3495(84)84209-5. [DOI] [PMC free article] [PubMed] [Google Scholar]
- Evans E. A. Detailed mechanics of membrane-membrane adhesion and separation. I. Continuum of molecular cross-bridges. Biophys J. 1985 Jul;48(1):175–183. doi: 10.1016/S0006-3495(85)83770-X. [DOI] [PMC free article] [PubMed] [Google Scholar]
- Evans E. A. Detailed mechanics of membrane-membrane adhesion and separation. II. Discrete kinetically trapped molecular cross-bridges. Biophys J. 1985 Jul;48(1):185–192. doi: 10.1016/S0006-3495(85)83771-1. [DOI] [PMC free article] [PubMed] [Google Scholar]
- Evans E. A., Parsegian V. A. Energetics of membrane deformation and adhesion in cell and vesicle aggregation. Ann N Y Acad Sci. 1983;416:13–33. doi: 10.1111/j.1749-6632.1983.tb35176.x. [DOI] [PubMed] [Google Scholar]
- Evans E., Buxbaum K. Affinity of red blood cell membrane for particle surfaces measured by the extent of particle encapsulation. Biophys J. 1981 Apr;34(1):1–12. doi: 10.1016/S0006-3495(81)84834-5. [DOI] [PMC free article] [PubMed] [Google Scholar]
- Evans E., Leung A. Adhesivity and rigidity of erythrocyte membrane in relation to wheat germ agglutinin binding. J Cell Biol. 1984 Apr;98(4):1201–1208. doi: 10.1083/jcb.98.4.1201. [DOI] [PMC free article] [PubMed] [Google Scholar]
- Goldstein B., Wiegel F. W. The distribution of cell surface proteins on spreading cells. Comparison of theory with experiment. Biophys J. 1988 Feb;53(2):175–184. doi: 10.1016/S0006-3495(88)83079-0. [DOI] [PMC free article] [PubMed] [Google Scholar]
- HUXLEY A. F. Muscle structure and theories of contraction. Prog Biophys Biophys Chem. 1957;7:255–318. [PubMed] [Google Scholar]
- Hill T. L. Theoretical formalism for the sliding filament model of contraction of striated muscle. Part I. Prog Biophys Mol Biol. 1974;28:267–340. doi: 10.1016/0079-6107(74)90020-0. [DOI] [PubMed] [Google Scholar]
- Mohandas N., Hochmuth R. M., Spaeth E. E. Adhesion of red cells to foreign surfaces in the presence of flow. J Biomed Mater Res. 1974 Mar;8(2):119–136. doi: 10.1002/jbm.820080203. [DOI] [PubMed] [Google Scholar]
- Roth S. A., Weston J. A. The measurement of intercellular adhesion. Proc Natl Acad Sci U S A. 1967 Sep;58(3):974–980. doi: 10.1073/pnas.58.3.974. [DOI] [PMC free article] [PubMed] [Google Scholar]
- Schmid-Schönbein G. W., Shih Y. Y., Chien S. Morphometry of human leukocytes. Blood. 1980 Nov;56(5):866–875. [PubMed] [Google Scholar]
- Skalak R., Zarda P. R., Jan K. M., Chien S. Mechanics of Rouleau formation. Biophys J. 1981 Sep;35(3):771–781. doi: 10.1016/S0006-3495(81)84826-6. [DOI] [PMC free article] [PubMed] [Google Scholar]
- Steinberg M. S. Does differential adhesion govern self-assembly processes in histogenesis? Equilibrium configurations and the emergence of a hierarchy among populations of embryonic cells. J Exp Zool. 1970 Apr;173(4):395–433. doi: 10.1002/jez.1401730406. [DOI] [PubMed] [Google Scholar]
- Sung K. L., Sung L. A., Crimmins M., Burakoff S. J., Chien S. Determination of junction avidity of cytolytic T cell and target cell. Science. 1986 Dec 12;234(4782):1405–1408. doi: 10.1126/science.3491426. [DOI] [PubMed] [Google Scholar]
- Tha S. P., Goldsmith H. L. Interaction forces between red cells agglutinated by antibody. III. Micromanipulation. Biophys J. 1988 May;53(5):677–687. doi: 10.1016/S0006-3495(88)83149-7. [DOI] [PMC free article] [PubMed] [Google Scholar]
- Tha S. P., Shuster J., Goldsmith H. L. Interaction forces between red cells agglutinated by antibody. II. Measurement of hydrodynamic force of breakup. Biophys J. 1986 Dec;50(6):1117–1126. doi: 10.1016/S0006-3495(86)83556-1. [DOI] [PMC free article] [PubMed] [Google Scholar]
- Vogler E. A. Thermodynamics of short-term cell adhesion in vitro. Biophys J. 1988 May;53(5):759–769. doi: 10.1016/S0006-3495(88)83156-4. [DOI] [PMC free article] [PubMed] [Google Scholar]
- Wiegel F. W. Hydrodynamics of a permeable patch in the fluid membrane. J Theor Biol. 1979 Mar 21;77(2):189–193. doi: 10.1016/0022-5193(79)90306-0. [DOI] [PubMed] [Google Scholar]