Abstract
For the purpose of determining net interactions between actin and myosin filaments in muscle cells, perhaps the single most informative view of the myofilament lattice is its averaged axial projection. We have studied frozen-hydrated transverse thin sections with the goal of obtaining axial projections that are not subject to the limitations of conventional thin sectioning (suspect preservation of native structure) or of equatorial x-ray diffraction analysis (lack of experimental phases). In principle, good preservation of native structure may be achieved with fast freezing, followed by low-dose electron imaging of unstained vitrified cryosections. In practice, however, cryosections undergo large-scale distortions, including irreversible compression; furthermore, phase contrast imaging results in a nonlinear relationship between the projected density of the specimen and the optical density of the micrograph. To overcome these limitations, we have devised methods of image restoration and generalized correlation averaging, and applied them to cryosections of rabbit psoas fibers in both the relaxed and rigor states. Thus visualized, myosin filaments appear thicker than actin filaments by a much smaller margin than in conventional thin sections, and particularly so for rigor muscle. This may result from a significant fraction of the myosin S1-cross-bridges averaging out in projection and thus contributing only to the baseline of projected density. Entering rigor incurs a loss of density from an annulus around the myosin filament, with a compensating accumulation of density around the actin filament. This redistribution of mass represents attachment of the fraction of cross-bridges that are visible above background. Myosin filaments in the "nonoverlap" zone appear to broaden on entering rigor, suggesting that on deprivation of ATP, cross-bridges in situ move outwards even without actin in their immediate proximity.
Full text
PDF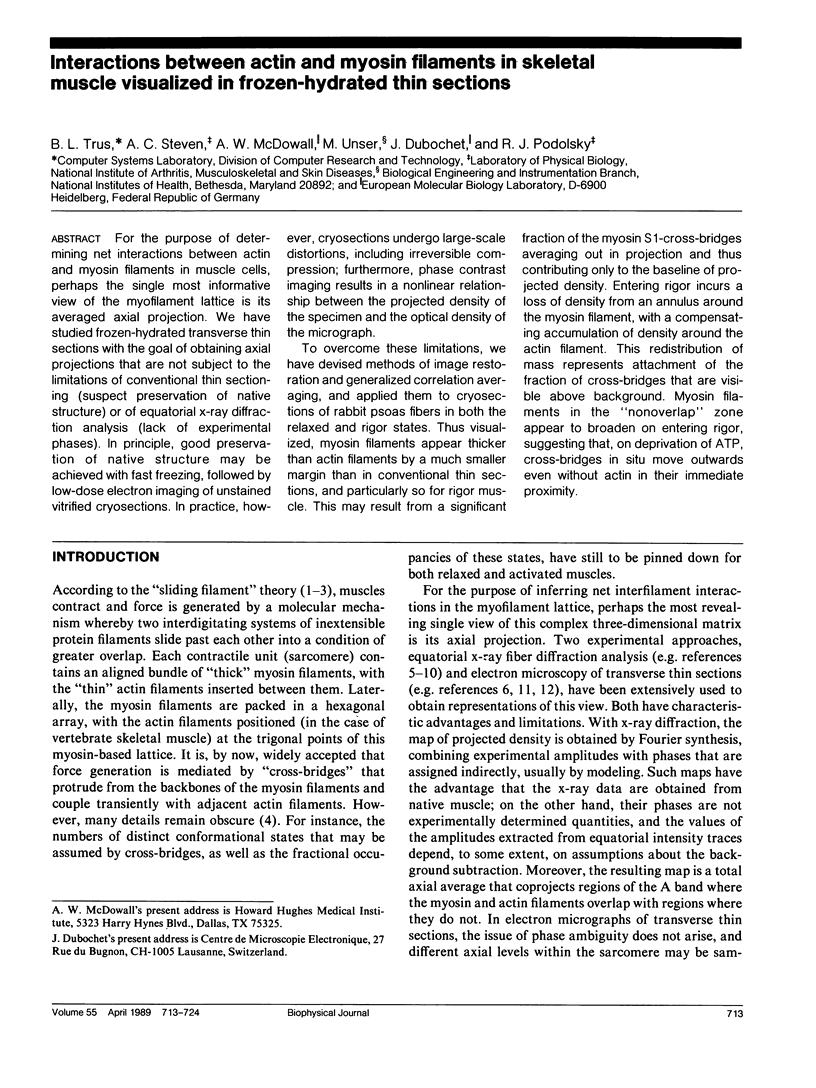
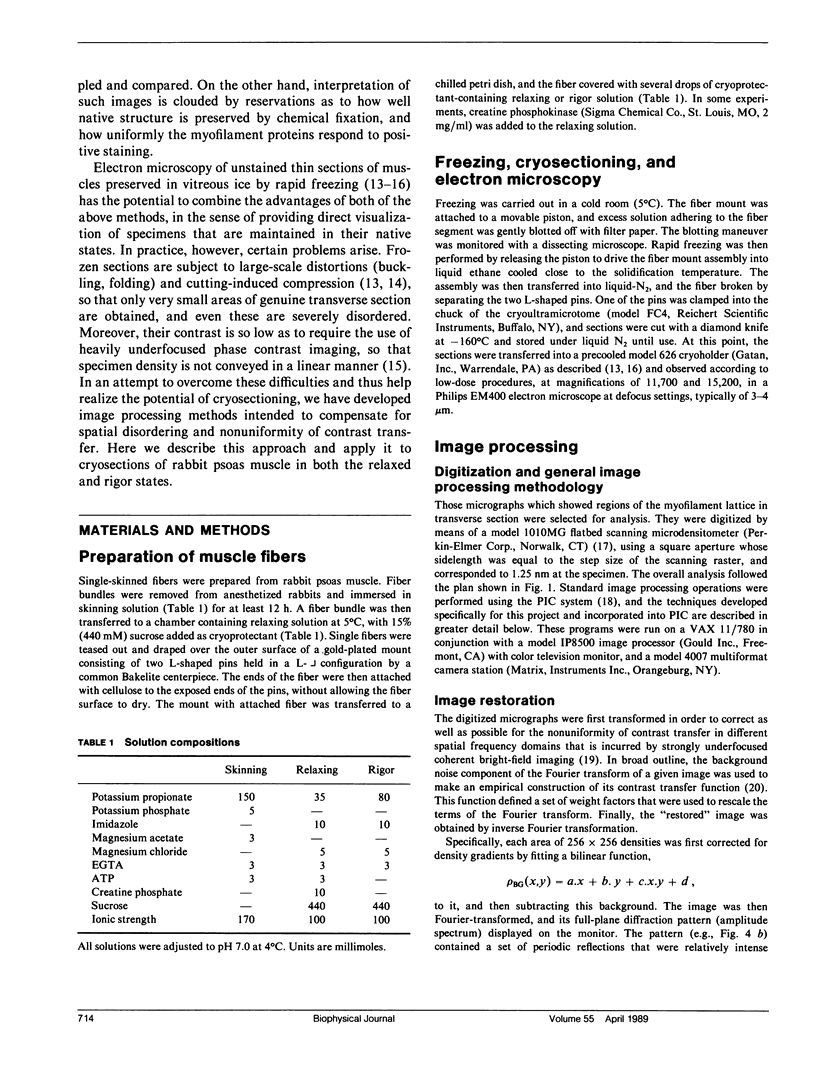
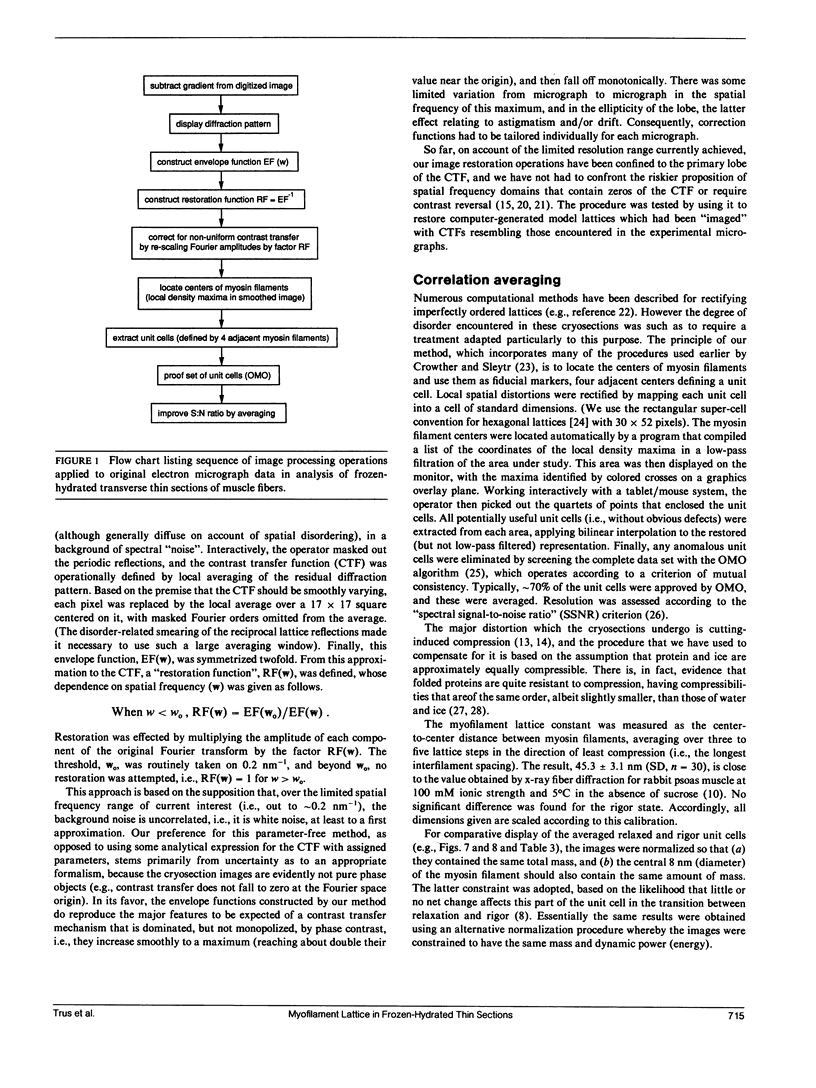
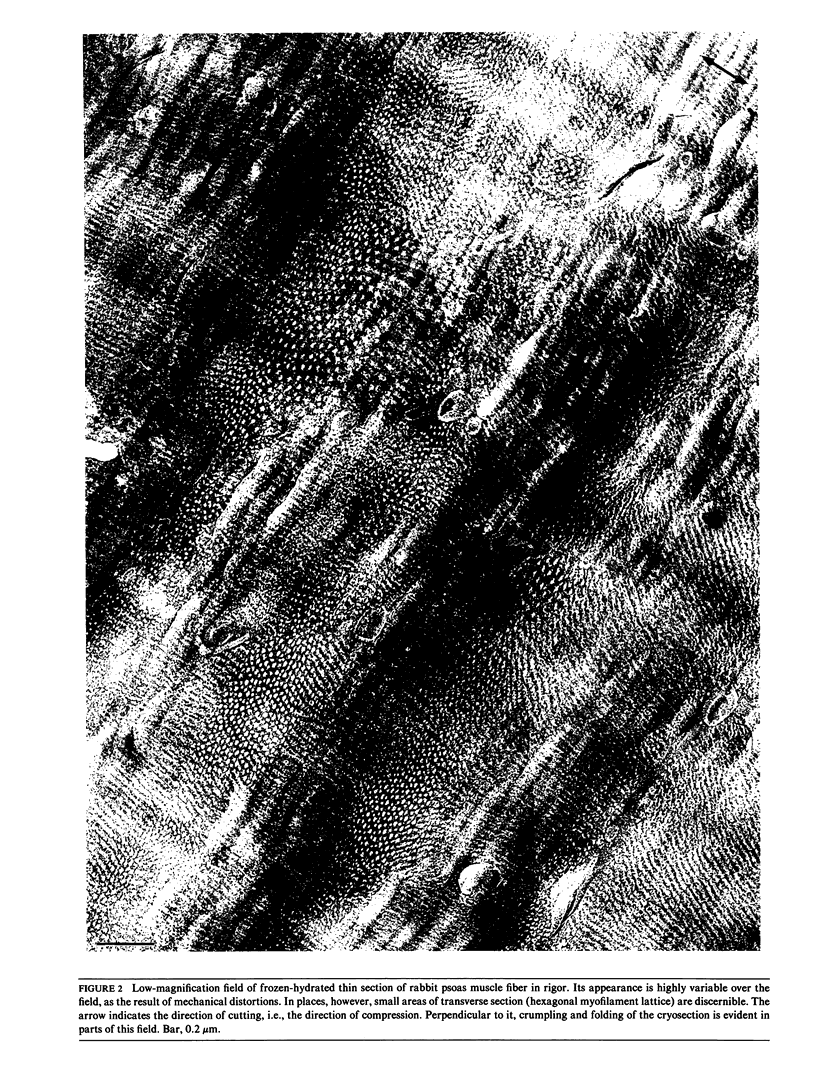
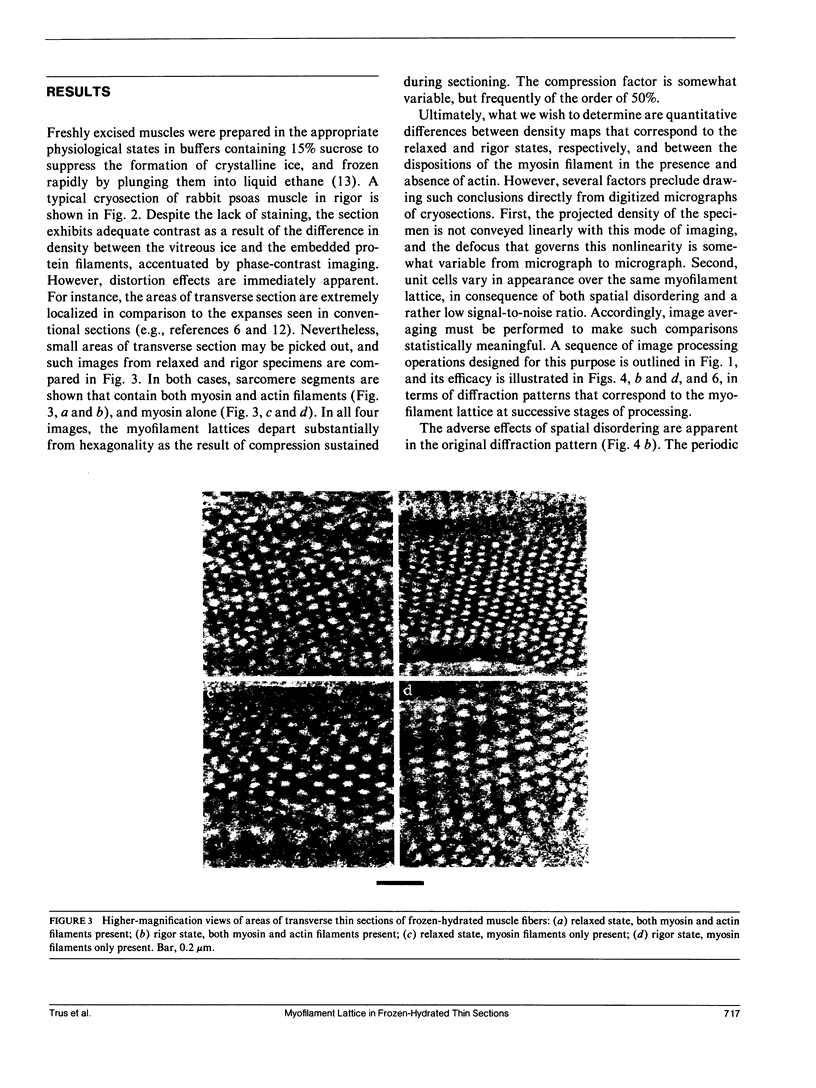
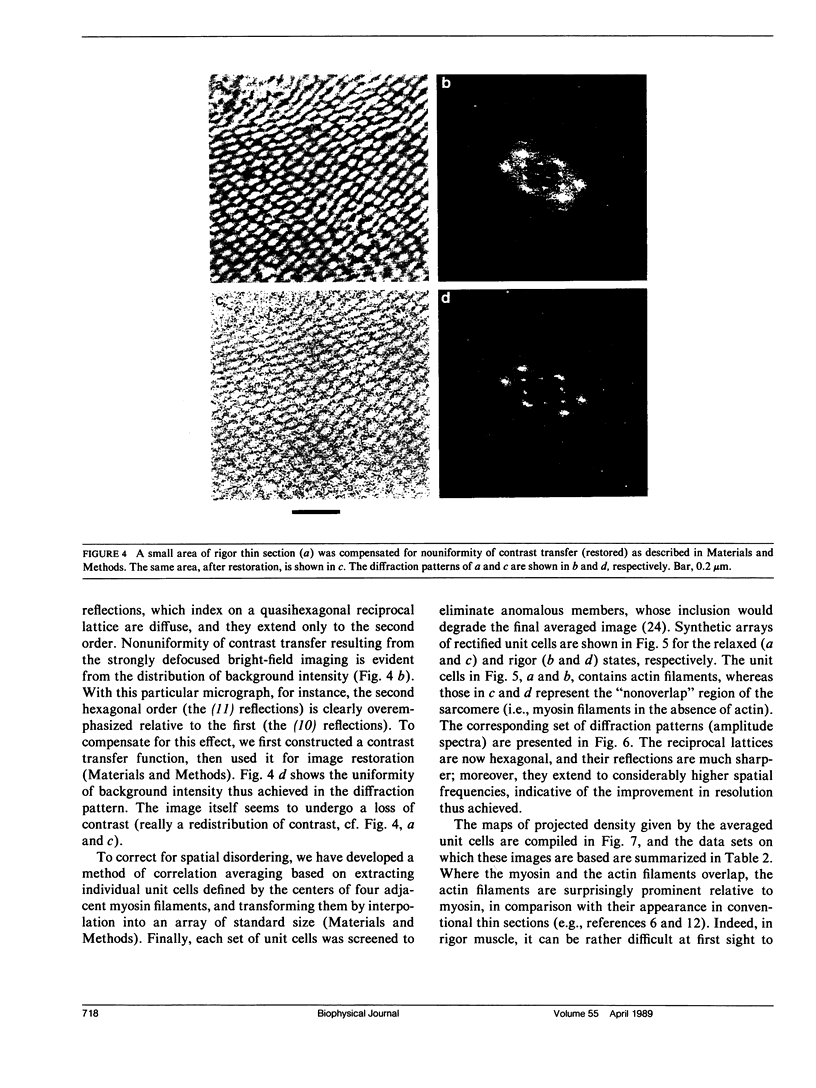
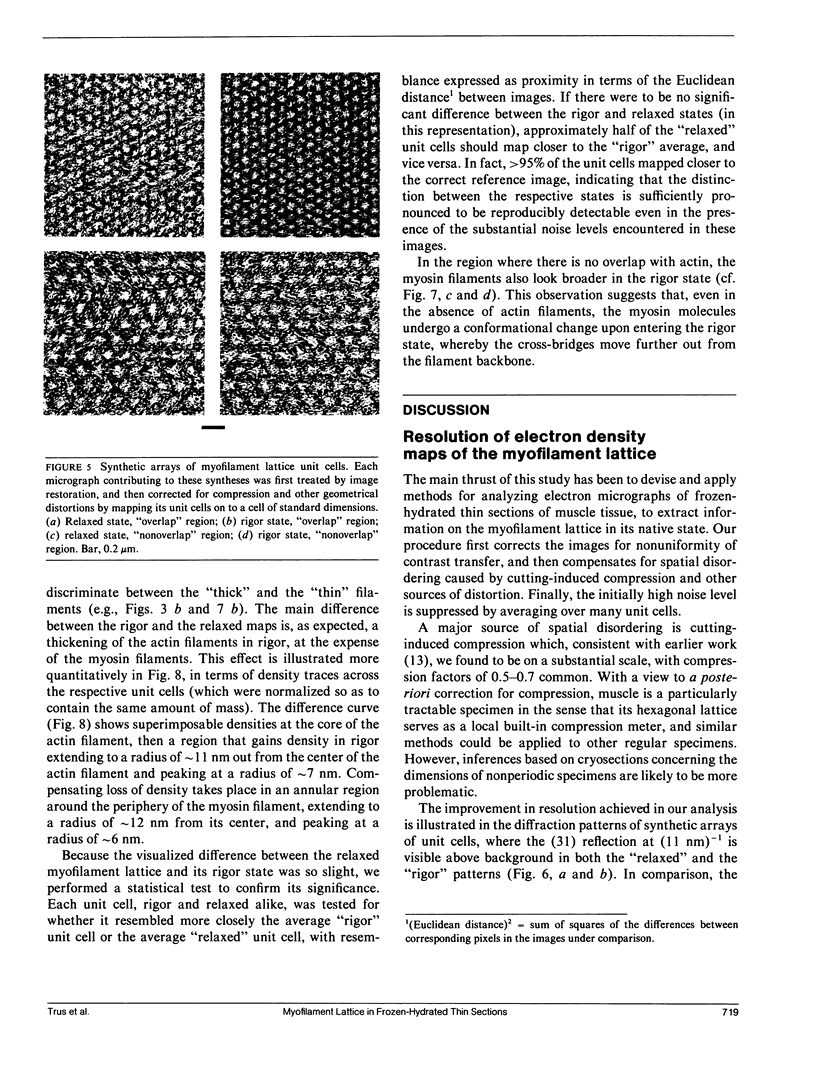
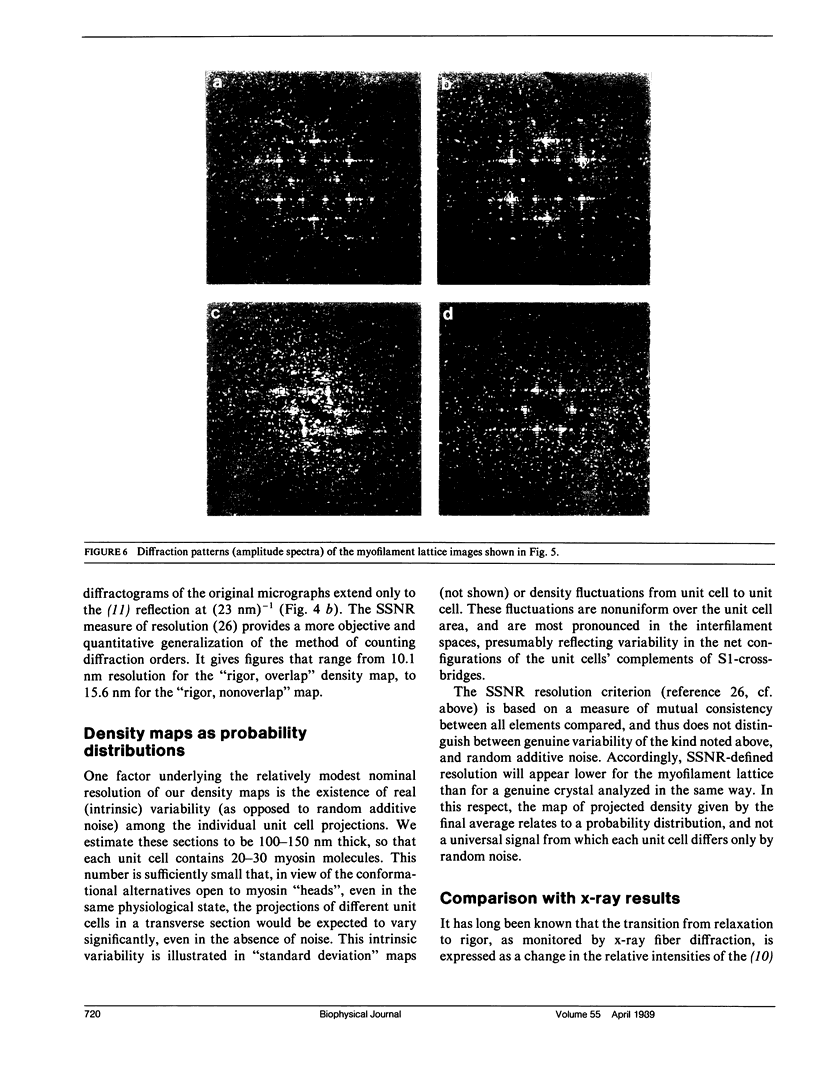
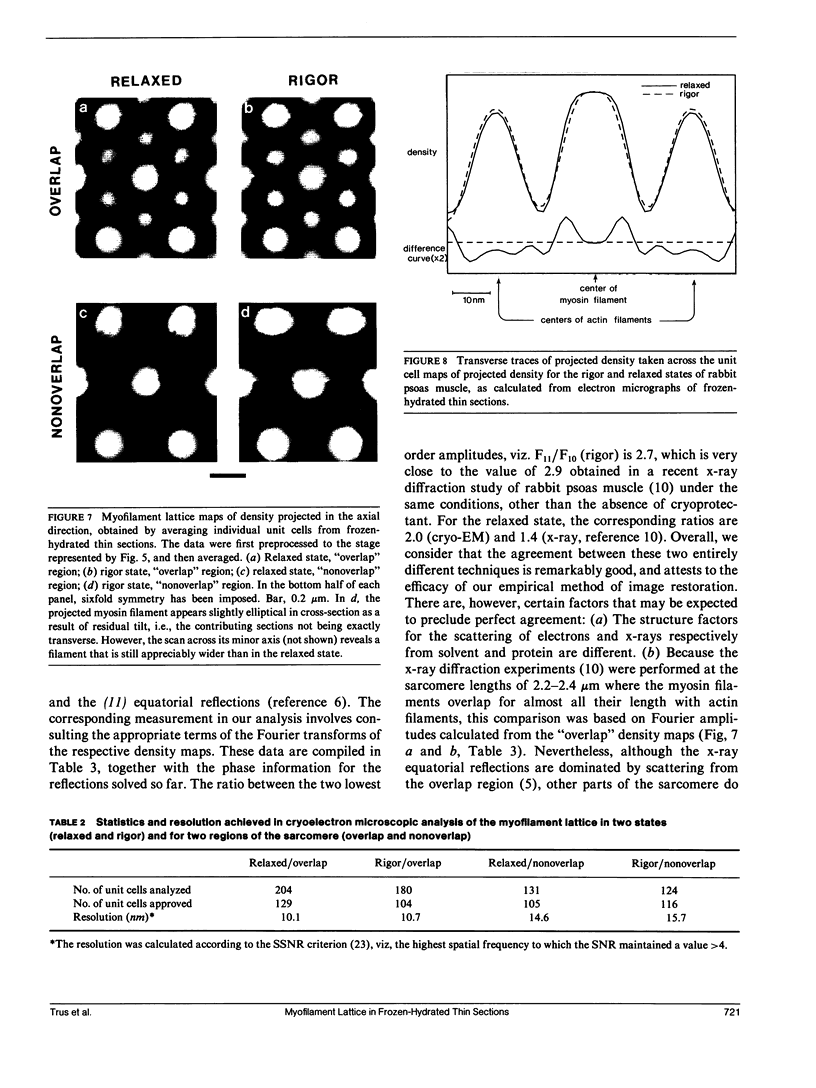
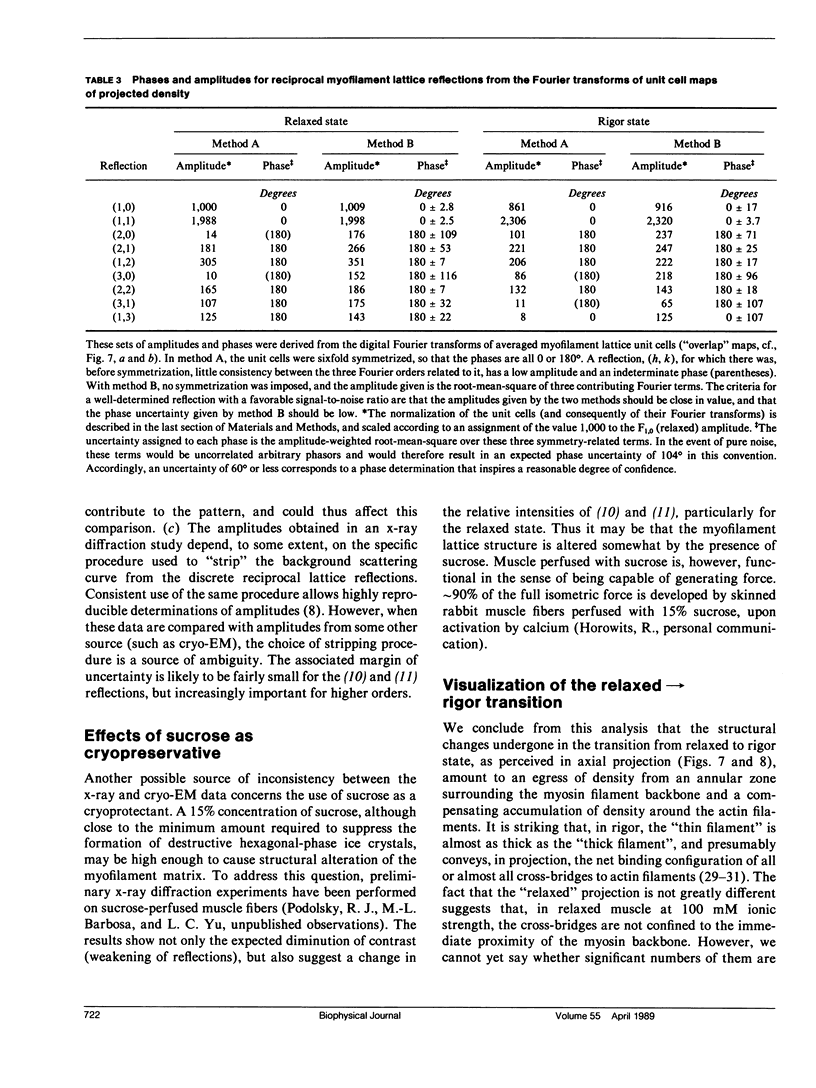
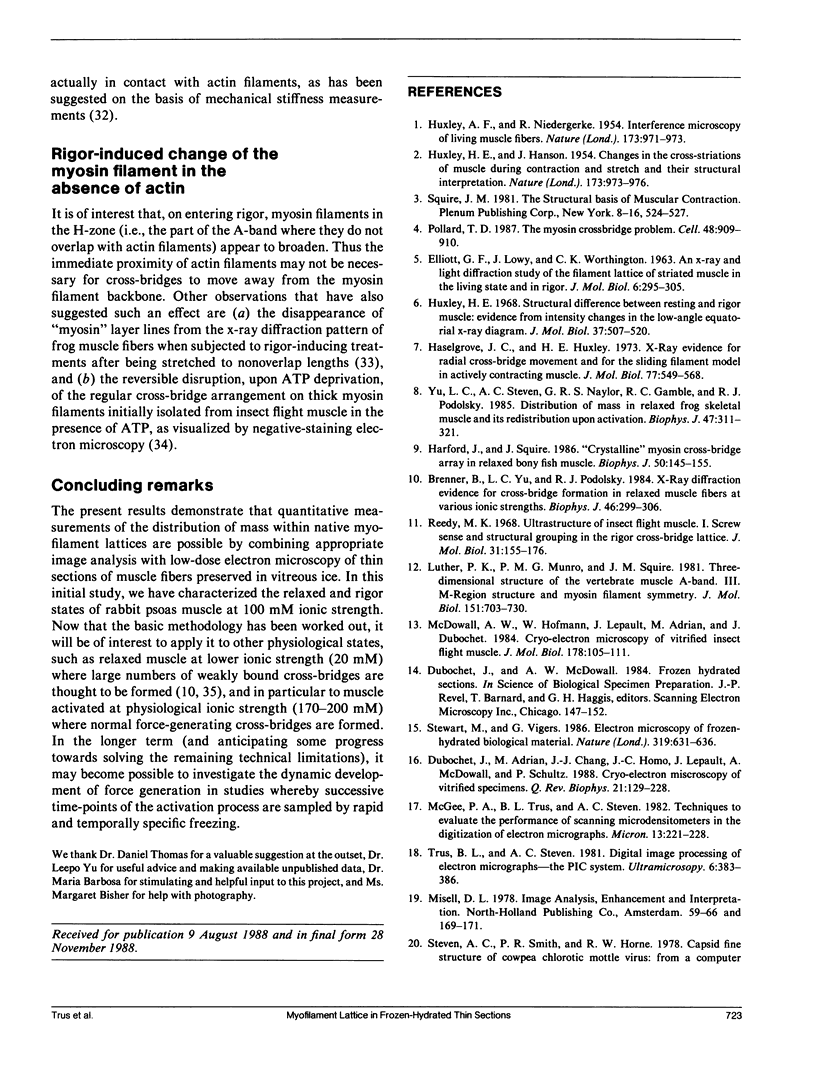
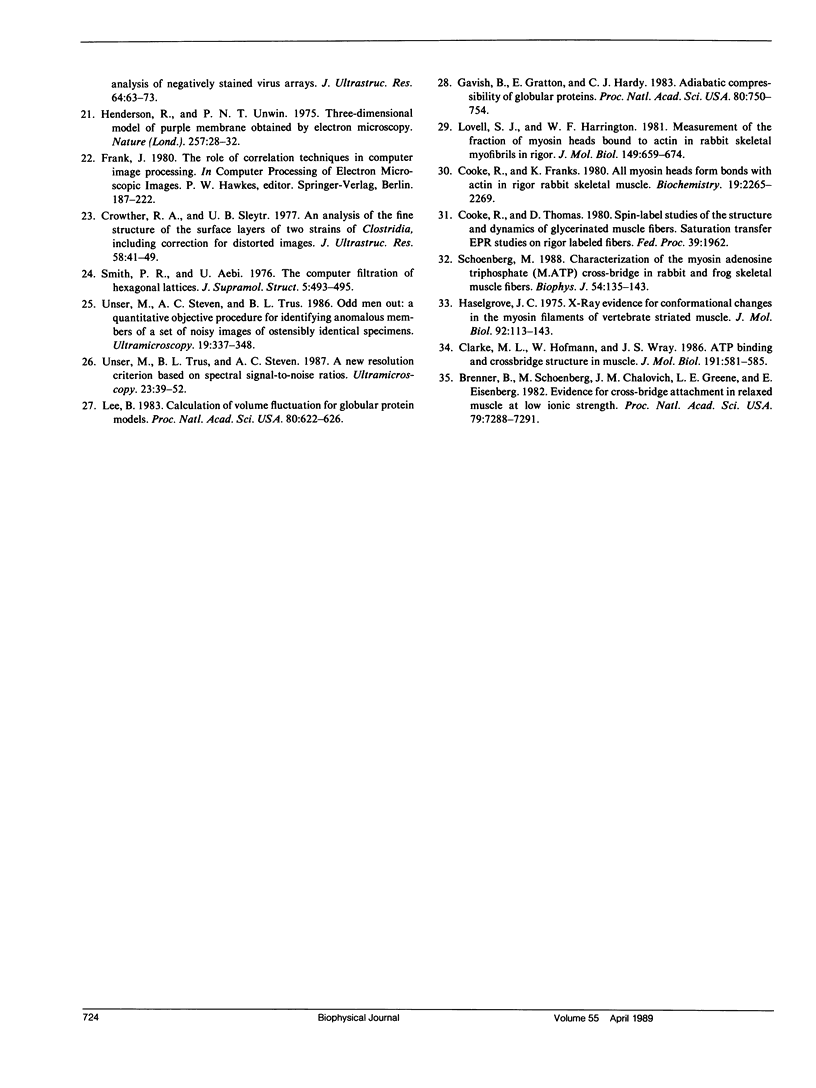
Images in this article
Selected References
These references are in PubMed. This may not be the complete list of references from this article.
- Aebi U., Bijlenga R. K., ten Heggeler B., Kistler J., Steven A. C., Smith P. R. Comparison of the structural and chemical composition of giant T-even phage heads. J Supramol Struct. 1976;5(4):475–495. doi: 10.1002/jss.400050406. [DOI] [PubMed] [Google Scholar]
- Brenner B., Schoenberg M., Chalovich J. M., Greene L. E., Eisenberg E. Evidence for cross-bridge attachment in relaxed muscle at low ionic strength. Proc Natl Acad Sci U S A. 1982 Dec;79(23):7288–7291. doi: 10.1073/pnas.79.23.7288. [DOI] [PMC free article] [PubMed] [Google Scholar]
- Brenner B., Yu L. C., Podolsky R. J. X-ray diffraction evidence for cross-bridge formation in relaxed muscle fibers at various ionic strengths. Biophys J. 1984 Sep;46(3):299–306. doi: 10.1016/S0006-3495(84)84026-6. [DOI] [PMC free article] [PubMed] [Google Scholar]
- Clarke M. L., Hofman W., Wray J. S. ATP binding and crossbridge structure in muscle. J Mol Biol. 1986 Oct 5;191(3):581–585. doi: 10.1016/0022-2836(86)90153-1. [DOI] [PubMed] [Google Scholar]
- Cooke R., Franks K. All myosin heads form bonds with actin in rigor rabbit skeletal muscle. Biochemistry. 1980 May 13;19(10):2265–2269. doi: 10.1021/bi00551a042. [DOI] [PubMed] [Google Scholar]
- Crowther R. A., Sleytr U. B. An analysis of the fine structure of the surface layers from two strains of Clostridia, including correction for distorted images. J Ultrastruct Res. 1977 Jan;58(1):41–49. doi: 10.1016/s0022-5320(77)80006-3. [DOI] [PubMed] [Google Scholar]
- Dubochet J., Adrian M., Chang J. J., Homo J. C., Lepault J., McDowall A. W., Schultz P. Cryo-electron microscopy of vitrified specimens. Q Rev Biophys. 1988 May;21(2):129–228. doi: 10.1017/s0033583500004297. [DOI] [PubMed] [Google Scholar]
- Gavish B., Gratton E., Hardy C. J. Adiabatic compressibility of globular proteins. Proc Natl Acad Sci U S A. 1983 Feb;80(3):750–754. doi: 10.1073/pnas.80.3.750. [DOI] [PMC free article] [PubMed] [Google Scholar]
- HUXLEY A. F., NIEDERGERKE R. Structural changes in muscle during contraction; interference microscopy of living muscle fibres. Nature. 1954 May 22;173(4412):971–973. doi: 10.1038/173971a0. [DOI] [PubMed] [Google Scholar]
- HUXLEY H., HANSON J. Changes in the cross-striations of muscle during contraction and stretch and their structural interpretation. Nature. 1954 May 22;173(4412):973–976. doi: 10.1038/173973a0. [DOI] [PubMed] [Google Scholar]
- Harford J., Squire J. "Crystalline" myosin cross-bridge array in relaxed bony fish muscle. Low-angle x-ray diffraction from plaice fin muscle and its interpretation. Biophys J. 1986 Jul;50(1):145–155. doi: 10.1016/S0006-3495(86)83447-6. [DOI] [PMC free article] [PubMed] [Google Scholar]
- Haselgrove J. C., Huxley H. E. X-ray evidence for radial cross-bridge movement and for the sliding filament model in actively contracting skeletal muscle. J Mol Biol. 1973 Jul 15;77(4):549–568. doi: 10.1016/0022-2836(73)90222-2. [DOI] [PubMed] [Google Scholar]
- Haselgrove J. C. X-ray evidence for conformational changes in the myosin filaments of vertebrate striated muscle. J Mol Biol. 1975 Feb 15;92(1):113–143. doi: 10.1016/0022-2836(75)90094-7. [DOI] [PubMed] [Google Scholar]
- Henderson R., Unwin P. N. Three-dimensional model of purple membrane obtained by electron microscopy. Nature. 1975 Sep 4;257(5521):28–32. doi: 10.1038/257028a0. [DOI] [PubMed] [Google Scholar]
- Huxley H. E. Structural difference between resting and rigor muscle; evidence from intensity changes in the lowangle equatorial x-ray diagram. J Mol Biol. 1968 Nov 14;37(3):507–520. doi: 10.1016/0022-2836(68)90118-6. [DOI] [PubMed] [Google Scholar]
- Lee B. Calculation of volume fluctuation for globular protein models. Proc Natl Acad Sci U S A. 1983 Jan;80(2):622–626. doi: 10.1073/pnas.80.2.622. [DOI] [PMC free article] [PubMed] [Google Scholar]
- Lovell S. J., Harrington W. F. Measurement of the fraction of myosin heads bound to actin in rabbit skeletal myofibrils in rigor. J Mol Biol. 1981 Jul 15;149(4):659–674. doi: 10.1016/0022-2836(81)90352-1. [DOI] [PubMed] [Google Scholar]
- Luther P. K., Munro P. M., Squire J. M. Three-dimensional structure of the vertebrate muscle A-band. III. M-region structure and myosin filament symmetry. J Mol Biol. 1981 Oct 5;151(4):703–730. doi: 10.1016/0022-2836(81)90430-7. [DOI] [PubMed] [Google Scholar]
- McDowall A. W., Hofmann W., Lepault J., Adrian M., Dubochet J. Cryo-electron microscopy of vitrified insect flight muscle. J Mol Biol. 1984 Sep 5;178(1):105–111. doi: 10.1016/0022-2836(84)90233-x. [DOI] [PubMed] [Google Scholar]
- Pollard T. D. The myosin crossbridge problem. Cell. 1987 Mar 27;48(6):909–910. doi: 10.1016/0092-8674(87)90087-0. [DOI] [PMC free article] [PubMed] [Google Scholar]
- Reedy M. K. Ultrastructure of insect flight muscle. I. Screw sense and structural grouping in the rigor cross-bridge lattice. J Mol Biol. 1968 Jan 28;31(2):155–176. doi: 10.1016/0022-2836(68)90437-3. [DOI] [PubMed] [Google Scholar]
- Schoenberg M. Characterization of the myosin adenosine triphosphate (M.ATP) crossbridge in rabbit and frog skeletal muscle fibers. Biophys J. 1988 Jul;54(1):135–148. doi: 10.1016/S0006-3495(88)82938-2. [DOI] [PMC free article] [PubMed] [Google Scholar]
- Steven A. C., Smith P. R., Horne R. W. Capsid fine structure of cowpea chlorotic mottle virus: from a computer analysis of negatively stained virus arrays. J Ultrastruct Res. 1978 Jul;64(1):63–73. doi: 10.1016/s0022-5320(78)90007-2. [DOI] [PubMed] [Google Scholar]
- Stewart M., Vigers G. Electron microscopy of frozen-hydrated biological material. Nature. 1986 Feb 20;319(6055):631–636. doi: 10.1038/319631a0. [DOI] [PubMed] [Google Scholar]
- Unser M., Steven A. C., Trus B. L. Odd men out: a quantitative objective procedure for identifying anomalous members of a set of noisy images of ostensibly identical specimens. Ultramicroscopy. 1986;19(4):337–347. doi: 10.1016/0304-3991(86)90094-x. [DOI] [PubMed] [Google Scholar]
- Unser M., Trus B. L., Steven A. C. A new resolution criterion based on spectral signal-to-noise ratios. Ultramicroscopy. 1987;23(1):39–51. doi: 10.1016/0304-3991(87)90225-7. [DOI] [PubMed] [Google Scholar]
- Yu L. C., Steven A. C., Naylor G. R., Gamble R. C., Podolsky R. J. Distribution of mass in relaxed frog skeletal muscle and its redistribution upon activation. Biophys J. 1985 Mar;47(3):311–321. doi: 10.1016/S0006-3495(85)83921-7. [DOI] [PMC free article] [PubMed] [Google Scholar]