Abstract
Several lines of evidence suggest that nonspecific drug interaction with the lipid bilayer plays an important role in subsequent recognition and binding to specific receptor sites in the membrane. The interaction of Bay K 8644, a 1,4-dihydropyridine (DHP) calcium channel agonist, with model and biological membranes was examined at the molecular level using small angle x-ray diffraction. Nonspecific drug partitioning into the membrane was examined by radiochemical assay. Nonspecific binding characteristics of [3H] Bay K 8644 were determined in both dipalmitoyl phosphatidylcholine (DPPC) vesicles above and below their thermal phase transition (Tm) and rabbit skeletal muscle light sarcoplasmic reticulum (LSR). In DPPC, the partition coefficient, Kp, was 14,000 above the Tm (55 degrees C) versus 160 in the gel phase (2 degrees C). The Kp determined in LSR membranes was 10,700. These values for both DPPC and LSR membranes can be compared with Kp = 290 in the traditional octanol/buffer system. Using small-angle x-ray diffraction, the equilibrium position of the electron-dense trifluoromethyl group of Bay K 8644 in DPPC (above Tm) and purified cardiac sarcolemmal (CSL) lipid bilayers was determined to be consistently located within the region of the first few methylene segments of the fatty acyl chains of these membranes. This position is similar to that observed for the DHP calcium channel antagonists nimodipine and Bay P 8857. We suggest this particular membrane location defines a region of local drug concentration and plane for lateral diffusion to a common receptor site. Below the DPPC membrane Tm, Bay K 8644 was shown to be excluded from this energetically favored position into the interbilayer water space. Heating the DPPC bilayer above the Tm (55 degrees C) showed that this exclusion was reversible and indicates that drug-membrane interaction is dependent on the bilayer physical state. The absence of any specific protein binding sites in these systems allows us to ascertain the potentially important role that the bulk lipid phase may play in the molecular mechanism of DHP binding to the specific receptor site associated with the calcium channel.
Full text
PDF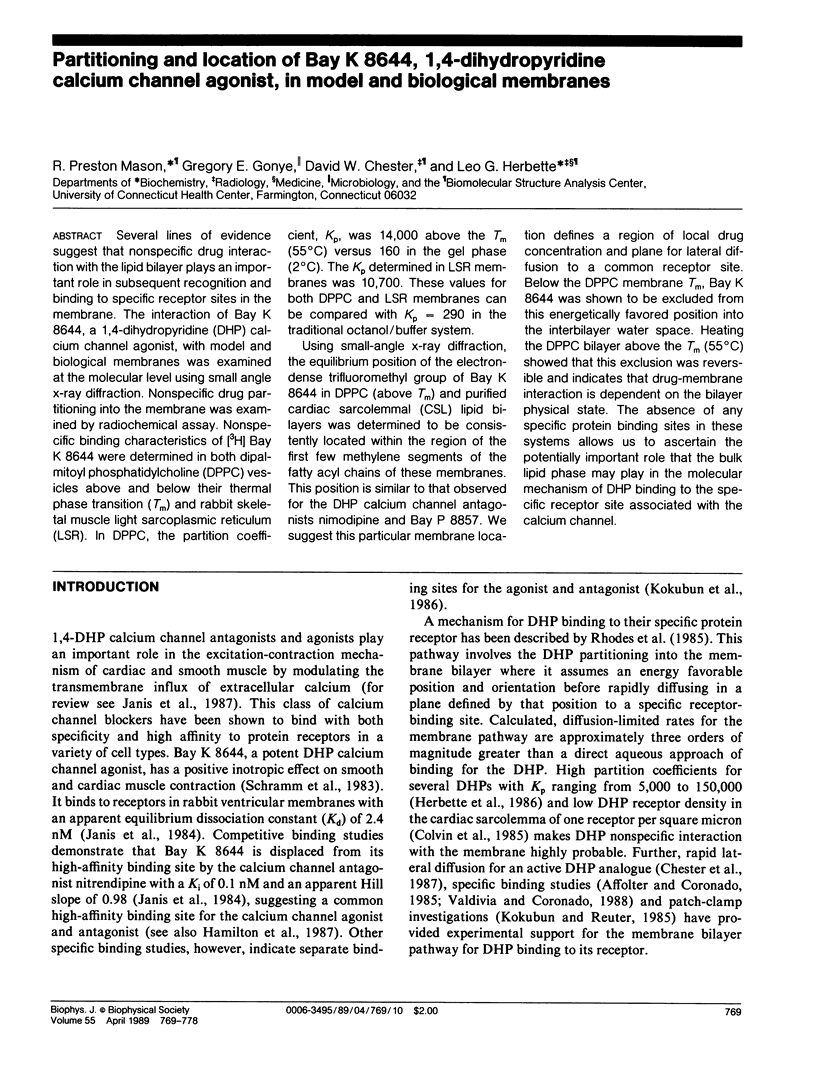
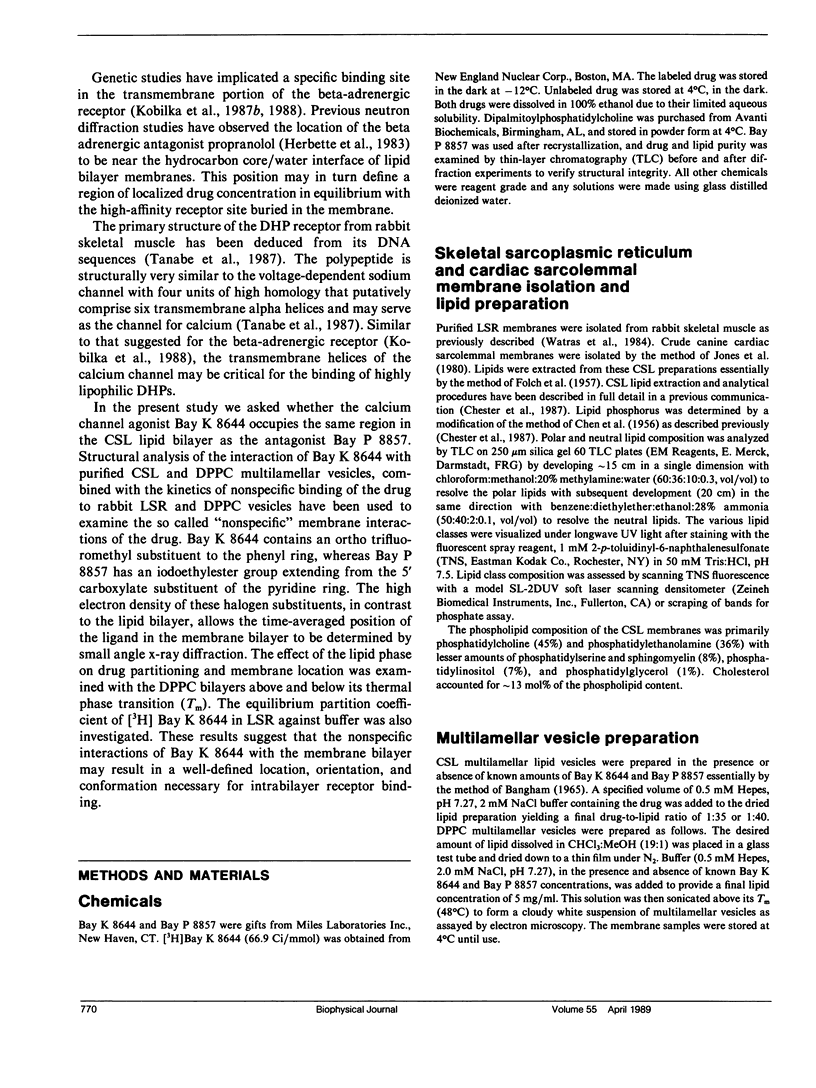
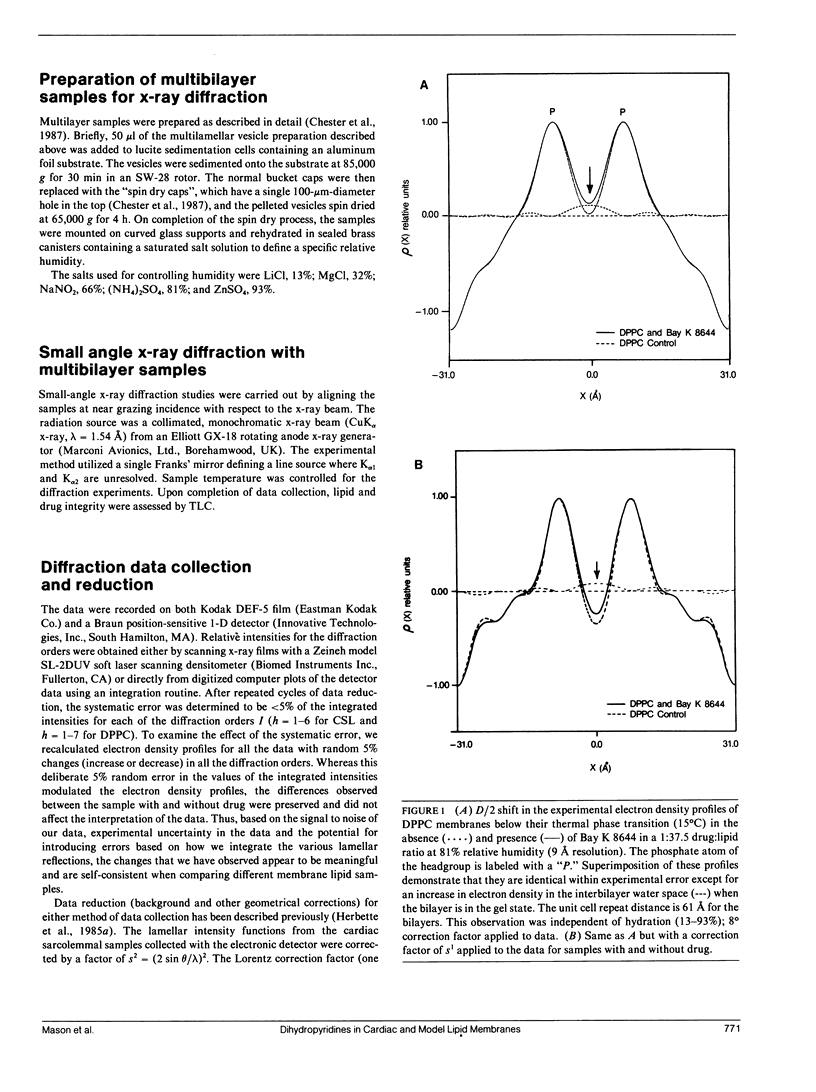
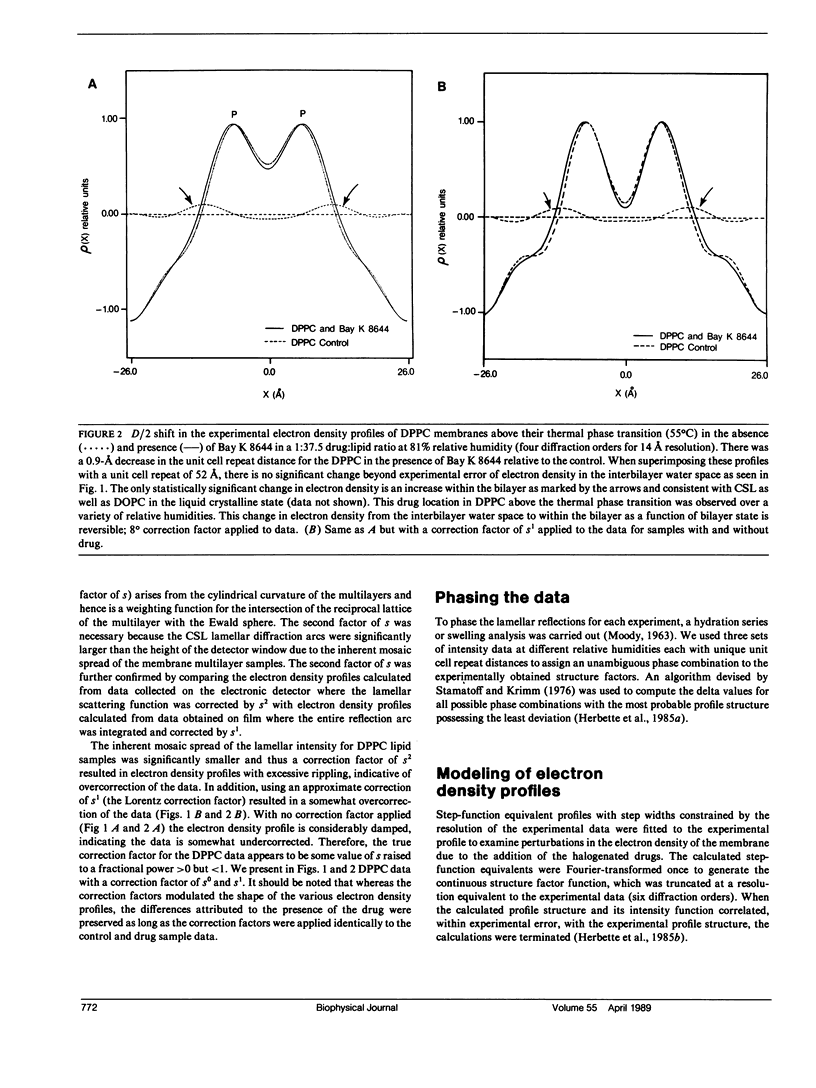
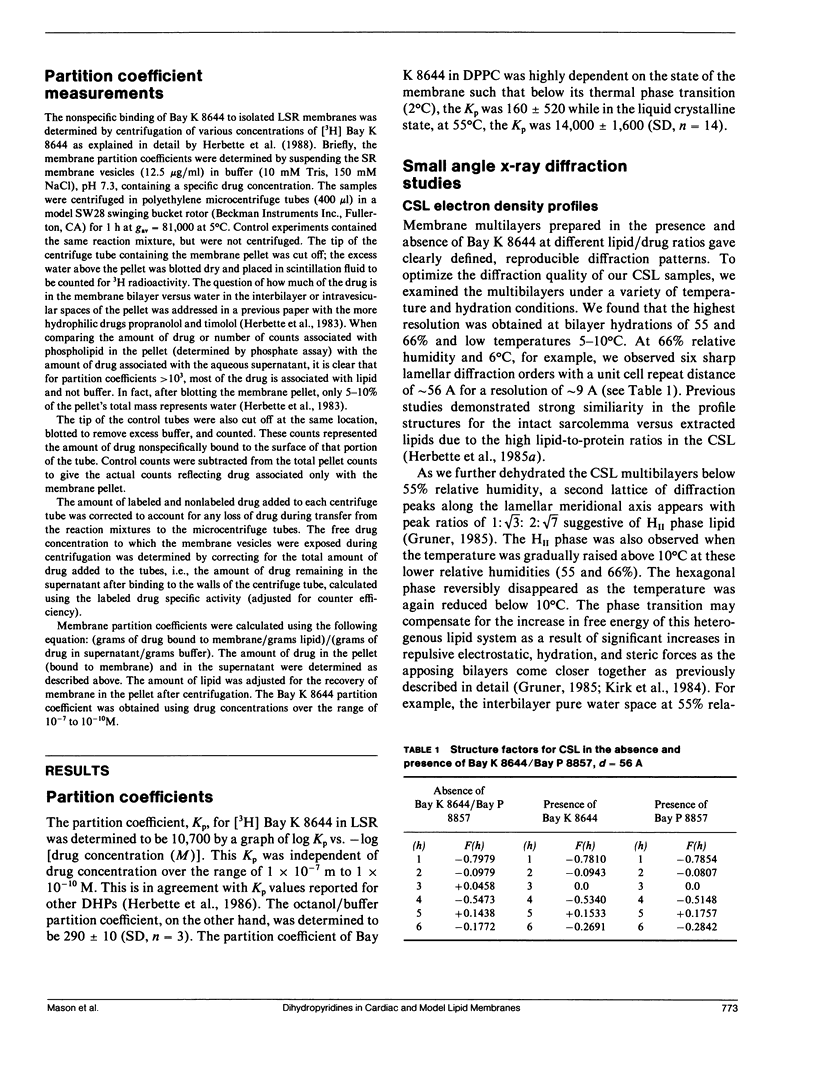
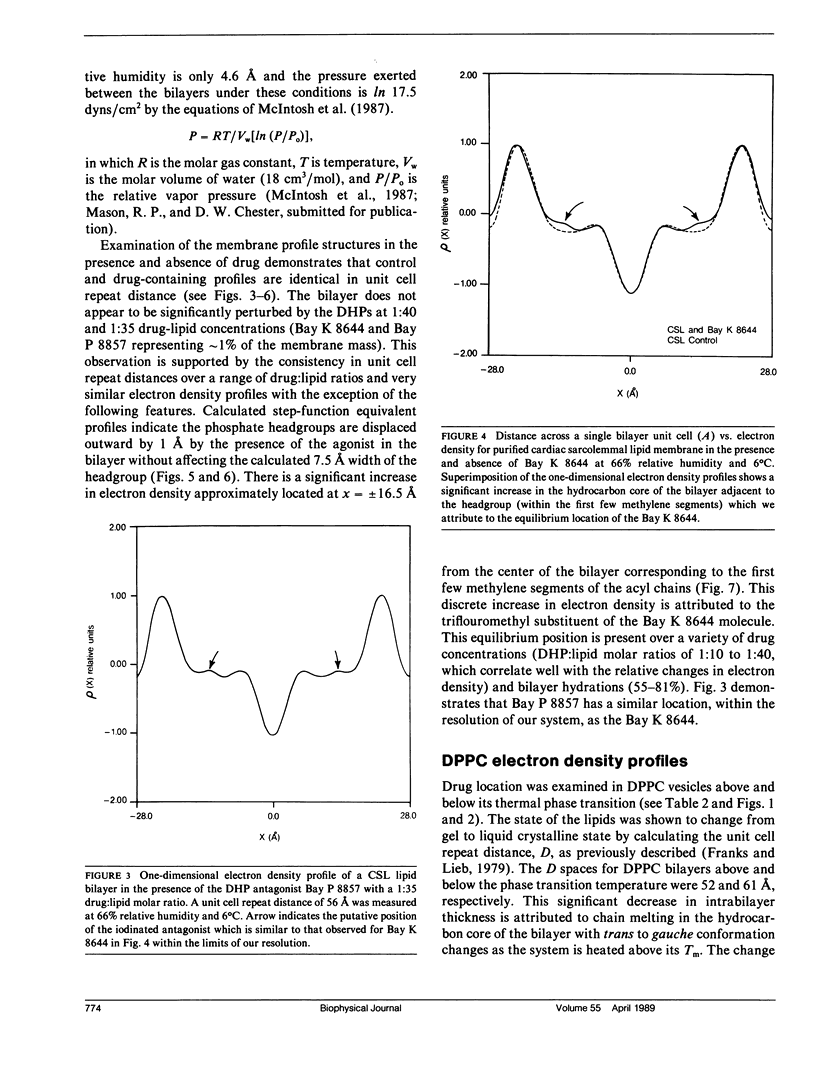
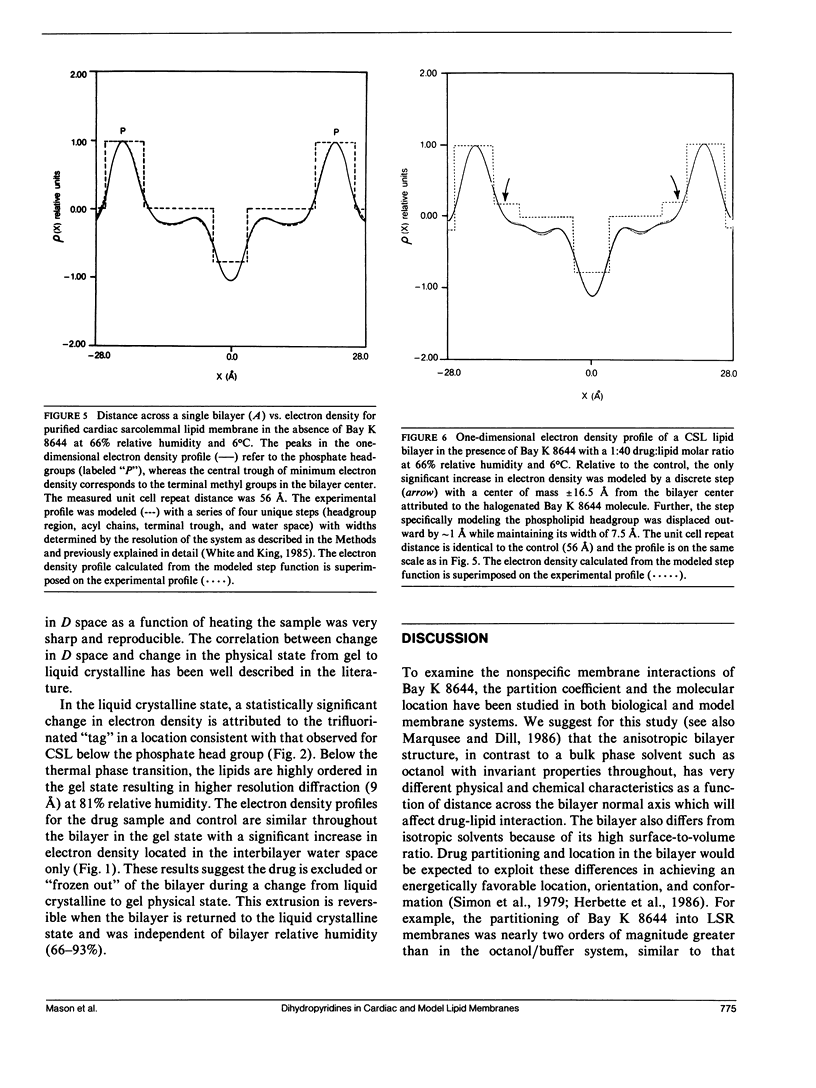
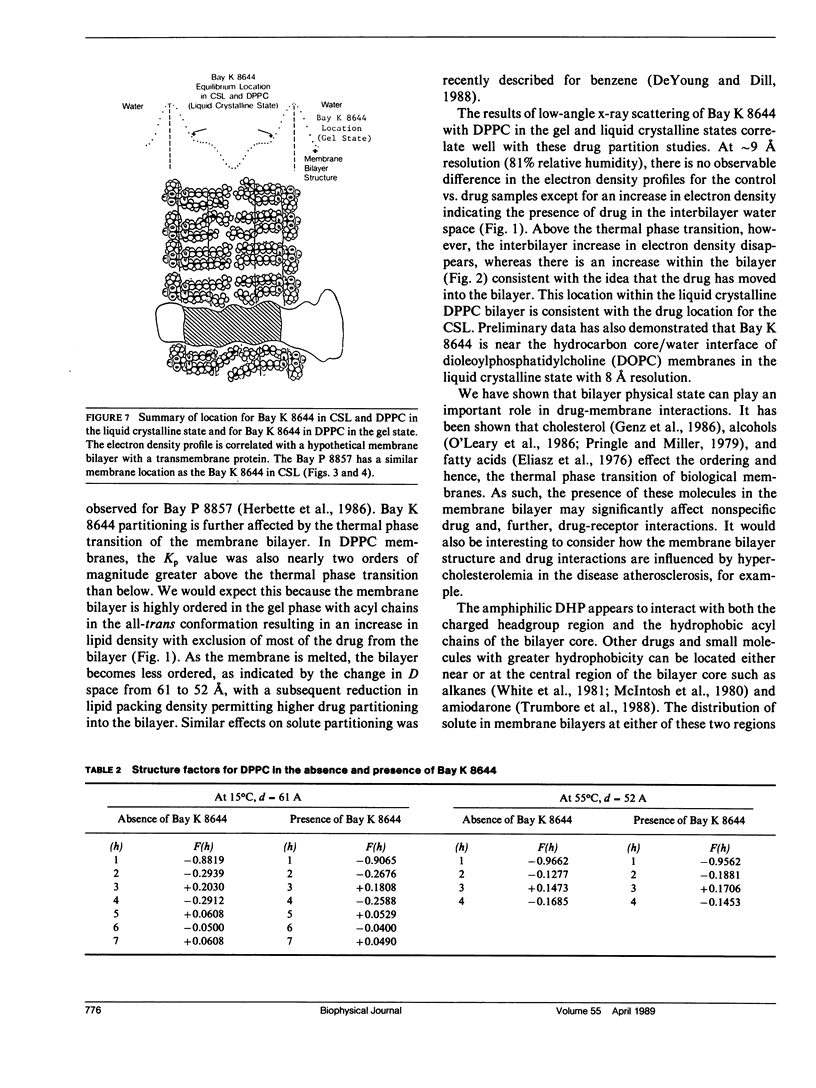
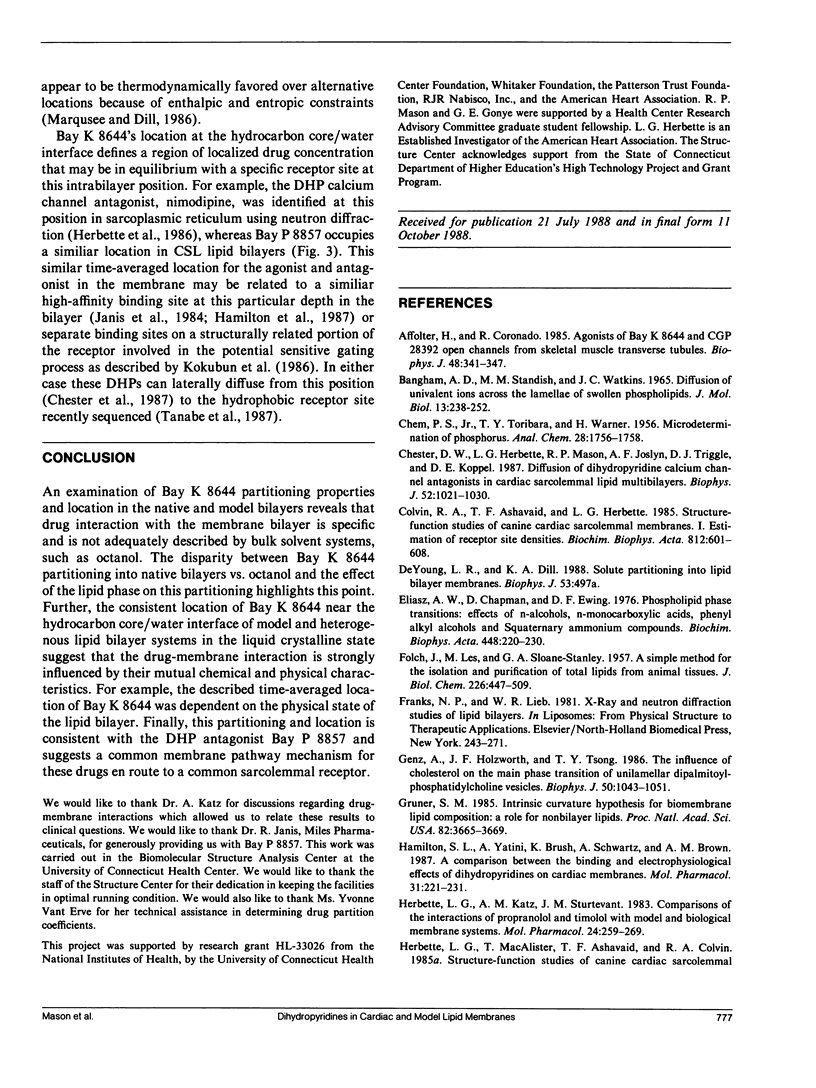
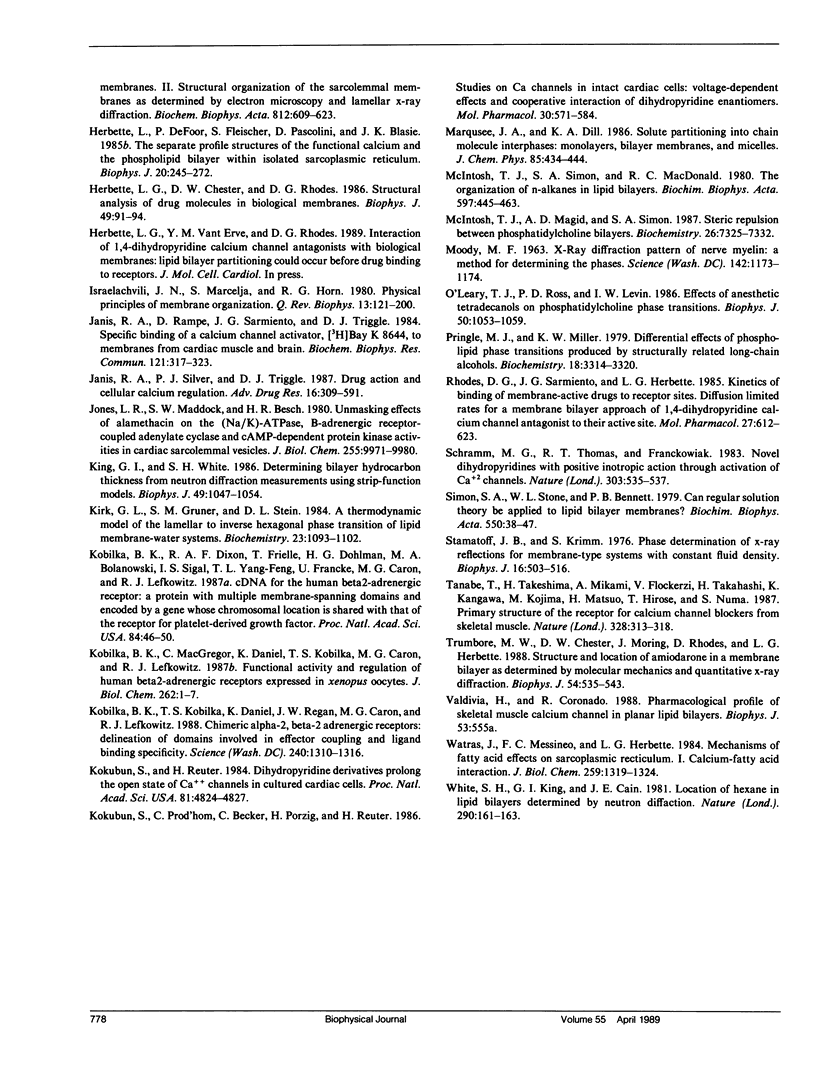
Selected References
These references are in PubMed. This may not be the complete list of references from this article.
- Affolter H., Coronado R. Agonists Bay-K8644 and CGP-28392 open calcium channels reconstituted from skeletal muscle transverse tubules. Biophys J. 1985 Aug;48(2):341–347. doi: 10.1016/S0006-3495(85)83789-9. [DOI] [PMC free article] [PubMed] [Google Scholar]
- Bangham A. D., Standish M. M., Watkins J. C. Diffusion of univalent ions across the lamellae of swollen phospholipids. J Mol Biol. 1965 Aug;13(1):238–252. doi: 10.1016/s0022-2836(65)80093-6. [DOI] [PubMed] [Google Scholar]
- Chester D. W., Herbette L. G., Mason R. P., Joslyn A. F., Triggle D. J., Koppel D. E. Diffusion of dihydropyridine calcium channel antagonists in cardiac sarcolemmal lipid multibilayers. Biophys J. 1987 Dec;52(6):1021–1030. doi: 10.1016/S0006-3495(87)83295-2. [DOI] [PMC free article] [PubMed] [Google Scholar]
- Colvin R. A., Ashavaid T. F., Herbette L. G. Structure-function studies of canine cardiac sarcolemmal membranes. I. Estimation of receptor site densities. Biochim Biophys Acta. 1985 Feb 14;812(3):601–608. doi: 10.1016/0005-2736(85)90253-6. [DOI] [PubMed] [Google Scholar]
- Eliasz A. W., Chapman D., Ewing D. F. Phospholipid phase transitions. Effects of n-alcohols, n-monocarboxylic acids, phenylalkyl alcohols and quaternary ammonium compounds. Biochim Biophys Acta. 1976 Oct 5;448(2):220–230. doi: 10.1016/0005-2736(76)90238-8. [DOI] [PubMed] [Google Scholar]
- FOLCH J., LEES M., SLOANE STANLEY G. H. A simple method for the isolation and purification of total lipides from animal tissues. J Biol Chem. 1957 May;226(1):497–509. [PubMed] [Google Scholar]
- Genz A., Holzwarth J. F., Tsong T. Y. The influence of cholesterol on the main phase transition of unilamellar dipalmytoylphosphatidylcholine vesicles. A differential scanning calorimetry and iodine laser T-jump study. Biophys J. 1986 Dec;50(6):1043–1051. doi: 10.1016/S0006-3495(86)83548-2. [DOI] [PMC free article] [PubMed] [Google Scholar]
- Gruner S. M. Intrinsic curvature hypothesis for biomembrane lipid composition: a role for nonbilayer lipids. Proc Natl Acad Sci U S A. 1985 Jun;82(11):3665–3669. doi: 10.1073/pnas.82.11.3665. [DOI] [PMC free article] [PubMed] [Google Scholar]
- Hamilton S. L., Yatani A., Brush K., Schwartz A., Brown A. M. A comparison between the binding and electrophysiological effects of dihydropyridines on cardiac membranes. Mol Pharmacol. 1987 Mar;31(3):221–231. [PubMed] [Google Scholar]
- Herbette L. G., Chester D. W., Rhodes D. G. Structural analysis of drug molecules in biological membranes. Biophys J. 1986 Jan;49(1):91–94. doi: 10.1016/S0006-3495(86)83605-0. [DOI] [PMC free article] [PubMed] [Google Scholar]
- Herbette L. G., MacAlister T., Ashavaid T. F., Colvin R. A. Structure-function studies of canine cardiac sarcolemmal membranes. II. Structural organization of the sarcolemmal membrane as determined by electron microscopy and lamellar X-ray diffraction. Biochim Biophys Acta. 1985 Feb 14;812(3):609–623. doi: 10.1016/0005-2736(85)90254-8. [DOI] [PubMed] [Google Scholar]
- Herbette L., Katz A. M., Sturtevant J. M. Comparisons of the interaction of propranolol and timolol with model and biological membrane systems. Mol Pharmacol. 1983 Sep;24(2):259–269. [PubMed] [Google Scholar]
- Herbette L., Marquardt J., Scarpa A., Blasie J. K. A direct analysis of lamellar x-ray diffraction from hydrated oriented multilayers of fully functional sarcoplasmic reticulum. Biophys J. 1977 Nov;20(2):245–272. doi: 10.1016/S0006-3495(77)85547-1. [DOI] [PMC free article] [PubMed] [Google Scholar]
- Israelachvili J. N., Marcelja S., Horn R. G. Physical principles of membrane organization. Q Rev Biophys. 1980 May;13(2):121–200. doi: 10.1017/s0033583500001645. [DOI] [PubMed] [Google Scholar]
- Janis R. A., Rampe D., Sarmiento J. G., Triggle D. J. Specific binding of a calcium channel activator, [3H]BAY k 8644, to membranes from cardiac muscle and brain. Biochem Biophys Res Commun. 1984 May 31;121(1):317–323. doi: 10.1016/0006-291x(84)90725-3. [DOI] [PubMed] [Google Scholar]
- Jones L. R., Maddock S. W., Besch H. R., Jr Unmasking effect of alamethicin on the (Na+,K+)-ATPase, beta-adrenergic receptor-coupled adenylate cyclase, and cAMP-dependent protein kinase activities of cardiac sarcolemmal vesicles. J Biol Chem. 1980 Oct 25;255(20):9971–9980. [PubMed] [Google Scholar]
- King G. I., White S. H. Determining bilayer hydrocarbon thickness from neutron diffraction measurements using strip-function models. Biophys J. 1986 May;49(5):1047–1054. doi: 10.1016/S0006-3495(86)83733-X. [DOI] [PMC free article] [PubMed] [Google Scholar]
- Kobilka B. K., Dixon R. A., Frielle T., Dohlman H. G., Bolanowski M. A., Sigal I. S., Yang-Feng T. L., Francke U., Caron M. G., Lefkowitz R. J. cDNA for the human beta 2-adrenergic receptor: a protein with multiple membrane-spanning domains and encoded by a gene whose chromosomal location is shared with that of the receptor for platelet-derived growth factor. Proc Natl Acad Sci U S A. 1987 Jan;84(1):46–50. doi: 10.1073/pnas.84.1.46. [DOI] [PMC free article] [PubMed] [Google Scholar]
- Kobilka B. K., Kobilka T. S., Daniel K., Regan J. W., Caron M. G., Lefkowitz R. J. Chimeric alpha 2-,beta 2-adrenergic receptors: delineation of domains involved in effector coupling and ligand binding specificity. Science. 1988 Jun 3;240(4857):1310–1316. doi: 10.1126/science.2836950. [DOI] [PubMed] [Google Scholar]
- Kokubun S., Prod'hom B., Becker C., Porzig H., Reuter H. Studies on Ca channels in intact cardiac cells: voltage-dependent effects and cooperative interactions of dihydropyridine enantiomers. Mol Pharmacol. 1986 Dec;30(6):571–584. [PubMed] [Google Scholar]
- Kokubun S., Reuter H. Dihydropyridine derivatives prolong the open state of Ca channels in cultured cardiac cells. Proc Natl Acad Sci U S A. 1984 Aug;81(15):4824–4827. doi: 10.1073/pnas.81.15.4824. [DOI] [PMC free article] [PubMed] [Google Scholar]
- MOODY M. F. X-RAY DIFFRACTION PATTERN OF NERVE MYELIN: A METHOD FOR DETERMINING THE PHASES. Science. 1963 Nov 29;142(3596):1173–1174. doi: 10.1126/science.142.3596.1173. [DOI] [PubMed] [Google Scholar]
- McIntosh T. J., Magid A. D., Simon S. A. Steric repulsion between phosphatidylcholine bilayers. Biochemistry. 1987 Nov 17;26(23):7325–7332. doi: 10.1021/bi00397a020. [DOI] [PubMed] [Google Scholar]
- McIntosh T. J., Simon S. A., MacDonald R. C. The organization of n-alkanes in lipid bilayers. Biochim Biophys Acta. 1980 Apr 24;597(3):445–463. doi: 10.1016/0005-2736(80)90219-9. [DOI] [PubMed] [Google Scholar]
- O'Leary T. J., Ross P. D., Levin I. W. Effects of anesthetic tetradecenols on phosphatidylcholine phase transitions. Implications for the mechanism of the bilayer pretransition. Biophys J. 1986 Dec;50(6):1053–1059. doi: 10.1016/S0006-3495(86)83549-4. [DOI] [PMC free article] [PubMed] [Google Scholar]
- Pringle M. J., Miller K. W. Differential effects on phospholipid phase transitions produced by structurally related long-chain alcohols. Biochemistry. 1979 Jul 24;18(15):3314–3320. doi: 10.1021/bi00582a018. [DOI] [PubMed] [Google Scholar]
- Rhodes D. G., Sarmiento J. G., Herbette L. G. Kinetics of binding of membrane-active drugs to receptor sites. Diffusion-limited rates for a membrane bilayer approach of 1,4-dihydropyridine calcium channel antagonists to their active site. Mol Pharmacol. 1985 Jun;27(6):612–623. [PubMed] [Google Scholar]
- Schramm M., Thomas G., Towart R., Franckowiak G. Novel dihydropyridines with positive inotropic action through activation of Ca2+ channels. Nature. 1983 Jun 9;303(5917):535–537. doi: 10.1038/303535a0. [DOI] [PubMed] [Google Scholar]
- Simon S. A., Stone W. L., Bennett P. B. Can regular solution theory be applied to lipid bilayer membranes? Biochim Biophys Acta. 1979 Jan 5;550(1):38–47. doi: 10.1016/0005-2736(79)90113-5. [DOI] [PubMed] [Google Scholar]
- Stamatoff J. B., Krimm S. Phase determination of x-ray reflections for membrane-type systems with constant fluid density. Biophys J. 1976 May;16(5):503–516. doi: 10.1016/S0006-3495(76)85705-0. [DOI] [PMC free article] [PubMed] [Google Scholar]
- Tanabe T., Takeshima H., Mikami A., Flockerzi V., Takahashi H., Kangawa K., Kojima M., Matsuo H., Hirose T., Numa S. Primary structure of the receptor for calcium channel blockers from skeletal muscle. Nature. 1987 Jul 23;328(6128):313–318. doi: 10.1038/328313a0. [DOI] [PubMed] [Google Scholar]
- Trumbore M., Chester D. W., Moring J., Rhodes D., Herbette L. G. Structure and location of amiodarone in a membrane bilayer as determined by molecular mechanics and quantitative x-ray diffraction. Biophys J. 1988 Sep;54(3):535–543. doi: 10.1016/S0006-3495(88)82986-2. [DOI] [PMC free article] [PubMed] [Google Scholar]
- Watras J., Messineo F. C., Herbette L. G. Mechanisms of fatty acid effects on sarcoplasmic reticulum. I. Calcium-fatty acid interaction. J Biol Chem. 1984 Jan 25;259(2):1319–1324. [PubMed] [Google Scholar]