Abstract
The voltage dependence of amiloride-induced inhibition of current flow through apical membrane sodium channels in toad urinary bladder was studied at different ionic conditions. The "inert" salt N-methyl-D-glucamine HCl (NMDG HCl) affected neither the apparent inhibition constant (Kl) for the amiloride-induced current inhibition nor the apparent fraction of the transmembrane voltage that falls between the mucosal solution and the amiloride-binding site (delta). When NMDG+ was replaced with Na+, Kl increased, reflecting amiloride-Na+ competition, whereas delta was unchanged. Similar results were obtained with another permeant cation, Li+. When NMDG+ was replaced by K+, an impermeant but channel-blocking cation, Kl increased whereas delta decreased. Similar results were obtained using another impermeant, channel-blocking cation guanidinium. The results are interpreted on the premise that Na+ and K+ compete with amiloride by binding to cation binding sites within the channel lumen such that ion occupancy of these sites vary with voltage. Occupancy by K+, which cannot traverse the channel, will increase as the mucosal solution becomes positive, relative to the serosal solution. Occupancy by Na+, which can traverse the channel, is comparatively voltage independent. Ion movement through the channels was simulated using discrete-state kinetic models. Two types of models could describe the shape of the current-voltage relationship and the voltage dependence of the amiloride-induced channel block. One model had a single ion-binding site with a broad energy barrier at the inner (cytoplasmic) side of the site. The other model had two binding sites separated from each other and from the aqueous solutions by sharp energy barriers.
Full text
PDF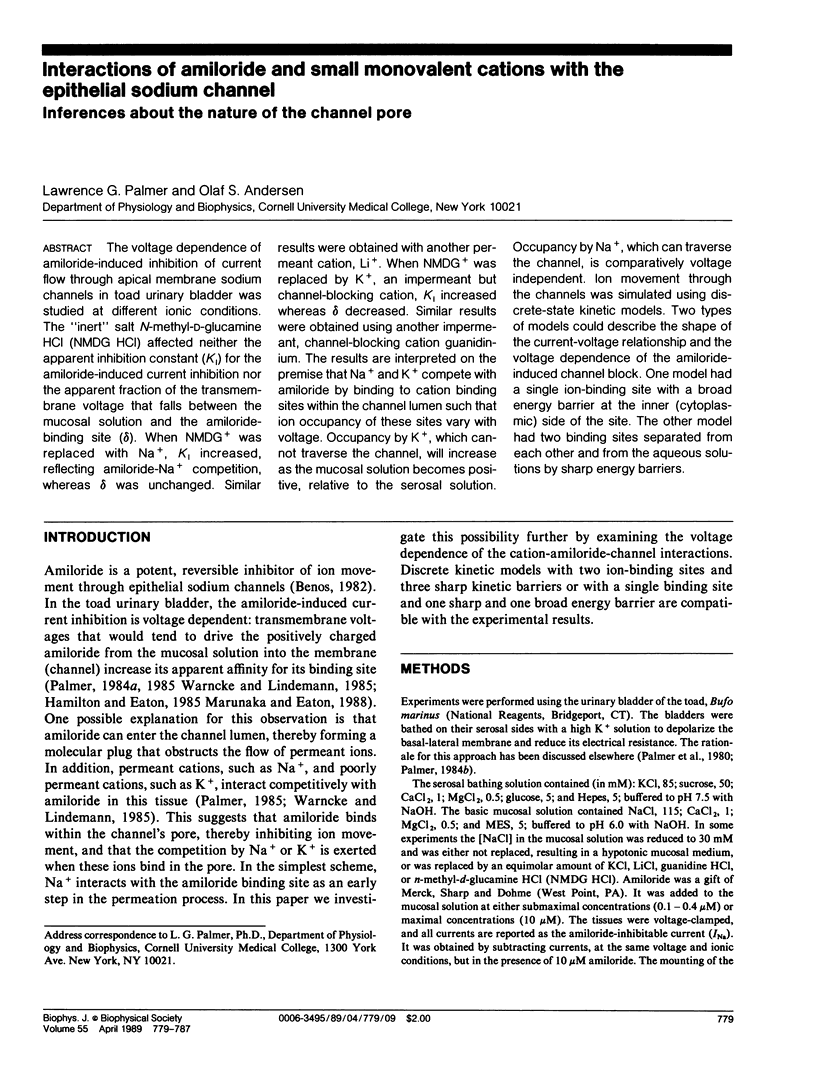
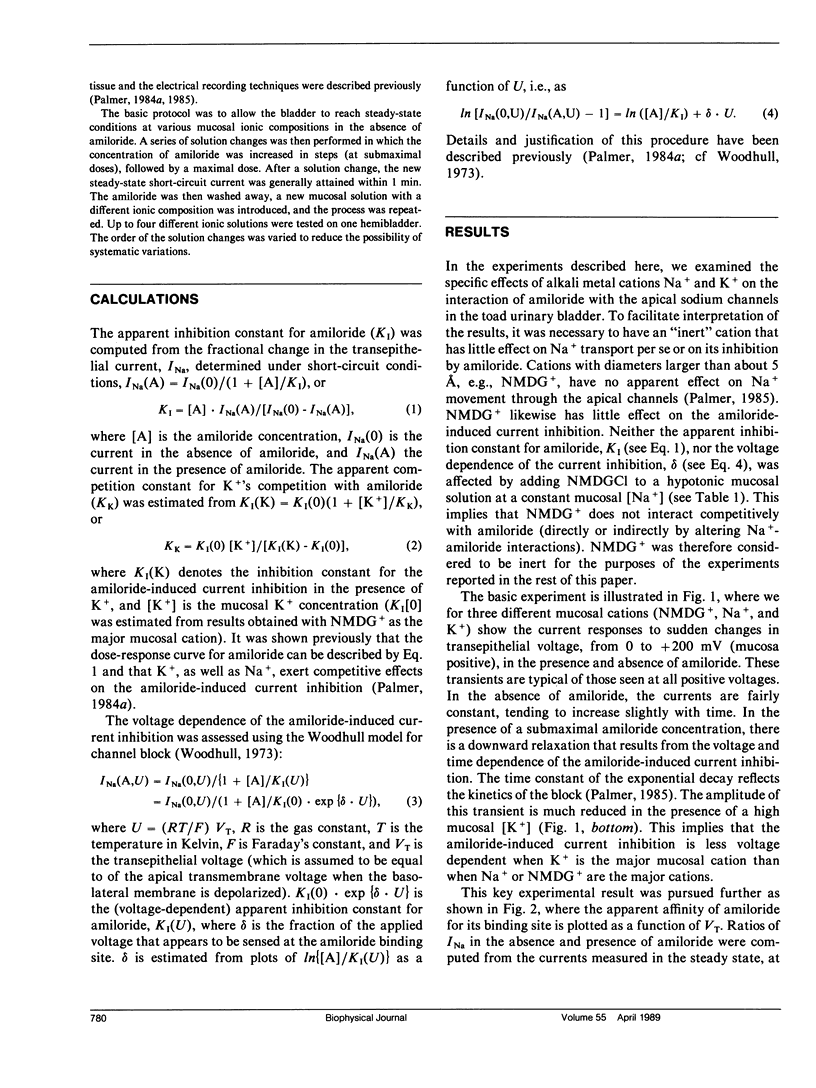
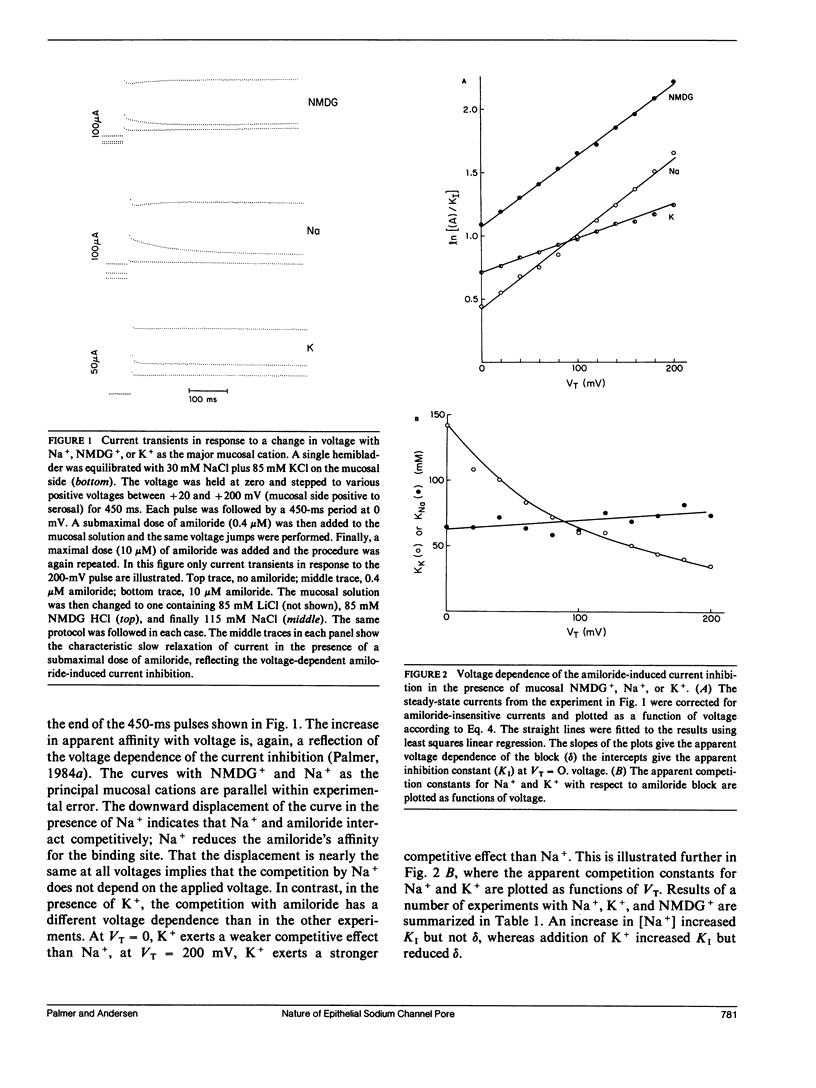
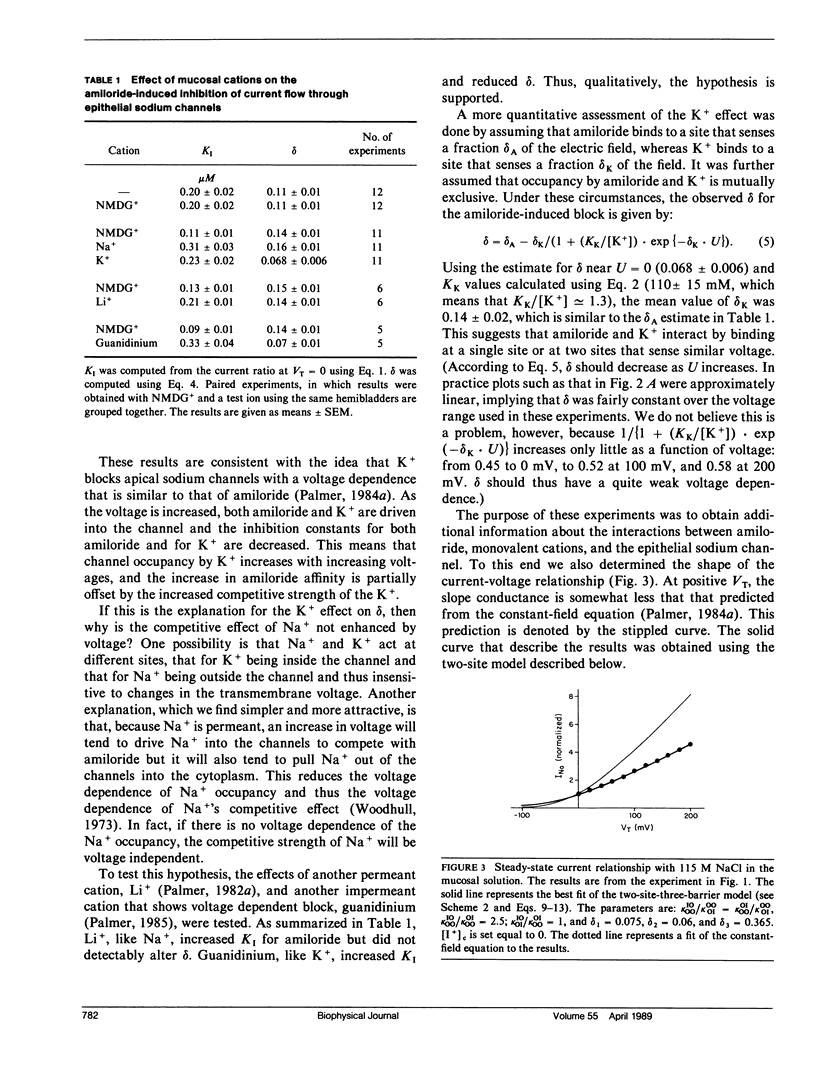
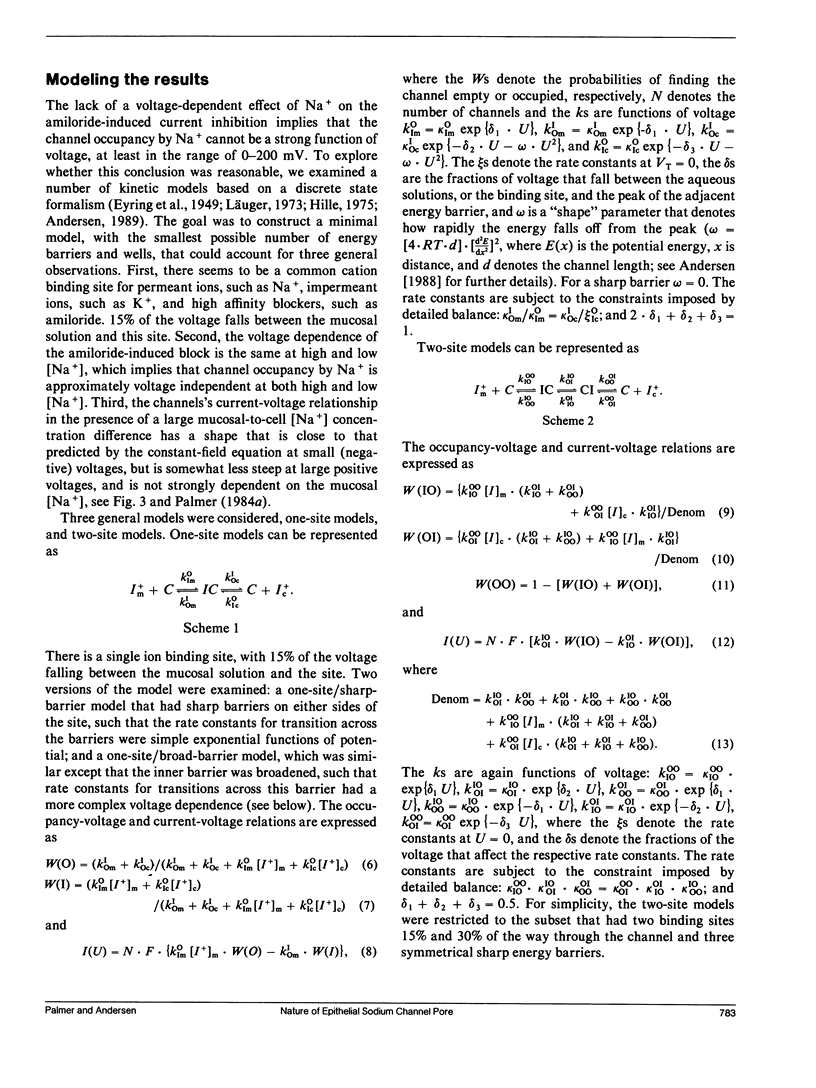
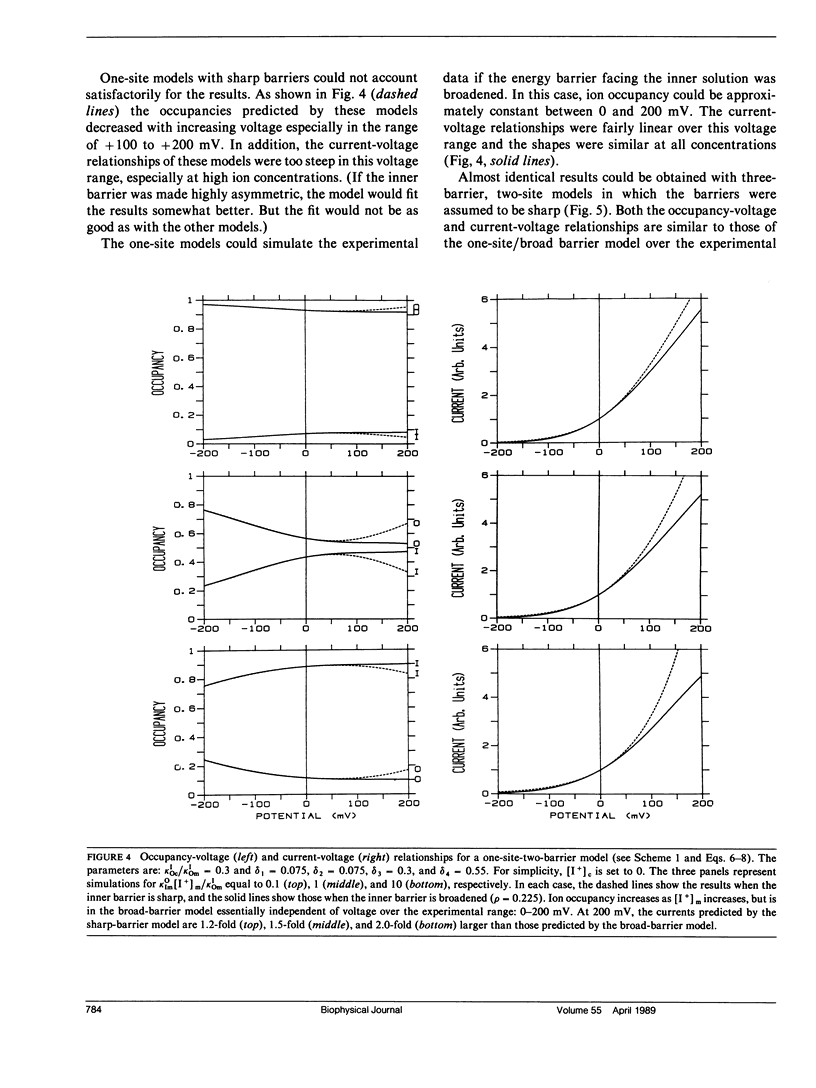
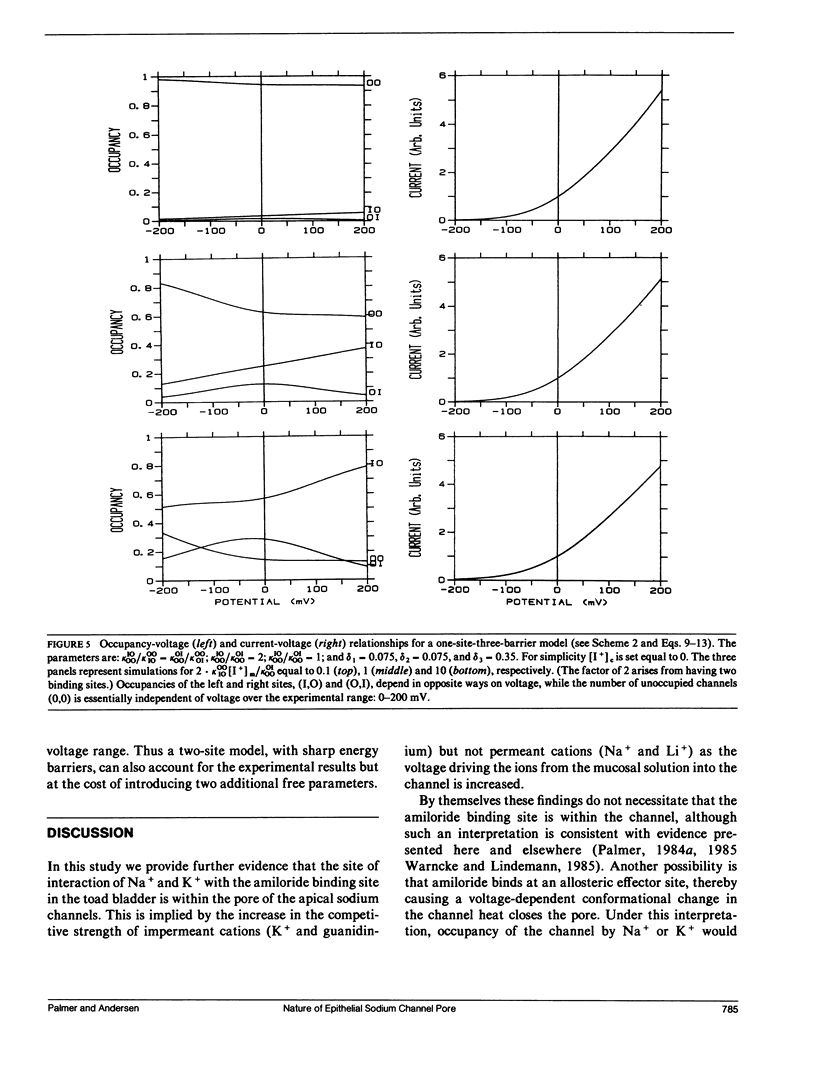
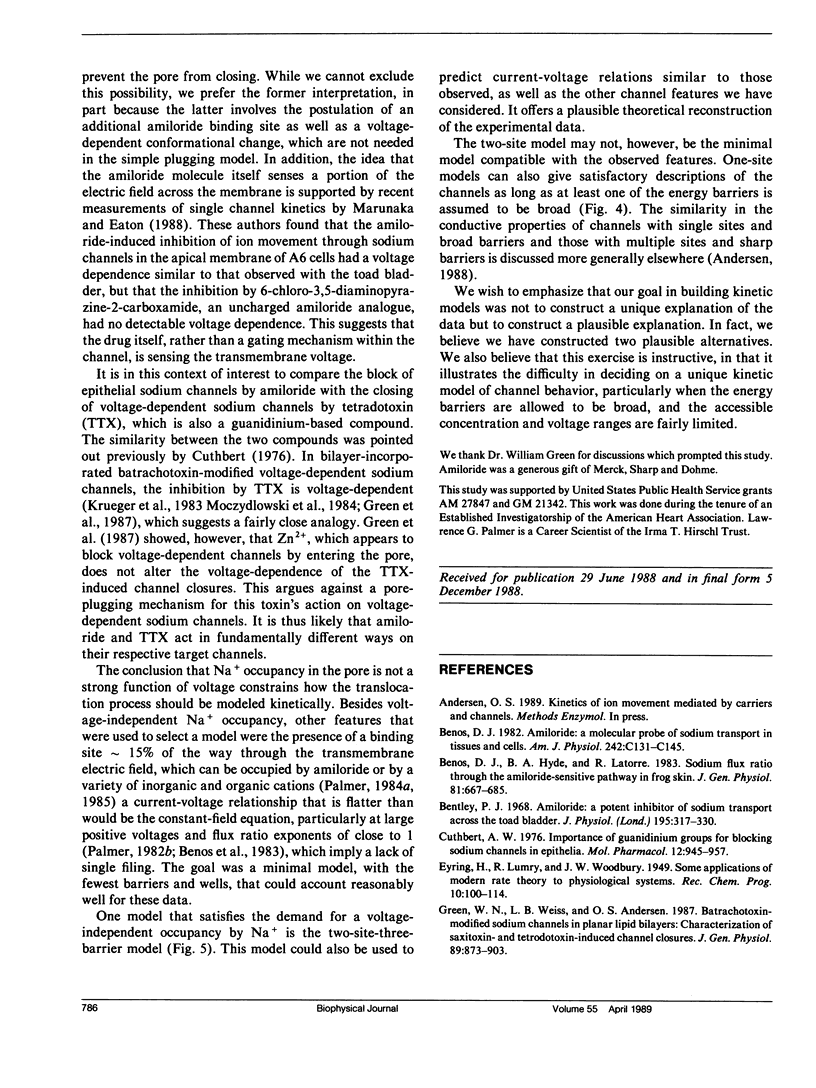
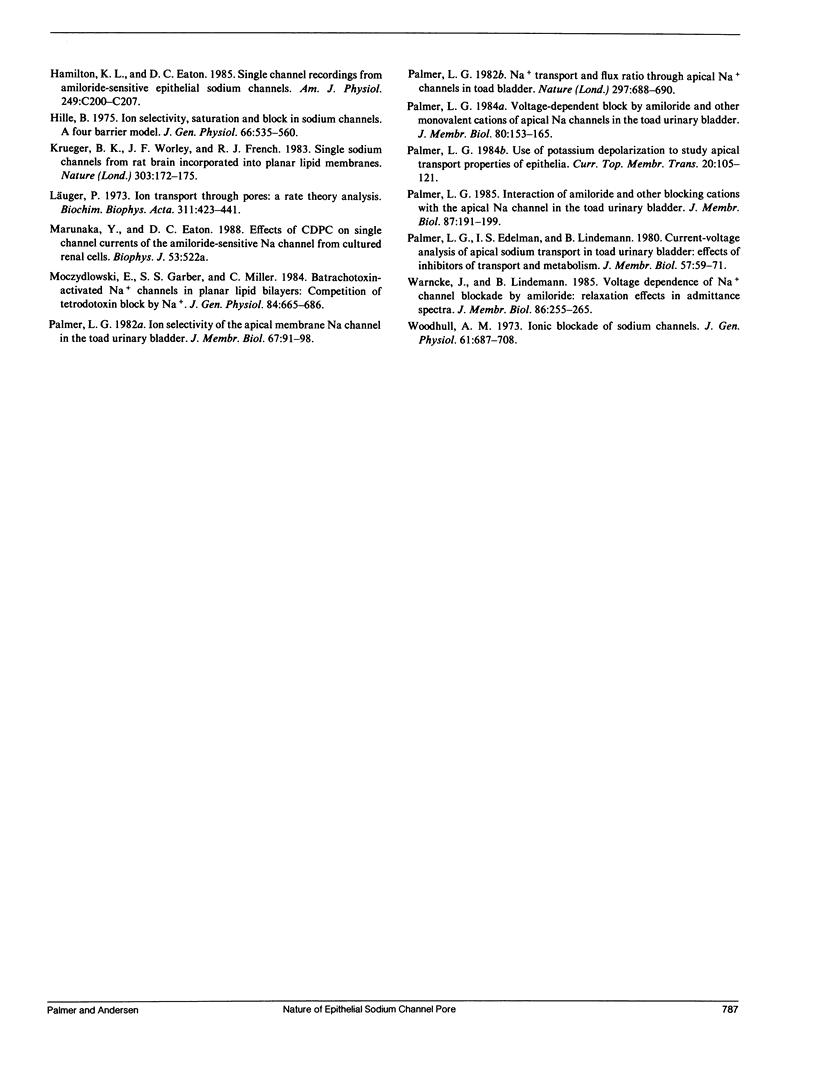
Selected References
These references are in PubMed. This may not be the complete list of references from this article.
- Benos D. J. Amiloride: a molecular probe of sodium transport in tissues and cells. Am J Physiol. 1982 Mar;242(3):C131–C145. doi: 10.1152/ajpcell.1982.242.3.C131. [DOI] [PubMed] [Google Scholar]
- Benos D. J., Hyde B. A., Latorre R. Sodium flux ratio through the amiloride-sensitive entry pathway in frog skin. J Gen Physiol. 1983 May;81(5):667–685. doi: 10.1085/jgp.81.5.667. [DOI] [PMC free article] [PubMed] [Google Scholar]
- Bentley P. J. Amiloride: a potent inhibitor of sodium transport across the toad bladder. J Physiol. 1968 Mar;195(2):317–330. doi: 10.1113/jphysiol.1968.sp008460. [DOI] [PMC free article] [PubMed] [Google Scholar]
- Cuthbert A. W. Importance of guanidinium groups of blocking sodium channels in epithelia. Mol Pharmacol. 1976 Nov;12(6):945–957. [PubMed] [Google Scholar]
- Green W. N., Weiss L. B., Andersen O. S. Batrachotoxin-modified sodium channels in planar lipid bilayers. Characterization of saxitoxin- and tetrodotoxin-induced channel closures. J Gen Physiol. 1987 Jun;89(6):873–903. doi: 10.1085/jgp.89.6.873. [DOI] [PMC free article] [PubMed] [Google Scholar]
- Hamilton K. L., Eaton D. C. Single-channel recordings from amiloride-sensitive epithelial sodium channel. Am J Physiol. 1985 Sep;249(3 Pt 1):C200–C207. doi: 10.1152/ajpcell.1985.249.3.C200. [DOI] [PubMed] [Google Scholar]
- Hille B. Ionic selectivity, saturation, and block in sodium channels. A four-barrier model. J Gen Physiol. 1975 Nov;66(5):535–560. doi: 10.1085/jgp.66.5.535. [DOI] [PMC free article] [PubMed] [Google Scholar]
- Krueger B. K., Worley J. F., 3rd, French R. J. Single sodium channels from rat brain incorporated into planar lipid bilayer membranes. Nature. 1983 May 12;303(5913):172–175. doi: 10.1038/303172a0. [DOI] [PubMed] [Google Scholar]
- Läuger P. Ion transport through pores: a rate-theory analysis. Biochim Biophys Acta. 1973 Jul 6;311(3):423–441. doi: 10.1016/0005-2736(73)90323-4. [DOI] [PubMed] [Google Scholar]
- Moczydlowski E., Garber S. S., Miller C. Batrachotoxin-activated Na+ channels in planar lipid bilayers. Competition of tetrodotoxin block by Na+. J Gen Physiol. 1984 Nov;84(5):665–686. doi: 10.1085/jgp.84.5.665. [DOI] [PMC free article] [PubMed] [Google Scholar]
- Palmer L. G., Edelman I. S., Lindemann B. Current-voltage analysis of apical sodium transport in toad urinary bladder: effects of inhibitors of transport and metabolism. J Membr Biol. 1980 Nov 15;57(1):59–71. doi: 10.1007/BF01868986. [DOI] [PubMed] [Google Scholar]
- Palmer L. G. Interactions of amiloride and other blocking cations with the apical Na channel in the toad urinary bladder. J Membr Biol. 1985;87(3):191–199. doi: 10.1007/BF01871218. [DOI] [PubMed] [Google Scholar]
- Palmer L. G. Ion selectivity of the apical membrane Na channel in the toad urinary bladder. J Membr Biol. 1982;67(2):91–98. doi: 10.1007/BF01868651. [DOI] [PubMed] [Google Scholar]
- Palmer L. G. Na+ transport and flux ratio through apical Na+ channels in toad bladder. Nature. 1982 Jun 24;297(5868):688–690. doi: 10.1038/297688a0. [DOI] [PubMed] [Google Scholar]
- Palmer L. G. Voltage-dependent block by amiloride and other monovalent cations of apical Na channels in the toad urinary bladder. J Membr Biol. 1984;80(2):153–165. doi: 10.1007/BF01868771. [DOI] [PubMed] [Google Scholar]
- Warncke J., Lindemann B. Voltage dependence of Na channel blockage by amiloride: relaxation effects in admittance spectra. J Membr Biol. 1985;86(3):255–265. doi: 10.1007/BF01870605. [DOI] [PubMed] [Google Scholar]
- Woodhull A. M. Ionic blockage of sodium channels in nerve. J Gen Physiol. 1973 Jun;61(6):687–708. doi: 10.1085/jgp.61.6.687. [DOI] [PMC free article] [PubMed] [Google Scholar]