Abstract
1. Two symmetrical giant neurones located in the cerebral ganglion of Aplysia californica contain 4-6 p-mole 5-hydroxytryptamine (5-HT) and are able to synthesize it (Weinreich, McCaman, McCaman & Vaughn, 1973; Eisenstadt, Goldman, Kandel, Koike, Koester & Schwartz, 1973). Stimulation of each of these neurones evokes excitatory and inhibitory potentials in various cells of the ipsilateral buccal ganglion. In nine buccal neurones it evokes excitatory potentials, in other three, `classical' inhibitory potentials and in one neurone an `atypical' inhibitory potential.
2. The connexion between the giant cerebral neurone and the cells receiving either an excitatory or a `classical' inhibitory input from it are monosynaptic. TEA injection into the cerebral giant neurone, which prolongs the presynaptic spike, causes a gradual increase of both the excitatory and the inhibitory potentials. On the other hand, high Ca2+ media, which block polysynaptic pathways, do not suppress these synaptic potentials.
3. The iontophoretic application of 5-HT to the buccal neurones receiving excitatory input from the giant cerebral neurones evokes depolarizations showing the pharmacological properties of both A- and A′-responses to 5-HT (see preceding paper). Antagonists which block only the A-receptors (curare, 7-methyltryptamine, LSD 25) block partially the synaptic depolarizing potentials. Bufotenine, which blocks both the A- and A′-receptors, completely blocks the excitatory potentials. Thus, the post-synaptic membrane of these buccal neurones appears to be endowed with both A- and A′-receptors to 5-HT.
4. The `classical' inhibitory potentials elicited in three buccal neurones are hyperpolarizations which reverse at — 80 mV and are due to an increase in K+-conductance. The iontophoretic application of 5-HT to these post-synaptic neurones evokes hyperpolarizing B-responses which are also generated by an increase in K+-conductance. Antagonists which block the B-responses (bufotenine, methoxygramine) also block the inhibitory potentials.
5. The `atypical' inhibitory potential evoked in one buccal neurone consists in an hyperpolarization which increases in amplitude with cell hyperpolarization. Iontophoretic application of 5-HT to this buccal cell evokes an hyperpolarizing β-response which also increases in amplitude with cell polarization and results from a decrease in both Na+- and K+- conductances. The monosynaptic character of the `atypical' inhibitory potential is not yet fully proven.
6. It can be concluded that the excitatory and inhibitory synaptic effects evoked in the buccal neurones by the stimulation of the 5-HT-containing-giant cerebral neurones are very likely mediated by 5-HT.
Full text
PDF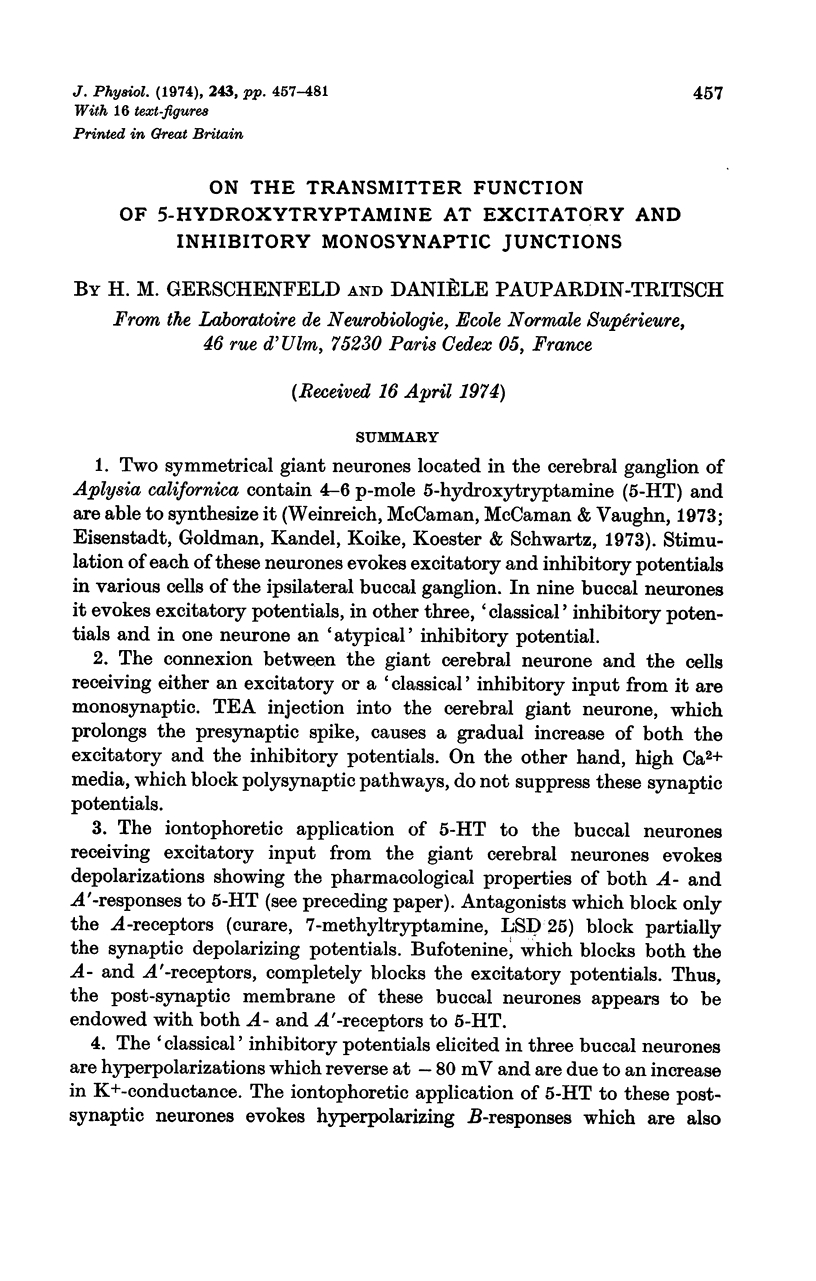
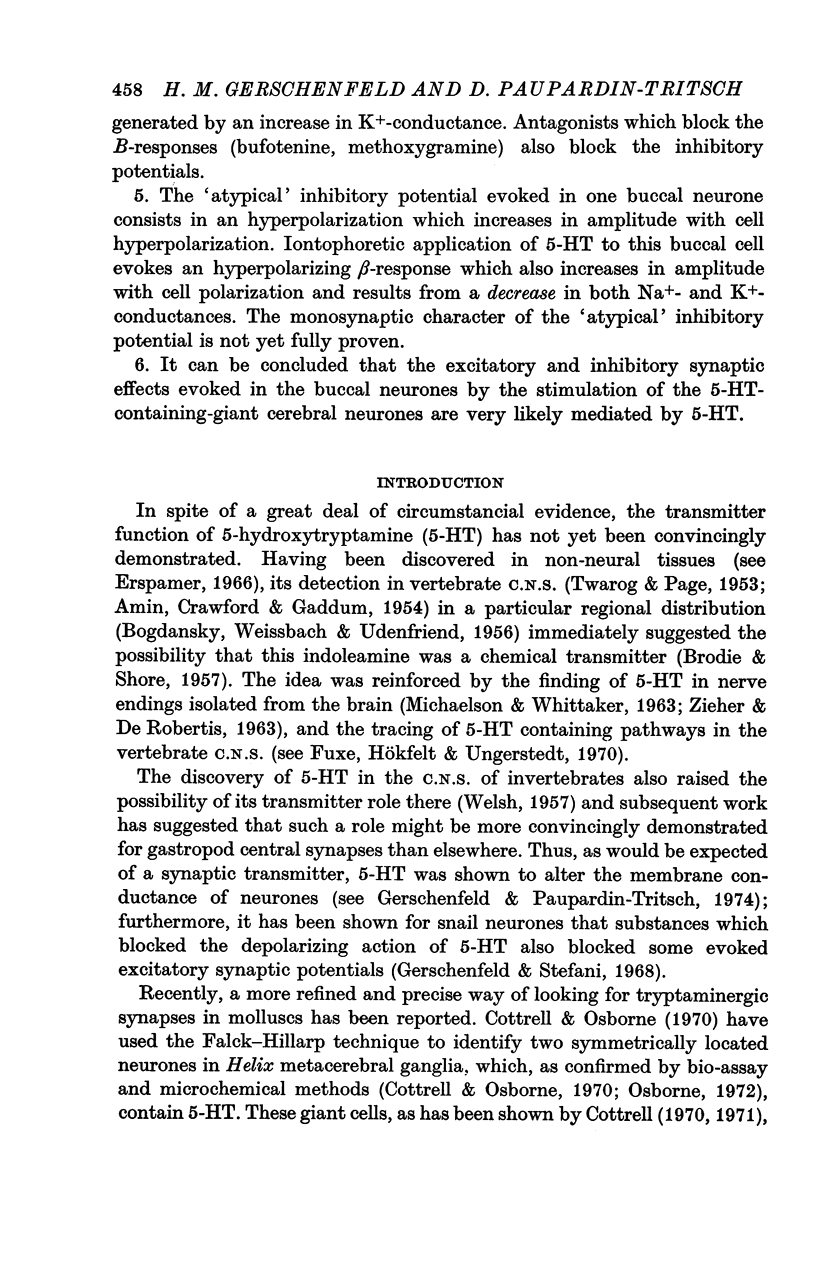
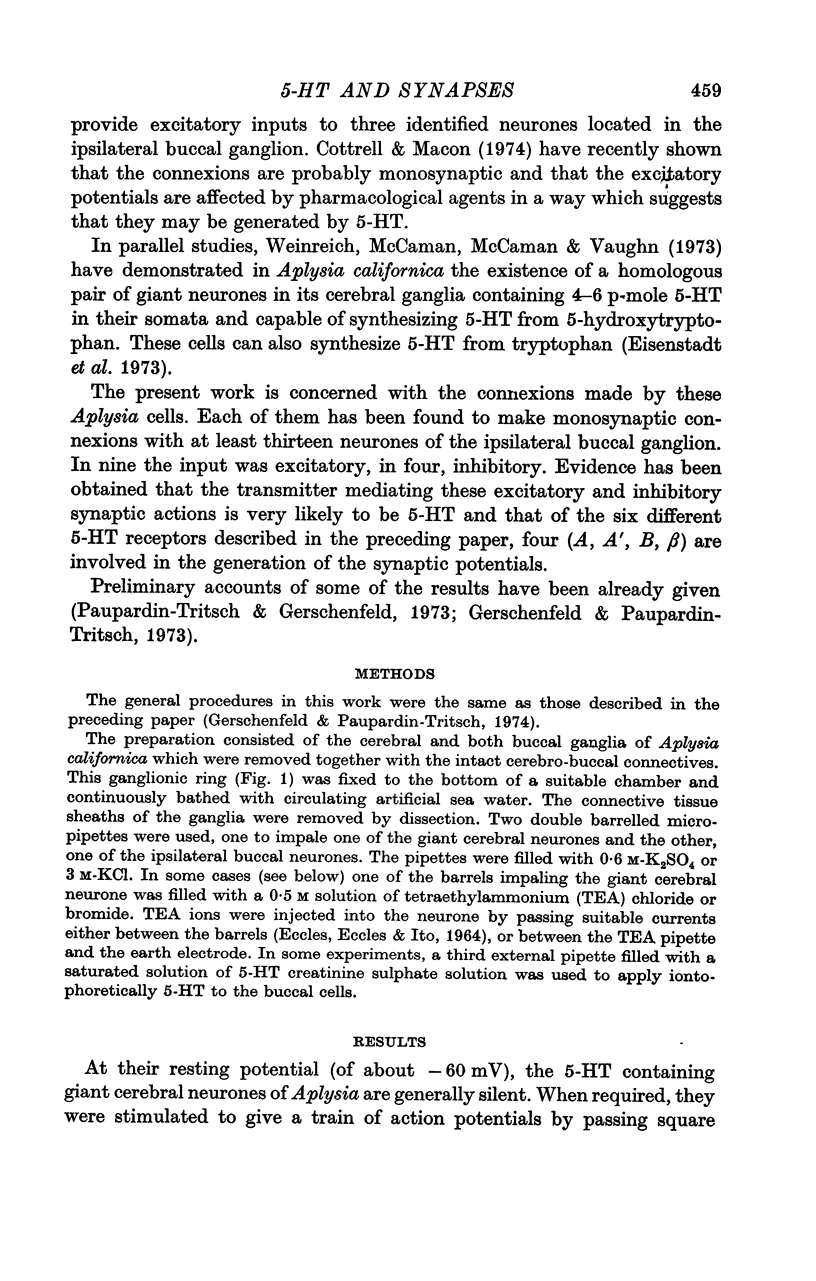
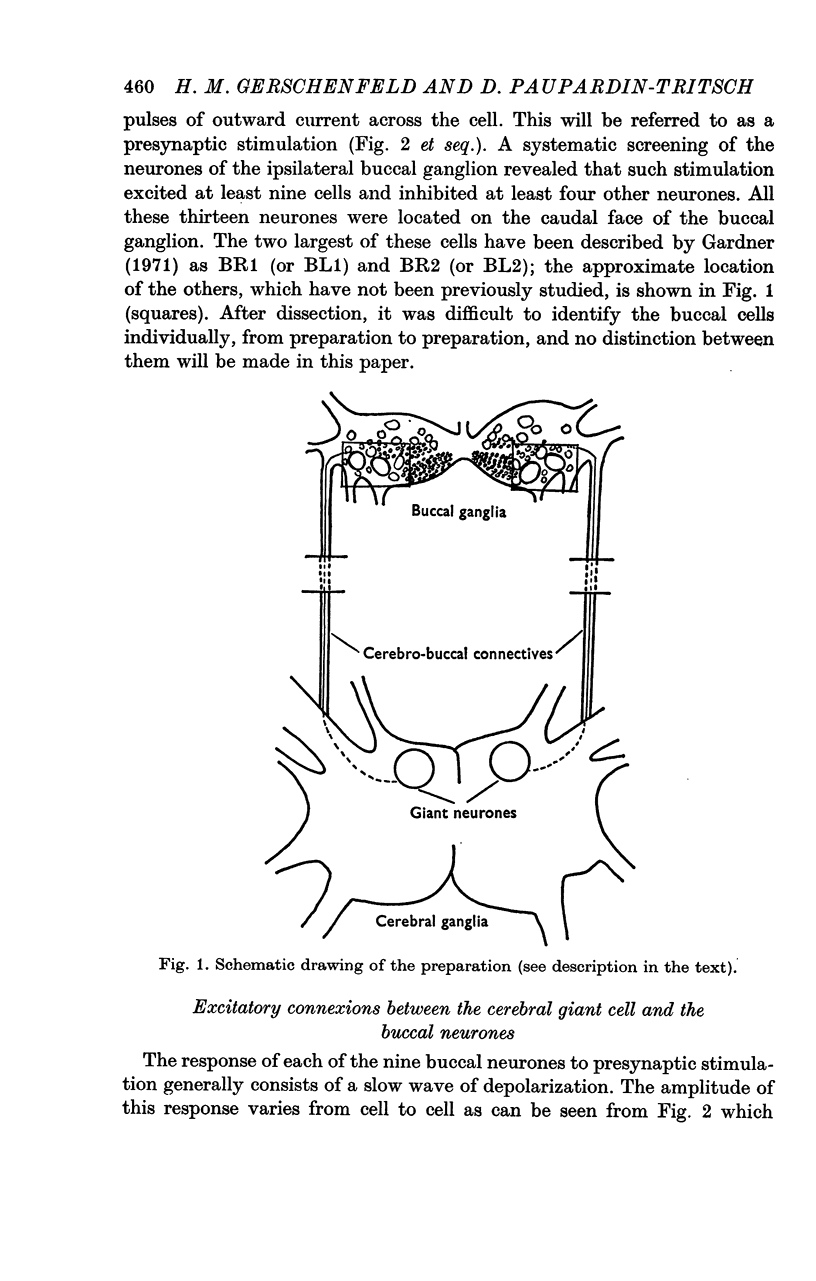
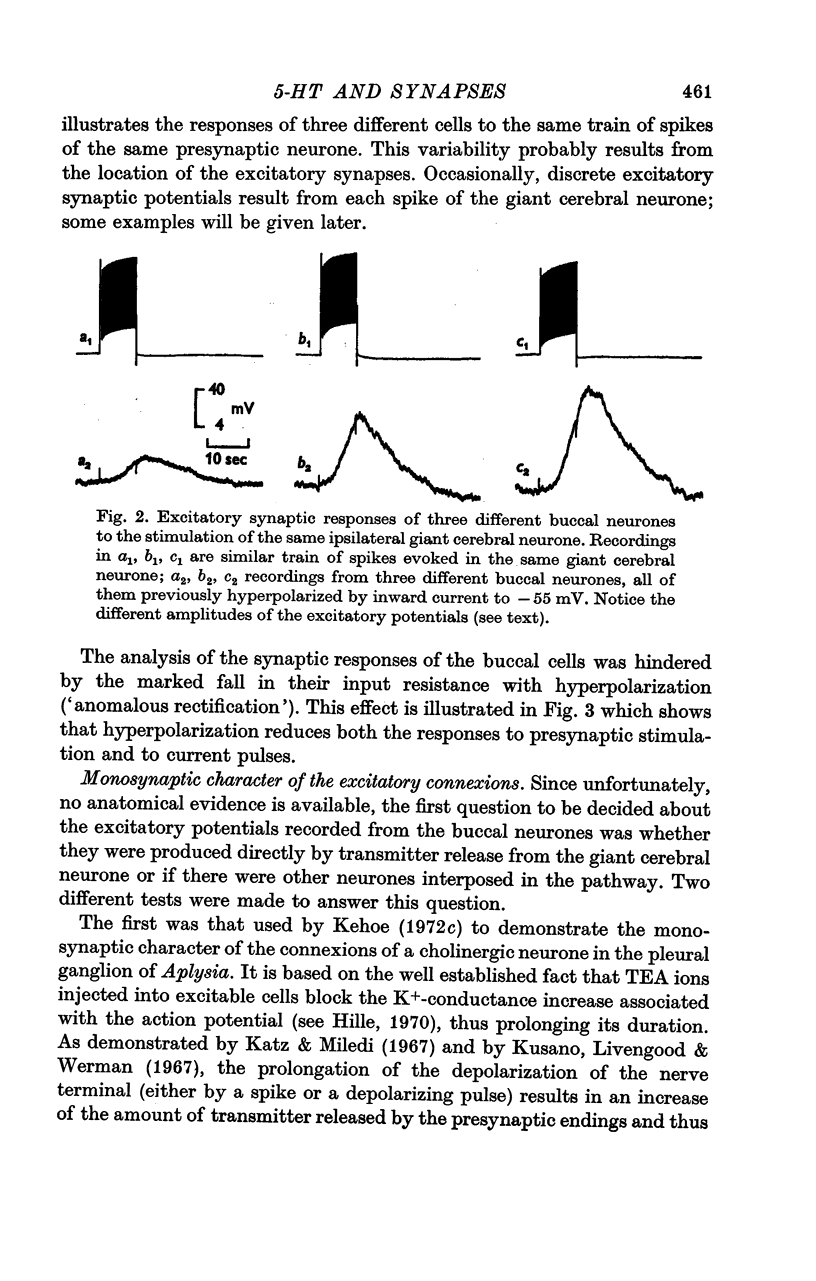
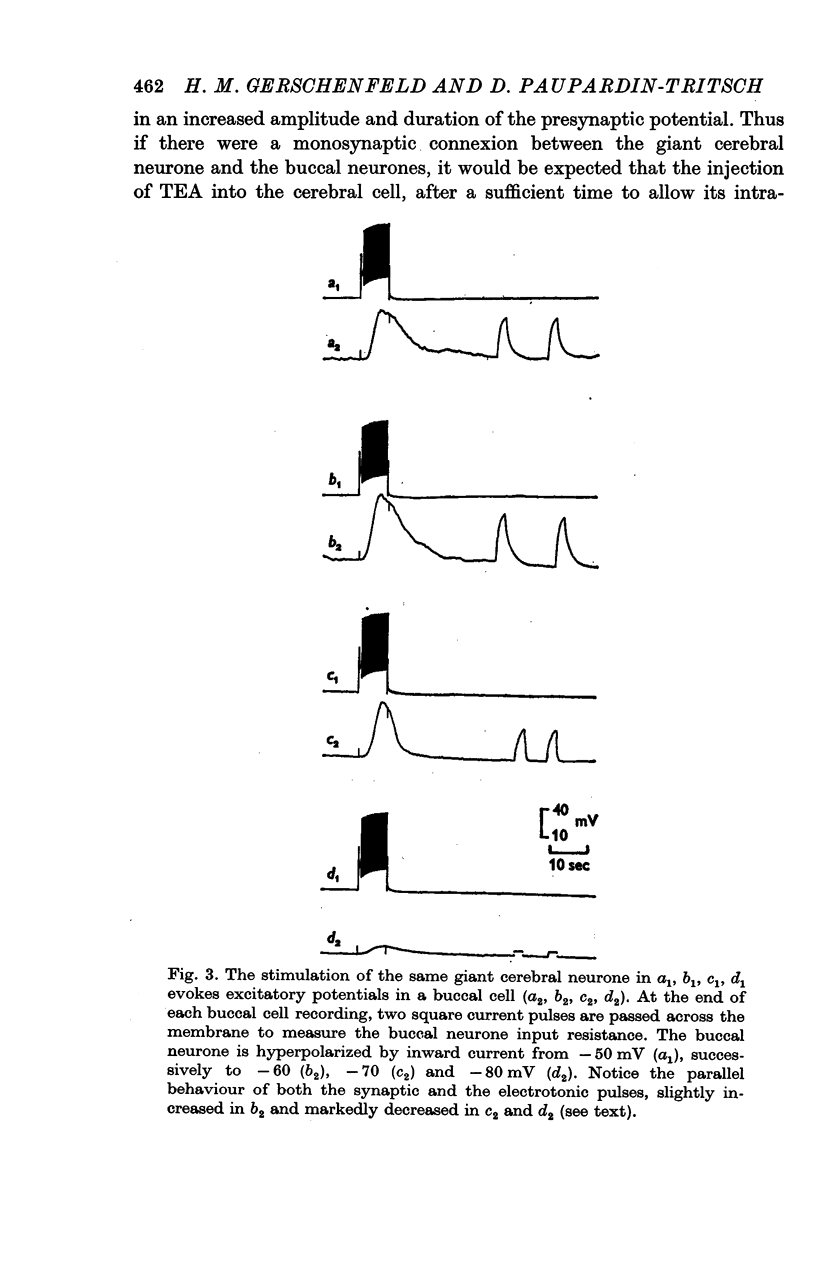
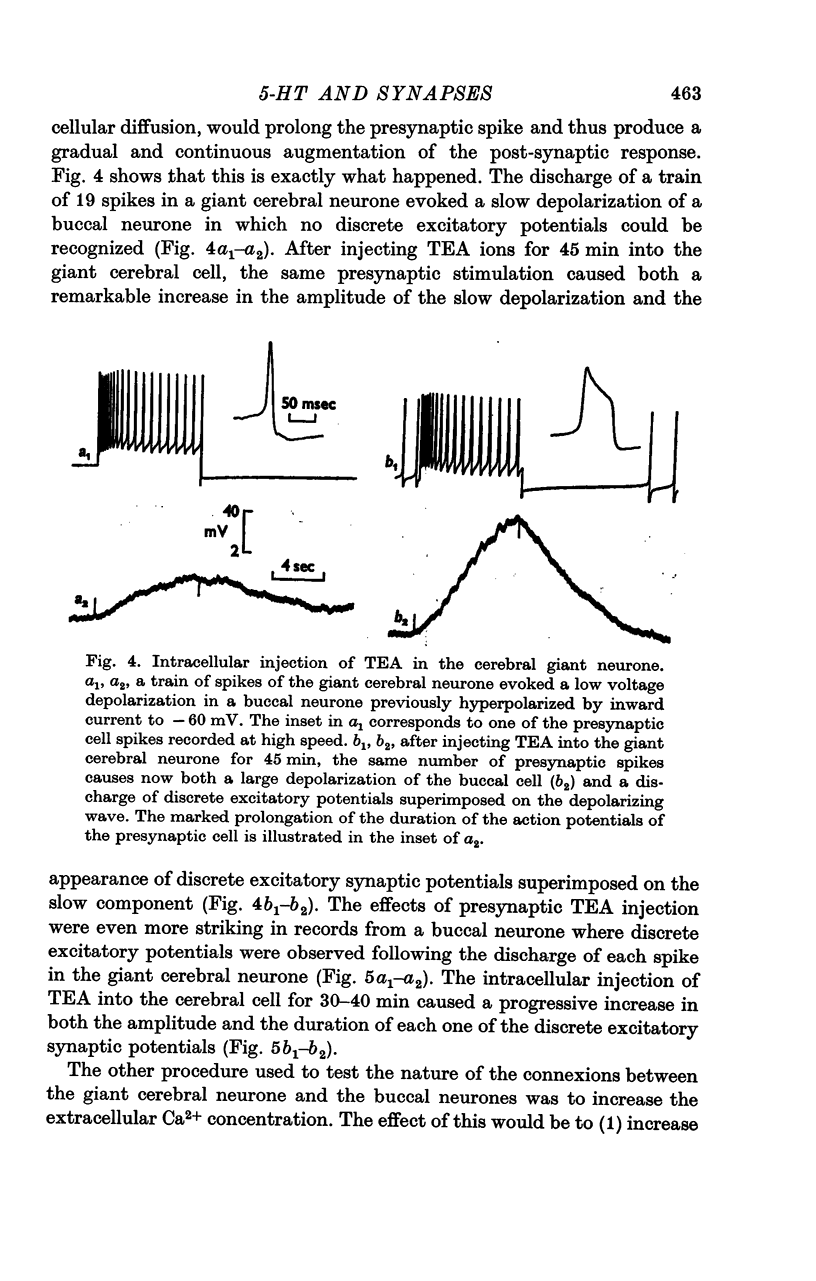
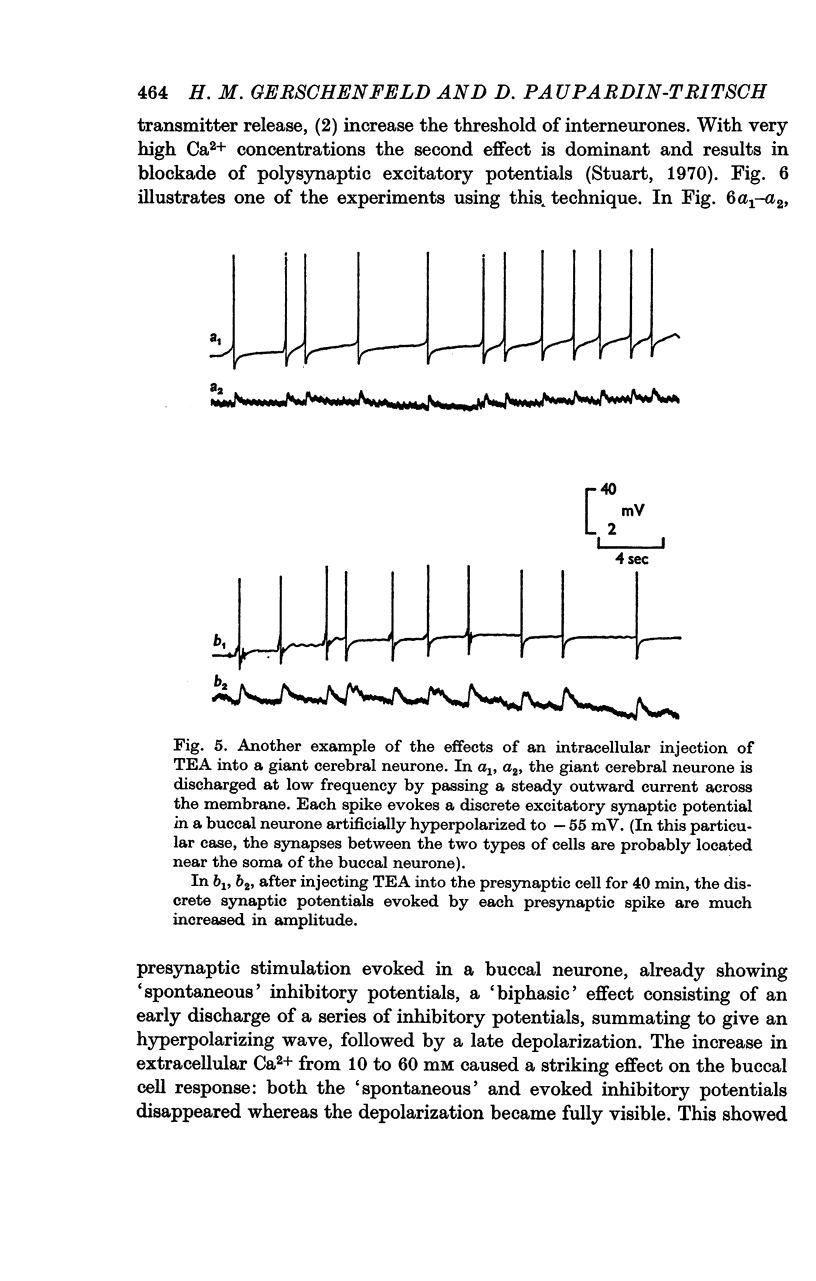
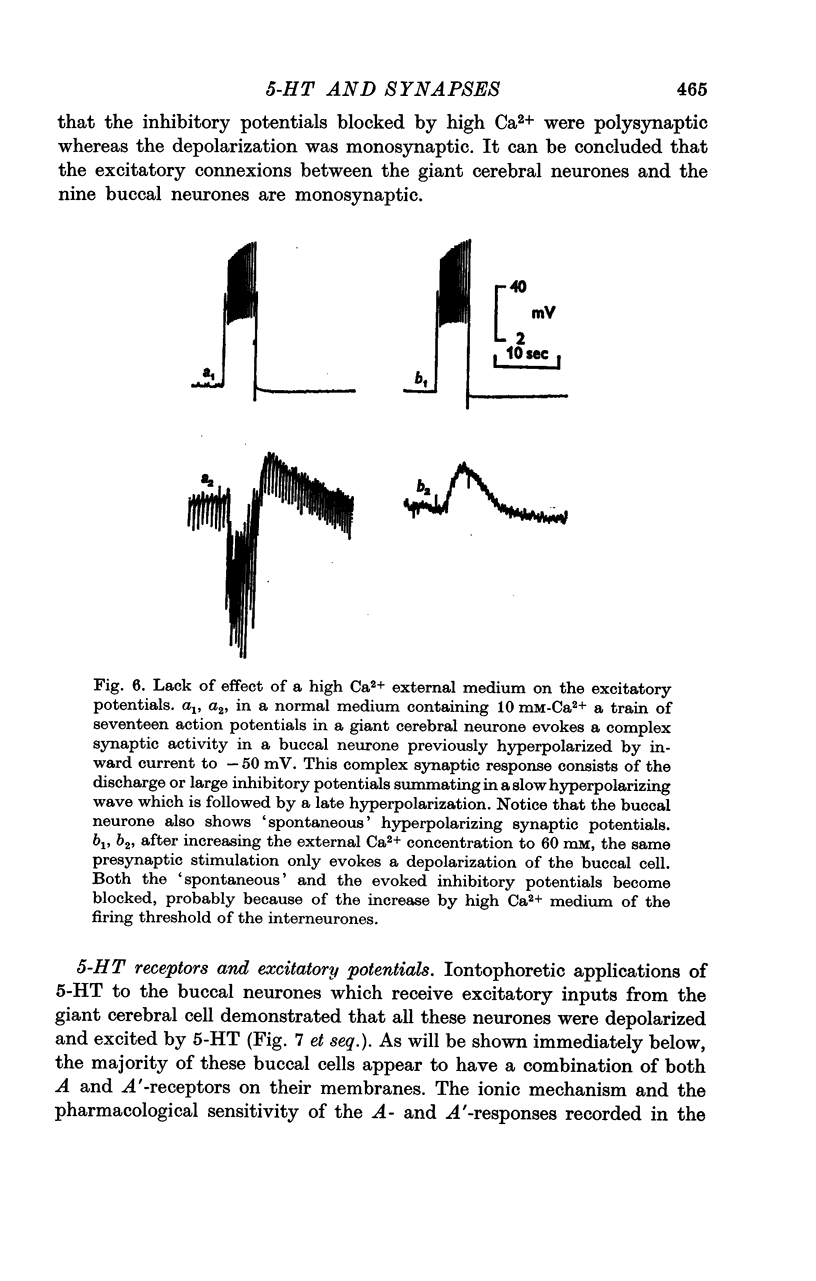
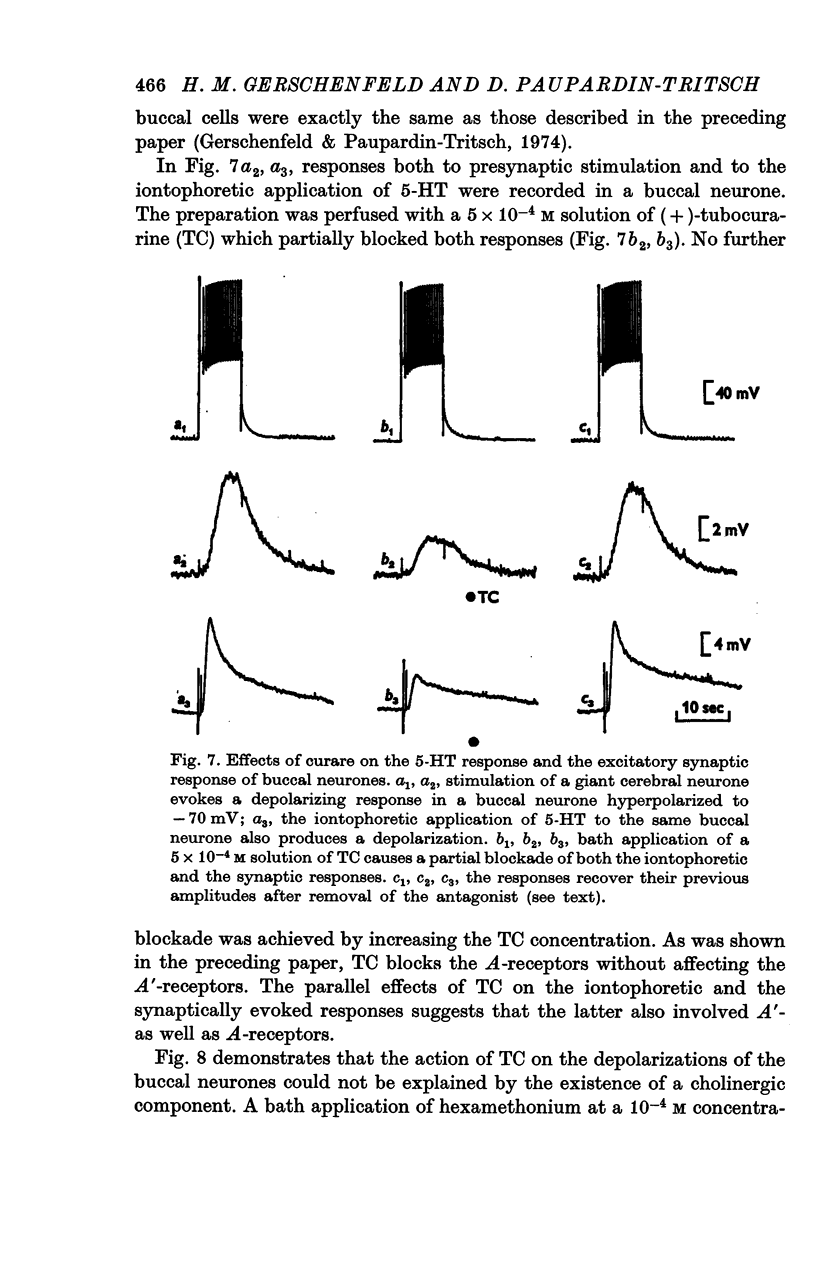
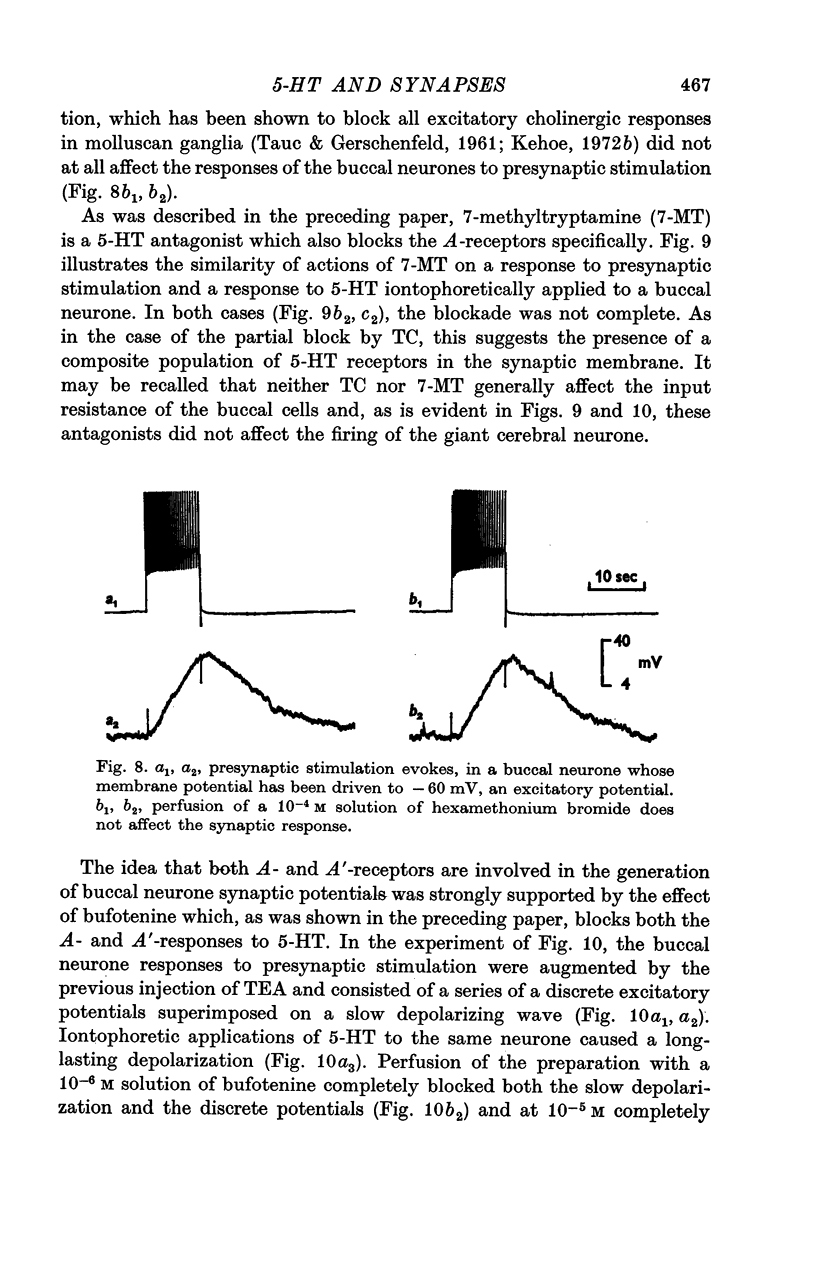
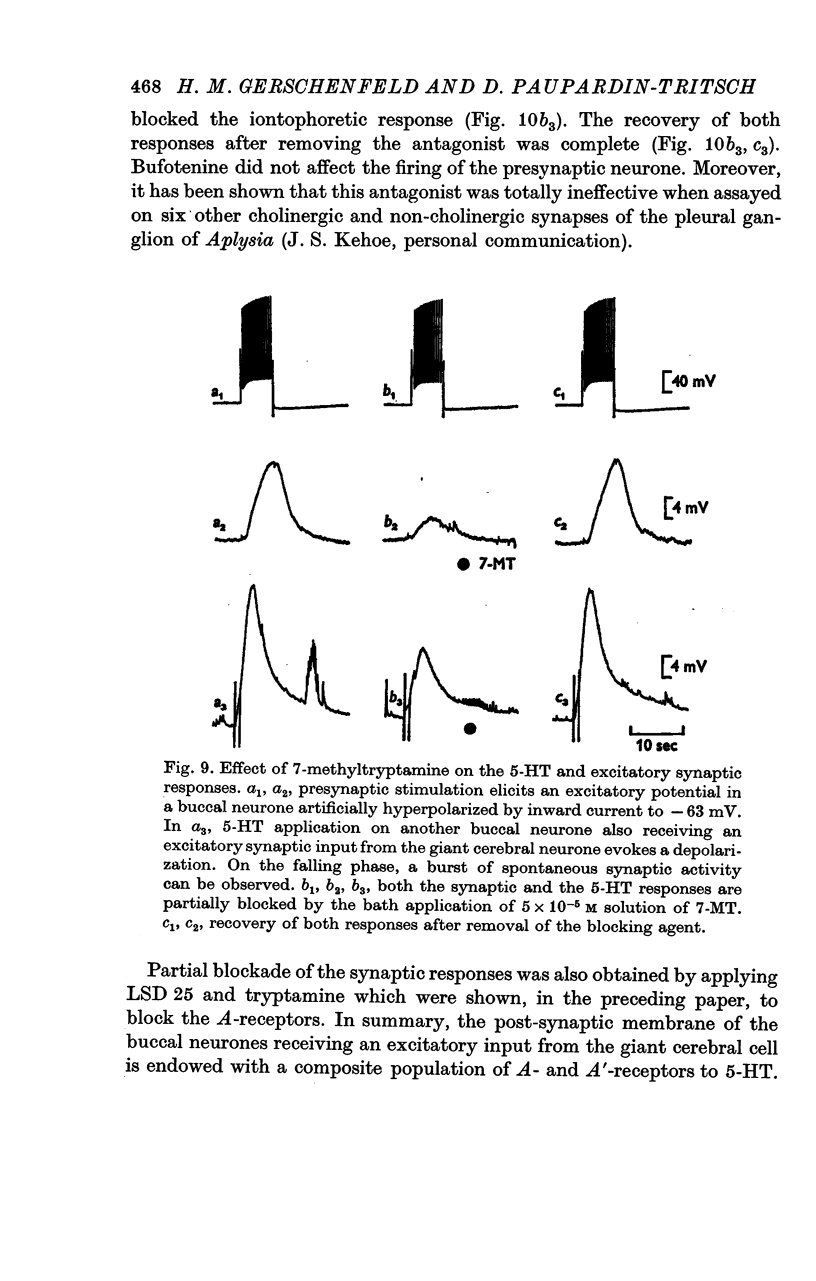
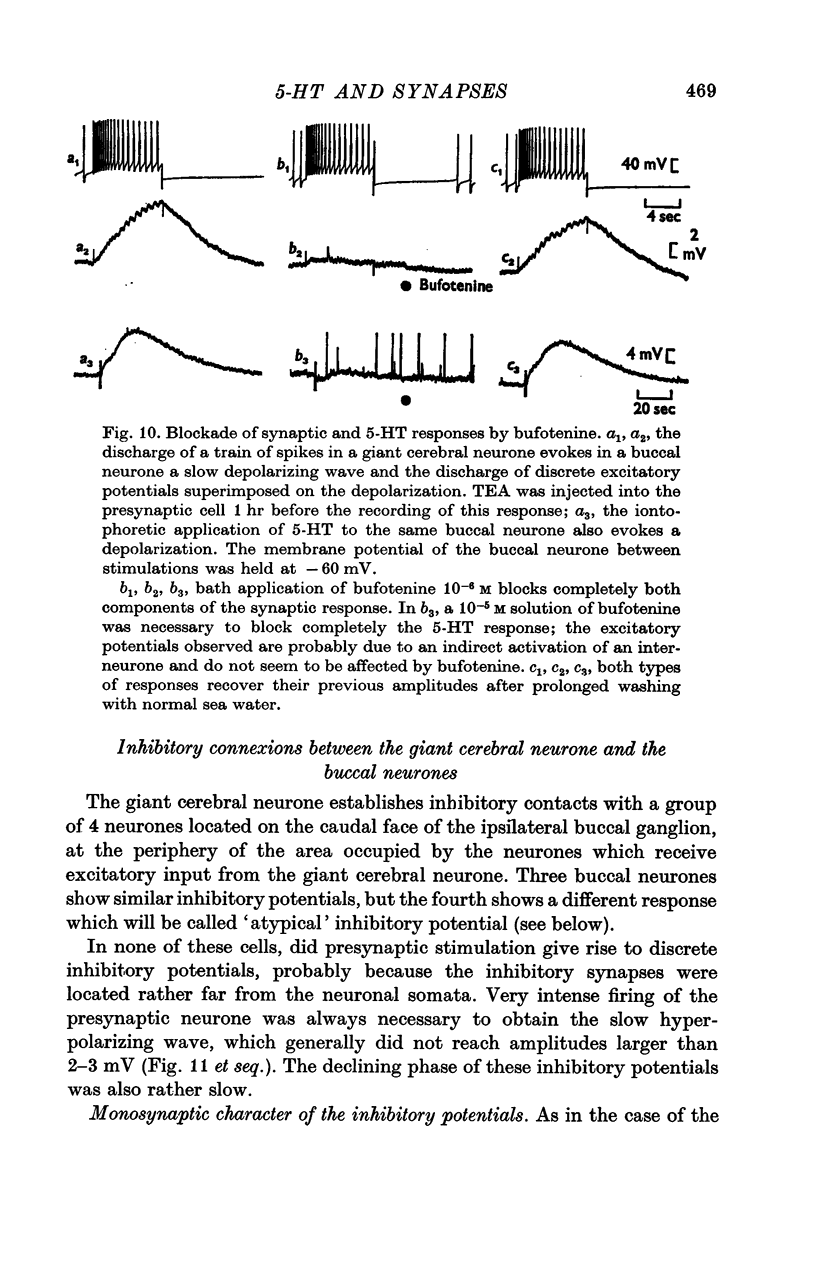
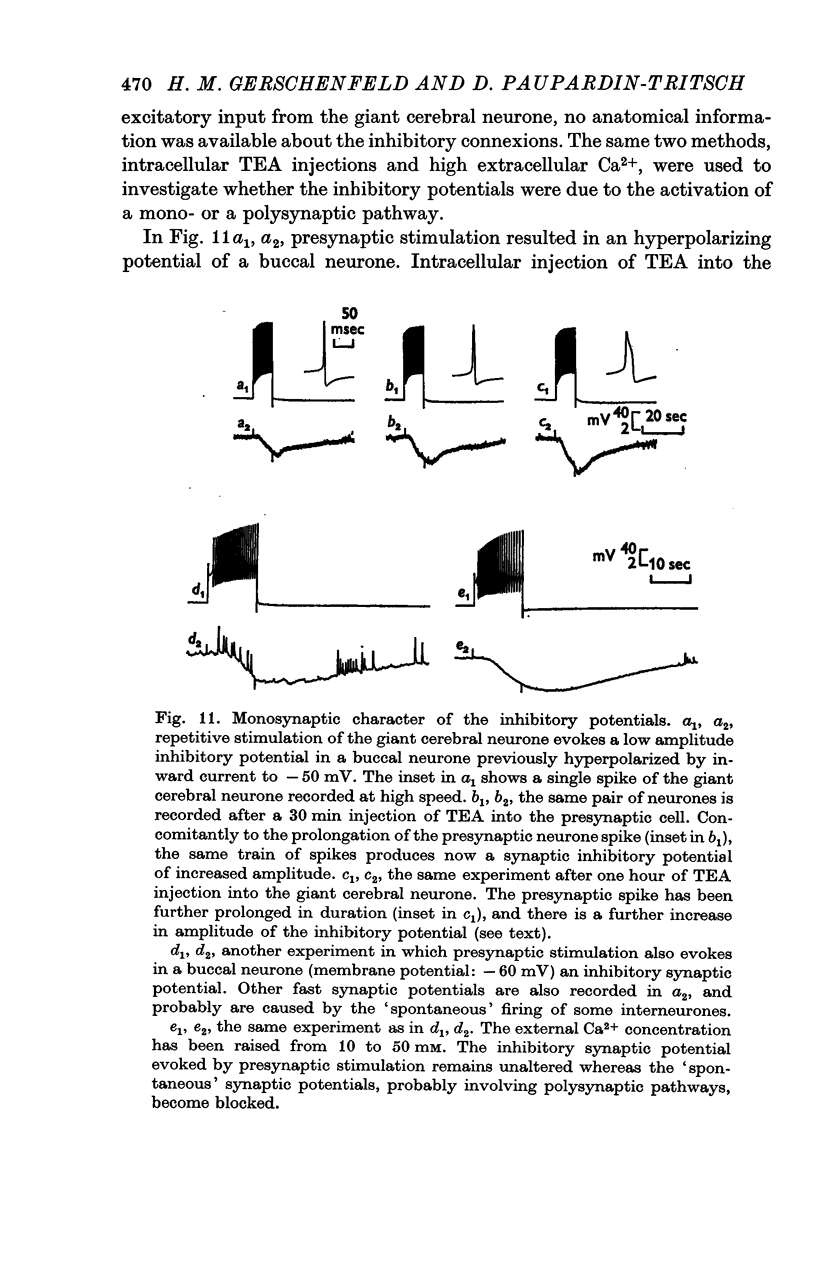
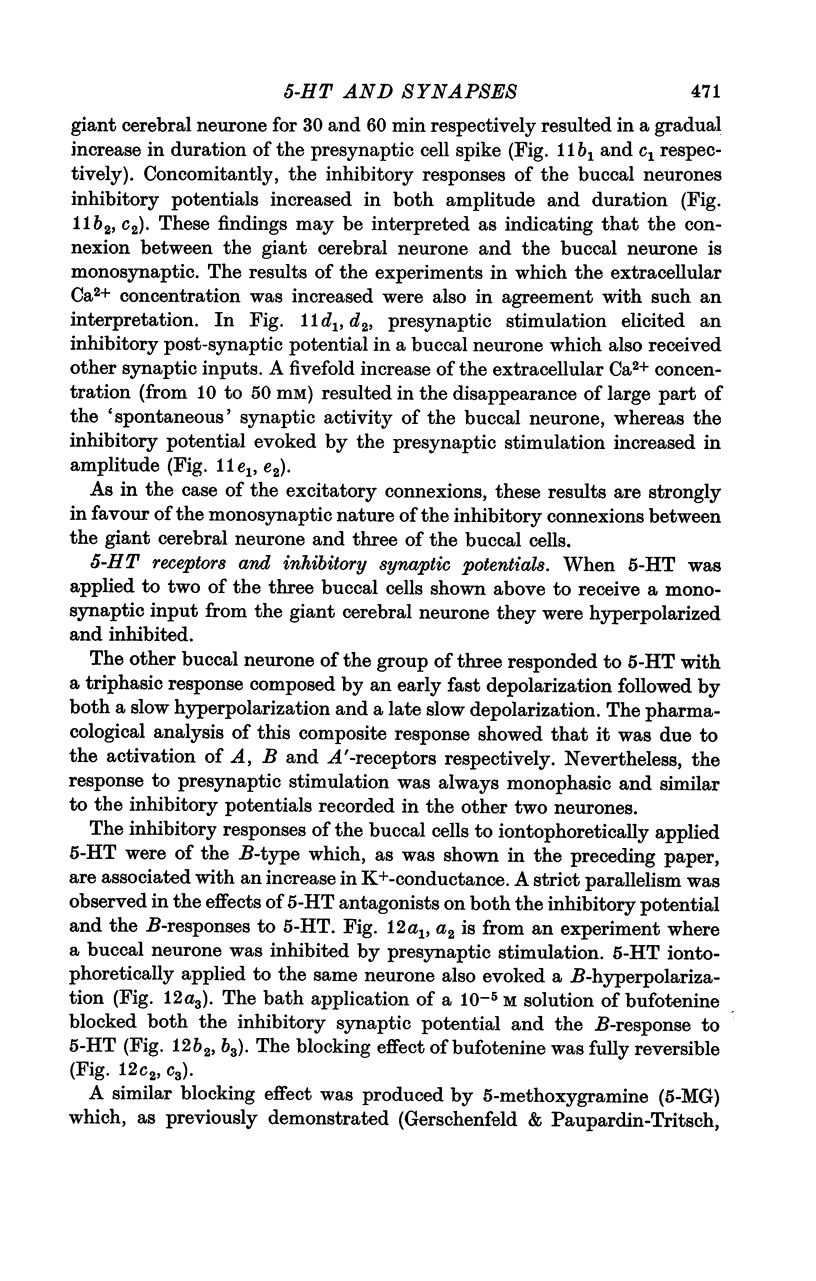
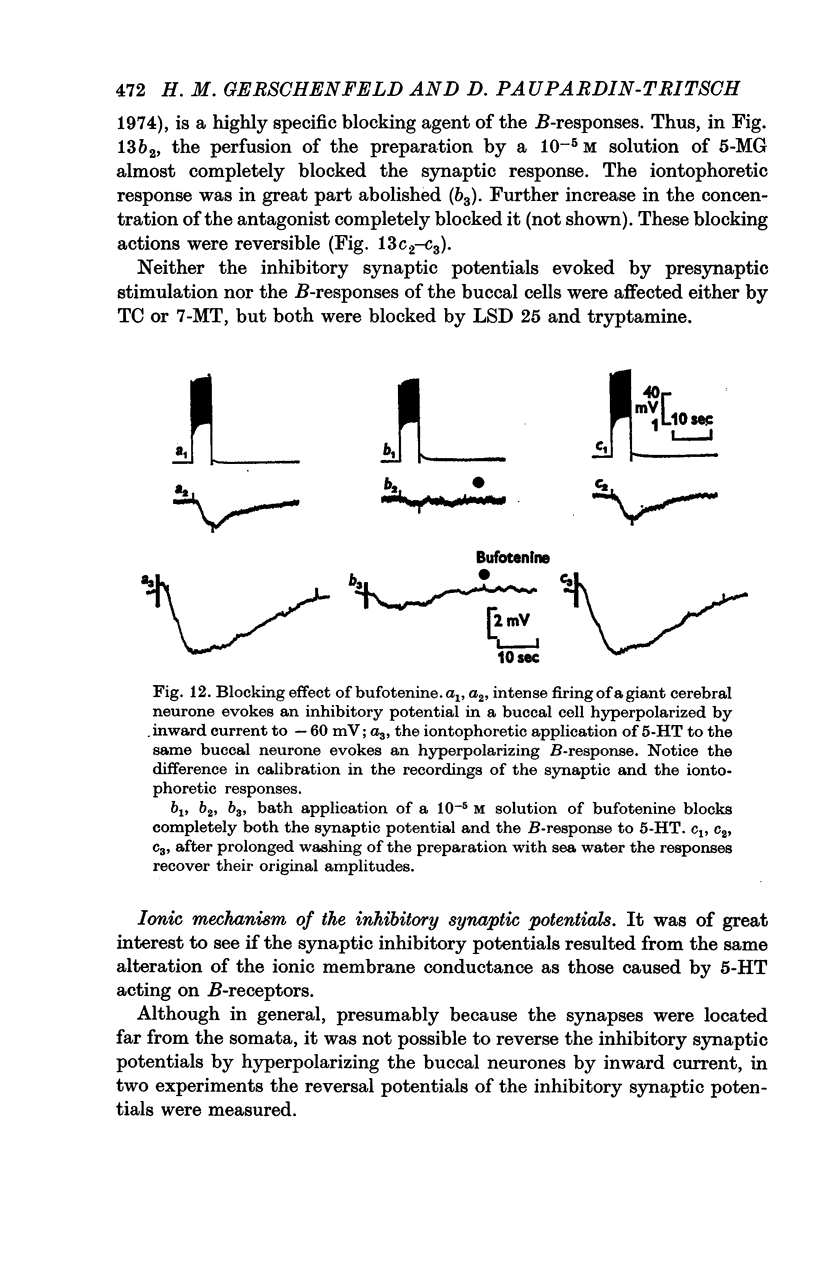
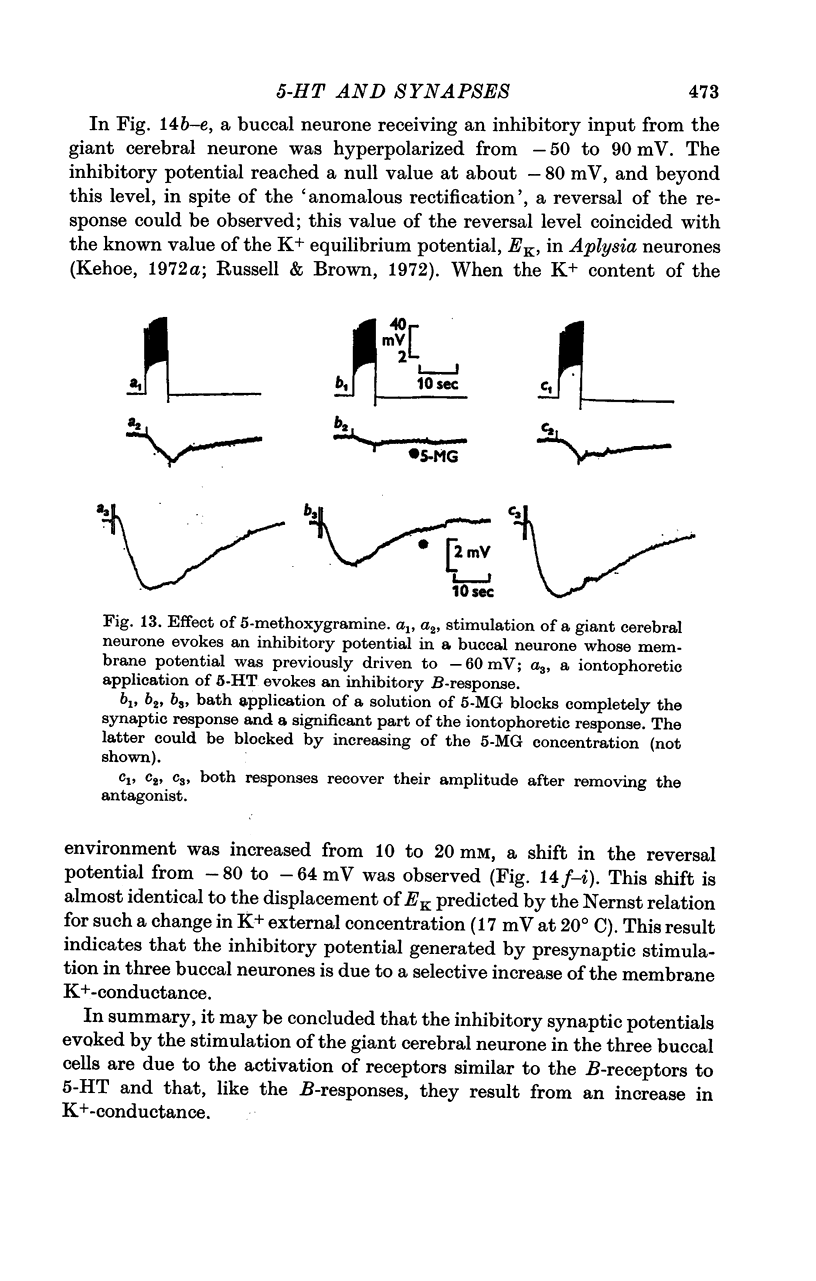
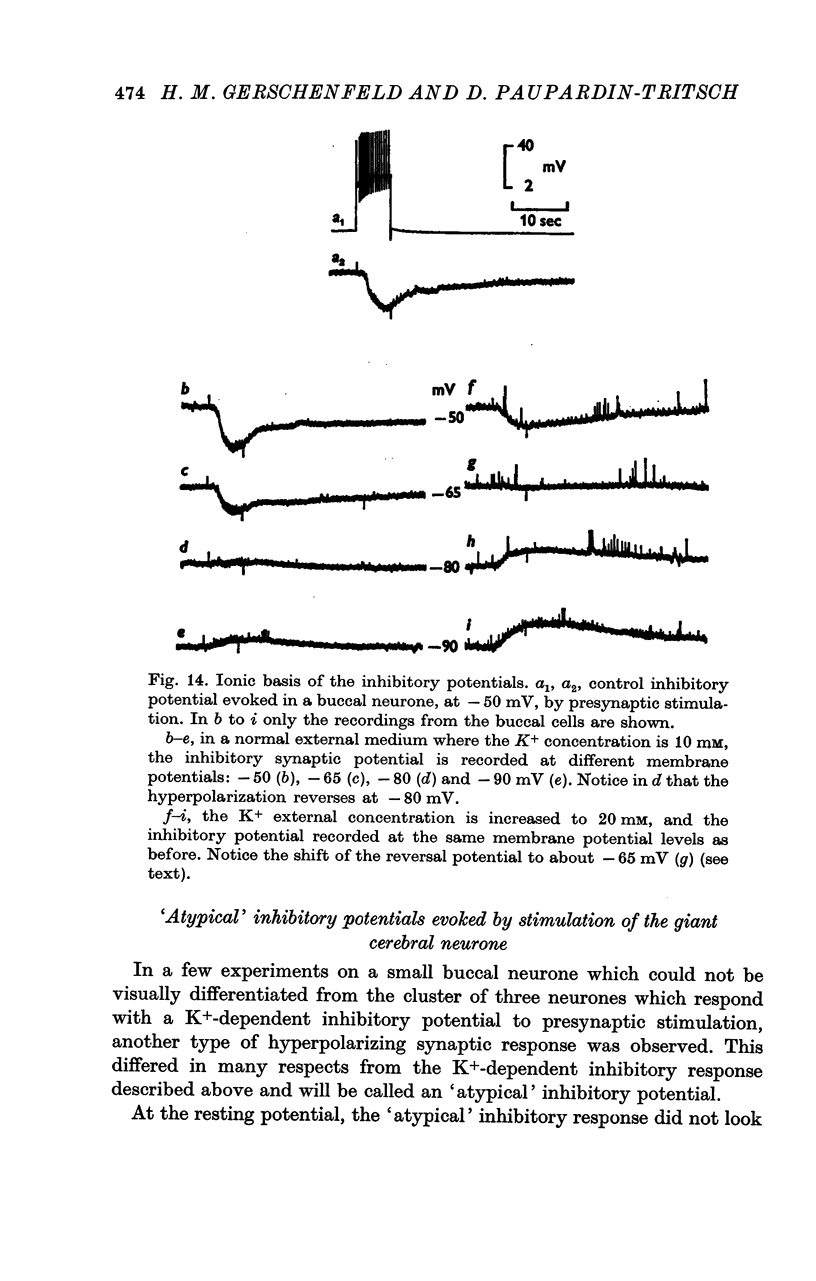
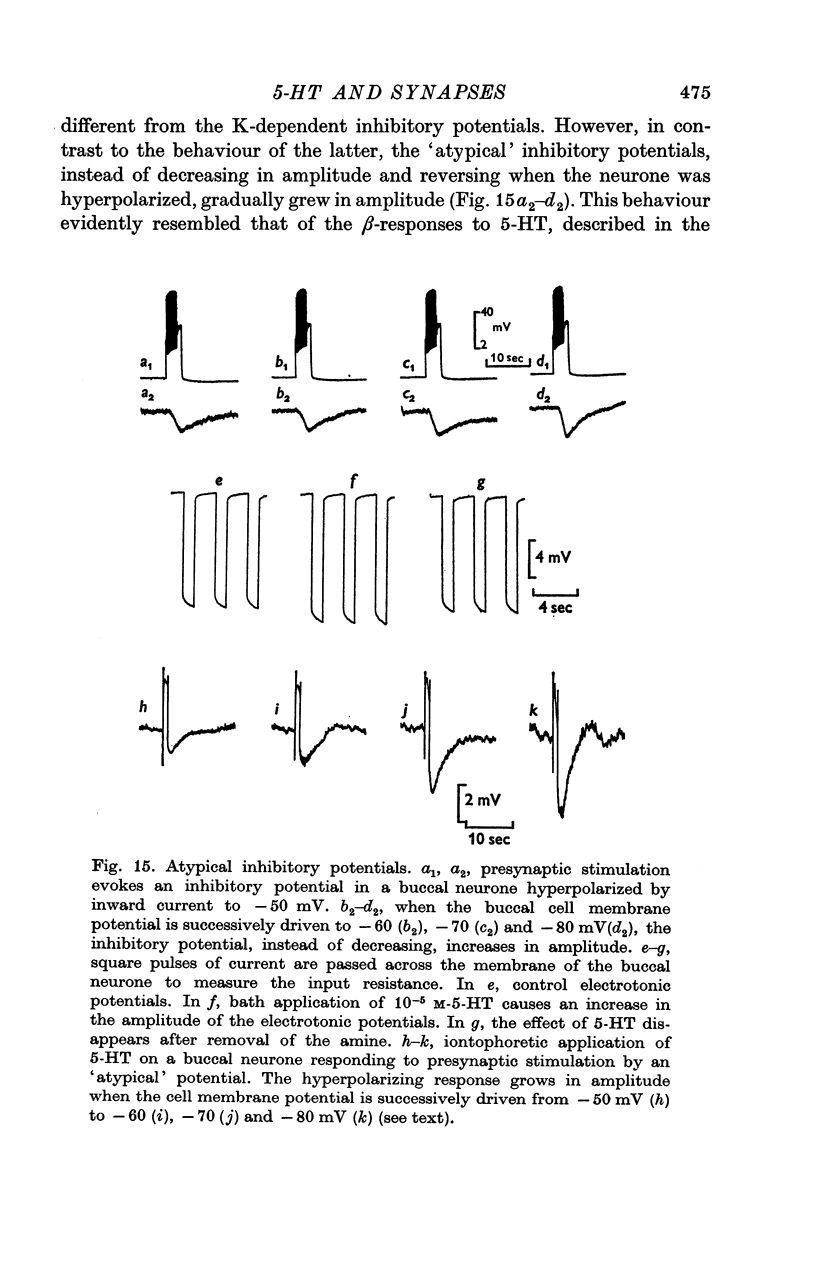
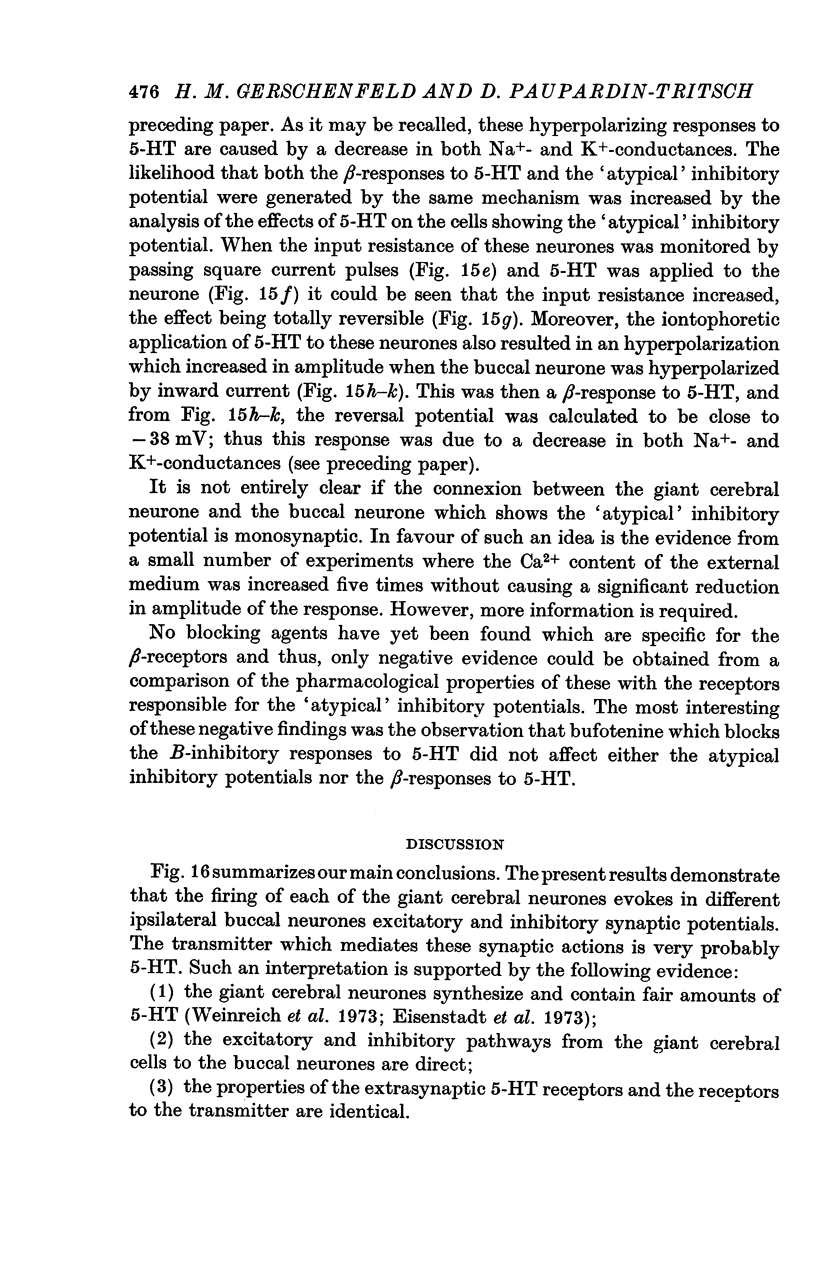
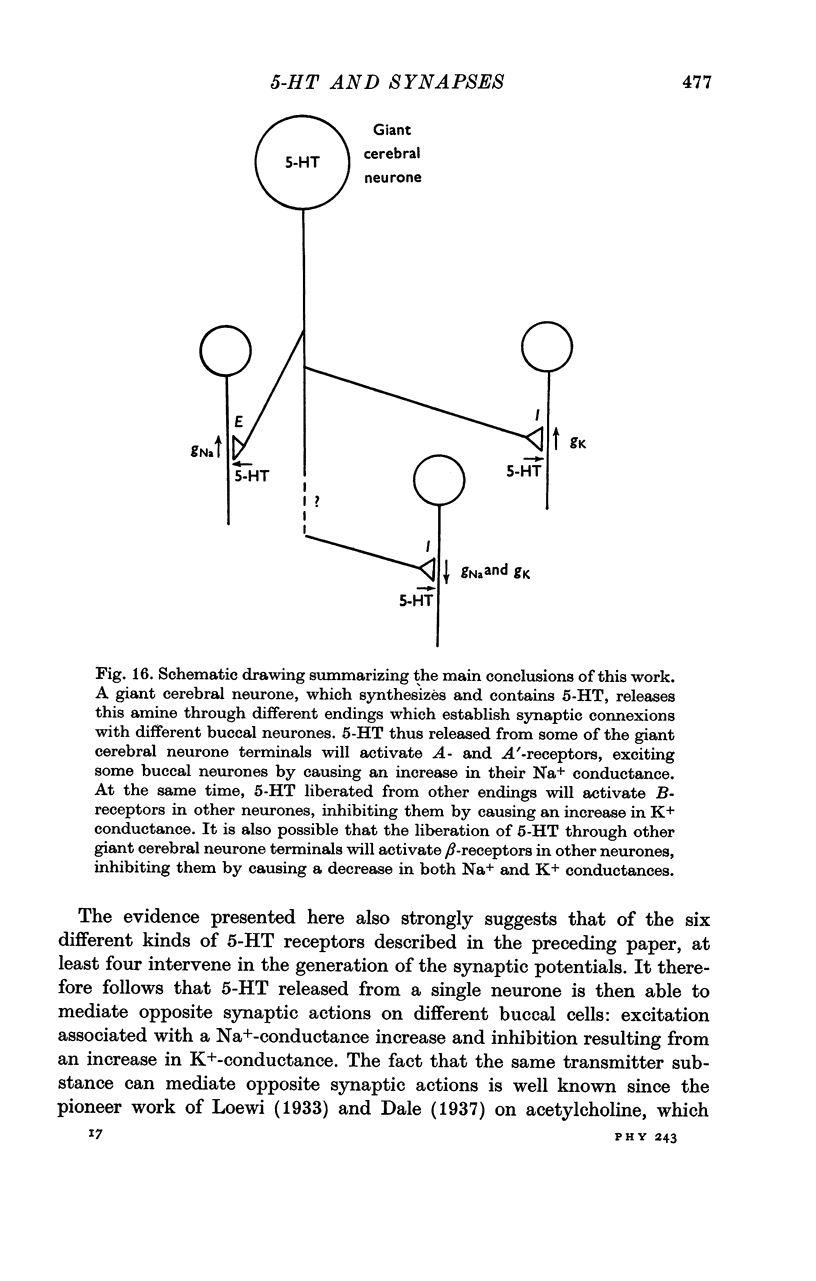
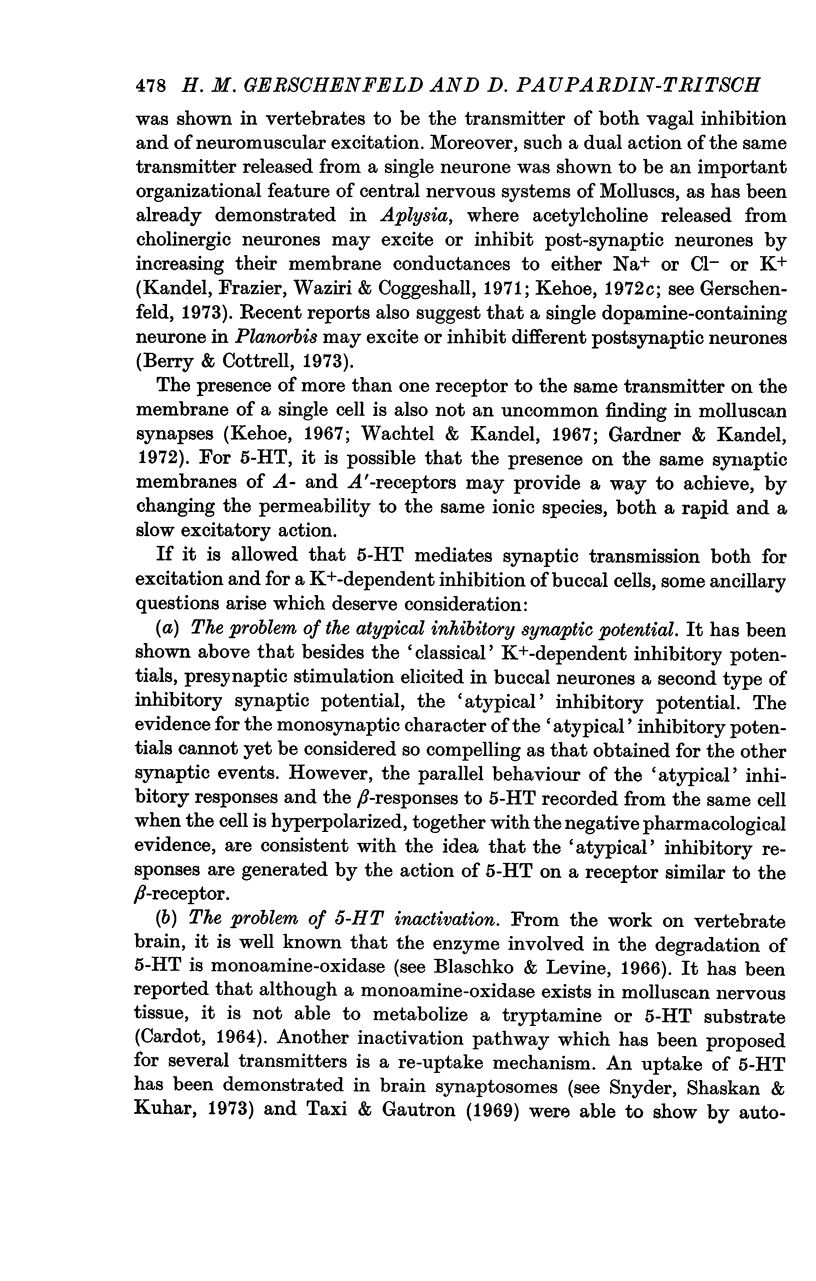
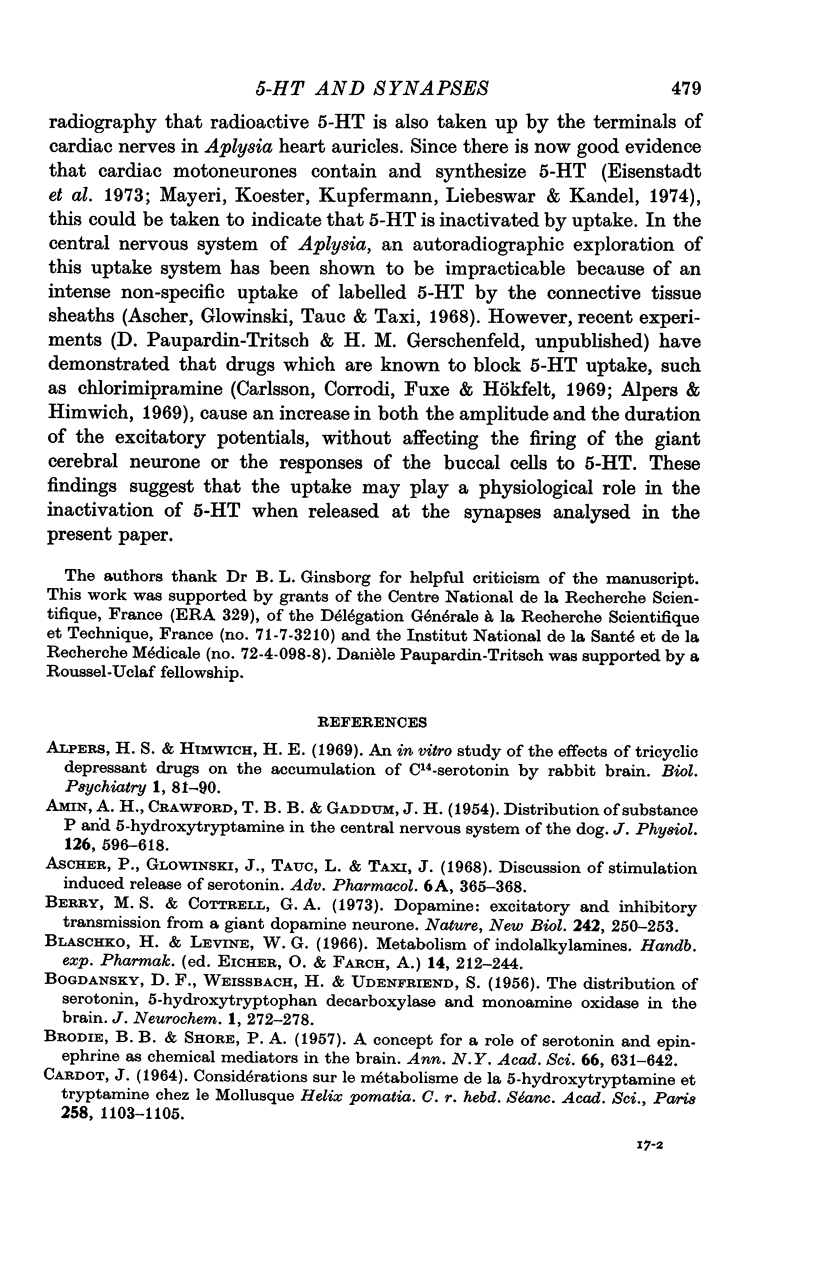
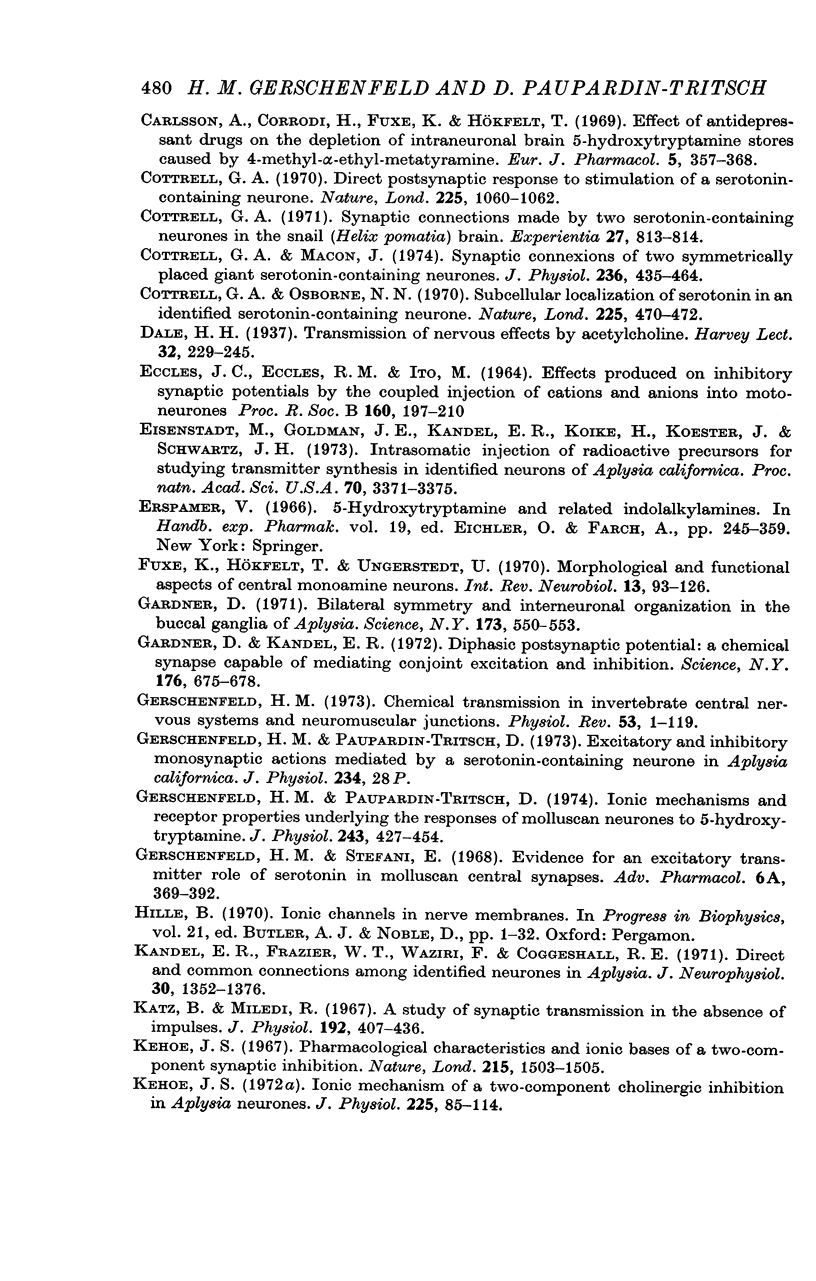
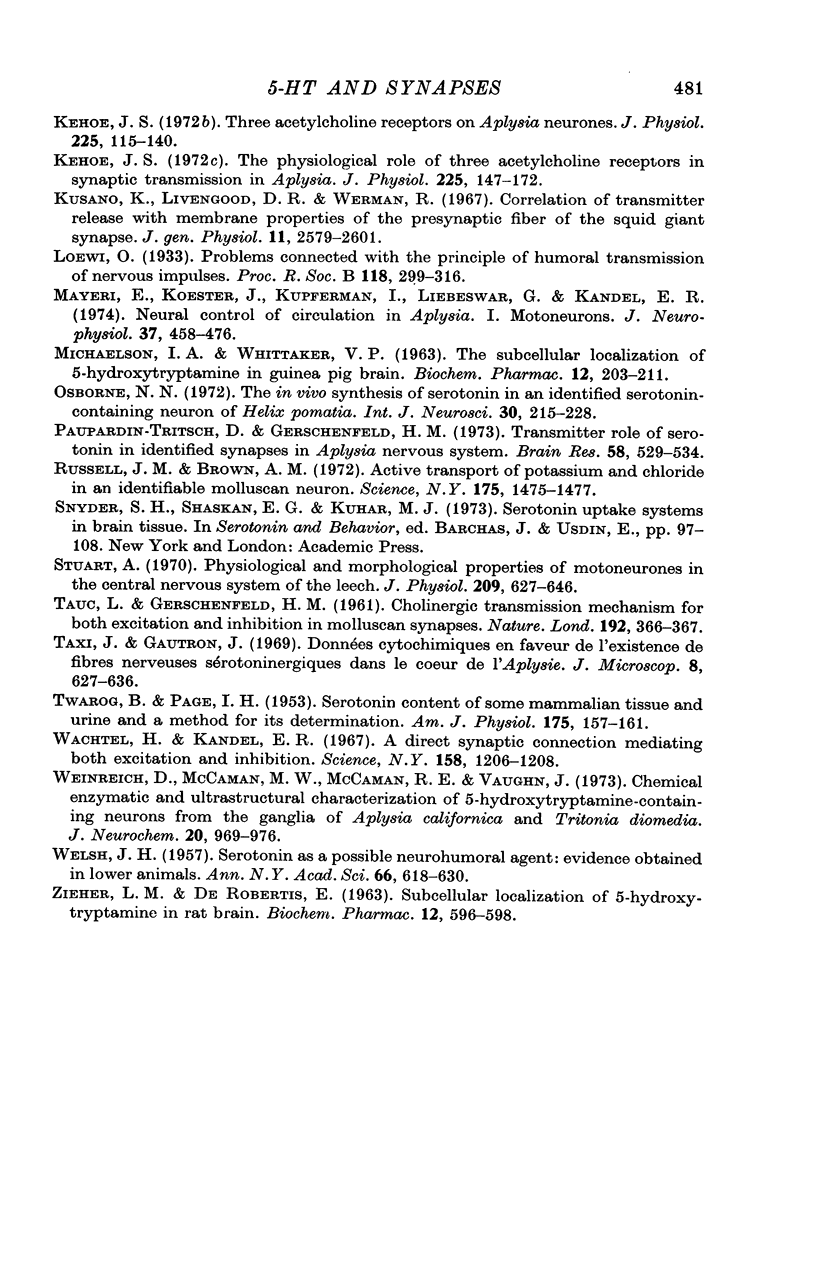
Selected References
These references are in PubMed. This may not be the complete list of references from this article.
- AMIN A. H., CRAWFORD T. B., GADDUM J. H. The distribution of substance P and 5-hydroxytryptamine in the central nervous system of the dog. J Physiol. 1954 Dec 10;126(3):596–618. doi: 10.1113/jphysiol.1954.sp005229. [DOI] [PMC free article] [PubMed] [Google Scholar]
- Alpers H. S., Himwich H. E. An in vitro study of the effects of tricyclic antidepressant drugs on the accumulation of C14-serotonin by rabbit brain. Biol Psychiatry. 1969 Jan;1(1):81–85. [PubMed] [Google Scholar]
- Ascher P., Glowinski J., Tauc L., Taxi J. Discussion of stimulation-induced release of serotonin. Adv Pharmacol. 1968;6(Pt A):365–368. doi: 10.1016/s1054-3589(08)61192-8. [DOI] [PubMed] [Google Scholar]
- BOGDANSKI D. F., WEISSBACH H., UDENFRIEND S. The distribution of serotonin, 5-hydroxytryptophan decarboxylase, and monoamine oxidase in brain. J Neurochem. 1957;1(3):272–278. doi: 10.1111/j.1471-4159.1957.tb12082.x. [DOI] [PubMed] [Google Scholar]
- BRODIE B. B., SHORE P. A. A concept for a role of serotonin and norepinephrine as chemical mediators in the brain. Ann N Y Acad Sci. 1957 Mar 14;66(3):631–642. doi: 10.1111/j.1749-6632.1957.tb40753.x. [DOI] [PubMed] [Google Scholar]
- Berry M. S., Cottrell G. A. Dopamine: excitatory and inhibitory transmission from a giant dopamine neurone. Nat New Biol. 1973 Apr 25;242(121):250–253. doi: 10.1038/newbio242250a0. [DOI] [PubMed] [Google Scholar]
- CARDOT J. CONSID'ERATIONS SUR LE M'ETABOLISME DE LA 5-HYDROXYTRYPTAMINE ET DE LA TRYPTAMINE CHEZ LE MOLLUSQUE HELIX POMATIA. C R Hebd Seances Acad Sci. 1964 Jan 20;258:1103–1105. [PubMed] [Google Scholar]
- Carlsson A., Corrodi H., Fuxe K., Hökfelt T. Effect of antidepressant drugs on the depletion of intraneuronal brain 5-hydroxytryptamine stores caused by 4-methyl-alpha-ethyl-meta-tyramine. Eur J Pharmacol. 1969 Mar;5(4):357–366. doi: 10.1016/0014-2999(69)90113-7. [DOI] [PubMed] [Google Scholar]
- Cottrell G. A. Direct postsynaptic responses to stimulation of serotonin-containing neurones. Nature. 1970 Mar 14;225(5237):1060–1062. doi: 10.1038/2251060a0. [DOI] [PubMed] [Google Scholar]
- Cottrell G. A., Macon J. B. Synaptic connexions of two symmetrically placed giant serotonin-containing neurones. J Physiol. 1974 Jan;236(2):435–464. doi: 10.1113/jphysiol.1974.sp010445. [DOI] [PMC free article] [PubMed] [Google Scholar]
- Cottrell G. A., Osborne N. N. Subcellular localization of serotonin in an identified serotonin-containing neurone. Nature. 1970 Jan 31;225(5231):470–472. doi: 10.1038/225470a0. [DOI] [PubMed] [Google Scholar]
- Cottrell G. A. Synaptic connections made by two serotonin-containing neurons in the snail (Helix pomatia) brain. Experientia. 1971 Jul;27(7):813–815. doi: 10.1007/BF02136882. [DOI] [PubMed] [Google Scholar]
- ECCLES J., ECCLES R. M., ITO M. EFFECTS PRODUCED ON INHIBITORY POSTSYNAPTIC POTENTIALS BY THE COUPLED INJECTIONS OF CATIONS AND ANIONS INTO MOTONEURONS. Proc R Soc Lond B Biol Sci. 1964 May 19;160:197–210. doi: 10.1098/rspb.1964.0036. [DOI] [PubMed] [Google Scholar]
- Eisenstadt M., Goldman J. E., Kandel E. R., Koike H., Koester J., Schwartz J. H. Intrasomatic injection of radioactive precursors for studying transmitter synthesis in identified neurons of Aplysia californica. Proc Natl Acad Sci U S A. 1973 Dec;70(12):3371–3375. doi: 10.1073/pnas.70.12.3371. [DOI] [PMC free article] [PubMed] [Google Scholar]
- Gardner D. Bilateral symmetry and interneuronal organization in the buccal ganglia of Aplysia. Science. 1971 Aug 6;173(3996):550–553. doi: 10.1126/science.173.3996.550. [DOI] [PubMed] [Google Scholar]
- Gardner D., Kandel E. R. Diphasic postsynaptic potential: a chemical synapse capable of mediating conjoint excitation and inhibition. Science. 1972 May 12;176(4035):675–678. doi: 10.1126/science.176.4035.675. [DOI] [PubMed] [Google Scholar]
- Gerschenfeld H. M. Chemical transmission in invertebrate central nervous systems and neuromuscular junctions. Physiol Rev. 1973 Jan;53(1):1–119. doi: 10.1152/physrev.1973.53.1.1. [DOI] [PubMed] [Google Scholar]
- Gerschenfeld H. M., Paupardin-Tritsch D. Ionic mechanisms and receptor properties underlying the responses of molluscan neurones to 5-hydroxytryptamine. J Physiol. 1974 Dec;243(2):427–456. doi: 10.1113/jphysiol.1974.sp010761. [DOI] [PMC free article] [PubMed] [Google Scholar]
- Gerschenfeld H. M., Stefani E. Evidence for an excitatory transmitter role of serotonin in molluscan central synapses. Adv Pharmacol. 1968;6(Pt A):369–392. doi: 10.1016/s1054-3589(08)61193-x. [DOI] [PubMed] [Google Scholar]
- Hille B. Ionic channels in nerve membranes. Prog Biophys Mol Biol. 1970;21:1–32. doi: 10.1016/0079-6107(70)90022-2. [DOI] [PubMed] [Google Scholar]
- Kandel E. R., Frazier W. T., Waziri R., Coggeshall R. E. Direct and common connections among identified neurons in Aplysia. J Neurophysiol. 1967 Nov;30(6):1352–1376. doi: 10.1152/jn.1967.30.6.1352. [DOI] [PubMed] [Google Scholar]
- Katz B., Miledi R. A study of synaptic transmission in the absence of nerve impulses. J Physiol. 1967 Sep;192(2):407–436. doi: 10.1113/jphysiol.1967.sp008307. [DOI] [PMC free article] [PubMed] [Google Scholar]
- Kehoe J. Ionic mechanisms of a two-component cholinergic inhibition in Aplysia neurones. J Physiol. 1972 Aug;225(1):85–114. doi: 10.1113/jphysiol.1972.sp009930. [DOI] [PMC free article] [PubMed] [Google Scholar]
- Kehoe J. Pharmacological characteristics and ionic bases of a 2 component postsynaptic inhibition. Nature. 1967 Sep 30;215(5109):1503–1505. doi: 10.1038/2151503b0. [DOI] [PubMed] [Google Scholar]
- Kehoe J. The physiological role of three acetylcholine receptors in synaptic transmission in Aplysia. J Physiol. 1972 Aug;225(1):147–172. doi: 10.1113/jphysiol.1972.sp009932. [DOI] [PMC free article] [PubMed] [Google Scholar]
- Kehoe J. Three acetylcholine receptors in Aplysia neurones. J Physiol. 1972 Aug;225(1):115–146. doi: 10.1113/jphysiol.1972.sp009931. [DOI] [PMC free article] [PubMed] [Google Scholar]
- Kusano K., Livengood D. R., Werman R. Correlation of transmitter release with membrane properties of the presynaptic fiber of the squid giant synapse. J Gen Physiol. 1967 Dec;50(11):2579–2601. doi: 10.1085/jgp.50.11.2579. [DOI] [PMC free article] [PubMed] [Google Scholar]
- MICHAELSON I. A., WHITTAKER V. P. The subcellular localization of 5-hydroxytryptamine in guinea pig brain. Biochem Pharmacol. 1963 Feb;12:203–211. doi: 10.1016/0006-2952(63)90185-0. [DOI] [PubMed] [Google Scholar]
- Mayeri E., Koester J., Kupfermann I., Liebeswar G., Kandel E. R. Neural control of circulation in Aplysia. I. Motoneurons. J Neurophysiol. 1974 May;37(3):458–475. doi: 10.1152/jn.1974.37.3.458. [DOI] [PubMed] [Google Scholar]
- Paupardin-Tritsch D., Gerschenfeld H. M. Transmitter role of serotonin in identified synapses in Aplysia nervous system. Brain Res. 1973 Aug 30;58(2):529–534. doi: 10.1016/0006-8993(73)90027-9. [DOI] [PubMed] [Google Scholar]
- Russell J. M., Brown A. M. Active transport of potassium and chloride in an identifiable molluscan neuron. Science. 1972 Mar 31;175(4029):1475–1477. doi: 10.1126/science.175.4029.1475. [DOI] [PubMed] [Google Scholar]
- Stuart A. E. Physiological and morphological properties of motoneurones in the central nervous system of the leech. J Physiol. 1970 Aug;209(3):627–646. doi: 10.1113/jphysiol.1970.sp009183. [DOI] [PMC free article] [PubMed] [Google Scholar]
- TAUC L., GERSCHENFELD H. M. Cholinergic transmission mechanisms for both excitation and inhibition in molluscan central synapses. Nature. 1961 Oct 28;192:366–367. doi: 10.1038/192366a0. [DOI] [PubMed] [Google Scholar]
- TWAROG B. M., PAGE I. H. Serotonin content of some mammalian tissues and urine and a method for its determination. Am J Physiol. 1953 Oct;175(1):157–161. doi: 10.1152/ajplegacy.1953.175.1.157. [DOI] [PubMed] [Google Scholar]
- WELSH J. H. Serotonin as a possible neurohumoral agent; evidence obtained in lower animals. Ann N Y Acad Sci. 1957 Mar 14;66(3):618–630. doi: 10.1111/j.1749-6632.1957.tb40752.x. [DOI] [PubMed] [Google Scholar]
- Wachtel H., Kandel E. R. A direct synaptic connection mediating both excitation and inhibition. Science. 1967 Dec 1;158(3805):1206–1208. doi: 10.1126/science.158.3805.1206. [DOI] [PubMed] [Google Scholar]
- Weinreich D., McCaman M. W., McCaman R. E., Vaughn J. E. Chemical, enzymatic and ultrastructural characterization of 5-hydroxytryptamine-containing neurons from the ganglia of Aplysia californica and Tritionia diomedia. J Neurochem. 1973 Apr;20(4):969–976. doi: 10.1111/j.1471-4159.1973.tb00067.x. [DOI] [PubMed] [Google Scholar]
- ZIEHER L. M., DE ROBERTIS E. Subcellular localization of 5-hydroxytryptamine in rat brain. Biochem Pharmacol. 1963 Jun;12:596–598. doi: 10.1016/0006-2952(63)90141-2. [DOI] [PubMed] [Google Scholar]