Abstract
1. Girardi and Hela cells (derived from human heart and cervix respectively) were grown as monolayer cultures in B.M.E. (Eagles basal medium) containing concentrations of ouabain up to 5 × 10-8 M for periods ranging up to 5 days. The cell sizes, numbers, Na, K, Cl, and Ca concentrations and fluxes were then measured.
2. Twenty-four hours incubation in ouabain concentrations equal to or less than 5 × 10-8 M caused a rise in [Na]i and an almost equal fall in [K]i to new steady levels. The concentrations so reached were linearly related to the ouabain concentrations, such that in 5 × 10-8 M ouabain [Na]i rose to 124 m-mole/l. intracellular water and [K]i fell to 55 m-mole/l. i.c. water in Girardi cells. In Hela cells the changes were smaller at any particular ouabain concentration. These levels were maintained constant for at least 5 days.
3. In cells in the logarithmic phase of growth, raising [Na]i and lowering [K]i by ouabain caused a slowing of growth rate proportional to the ouabain concentration used. In cells in the stationary phase there was no change in the cell numbers over 24 hr. The volume of the cells was not directly affected by the treatment.
4. Reducing [K]o from the normal value of 5·4 to 2·5 mM increased the effect of any ouabain concentration, whereas increasing [K]o to 7·5 decreased the effect of ouabain.
5. Reduction of [K]o to 2·5 mM had no effect on the [K]i or [Na]i but halved the cell numbers, probably by a reduction in the growth rate. The mechanism of this effect is obscure.
6. In Girardi cells raising [Na]i and lowering [K]i by prolonged treatment increases the total Na fluxes and decreases the total K fluxes but keeps the total Na + K flux constant. High-Na, low-K cells had a reduced Na:K exchange compared to fresh cells and also had a Na:K pumped ratio nearer 4:1 than the 3:2 normally found.
7. These cells also show ouabain-sensitive and ouabain-insensitive Na:Na exchanges. In high-Na, low-K cells the ouabain sensitive Na:Na exchange is the same as in fresh cells. The effect of treatment on the ouabain insensitive Na:Na exchanges has not been elucidated.
8. The Cl content and fluxes are not altered by prolonged ouabain treatment. From this it is inferred that the membrane potential in high-Na, low-K cells is the same as in normal cells.
9. High-Na, low-K cells have the same calcium content and fluxes as fresh cells. From this it is concluded that there is no Na:Ca coupling in these cells.
Full text
PDF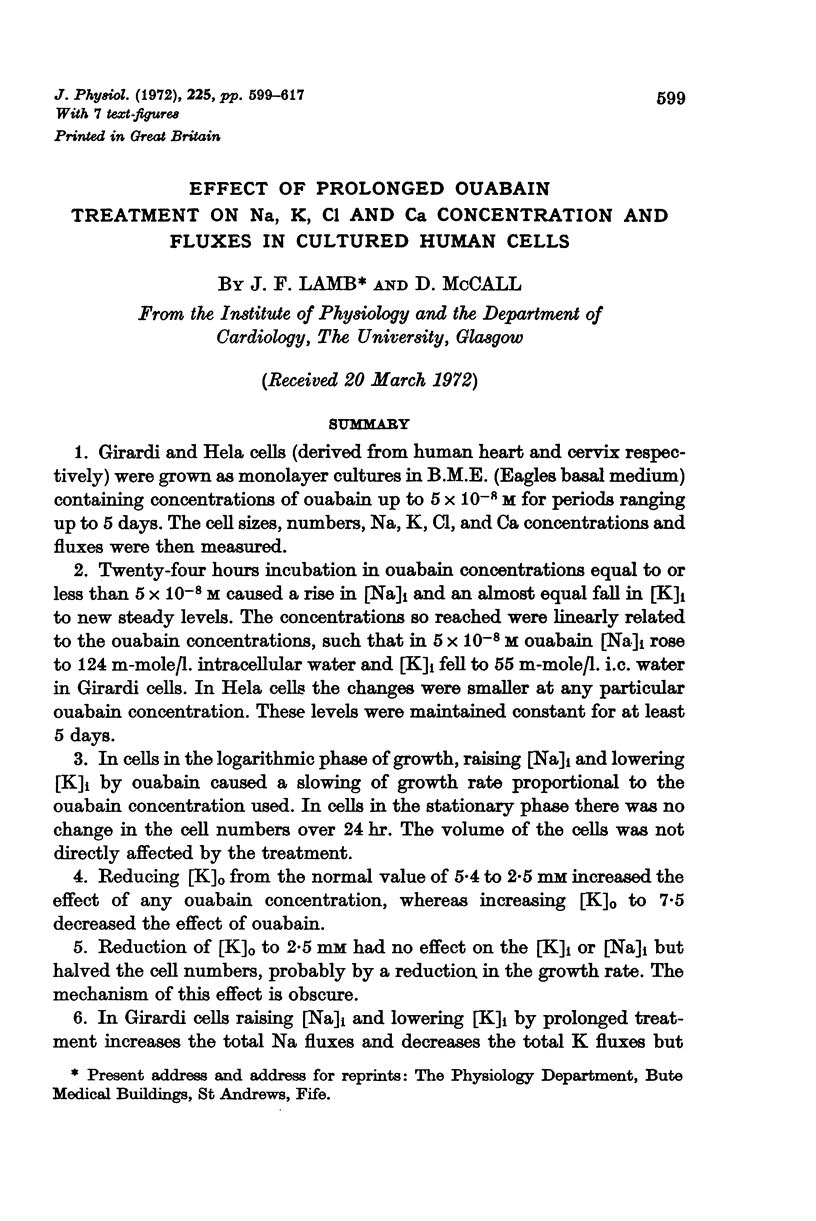
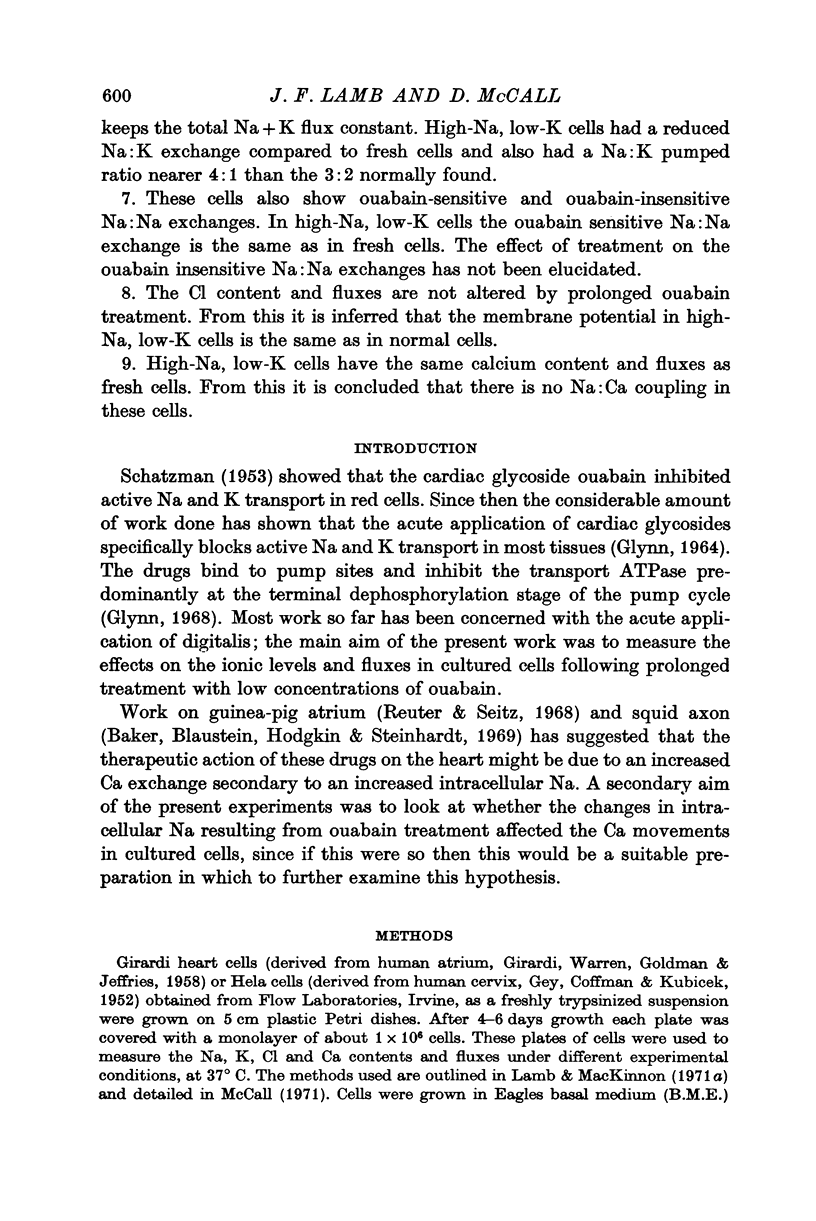
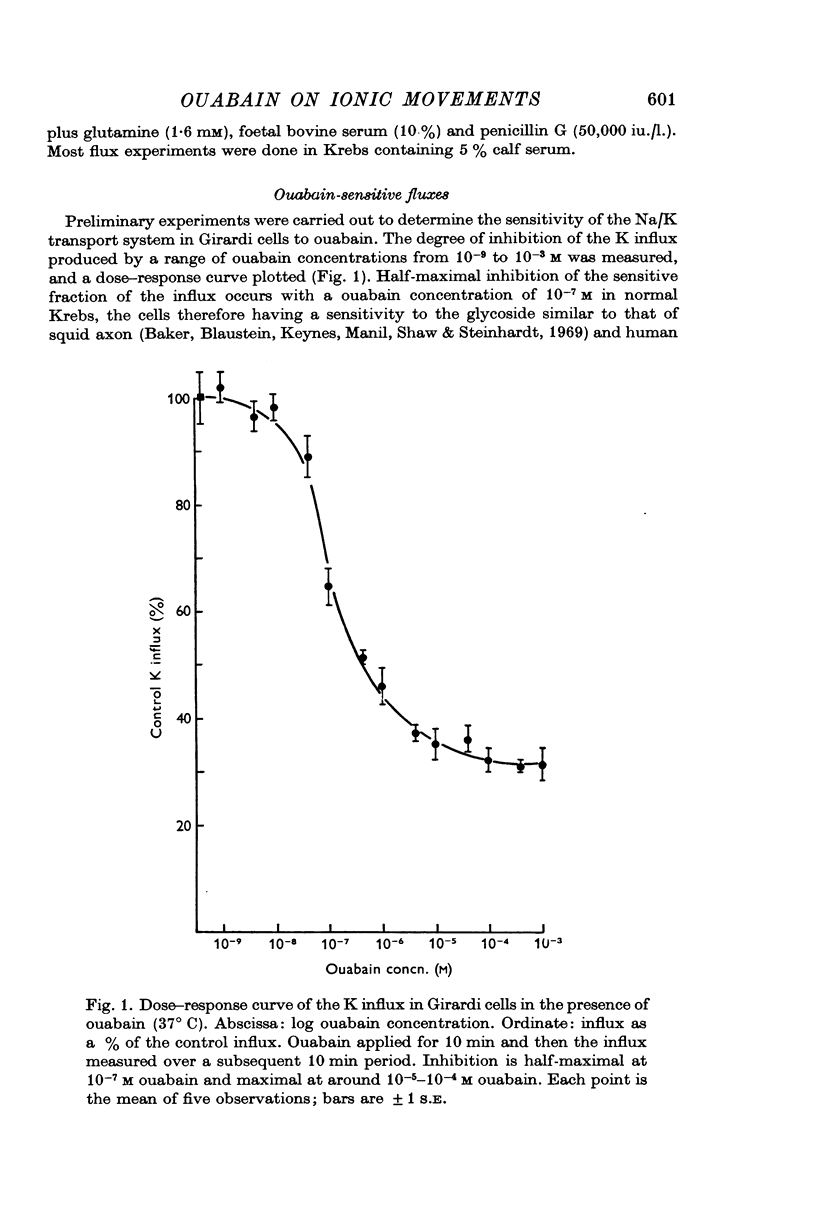
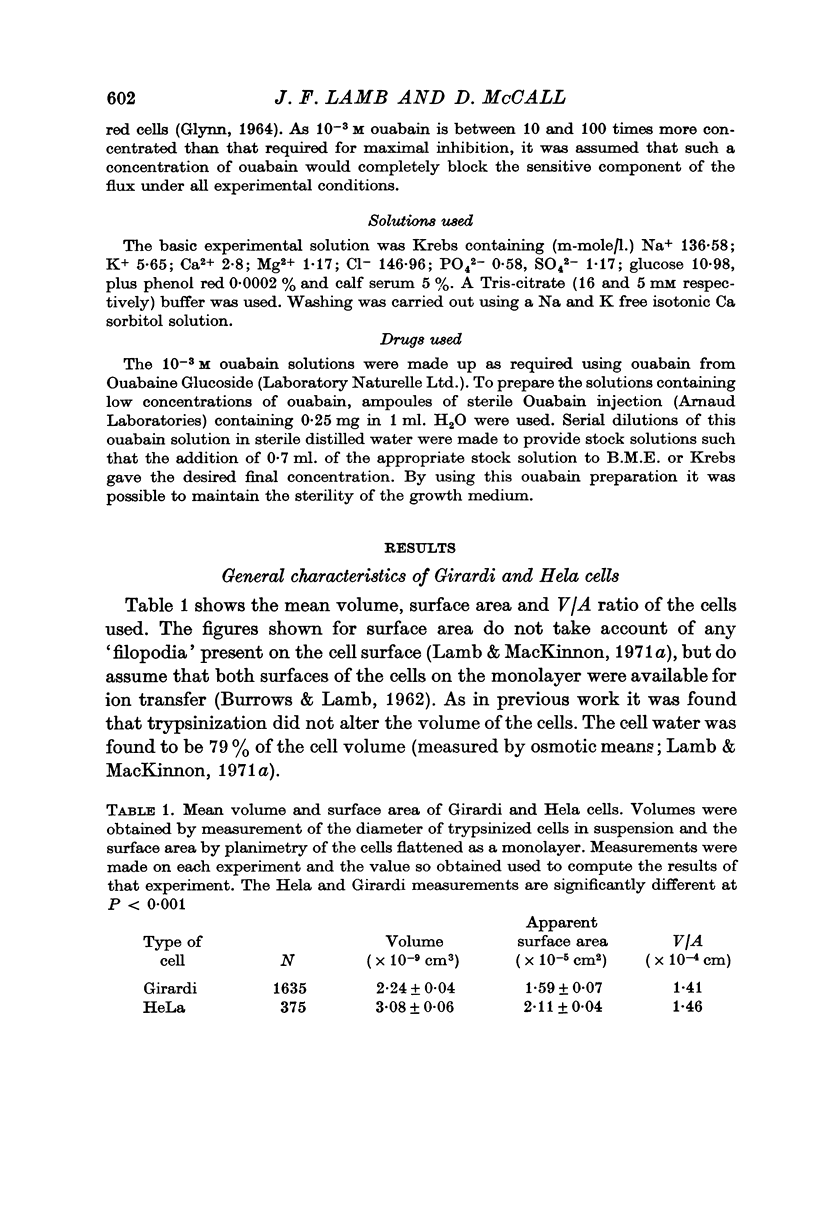
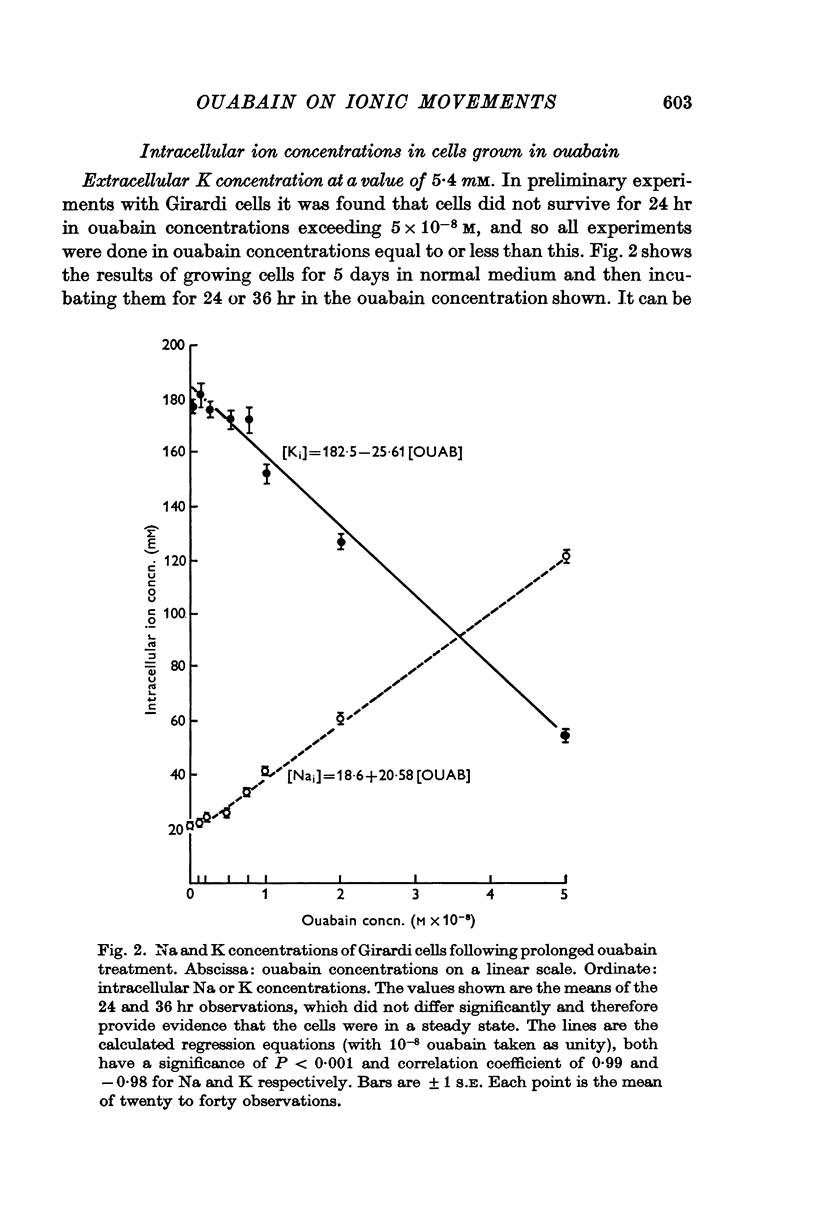
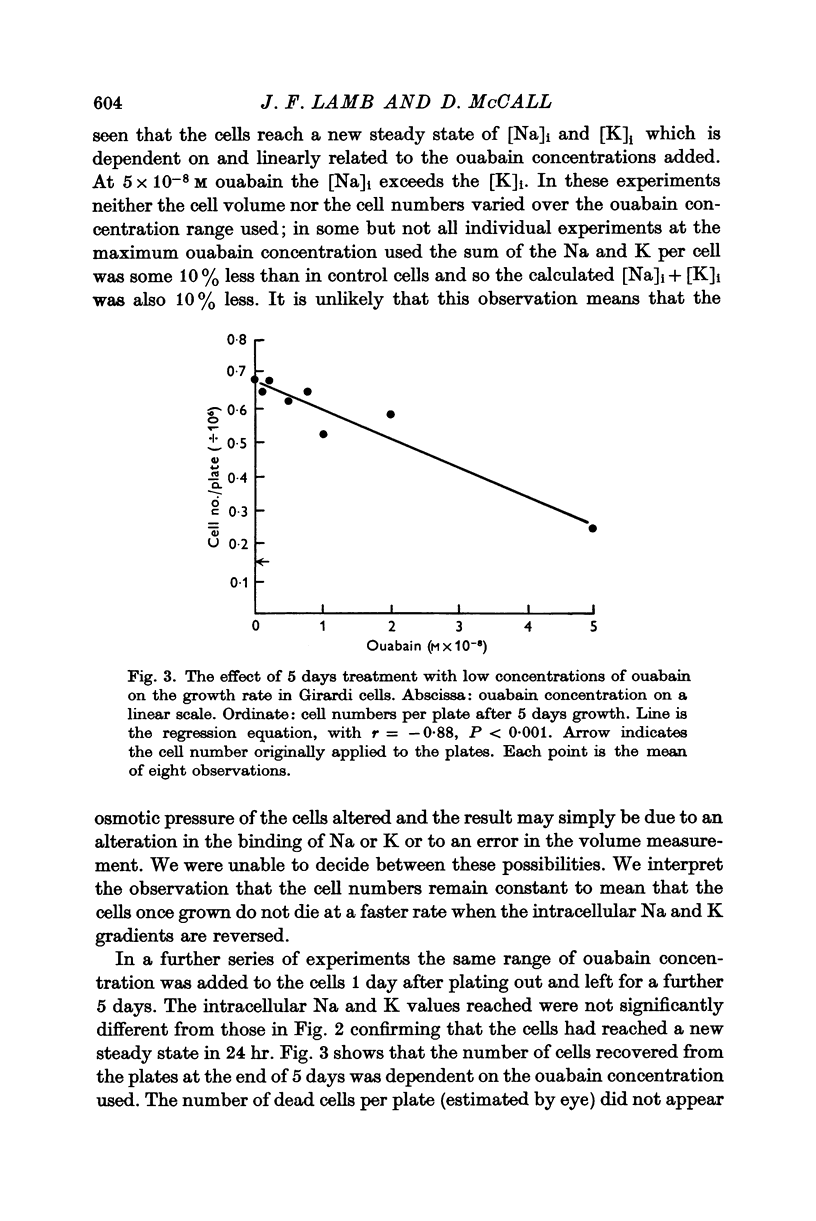
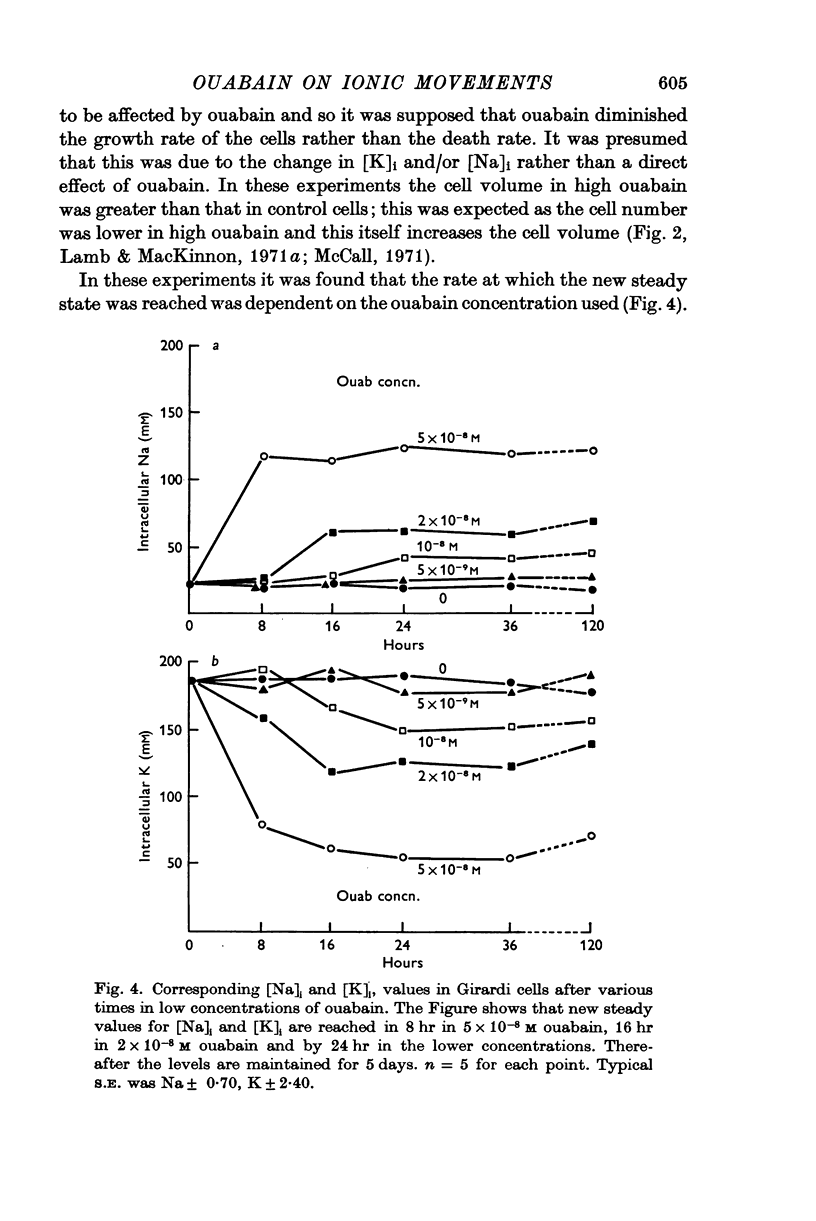
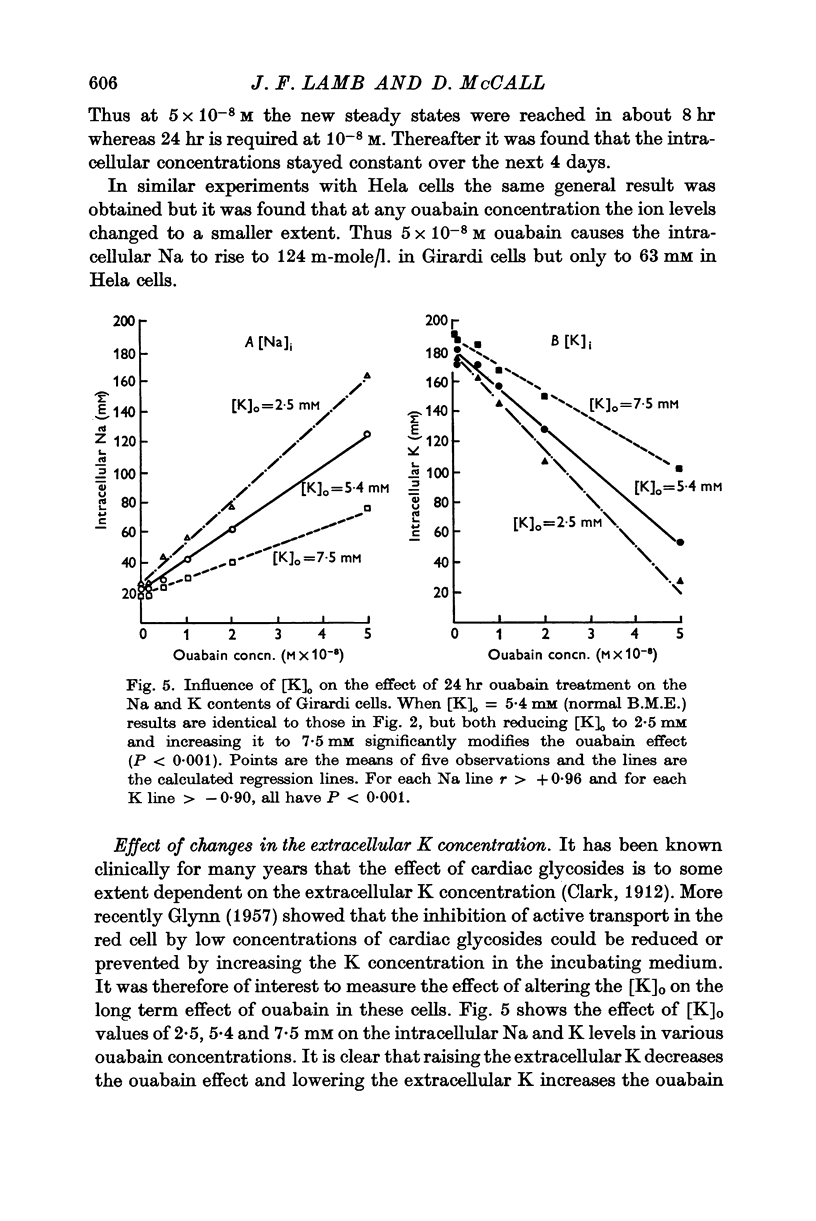
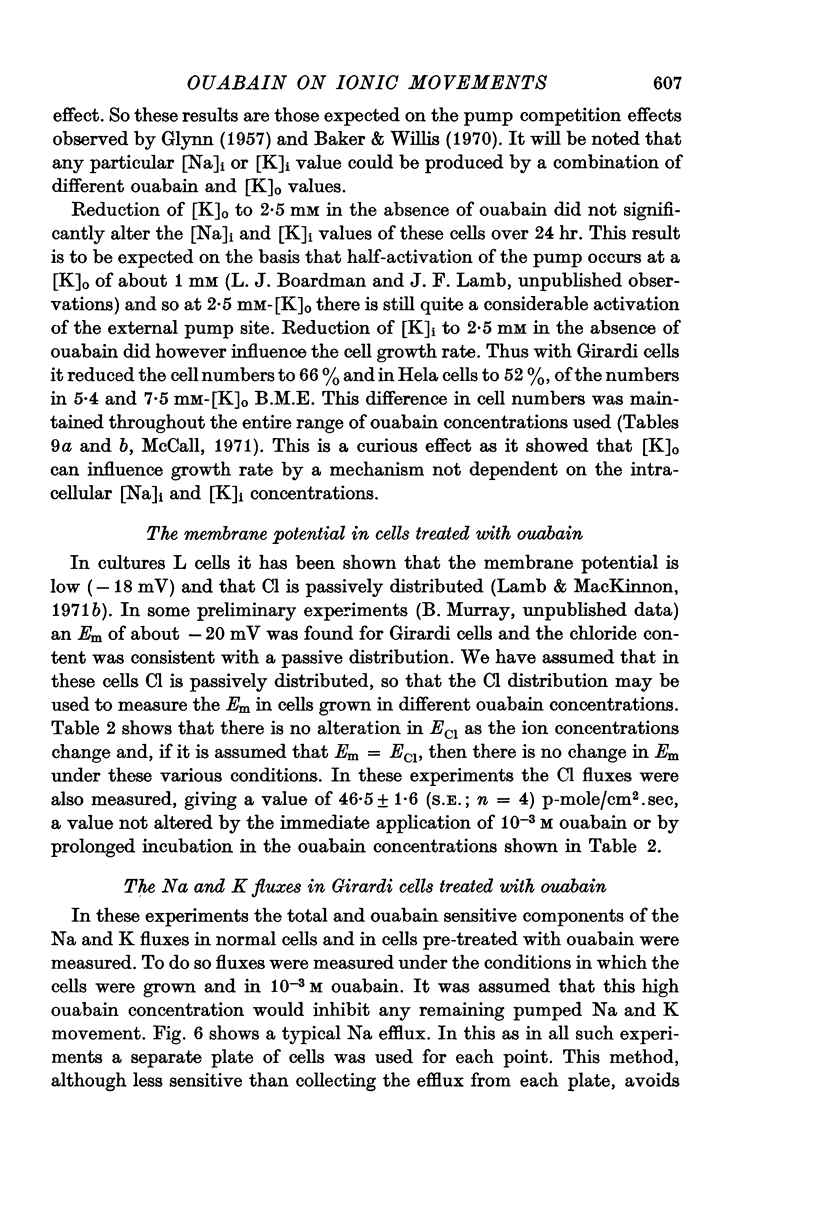
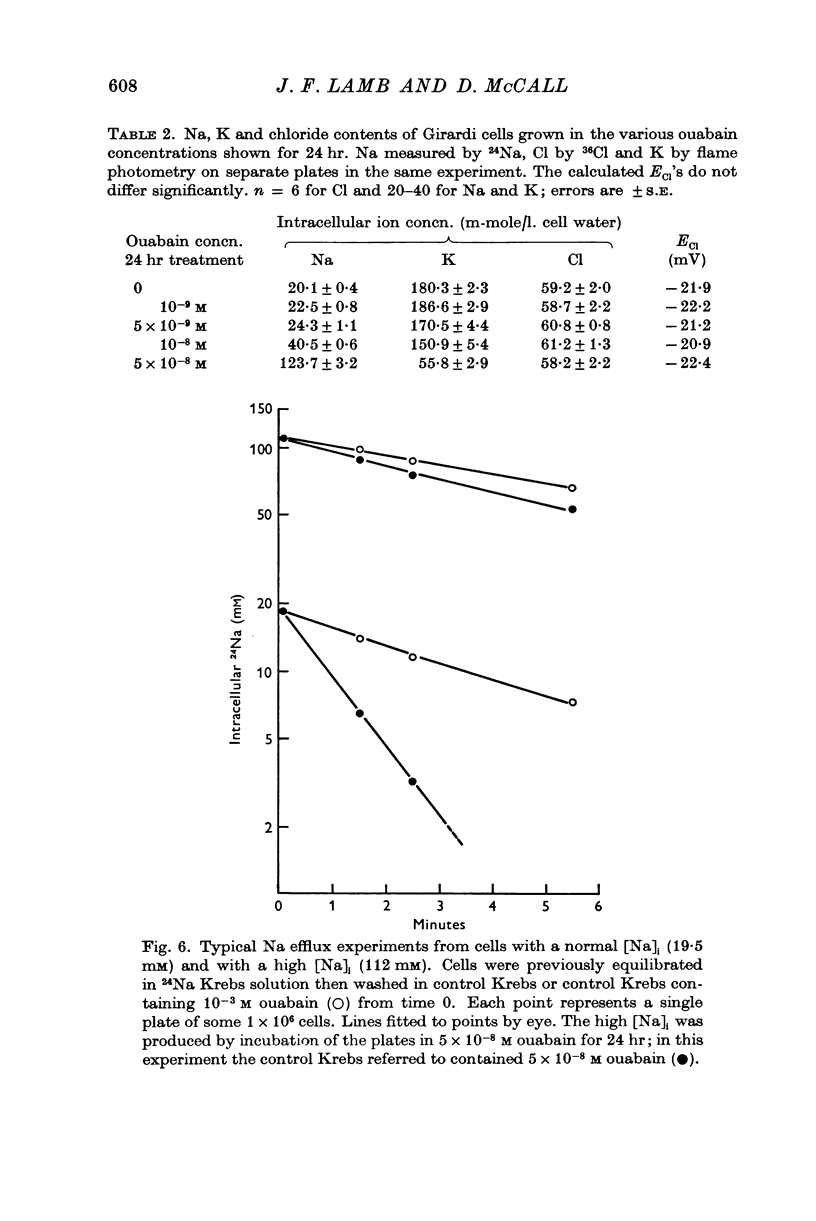
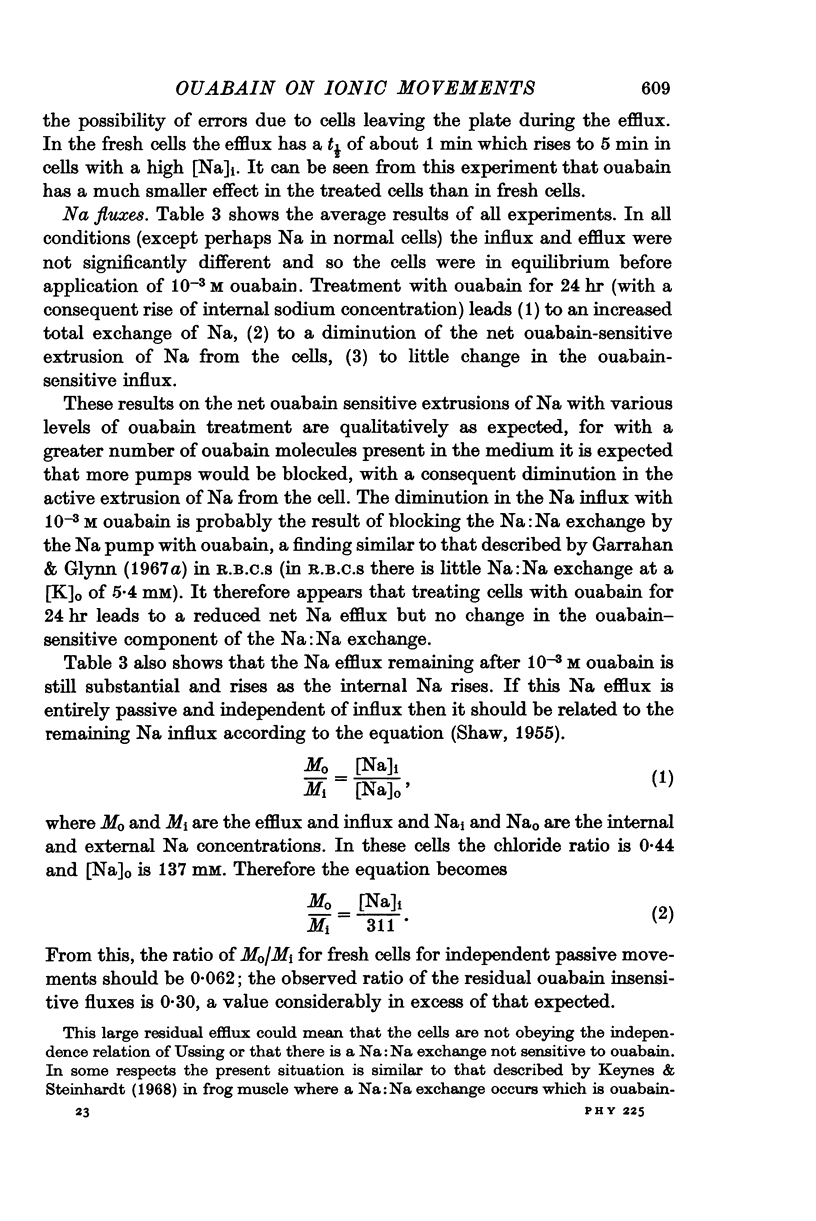
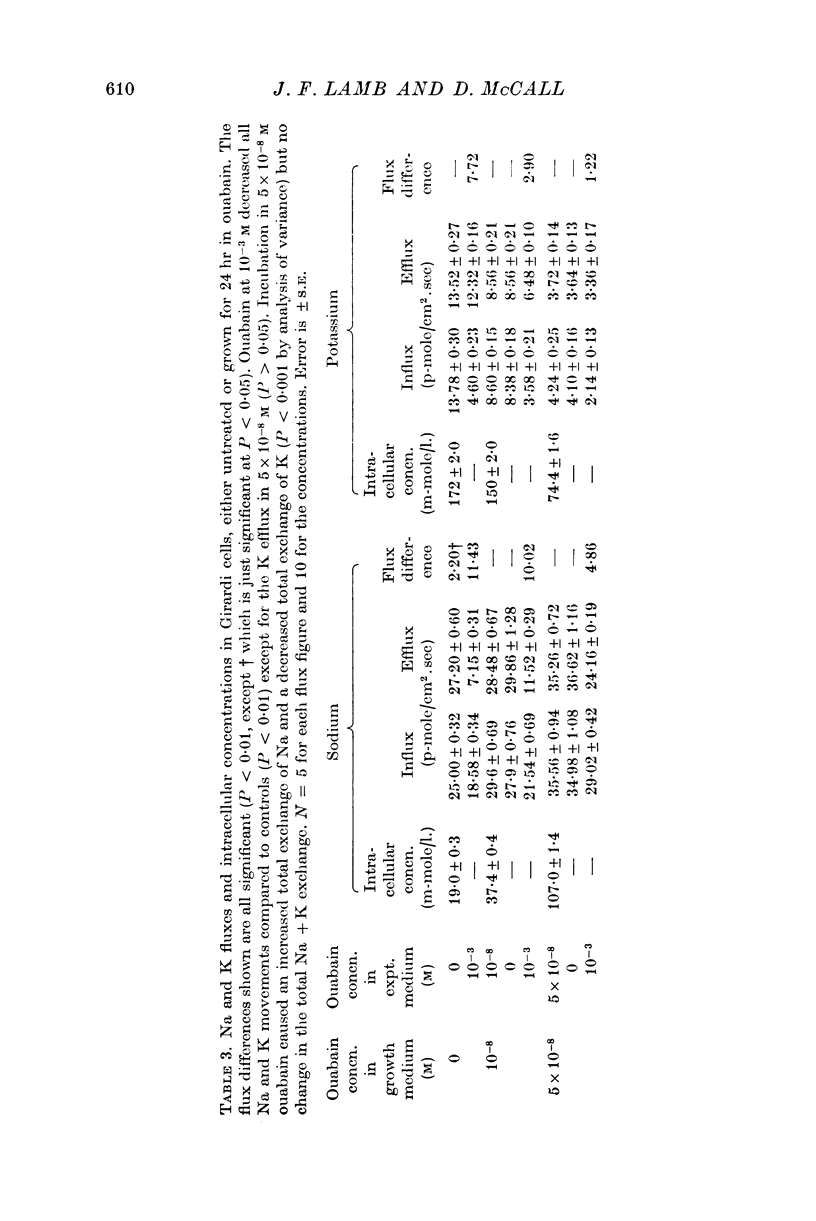
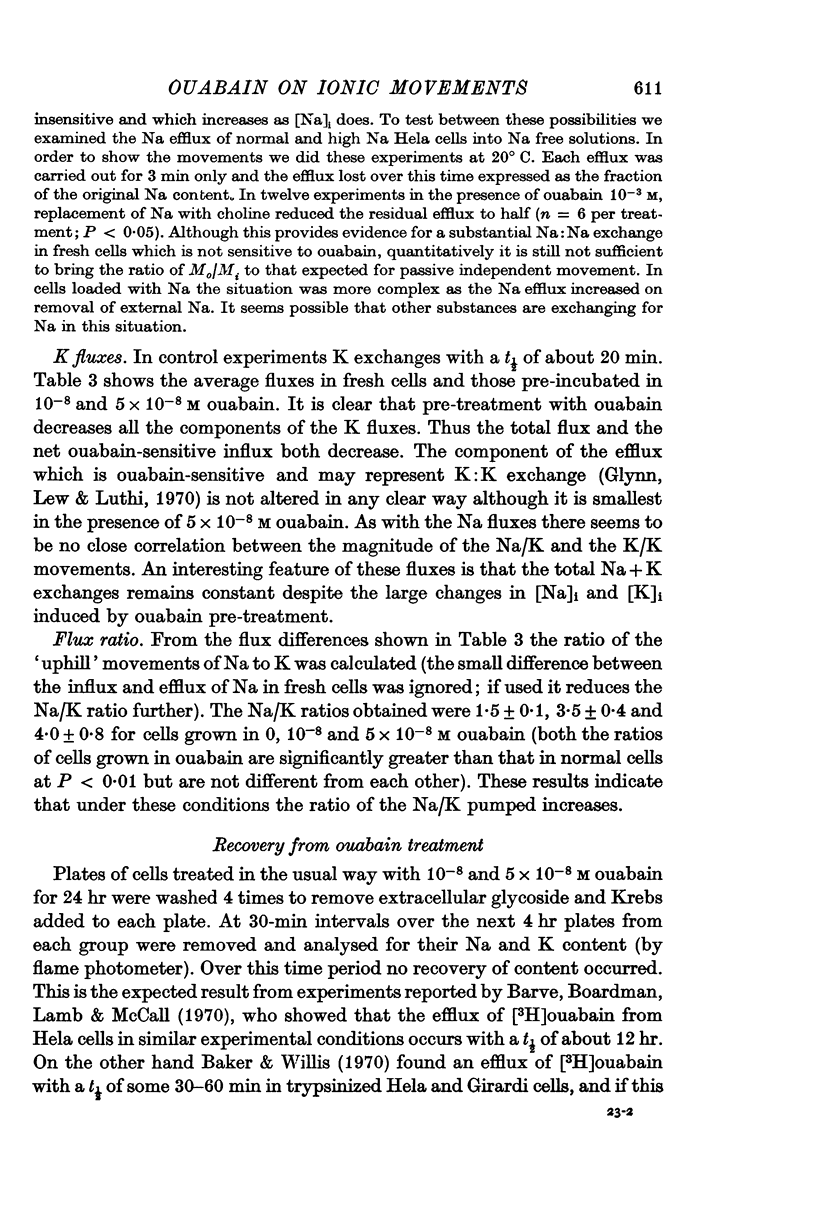
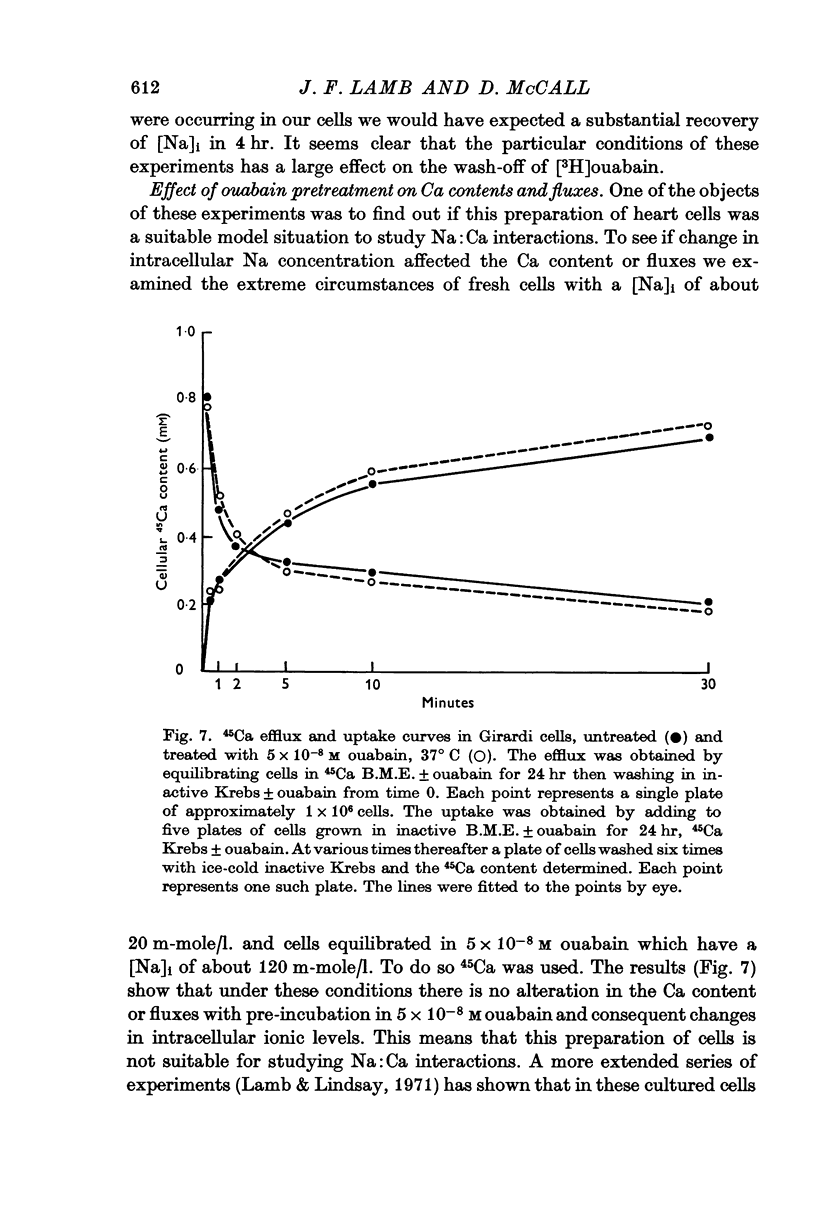
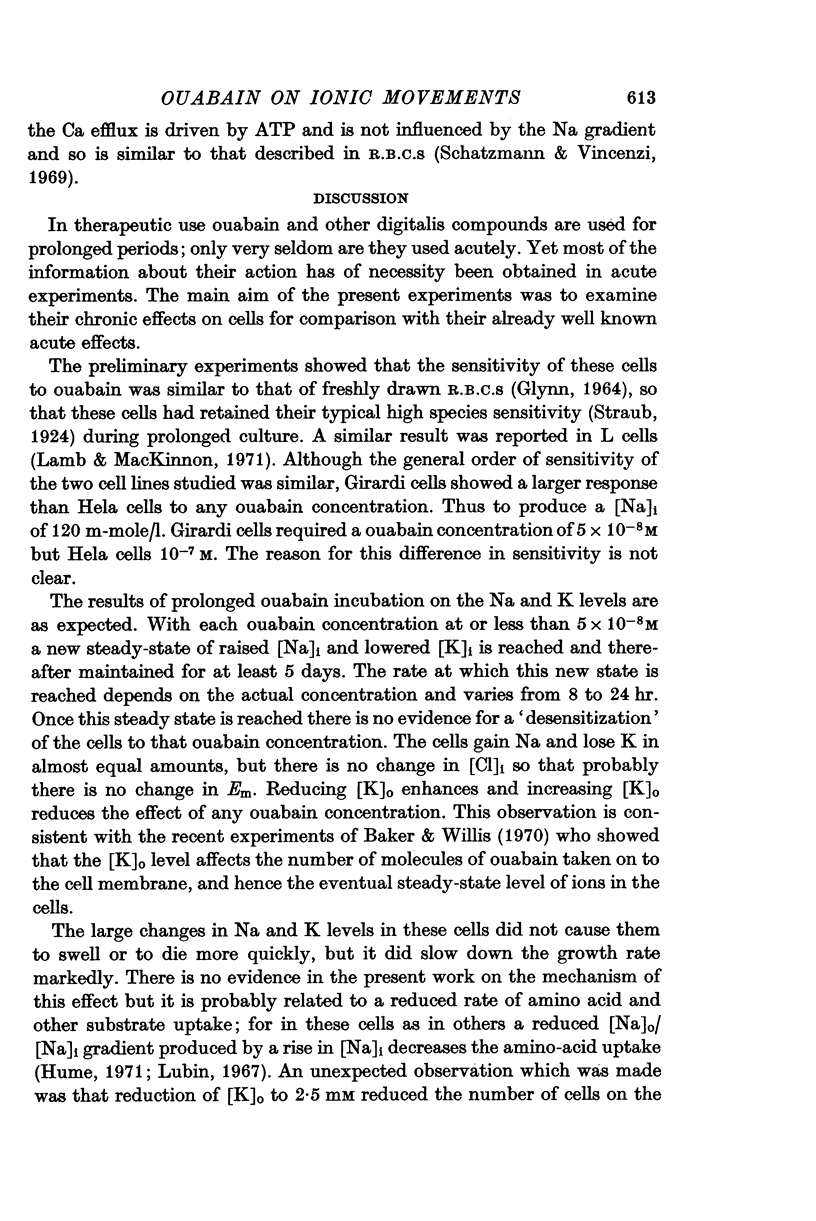
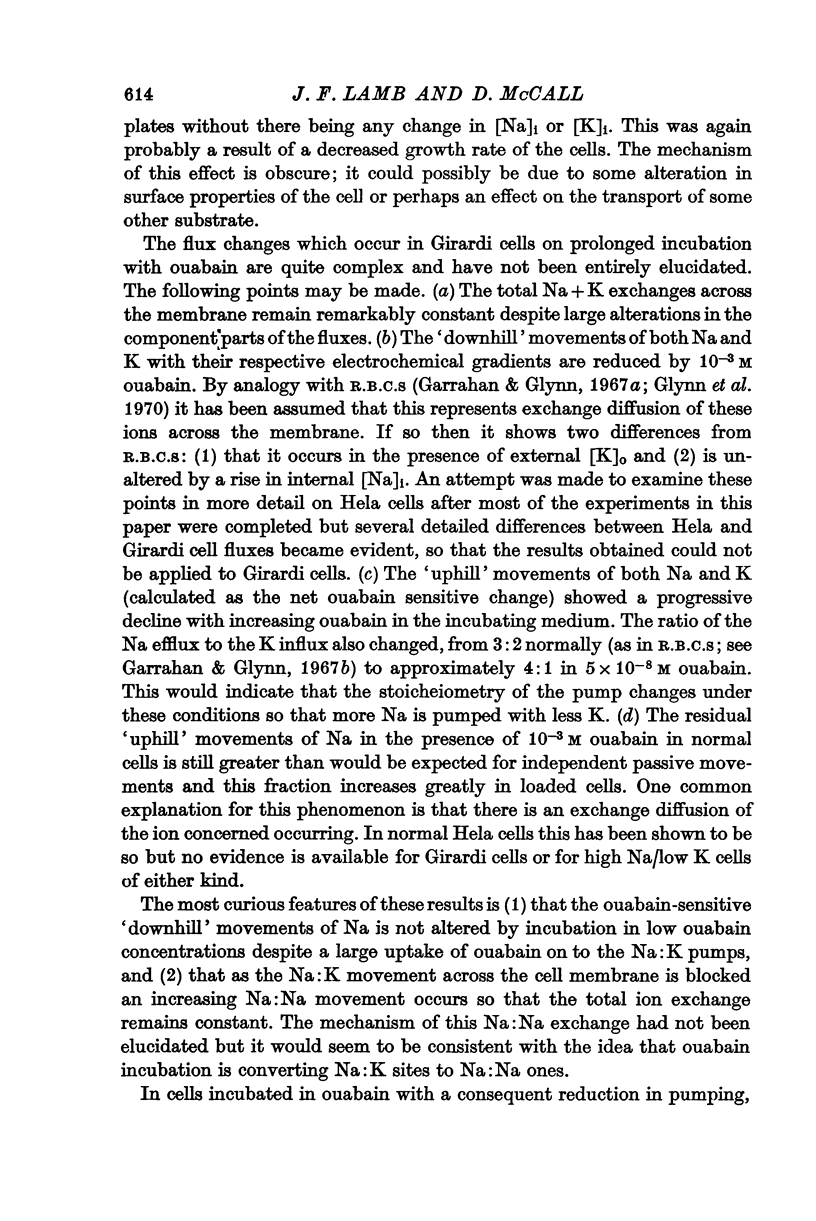
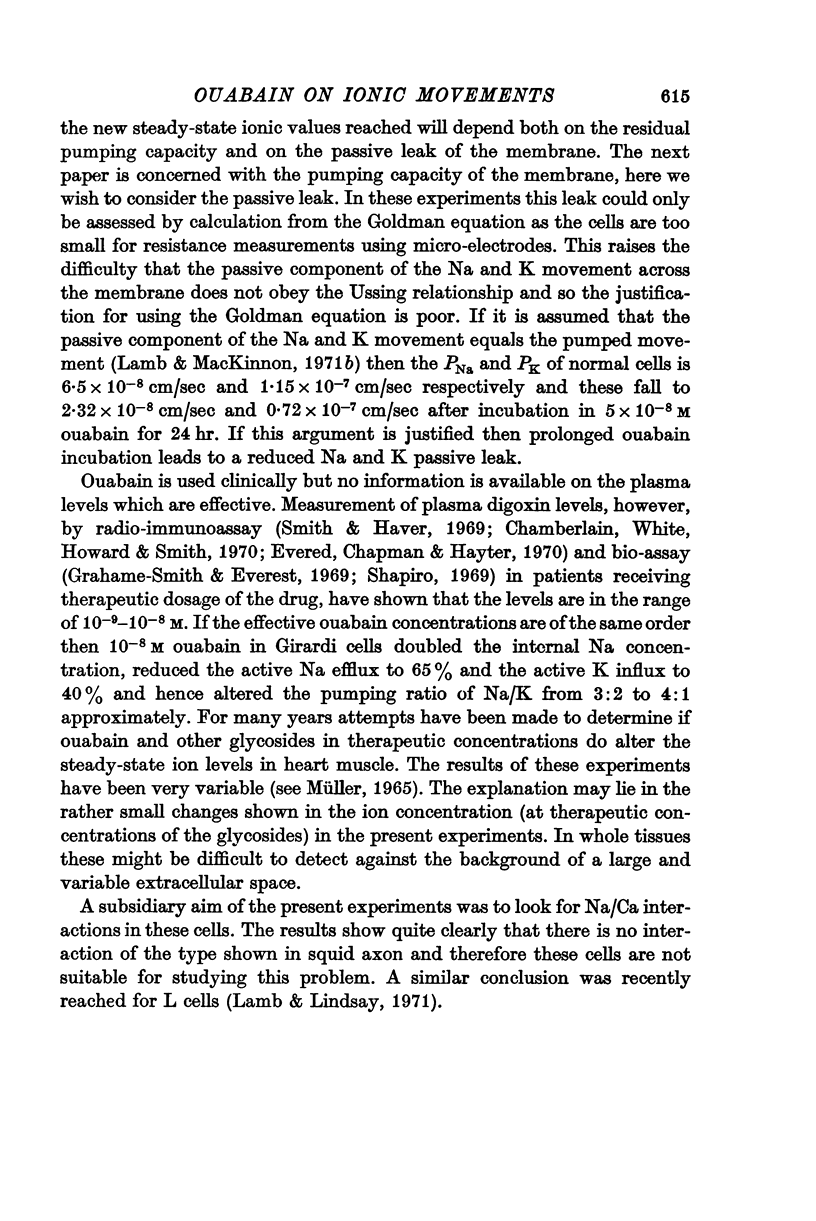
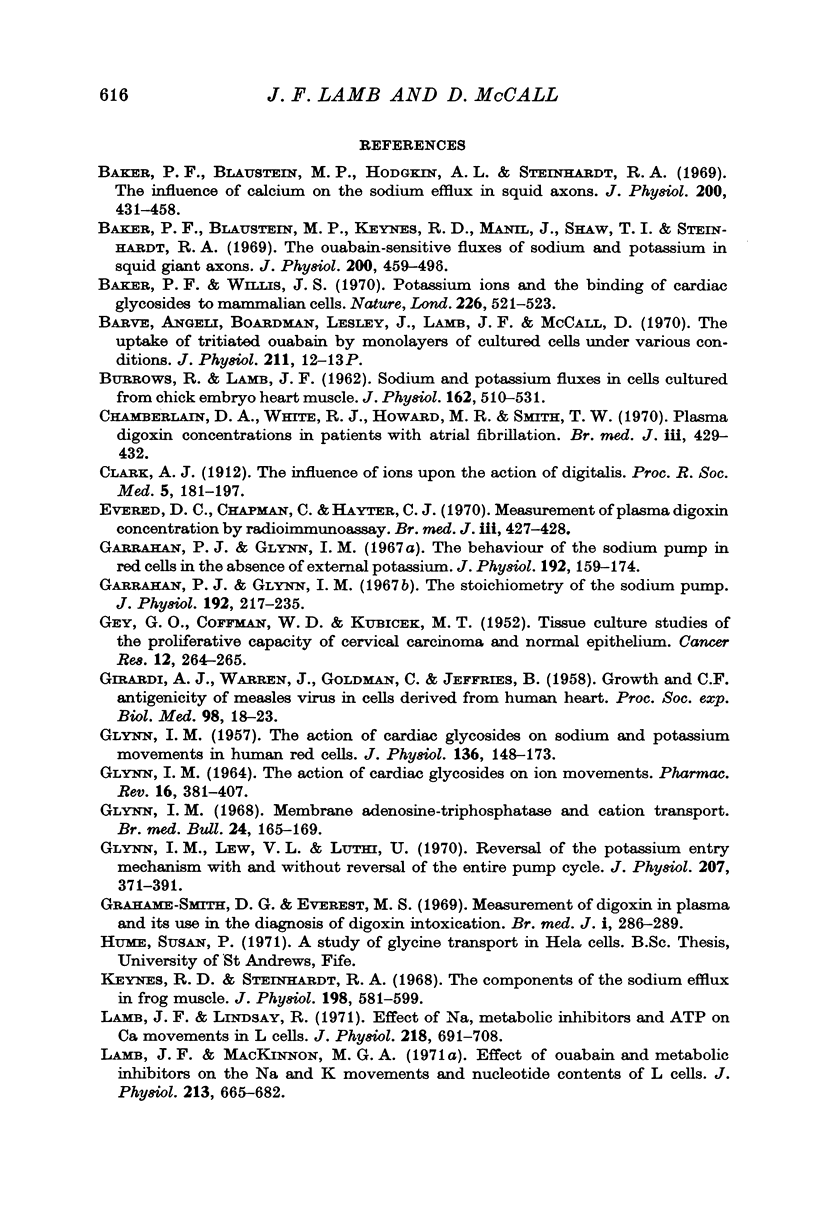
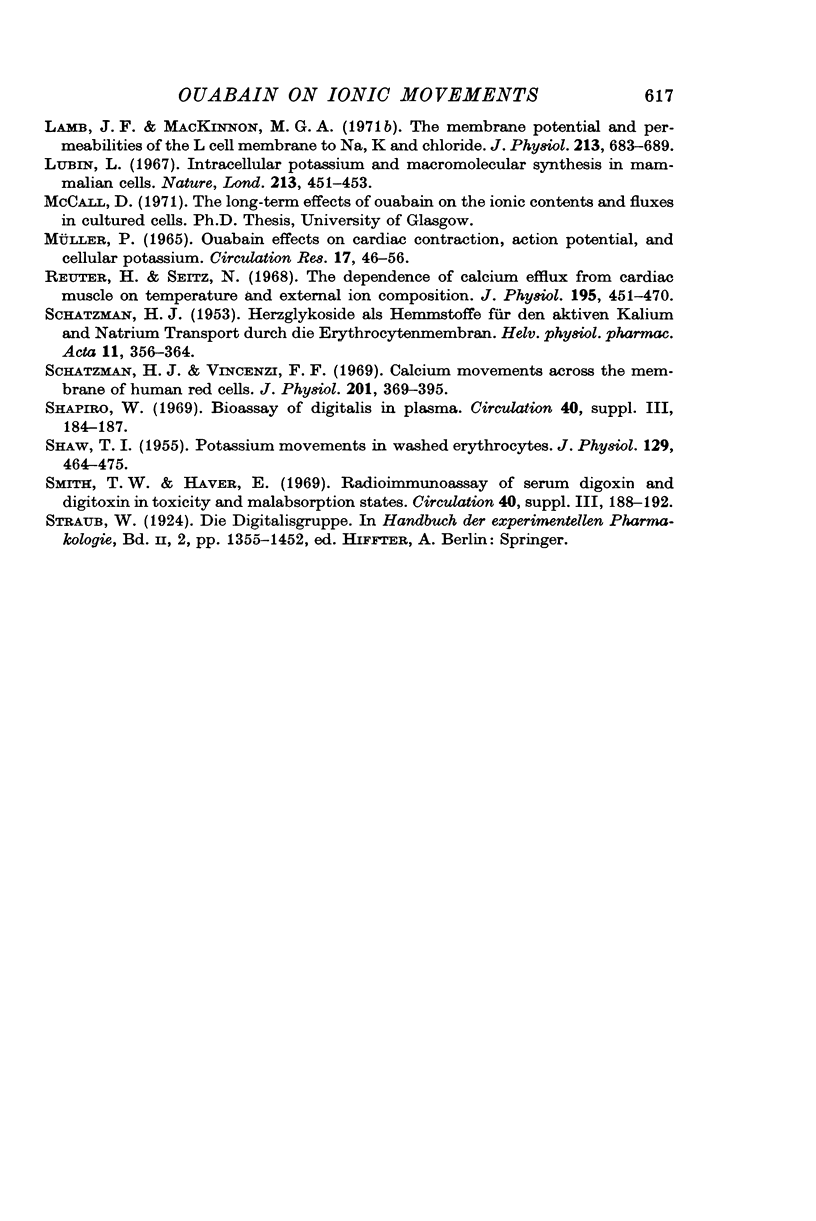
Selected References
These references are in PubMed. This may not be the complete list of references from this article.
- BURROWS R., LAMB J. F. Sodium and potassium fluxes in cells cultured from chick embryo heart muscle. J Physiol. 1962 Aug;162:510–531. doi: 10.1113/jphysiol.1962.sp006947. [DOI] [PMC free article] [PubMed] [Google Scholar]
- Baker P. F., Blaustein M. P., Hodgkin A. L., Steinhardt R. A. The influence of calcium on sodium efflux in squid axons. J Physiol. 1969 Feb;200(2):431–458. doi: 10.1113/jphysiol.1969.sp008702. [DOI] [PMC free article] [PubMed] [Google Scholar]
- Baker P. F., Blaustein M. P., Keynes R. D., Manil J., Shaw T. I., Steinhardt R. A. The ouabain-sensitive fluxes of sodium and potassium in squid giant axons. J Physiol. 1969 Feb;200(2):459–496. doi: 10.1113/jphysiol.1969.sp008703. [DOI] [PMC free article] [PubMed] [Google Scholar]
- Baker P. F., Willis J. S. Potassium ions and the binding of cardiac glycosides to mammalian cells. Nature. 1970 May 9;226(5245):521–523. doi: 10.1038/226521a0. [DOI] [PubMed] [Google Scholar]
- Chamberlain D. A., White R. J., Howard M. R., Smith T. W. Plasma digoxin concentrations in patients with atrial fibrillation. Br Med J. 1970 Aug 22;3(5720):429–432. doi: 10.1136/bmj.3.5720.429. [DOI] [PMC free article] [PubMed] [Google Scholar]
- Clark A. J. The Influence of Ions upon the Action of Digitalis. Proc R Soc Med. 1912;5(THER):181–199. [PMC free article] [PubMed] [Google Scholar]
- Evered D. C., Chapman C., Hayter C. J. Measurement of plasma digoxin concentration by radioimmunoassay. Br Med J. 1970 Aug 22;3(5720):427–428. doi: 10.1136/bmj.3.5720.427. [DOI] [PMC free article] [PubMed] [Google Scholar]
- GIRARDI A. J., WARREN J., GOLDMAN C., JEFFRIES B. Growth and CF antigenicity of measles virus in cells deriving from human heart. Proc Soc Exp Biol Med. 1958 May;98(1):18–22. doi: 10.3181/00379727-98-23926. [DOI] [PubMed] [Google Scholar]
- GLYNN I. M. THE ACTION OF CARDIAC GLYCOSIDES ON ION MOVEMENTS. Pharmacol Rev. 1964 Dec;16:381–407. [PubMed] [Google Scholar]
- GLYNN I. M. The action of cardiac glycosides on sodium and potassium movements in human red cells. J Physiol. 1957 Apr 3;136(1):148–173. doi: 10.1113/jphysiol.1957.sp005749. [DOI] [PMC free article] [PubMed] [Google Scholar]
- Garrahan P. J., Glynn I. M. The behaviour of the sodium pump in red cells in the absence of external potassium. J Physiol. 1967 Sep;192(1):159–174. doi: 10.1113/jphysiol.1967.sp008294. [DOI] [PMC free article] [PubMed] [Google Scholar]
- Garrahan P. J., Glynn I. M. The stoicheiometry of the sodium pump. J Physiol. 1967 Sep;192(1):217–235. doi: 10.1113/jphysiol.1967.sp008297. [DOI] [PMC free article] [PubMed] [Google Scholar]
- Glynn I. M., Lew V. L., Lüthi U. Reversal of the potassium entry mechanism in red cells, with and without reversal of the entire pump cycle. J Physiol. 1970 Apr;207(2):371–391. doi: 10.1113/jphysiol.1970.sp009067. [DOI] [PMC free article] [PubMed] [Google Scholar]
- Glynn I. M. Membrane adenosine triphosphatase and cation transport. Br Med Bull. 1968 May;24(2):165–169. doi: 10.1093/oxfordjournals.bmb.a070620. [DOI] [PubMed] [Google Scholar]
- Grahame-Smith D. G. Everest MS: Measurement of digoxin in plasma and its use in diagnosis of digoxin intoxication. Br Med J. 1969 Feb 1;1(5639):286–289. doi: 10.1136/bmj.1.5639.286. [DOI] [PMC free article] [PubMed] [Google Scholar]
- Keynes R. D., Steinhardt R. A. The components of the sodium efflux in frog muscle. J Physiol. 1968 Oct;198(3):581–599. doi: 10.1113/jphysiol.1968.sp008627. [DOI] [PMC free article] [PubMed] [Google Scholar]
- Lamb J. F., Lindsay R. Effect of Na, metabolic inhibitors and ATP on Ca movements in L cells. J Physiol. 1971 Nov;218(3):691–708. doi: 10.1113/jphysiol.1971.sp009640. [DOI] [PMC free article] [PubMed] [Google Scholar]
- Lamb J. F., MacKinnon M. G. Effect of ouabain and metabolic inhibitors on the Na and K movements and nucleotide contents of L cells. J Physiol. 1971 Mar;213(3):665–682. doi: 10.1113/jphysiol.1971.sp009407. [DOI] [PMC free article] [PubMed] [Google Scholar]
- Lubin M. Intracellular potassium and macromolecular synthesis in mammalian cells. Nature. 1967 Feb 4;213(5075):451–453. doi: 10.1038/213451a0. [DOI] [PubMed] [Google Scholar]
- MUELLER P. OUABAIN EFFECTS ON CARDIAC CONTRACTION, ACTION POTENTIAL, AND CELLULAR POTASSIUM. Circ Res. 1965 Jul;17:46–56. doi: 10.1161/01.res.17.1.46. [DOI] [PubMed] [Google Scholar]
- Reuter H., Seitz N. The dependence of calcium efflux from cardiac muscle on temperature and external ion composition. J Physiol. 1968 Mar;195(2):451–470. doi: 10.1113/jphysiol.1968.sp008467. [DOI] [PMC free article] [PubMed] [Google Scholar]
- SHAW T. I. Potassium movements in washed erythrocytes. J Physiol. 1955 Sep 28;129(3):464–475. doi: 10.1113/jphysiol.1955.sp005371. [DOI] [PMC free article] [PubMed] [Google Scholar]
- Schatzmann H. J., Vincenzi F. F. Calcium movements across the membrane of human red cells. J Physiol. 1969 Apr;201(2):369–395. doi: 10.1113/jphysiol.1969.sp008761. [DOI] [PMC free article] [PubMed] [Google Scholar]