Abstract
1. The early membrane currents in skeletal muscle fibres have been studied with the double sucrose-gap method, according to the experimental procedure used for the study of the giant axon of the squid.
2. The earliest observable current is a capacitative transient. An equivalent circuit with two capacity-resistance systems can account for such a current. The components of the electrical circuit are estimated from the records.
3. The capacitative transient current is followed by an initial current, the time and voltage dependence of which are analogous to those of nerve fibres. During this phase of current, the membrane behaves like a sodium electrode for modifications of the external sodium concentration.
4. Using tetrodotoxin, a specific inhibitor of the sodium permeability, it is possible to separate two current components, (i) a transient component, suppressed by tetrodotoxin, which reflects the modifications, with time and voltage, of the membrane permeability towards sodium ions; the larger the depolarization, the shorter the time to reach peak current. This current is inward for depolarizations (V) of about +30 mV to about +130 mV (VNa). There was some variation in these values between individual fibres. Beyond VNa, the current is outward. This current shows a small rectification — inward going — for high depolarizations which is comparable to the behaviour of the Ranvier node membrane. (ii) A quasi-instantaneous outward component, only voltage dependent. The current—voltage relation of this current shows an outward going rectification for high depolarizations. This current can be considered as a `leak' current, in which chloride ions could play an important role.
5. A sodium tail current is observed when the imposed potential fall occurs during the time course of the initial current. It corresponds to the deactivation of the sodium current previously activated. Because of the large contemporary capacitative currents, it is difficult to measure accurately its maximum amplitude and its time course: yet, the instantaneous current—voltage relation seems to show a slight rectification for the largest depolarizations, as observed on the Ranvier node membrane.
6. The inactivation properties of the sodium current are studied in experiments similar to those described by Hodgkin & Huxley. As in nerve fibres the ability of the membrane to undergo an increase of the sodium permeability depends on the membrane potential. In our experimental conditions, the availability of the sodium carrying system is 90 per cent at the resting potential; the half-inactivation occurs for V = +11 mV. This potential value corresponds to the smallest value of τh-1, in agreement with the equations proposed by Hodgkin & Huxley (1952d) for the inactivation process.
7. The activation parameters (m∞, τm) are determined by a fitting method, taking account of the values of τh previously established. The potential of half-activation is near V = +40 mV.
Full text
PDF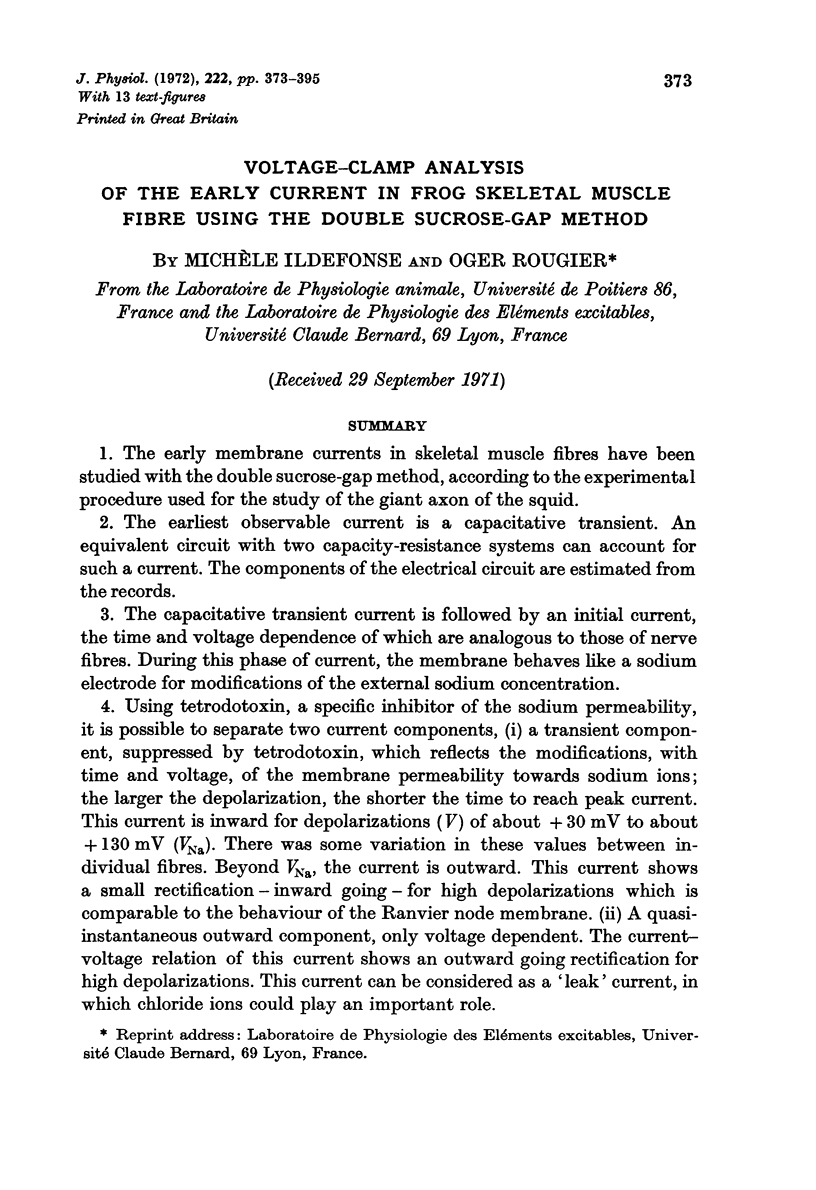
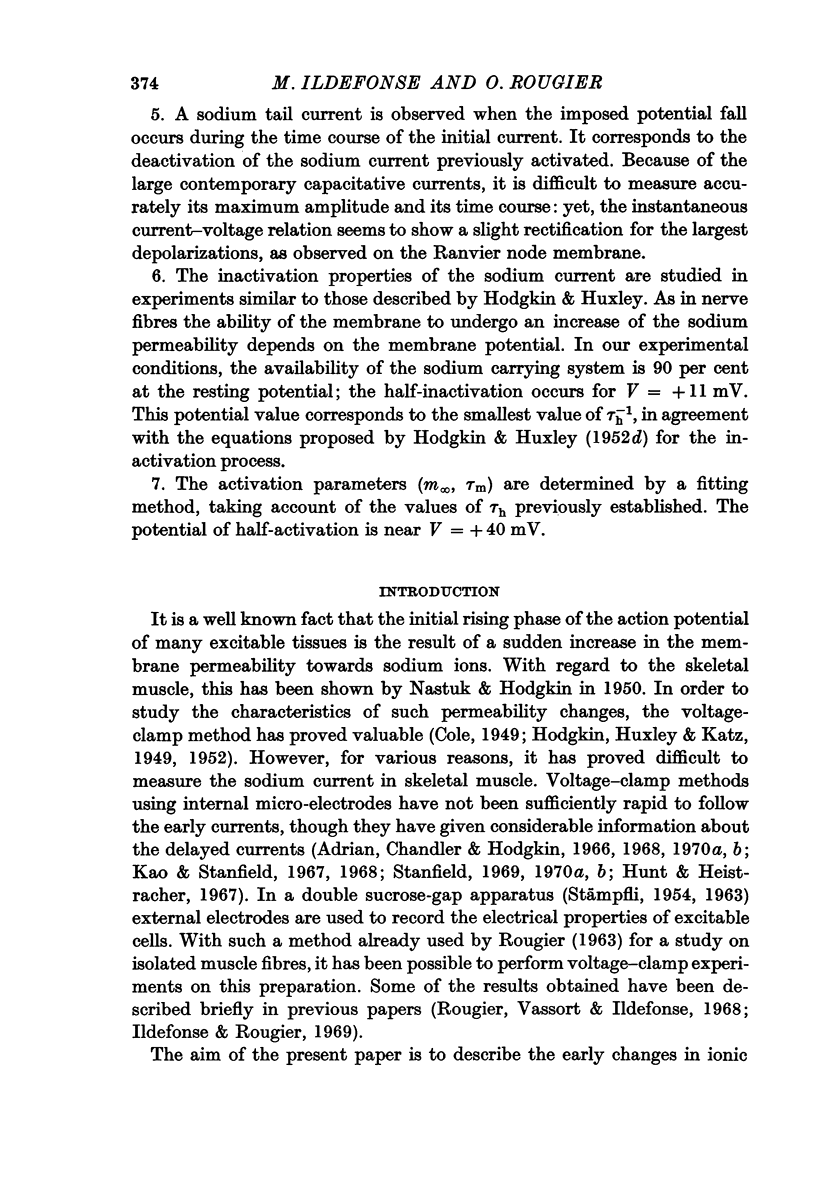
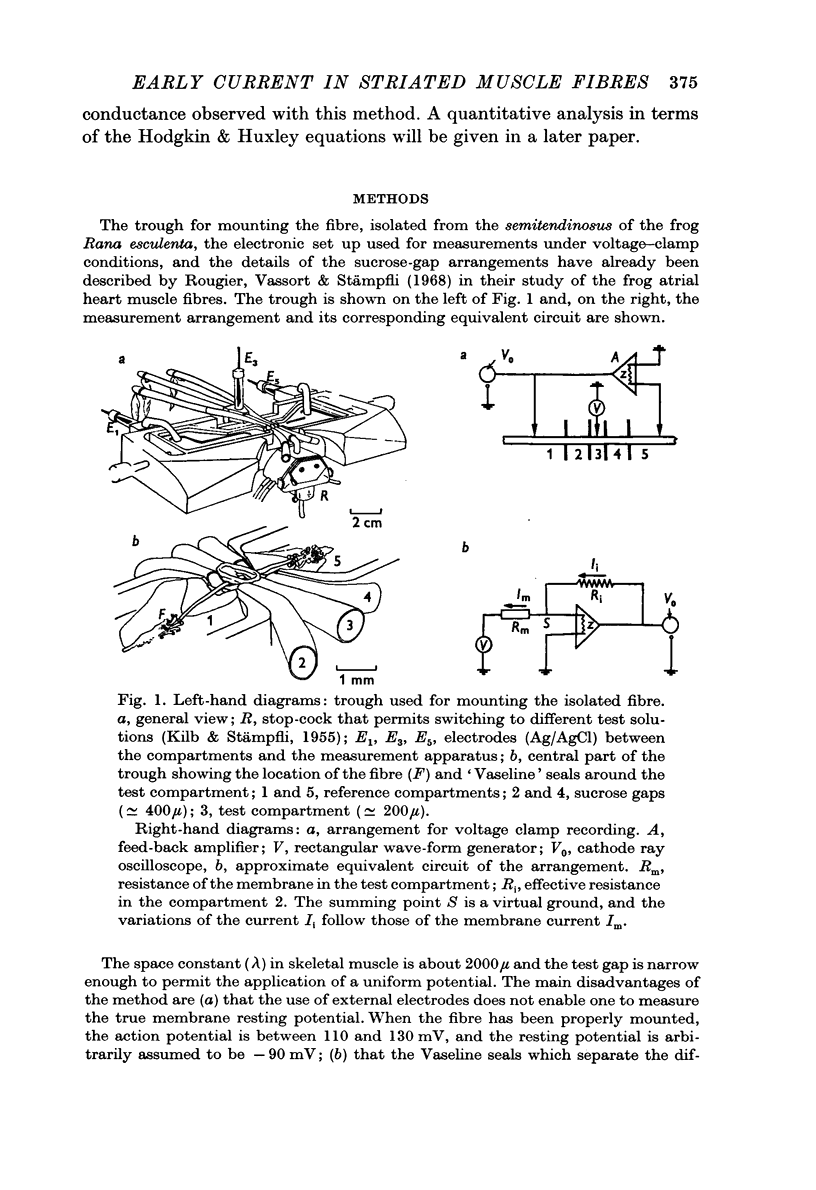
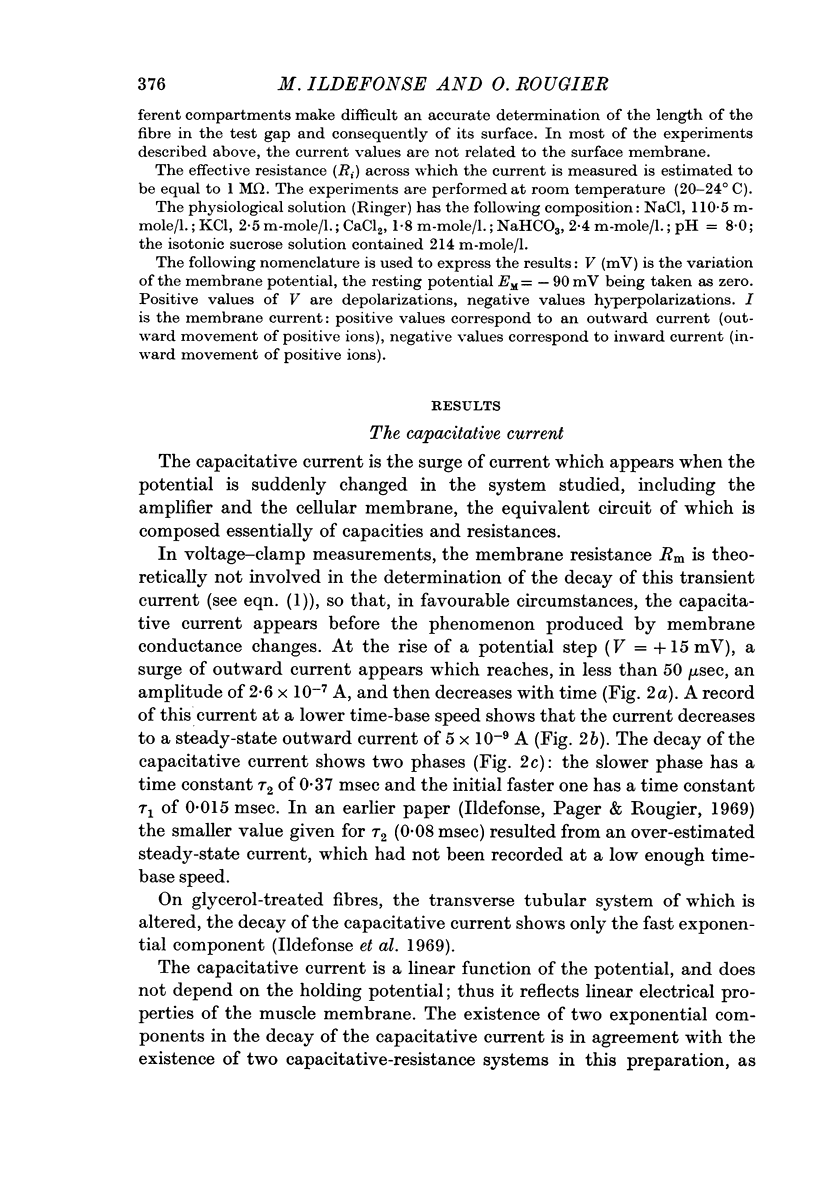
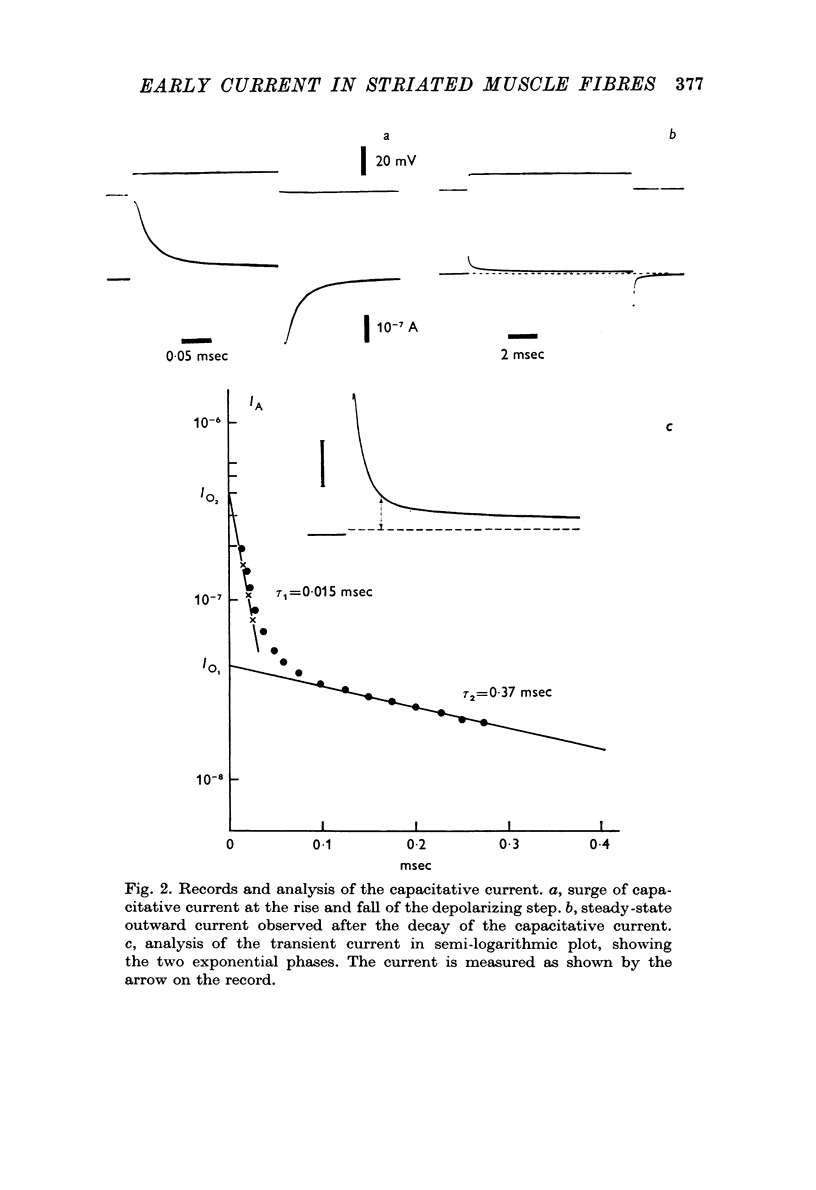
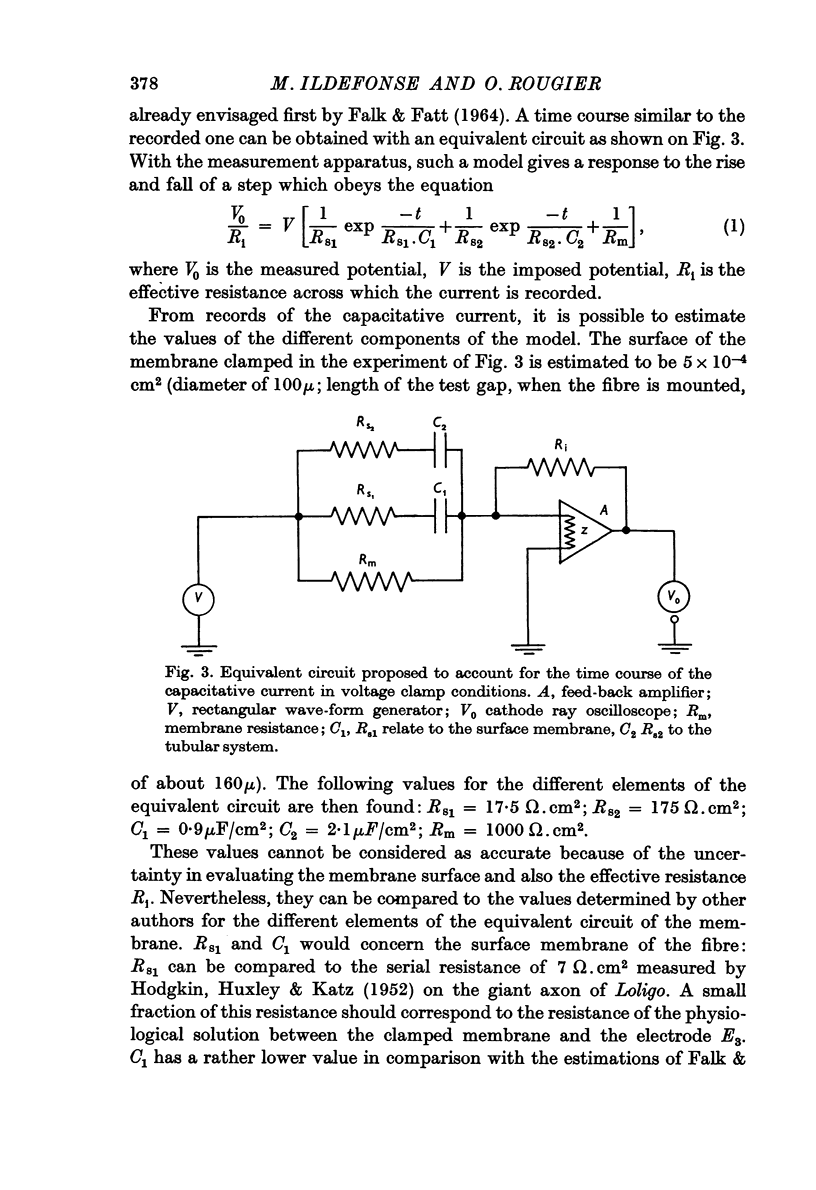
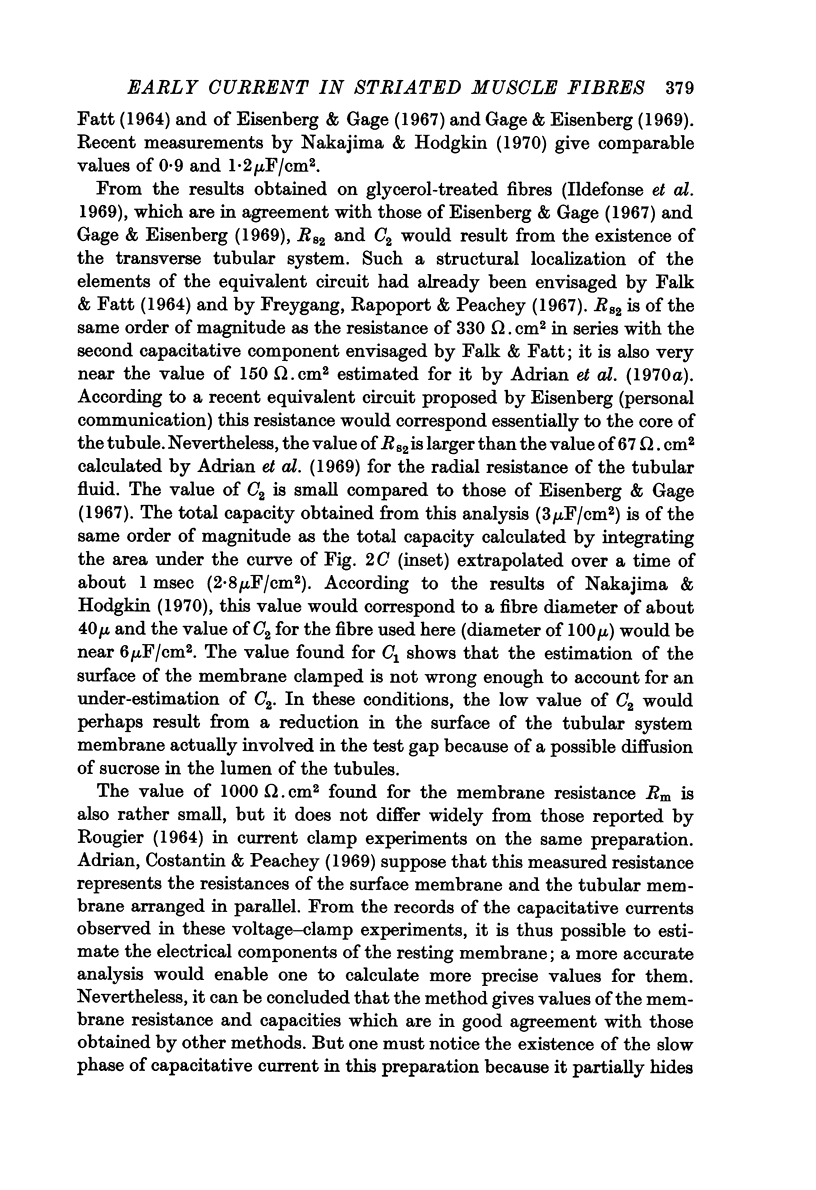
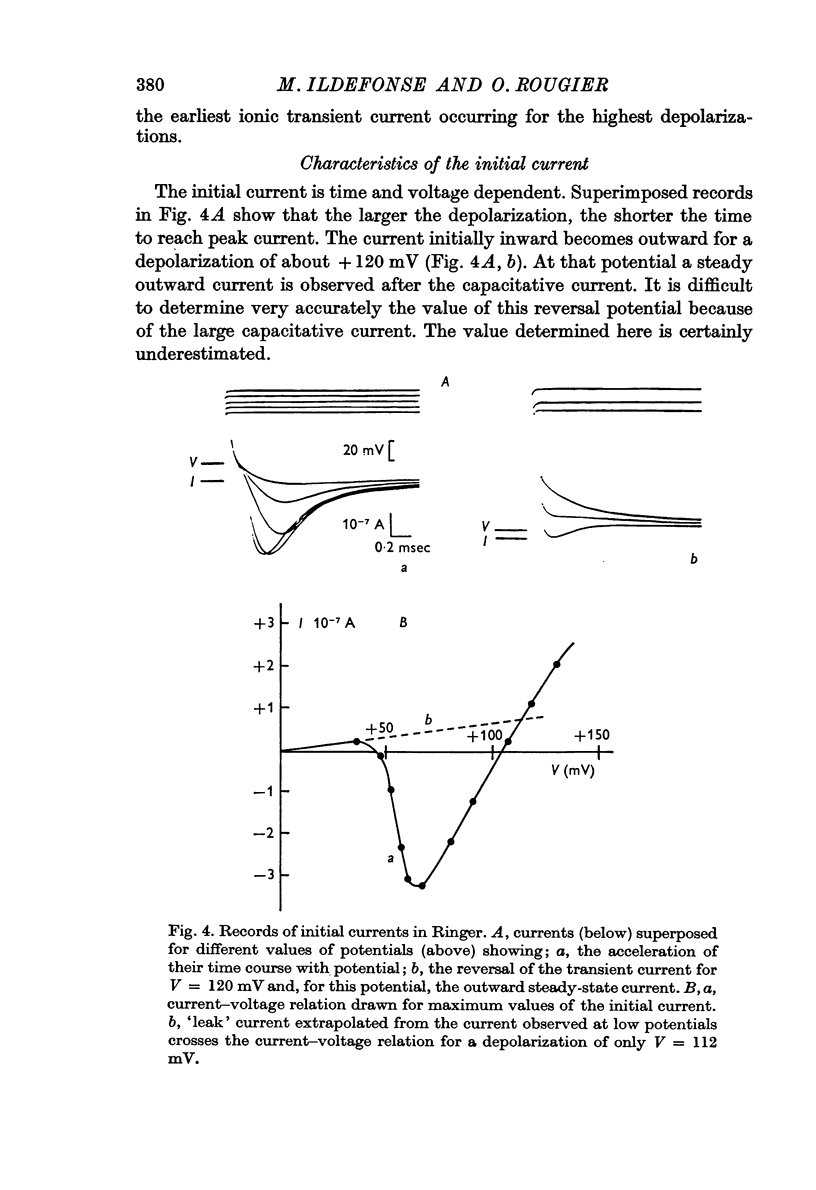
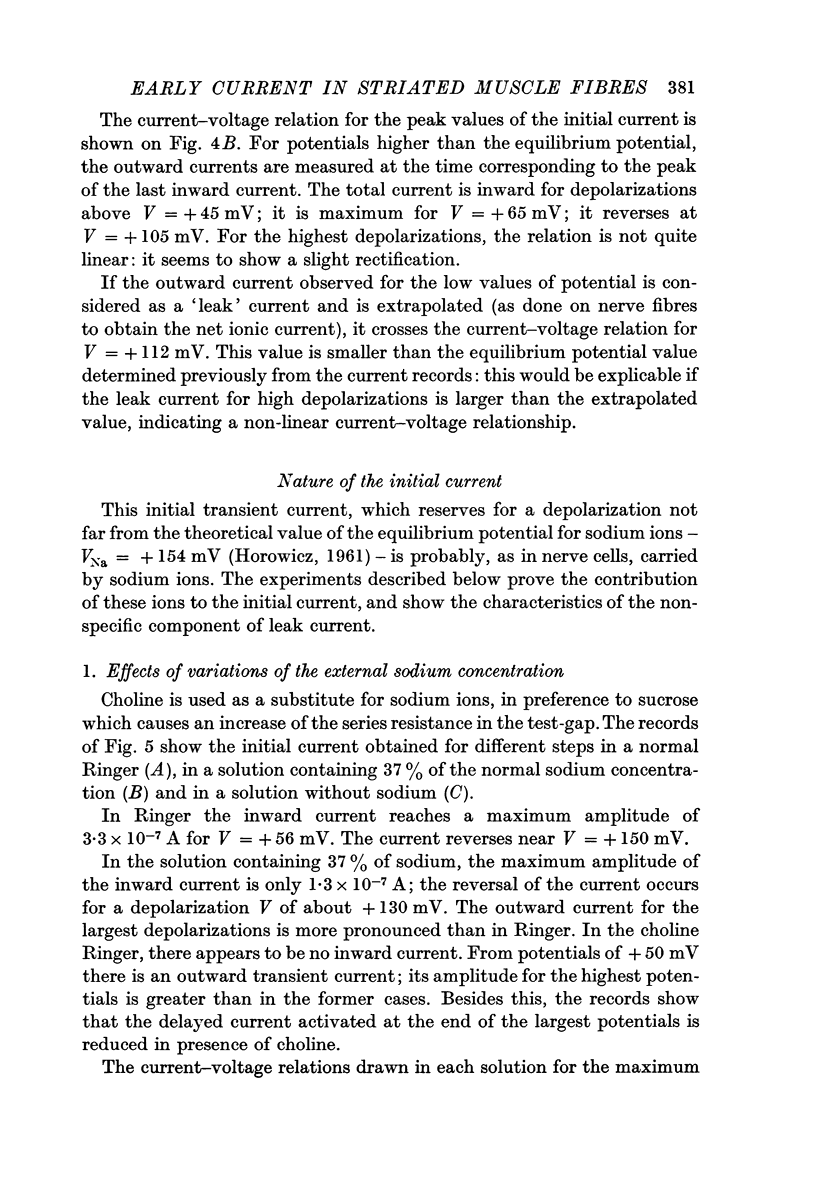
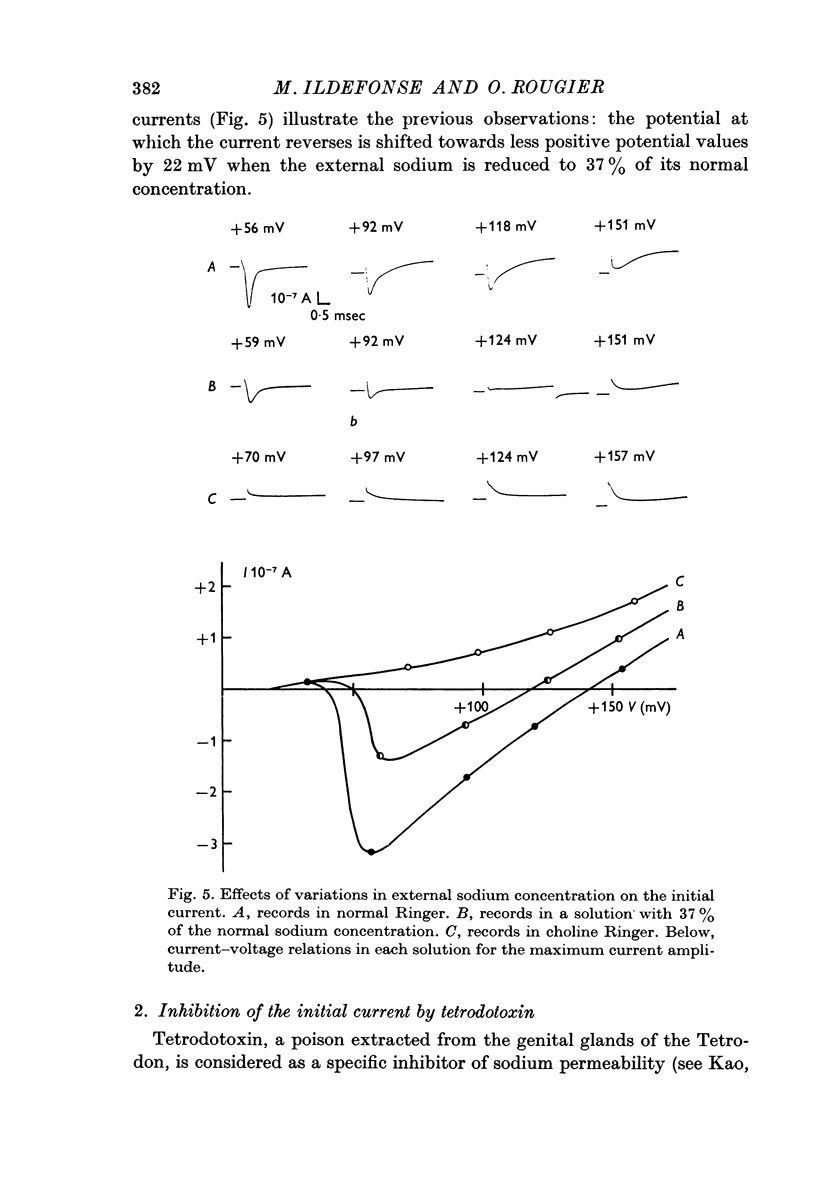
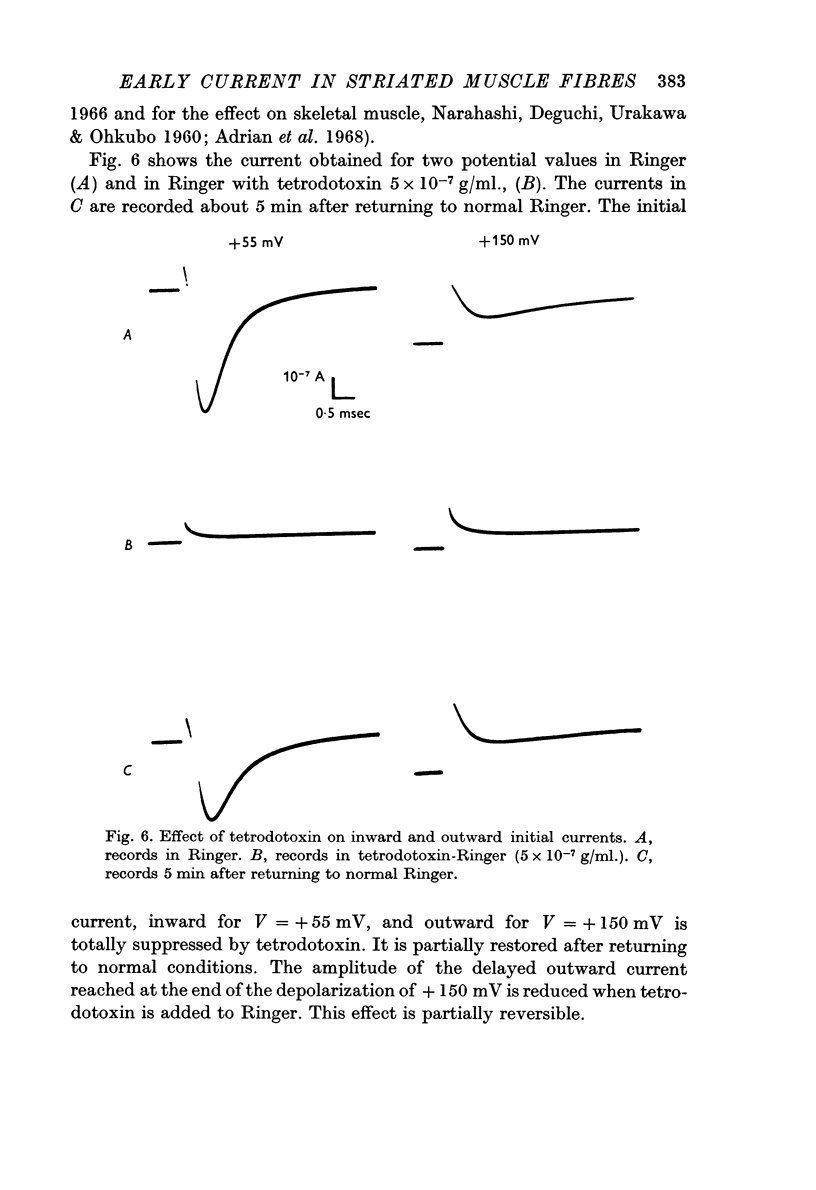
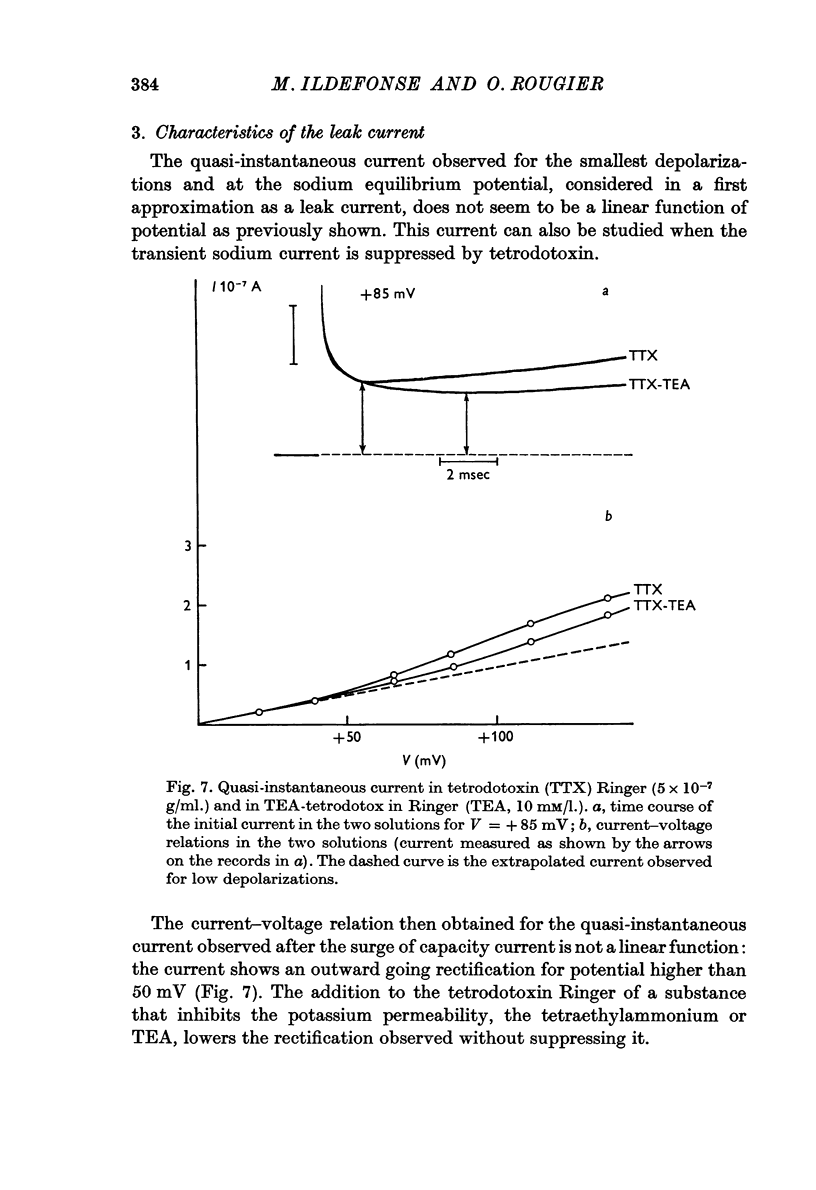
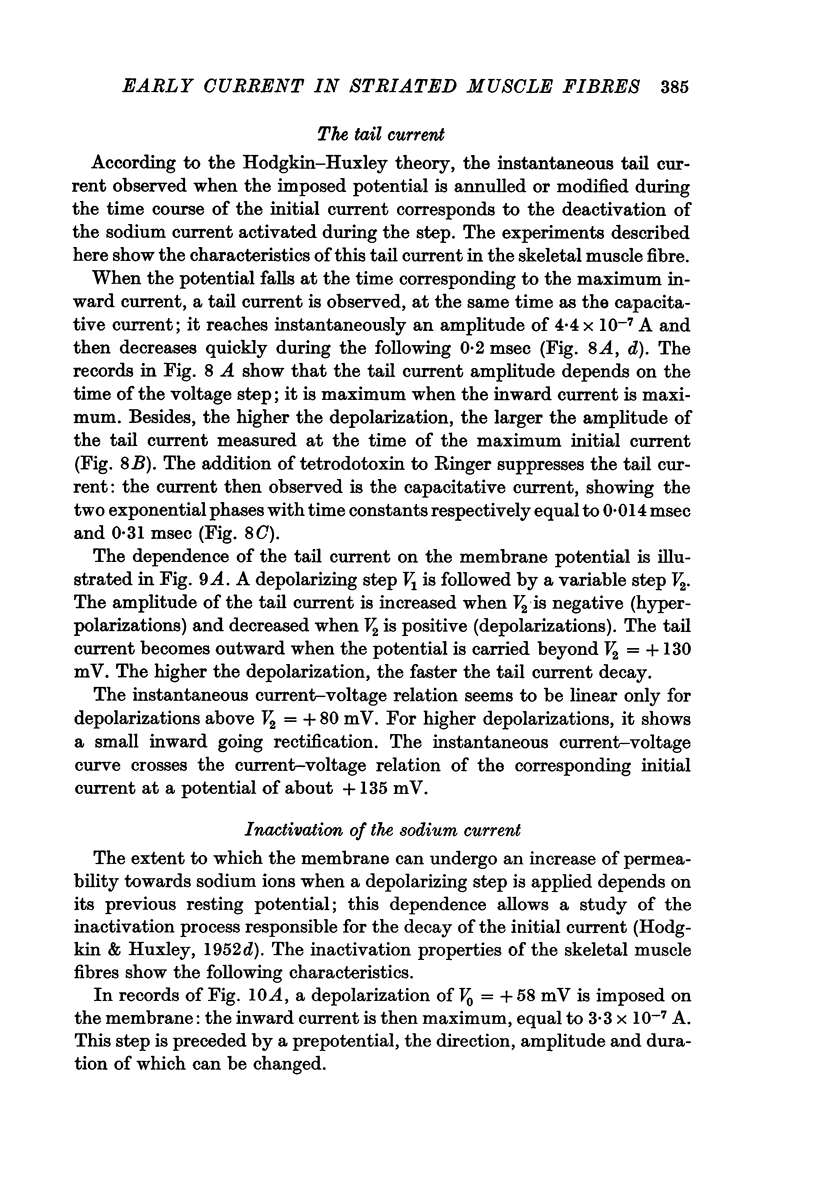
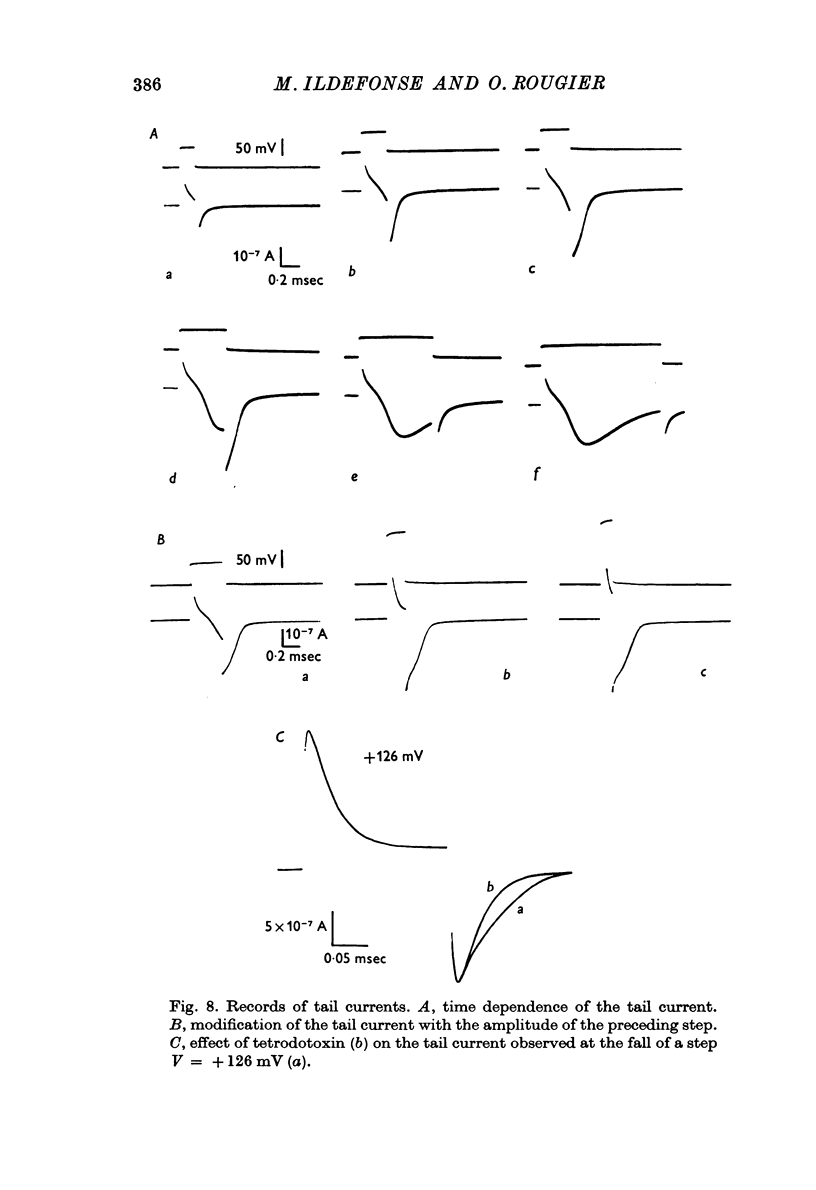
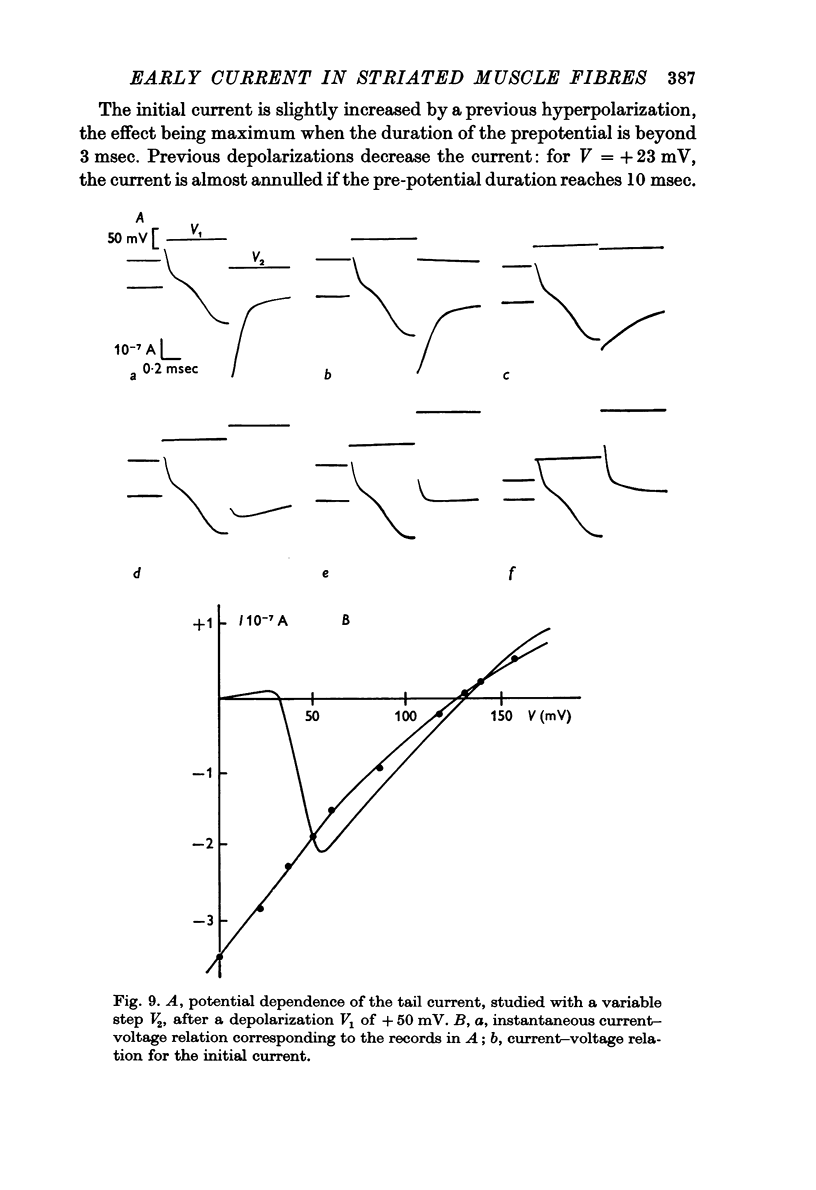
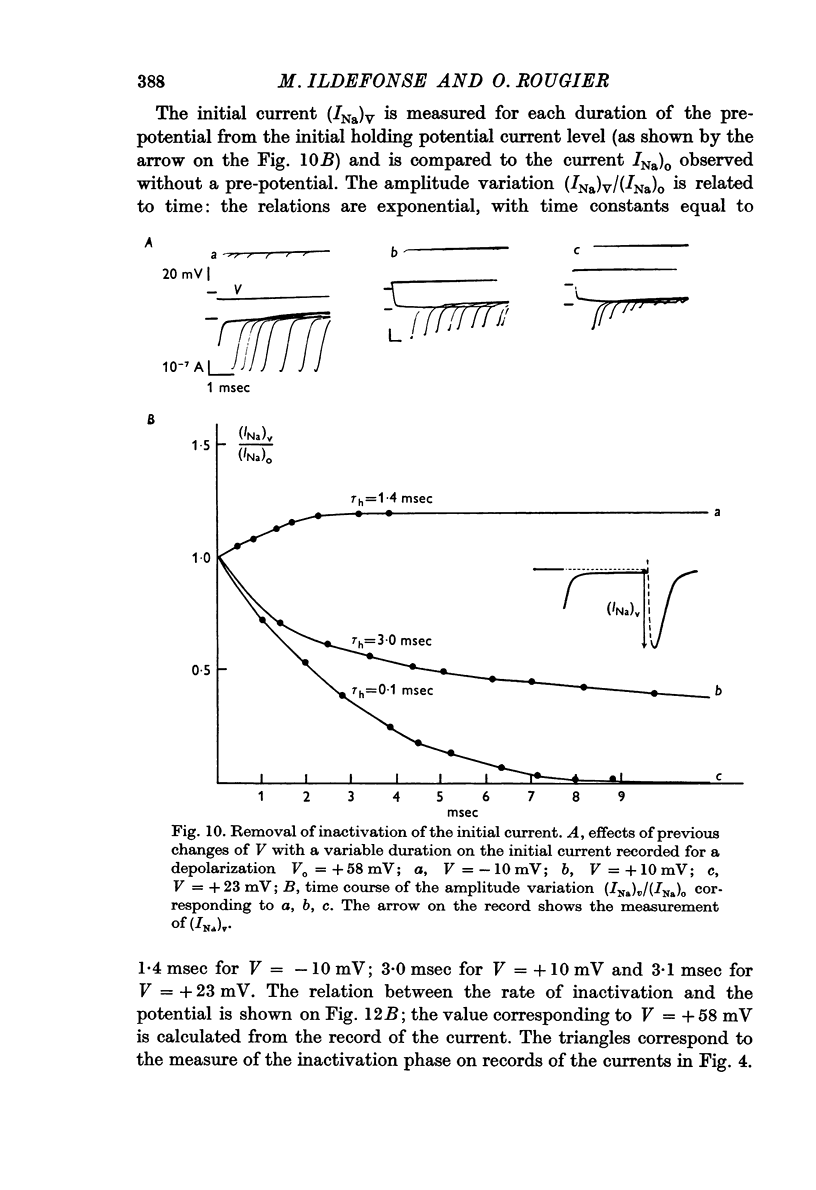
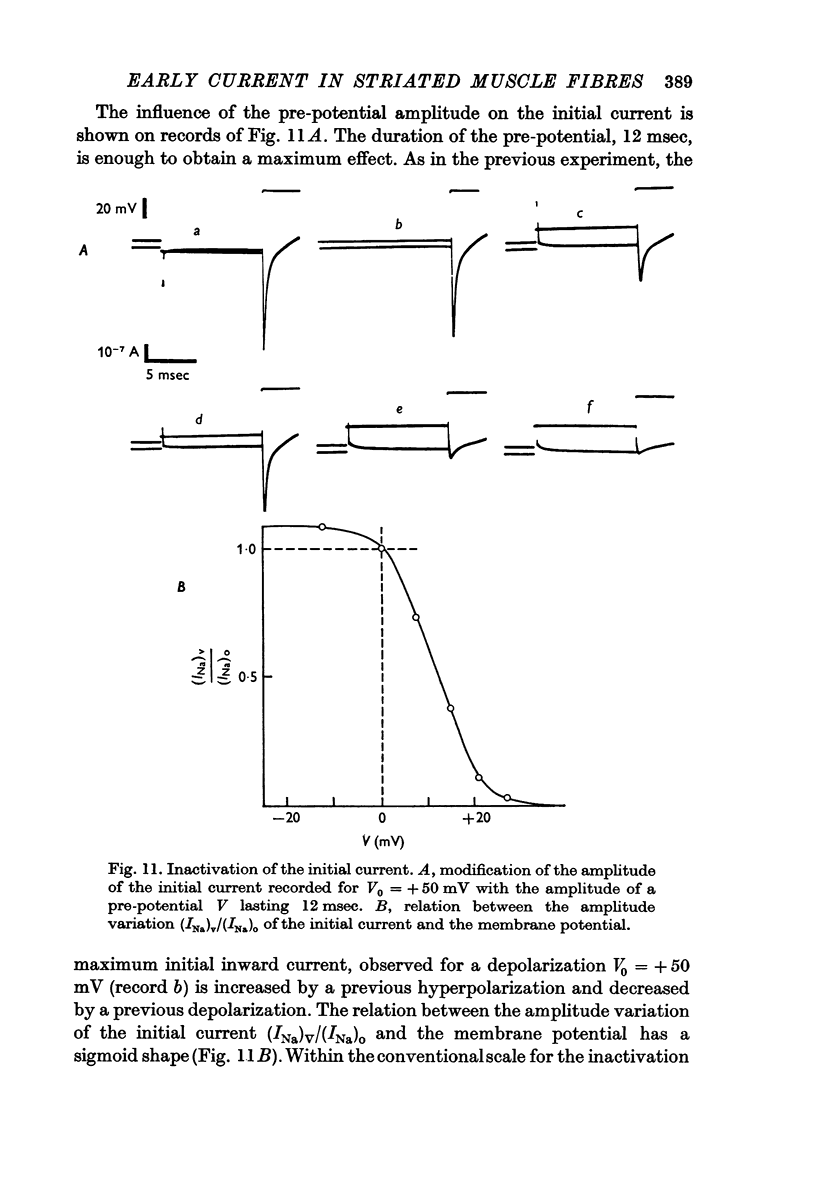
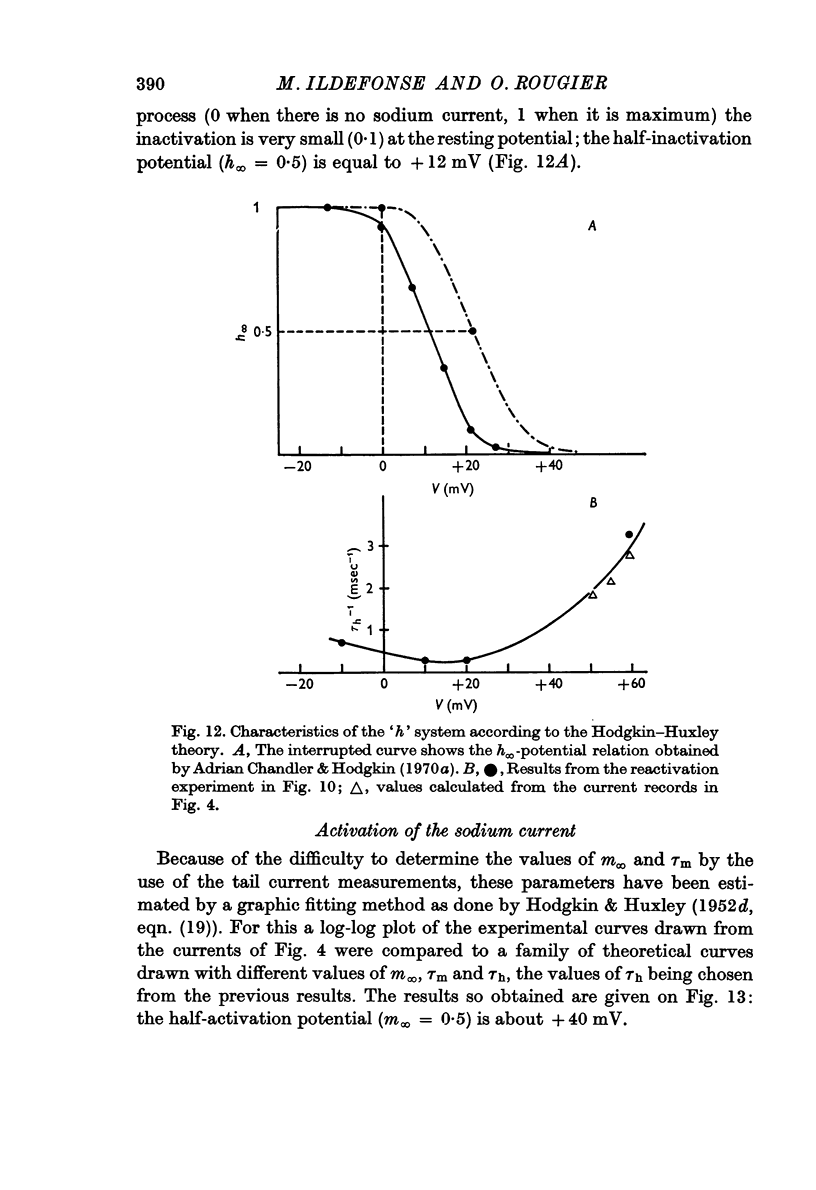
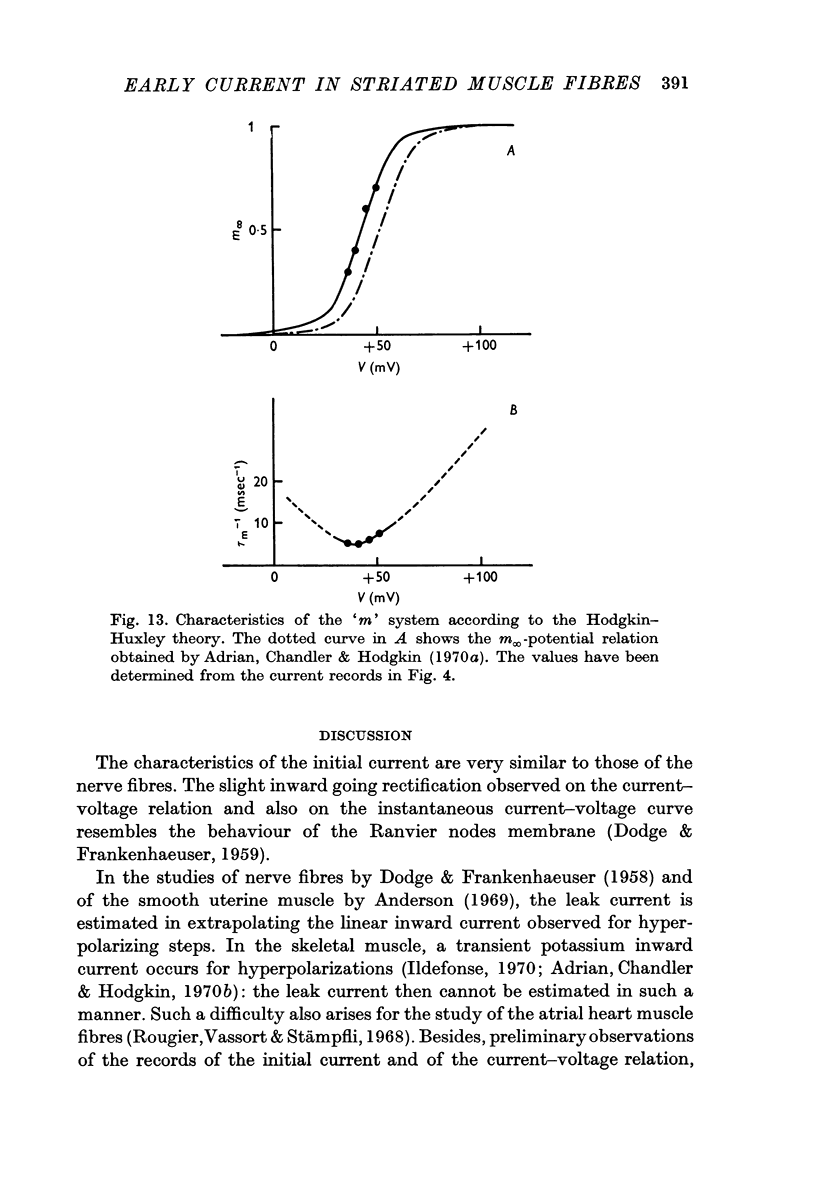
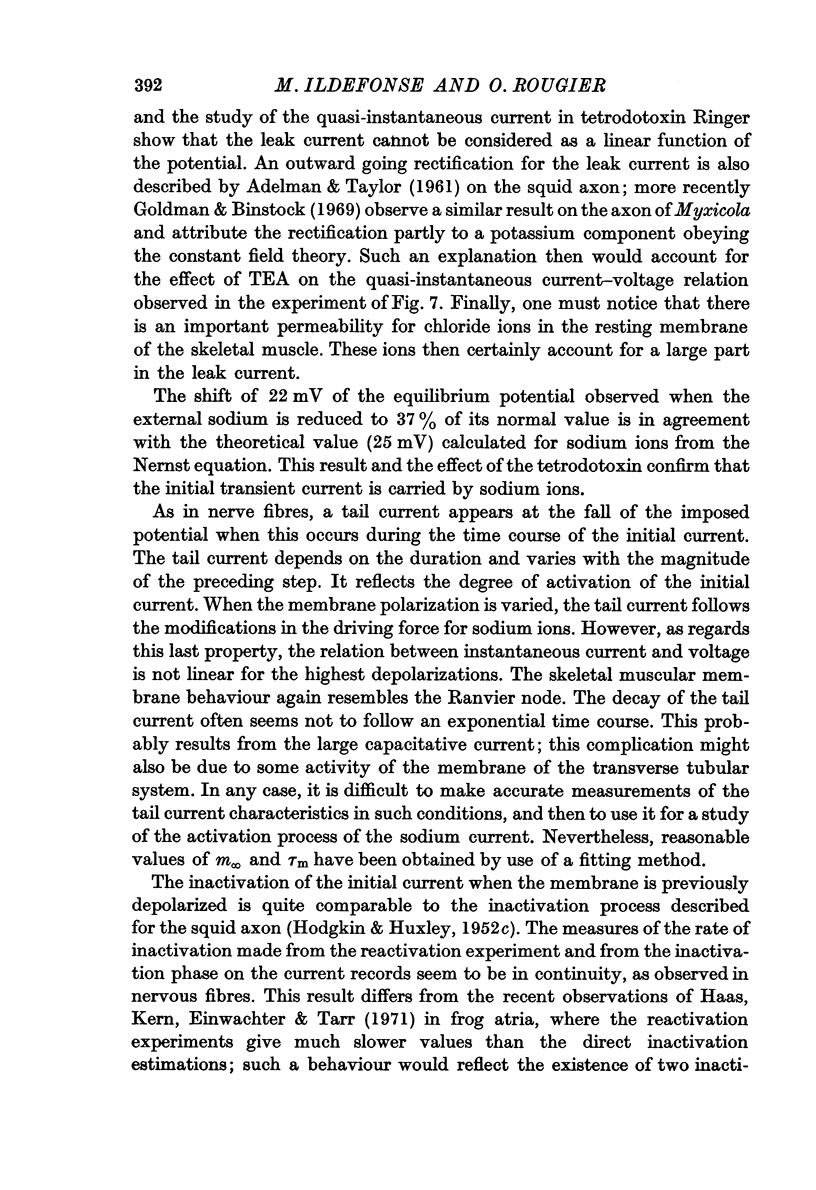
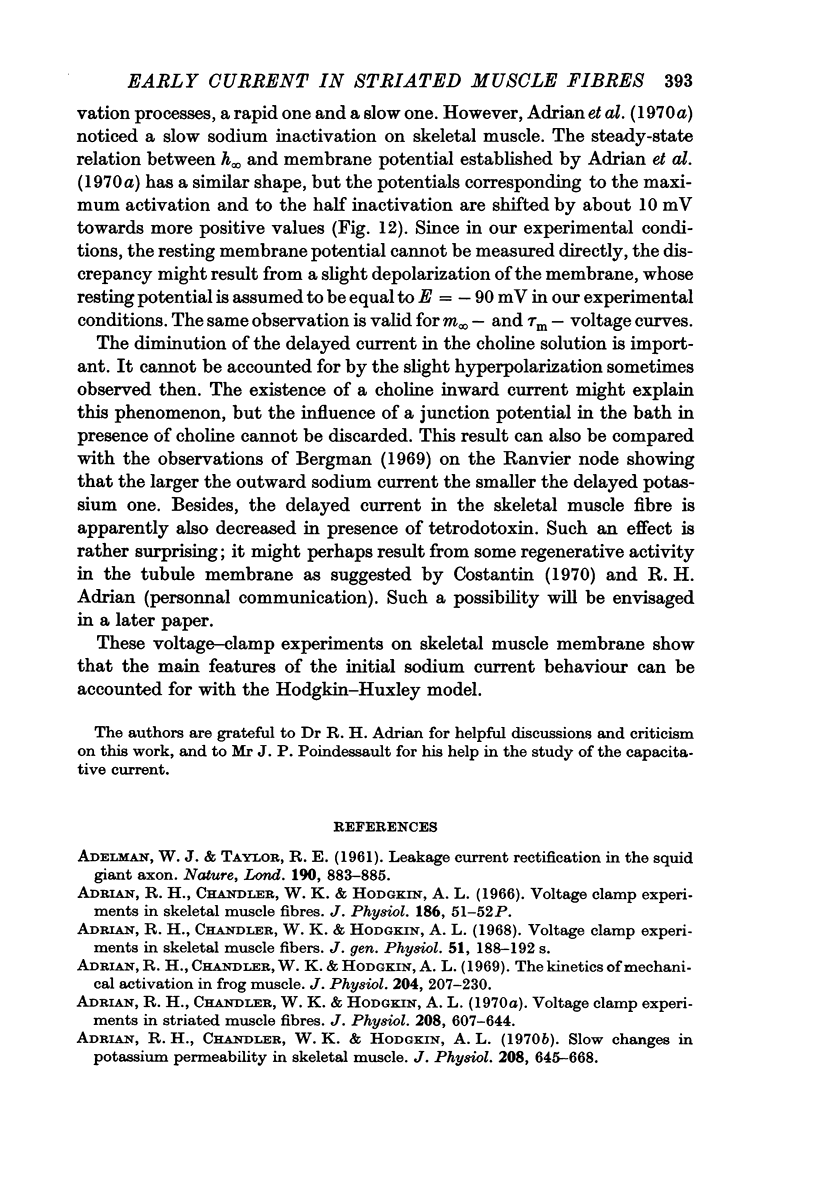
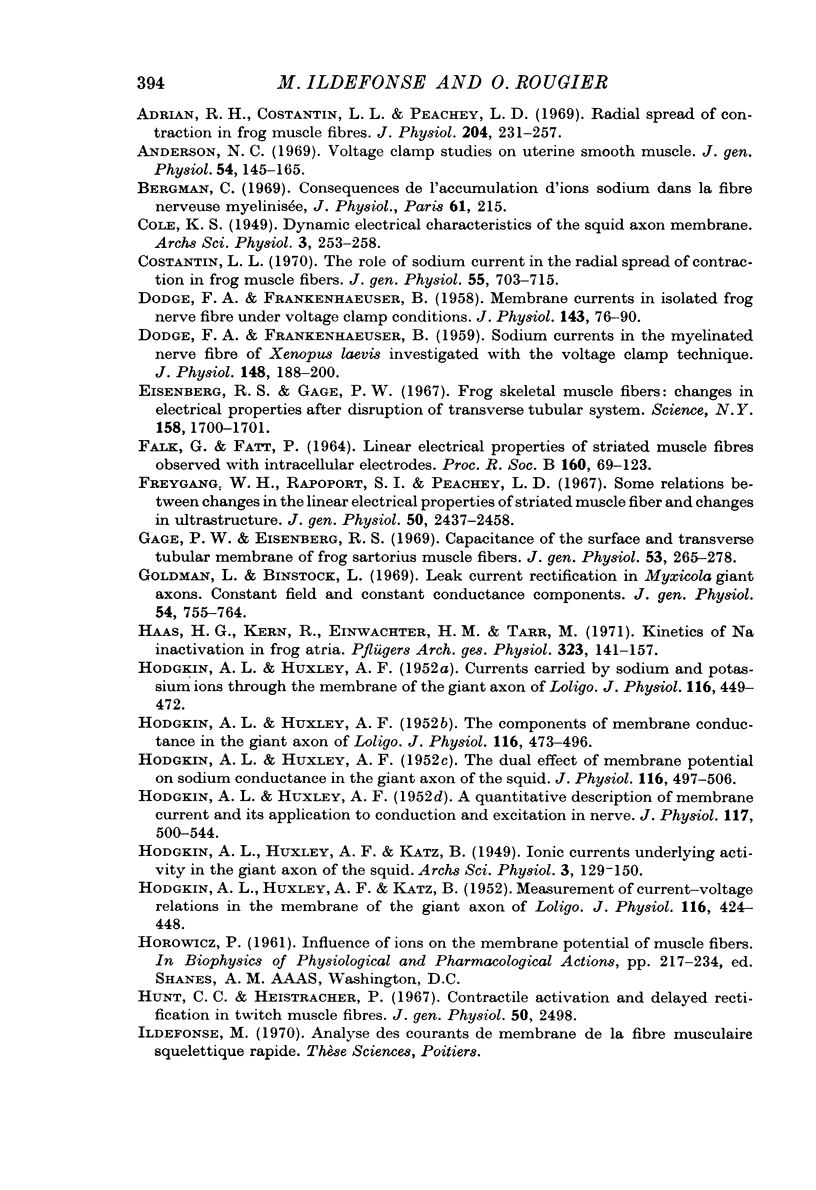
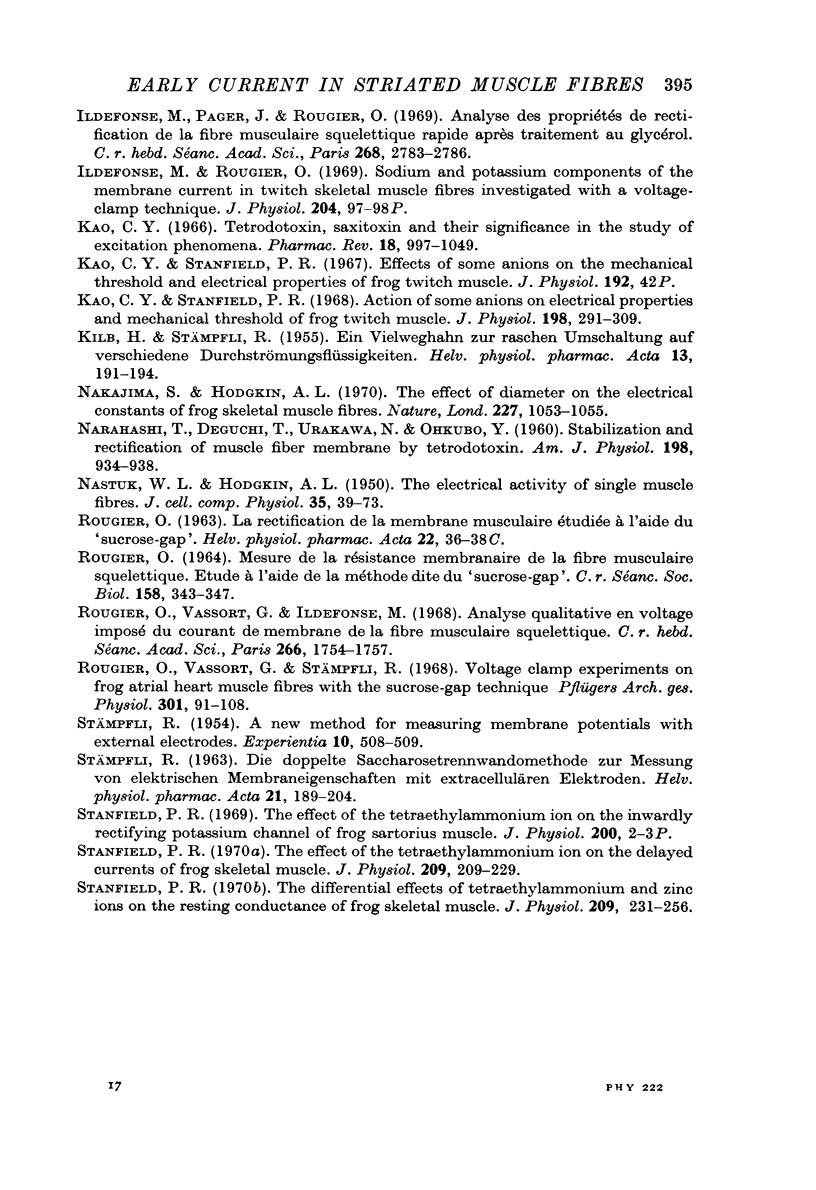
Selected References
These references are in PubMed. This may not be the complete list of references from this article.
- ADELMAN W. J., TAYLOR R. E. Leakage current rectification in the squid giant axon. Nature. 1961 Jun 3;190:883–885. doi: 10.1038/190883a0. [DOI] [PubMed] [Google Scholar]
- Adrian R. H., Chandler W. K., Hodgkin A. L. Slow changes in potassium permeability in skeletal muscle. J Physiol. 1970 Jul;208(3):645–668. doi: 10.1113/jphysiol.1970.sp009140. [DOI] [PMC free article] [PubMed] [Google Scholar]
- Adrian R. H., Chandler W. K., Hodgkin A. L. The kinetics of mechanical activation in frog muscle. J Physiol. 1969 Sep;204(1):207–230. doi: 10.1113/jphysiol.1969.sp008909. [DOI] [PMC free article] [PubMed] [Google Scholar]
- Adrian R. H., Chandler W. K., Hodgkin A. L. Voltage clamp experiments in skeletal muscle fibres. J Physiol. 1966 Oct;186(2):51P–52P. [PubMed] [Google Scholar]
- Adrian R. H., Chandler W. K., Hodgkin A. L. Voltage clamp experiments in striated muscle fibers. J Gen Physiol. 1968 May 1;51(5):188–192. [PMC free article] [PubMed] [Google Scholar]
- Adrian R. H., Chandler W. K., Hodgkin A. L. Voltage clamp experiments in striated muscle fibres. J Physiol. 1970 Jul;208(3):607–644. doi: 10.1113/jphysiol.1970.sp009139. [DOI] [PMC free article] [PubMed] [Google Scholar]
- Adrian R. H., Costantin L. L., Peachey L. D. Radial spread of contraction in frog muscle fibres. J Physiol. 1969 Sep;204(1):231–257. doi: 10.1113/jphysiol.1969.sp008910. [DOI] [PMC free article] [PubMed] [Google Scholar]
- Anderson N. C., Jr Voltage-clamp studies on uterine smooth muscle. J Gen Physiol. 1969 Aug;54(2):145–165. doi: 10.1085/jgp.54.2.145. [DOI] [PMC free article] [PubMed] [Google Scholar]
- Bergman C. Conséquences de l'accumulation d'ions sodium dans la fibre nerveuse myélinisée. J Physiol (Paris) 1969;61 (Suppl 2):215–215. [PubMed] [Google Scholar]
- Costantin L. L. The role of sodium current in the radial spread of contraction in frog muscle fibers. J Gen Physiol. 1970 Jun;55(6):703–715. doi: 10.1085/jgp.55.6.703. [DOI] [PMC free article] [PubMed] [Google Scholar]
- DODGE F. A., FRANKENHAEUSER B. Membrane currents in isolated frog nerve fibre under voltage clamp conditions. J Physiol. 1958 Aug 29;143(1):76–90. doi: 10.1113/jphysiol.1958.sp006045. [DOI] [PMC free article] [PubMed] [Google Scholar]
- DODGE F. A., FRANKENHAEUSER B. Sodium currents in the myelinated nerve fibre of Xenopus laevis investigated with the voltage clamp technique. J Physiol. 1959 Oct;148:188–200. doi: 10.1113/jphysiol.1959.sp006281. [DOI] [PMC free article] [PubMed] [Google Scholar]
- Eisenberg R. S., Gage P. W. Frog skeletal muscle fibers: changes in electrical properties after disruption of transverse tubular system. Science. 1967 Dec 29;158(3809):1700–1701. doi: 10.1126/science.158.3809.1700. [DOI] [PubMed] [Google Scholar]
- FALK G., FATT P. LINEAR ELECTRICAL PROPERTIES OF STRIATED MUSCLE FIBRES OBSERVED WITH INTRACELLULAR ELECTRODES. Proc R Soc Lond B Biol Sci. 1964 Apr 14;160:69–123. doi: 10.1098/rspb.1964.0030. [DOI] [PubMed] [Google Scholar]
- Freygang W. H., Jr, Rapoport S. I., Peachey L. D. Some relations between changes in the linear electrical properties of striated muscle fibers and changes in ultrastructure. J Gen Physiol. 1967 Nov;50(10):2437–2458. doi: 10.1085/jgp.50.10.2437. [DOI] [PMC free article] [PubMed] [Google Scholar]
- Gage P. W., Eisenberg R. S. Capacitance of the surface and transverse tubular membrane of frog sartorius muscle fibers. J Gen Physiol. 1969 Mar;53(3):265–278. doi: 10.1085/jgp.53.3.265. [DOI] [PMC free article] [PubMed] [Google Scholar]
- Goldman L., Binstock L. Leak current rectification in Myxicola giant axons. Constant field and constant conductance components. J Gen Physiol. 1969 Dec;54(6):755–764. doi: 10.1085/jgp.54.6.755. [DOI] [PMC free article] [PubMed] [Google Scholar]
- HODGKIN A. L., HUXLEY A. F. Currents carried by sodium and potassium ions through the membrane of the giant axon of Loligo. J Physiol. 1952 Apr;116(4):449–472. doi: 10.1113/jphysiol.1952.sp004717. [DOI] [PMC free article] [PubMed] [Google Scholar]
- HODGKIN A. L., HUXLEY A. F., KATZ B. Measurement of current-voltage relations in the membrane of the giant axon of Loligo. J Physiol. 1952 Apr;116(4):424–448. doi: 10.1113/jphysiol.1952.sp004716. [DOI] [PMC free article] [PubMed] [Google Scholar]
- HODGKIN A. L., HUXLEY A. F. The components of membrane conductance in the giant axon of Loligo. J Physiol. 1952 Apr;116(4):473–496. doi: 10.1113/jphysiol.1952.sp004718. [DOI] [PMC free article] [PubMed] [Google Scholar]
- HODGKIN A. L., HUXLEY A. F. The dual effect of membrane potential on sodium conductance in the giant axon of Loligo. J Physiol. 1952 Apr;116(4):497–506. doi: 10.1113/jphysiol.1952.sp004719. [DOI] [PMC free article] [PubMed] [Google Scholar]
- Haas H. G., Kern R., Einwächter H. M., Tarr M. Kinetics of Na inactivation in frog atria. Pflugers Arch. 1971;323(2):141–157. doi: 10.1007/BF00586445. [DOI] [PubMed] [Google Scholar]
- Ildefonse M., Pager J., Rougier O. Analyse des propriétés de rectification de la fibre musculaire squelettique rapide après traitement au glycérol. C R Acad Sci Hebd Seances Acad Sci D. 1969 Jun 3;268(23):2783–2786. [PubMed] [Google Scholar]
- Ildefonse M., Rougier O. Sodium and potassium components of the membrane current in twitch skeletal muscle fibres investigated with a voltage-clamp technique. J Physiol. 1969 Oct;204(2):97P–98P. [PubMed] [Google Scholar]
- KILB H., STAMPFLI R. Ein Vielweghahn zur raschen Umschaltung auf verschiedene Durchströmungsflüssigkeiten. Helv Physiol Pharmacol Acta. 1955;13(3):191–194. [PubMed] [Google Scholar]
- Kao C. Y., Stanfield P. R. Actions of some anions on electrical properties and mechanical threshold of frog twitch muscle. J Physiol. 1968 Sep;198(2):291–309. doi: 10.1113/jphysiol.1968.sp008607. [DOI] [PMC free article] [PubMed] [Google Scholar]
- Kao C. Y. Tetrodotoxin, saxitoxin and their significance in the study of excitation phenomena. Pharmacol Rev. 1966 Jun;18(2):997–1049. [PubMed] [Google Scholar]
- NARAHASHI T., DEGUCHI T., URAKAWA N., OHKUBO Y. Stabilization and rectification of muscle fiber membrane by tetrodotoxin. Am J Physiol. 1960 May;198:934–938. doi: 10.1152/ajplegacy.1960.198.5.934. [DOI] [PubMed] [Google Scholar]
- Nakajima S., Hodgkin A. L. Effect of diameter on the electrical constants of frog skeletal muscle fibres. Nature. 1970 Sep 5;227(5262):1053–1055. doi: 10.1038/2271053a0. [DOI] [PubMed] [Google Scholar]
- ROUGIER O. MESURE DE LA R'ESISTANCE MEMBRANAIRE DE LA FIBRE MUSCULAIRE SQUELETTIQUE. ETUDE 'A L'AIDE DE LA M'ETHODE DITE DU "SUCROSE GAP". C R Seances Soc Biol Fil. 1964;158:343–347. [PubMed] [Google Scholar]
- Rougier O., Vassort G., Ildefonse M. Analyse qualitative en voltage imposé du courant de membrane de la fibre musculaire squelettique. C R Acad Sci Hebd Seances Acad Sci D. 1968 Apr 22;266(17):1754–1757. [PubMed] [Google Scholar]
- Rougier O., Vassort G., Stämpfli R. Voltage clamp experiments on frog atrial heart muscle fibres with the sucrose gap technique. Pflugers Arch Gesamte Physiol Menschen Tiere. 1968;301(2):91–108. doi: 10.1007/BF00362729. [DOI] [PubMed] [Google Scholar]
- STAMPFLI R. A new method for measuring membrane potentials with external electrodes. Experientia. 1954 Dec 15;10(12):508–509. doi: 10.1007/BF02166189. [DOI] [PubMed] [Google Scholar]
- Stanfield P. R. The differential effects of tetraethylammonium and zinc ions on the resting conductance of frog skeletal muscle. J Physiol. 1970 Jul;209(1):231–256. doi: 10.1113/jphysiol.1970.sp009164. [DOI] [PMC free article] [PubMed] [Google Scholar]
- Stanfield P. R. The effect of the tetraethylammonium ion on the delayed currents of frog skeletal muscle. J Physiol. 1970 Jul;209(1):209–229. doi: 10.1113/jphysiol.1970.sp009163. [DOI] [PMC free article] [PubMed] [Google Scholar]
- Stanfield P. R. The effect of the tetraethylammonium ion on the inwardly rectifying potassium channel of frog sartorius muscle. J Physiol. 1969 Jan;200(1):2P–3P. [PubMed] [Google Scholar]